ABSTRACT
Production facilities serve to transform raw materials into products, usually with the goal of achieving a designated output, in terms of quantity and quality, with the minimum of cost and labour. With the aid of production planning and controlling (PPC) systems, raw material inputs, and labour can be planned to achieve a determined output of products. In general, the role of environmental and social aspects is neglected in production planning processes. Because of the growing pressure from politics and customers, sustainable production of products is becoming more important. One possibility for supporting sustainable manufacturing is, to integrate sustainable aspects in the production planning process. This paper presents input and output information for current PPC systems and discusses the need for additional information necessary for sustainable PPC. For this approach, a text review of cooperate social responsibility (CSR) reporting indicators provided by the GRI was performed. Based on the text review, an input-output model has been developed for conventional and another one for sustainable PPC systems. Through the comparison of the two input-output models, challenges and requirements for sustainable PPC systems have been defined as a basis for future work and analyses.
Introduction
The Brundtland Commission defined sustainability as ‘development that meets the needs of the present without compromising the ability of future generations to meet their own needs’ (WCED Citation1987). Moreover, the principal objectives of sustainability are bonded by the triple bottom line which balances economic goals, environmental cleanness, and social responsibility (Elkington Citation1997). Driven by the expectations of financial markets, most companies still consider the economic aspect as more important than the other two (Hauschild, Herrmann, and Kara Citation2017). Because of new laws and policies for sustainability in companies, the development of new technologies, processes, applications, and products considering also environmental and social aspects are becoming more essential for companies. For example, the United Nations (Citation2015) adopted a set of goals as part of a new sustainable agenda to end poverty, protect the planet and ensure prosperity for all. Each goal is complemented by specific targets to be achieved over the next 15 years. Goal number 12, in particular, has a special impact for enterprises: ‘Ensure sustainable consumption and production patterns’. According to this goal, companies are advised to increase net welfare gains from economic activities by reducing resource use and avoid social issues throughout the entire life cycle of processes and products.
Within their lifetime, products and processes pass through characteristic phases, such as design, production, use, and disposal. However, a product is sustainable only if it is manufactured in a sustainable way (Inoue et al. Citation2012). Through a systematic overview of required resources and caused emissions already during the production planning life-cycle phase, sustainable impacts (e.g. financial losses, environmental damages, social issues) can be identified and possibly avoided (Vorderwinkler and Helga Citation2011). In general, conventional production planning and controlling (PPC) systems consider resources such as material, labour, production capacity, and its respective costs, but neglect the role of sustainability aspects. Investigations for sustainable PPC processes are usually focused on partial aspects of sustainability (economic and environmental) and neglect the social aspect (Giret, Trentesaux, and Prabhu Citation2015; Gbededo, Liyanage, and Garza-Reyes Citation2018).
To evaluate the sustainable performance of an enterprise, several methods exist, such as Life Cycle Sustainable Assessment (LCSA) (Kloepffer Citation2008); Sustainable Performance Measurement Systems (PMS) (Schmidt et al. Citation2014; Vimal, Vinodh, and Muralidharan Citation2014); and Corporate Social Responsibility (CSR) Reporting (Dahlsrud Citation2008; Cavaco and Crifo Citation2014). For these methods, indicator frameworks which consider all three dimensions of sustainability (economic, social, and environmental) are still under development, and indicator frameworks considering different processes and product life-cycle phases are still limited. This is substantiated not only by the lack of data and experience (Bhanot, Rao, and Deshmukh Citation2017) but also by the high number of indicators provided by communities. Several indicators are available for the three sustainability dimensions, but guidance for the selection of applicable indicators for specific applications is missing (Neugebauer et al. Citation2015).
For the sustainability assessment of manufacturing systems, Steingrimsson, Heyer, and Seliger (Citation2012) presented the rapid sustainable plant assessment tool. This tool enables decision-maker in enterprises to obtain an indication on potentials for improvements. The focus is on reduce, reuse, recycle, remanufacturing and to use locally available resources and to a greater extent renewable energies. Moreover, Joung et al. (Citation2013) develop an indicator framework to evaluate the sustainable performances of manufacturing systems based on publicly available indicator sets from CSR reporting sources. The advantage of using CSR reporting indicators is that when enterprises have already integrate indicators for CSR reporting, the information is already available for the sustainable evaluation of processes and products. A similar study is proposed by Speziale and Klovienė (Citation2014). For the integration of sustainability indicators in production planning processes, Vorderwinkler and Helga (Citation2011) presented a simulation tool for production planning also considering environmental and social aspects. The simulation tool uses a set of rules for the creation of a production plan according to indicators for the efficiency of the resources as well as for the well-being of employees during manufacturing processes. An overview of applicable sustainability indicators and/or guides for the selection of applicable indicators for sustainable PPC processes are not presented.
To fill this gap, the paper presents input and output information for current PPC systems and shows the additional information necessary for sustainable PPC. For this approach, a literature review has been done to define sustainability and sustainable production, methods for developing an input-output model and available sources for sustainable indicators. For the selection of applicable indicator, sources for CSR reporting indicator were considered. A CSR report is an organisational report that provides information about sustainable performance. CSR reporting is not just report generation from collected data; instead, it is a method for internalising and improving an organisation’s commitment to sustainable development in a way that can be demonstrated to both internal and external stakeholders (Dahlsrud Citation2008). With the aid of the CSR reporting indicator, an input-output model has been developed for conventional and sustainable PPC systems. Through the comparison of input-output models for conventional and sustainable PPC, challenges and requirements for sustainable PPC systems have been defined as a basis for future work and analyses.
Following the introduction in Section 1, Section 2 discusses related work. Section 3 presents the results from the literature review to define sustainability, to present a method developing an input/output model, and sources for sustainability indicators. Section 4 presents the input-output model for conventional PPC systems. Based on this model, Section 5 presents possibilities for how the model can be extended with sustainable aspects. Section 6 contains the conclusion of the paper, followed by references.
Related work
The following section presents related works on sustainable production planning; indicators frameworks for sustainable manufacturing; and methods for selecting sustainable indicators for specific applications.
Sustainable PPC
In general, Giret, Trentesaux, and Prabhu (Citation2015) pointed out that previous studies for sustainable PPC are focused only on single aspects of sustainability, such as energy planning, waste management, and/or their respective costs, and environmental impacts. The social dimension of sustainability, in particular, has been neglected in previous studies (Gunasekaran and Subramanian Citation2018; Sutherland et al. Citation2016; Giret, Trentesaux, and Prabhu Citation2015)
Anyway, several studies exist which consider all three dimension of sustainability for specific PPC processes. In an early stage of production planning, the selection of the supplier of resources plays an important role. The selection of a supplier is one part of supply chain management and has recently garnered great attention among researchers (see literature review [Yawar and Seuring Citation2017]). Several tools and models have been developed for the selection process (Ghadimi, Dargi, and Heavey Citation2017; Azadnia, Saman, and Wong Citation2014; Nurjanni, Carvalho, and Costa Citation2017; Torabi and Hassini Citation2009; Boosothonsatit, Kara, and Ibbotson Citation2012), which select supplier according to sustainability criteria, such as costs, quality, delivery, CO2 emissions, worker safety and labour health, and locality.
For social aspects in the enterprises, several papers exist on the investigation of the social impacts of products and processes. The investigations are focused on developing frameworks of social indicators (Joung et al. Citation2013; Benoît, Mazijn, and Andrews Citation2009; Hutchins and Sutherland Citation2008; Weidema Citation2006; Husgafvel et al. Citation2014); connection of sustainable aspects with social aspects (Hanson Citation2013; Thomas Citation2010); and sustainability from an ergonomic perspective considering the workplace conditions (Martin, Legg, and Brown Citation2013; Imada Citation2008). Trost, Claus, and F. Herrmann (Citation2017) considered employees well-being in production planning processes and Sang-Oh et al. (Citation2017) integrates costumers stratification in PPC systems.
Several papers on environmental aspects of planning resources and environmental impacts exist in the literature. The investigations are focused on resource management (Kreimeier Citation2012; Torres et al. Citation2008); energy planning (Alasseri et al. Citation2017; Bossink Citation2017; May et al. Citation2016; Singh and Sharma Citation2017); energy efficient planning (Gahm et al. Citation2016; Biel and Glock Citation2016); emission reduction (Summerbell et al. Citation2017); water management (Chen et al. Citation2017); and waste management (Hashemi, Pourzamani, and Rahmani Samani Citation2014)
Indicator frameworks for sustainable manufacturing
To plan and control the production, indicators are required, which measure a specific aspect of a company (e.g. production costs; energy demand). There are several sources and methods for selecting indicators to measure the sustainable performances of products, processes, and companies.
Speziale and Klovienė (Citation2014) present results of scientific literature review comparing the available methods and indicators for sustainable PMS and CSR reporting. One outcome of the comparison is that the integration of financial and non-financial measures and indicators is quite similar in PMS and CSR reporting. The main aim in both fields is to qualify and quantify sustainable measures and indicators to evaluate internal and external processes of the enterprises and to use the information for sustainable decision-making.
Neugebauer et al. (Citation2015) and Chang et al. (Citation2017) presents a 'tiered approach', providing an indicator hierarchy and proposing a stepwise implementation concept for the LCSA of products and processes. The concept provides a comprehensive indicator review as a basis for sustainable assessment and comparison of products and processes.
Joung et al. (Citation2013) reviewed a set of publicly available indicator sets from CSR reporting sources. Based on this review, an indicator framework is presented for sustainable manufacturing, which can be used to evaluate the sustainable performance of manufacturing systems. Similar investigations are proposed by Balachandran et al. (Citation2011) for small and medium-sized enterprises.
Giret, Trentesaux, and Prabhu (Citation2015) reviewed investigations into sustainable PPC systems and introduce the relevant challenges and issues for enterprises. The authors review papers with a focus on the sustainable aspects of the economy and the environment and neglect the social aspect.
Methods for the selection of indicators for sustainable manufacturing
Several investigations use the method LCSA to evaluate the sustainability of the possible product and/or process alternatives in the design phase of the process and product life-cycle (Schau, Traverso, and Finkbeiner Citation2012; Traverso et al. Citation2012a; Neugebauer et al. Citation2015). LCSA compares products and processes, from selecting the raw materials through disposal under sustainable aspects (Kloepffer Citation2008). To results of the comparison, Traverso et al. (Citation2012b) present a sustainable dashboard as a suitable and manageable tool for comparing products in terms of their sustainability performance in an overall index; by individual environmental, economic and social factors; and by three groups of indicators.
Results of the literature review
The related work gives an overview of sustainable production planning, available sources and methods for selecting sustainable indicators, and methods presenting sustainable indicators. Current research on production planning is driven by fragmented interests and disciplines of sustainability. There is no comprehensive guidelines and indicator frameworks to select indicators for production planning considering sustainable aspects.
Theoretical background and methods
The following subsections describe theoretical background about sustainability and sustainability in production, the methods for developing an input-output model, and available sources for the selection of sustainability indicators.
Definition of sustainability and sustainability in production
To categorise indicators in terms of their relevance and importance to sustainable manufacturing, a clear understanding of sustainable manufacturing is needed.
As already mentioned in the introduction, the WCED (Citation1987) defines sustainability ‘as development which meets the needs of the present without compromising the ability of future generations to meet their own needs’. The organisation define that sustainable development must follow the economic, environmental, and social aspects at the same time. This leads to the concept of the triple bottom line presented in (Elkington Citation1997). J. Elkington considers sustainability as a fragmented three-dimensional relationship between social, environmental, and economic factors. New technologies, processes, and products must be compatible and in balance with all three dimensions.
Figure 1. Considering sustainable development as a fragmented relationship between economic, social, and environmental aspects (Elkington Citation1997).
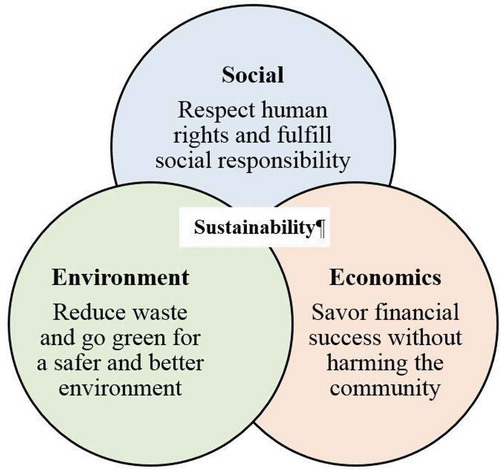
Driven by the expectations of financial markets, most companies still consider the economic bottom line as more important than the other two (Hauschild, Herrmann, and Kara Citation2017). Recent research focused on this imbalance of sustainability. Rockström et al. (Citation2009) criticised the fragmented perspective on sustainable development because, despite progress in reducing environmental impacts at the local level, cumulative global effects have increased in an uncontrolled way. Rockström suggests an integrated concept (see ), instead of the fragmented perspective. The defined concept reconnects economic human development with the environment. Such a shift necessitates a new aspect in which the economy is defined as the basis to achieve social and environmental goals.
Figure 2. Considering sustainable development as an integrated relationship between economics, social, and environment (Rockström Citation2015).
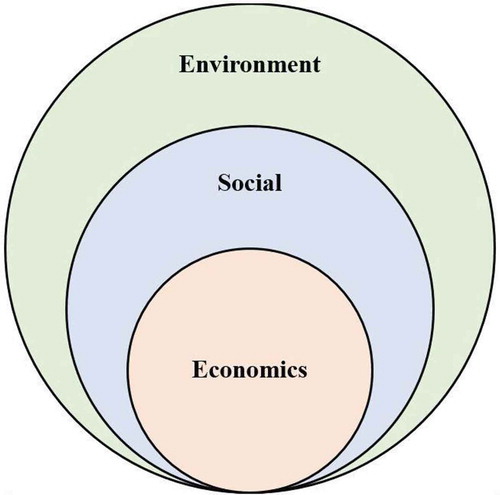
For sustainability in production, Straub (Citation2015) defines the sustainable aspects (economics, social, and environmental) as follows: The economic aspect in production means that a maximal number of products are produced with minimal use of resources to achieve minimal costs. This is the essential aspect because if production is not competitive, the enterprise will not survive in the future and cannot meet the other aspects. The social aspect in production moves human requirements into focus. This aspect considers the working conditions at the workplace for employees, such as ergonomics and requirements for customers, e.g. transparency about production processes. The environmental aspect considers the influence of the production on the environment, for example, the composition of the energy used (conventional energies vs. renewable energies) and the usage of recyclable materials in production. The definition of sustainability in production by Straub is also used in this paper.
Method developing an input-output model
To analyse the input and output streams of a system, the standard method of energy and material flow analysis (EMFA) can be used. The standard is described in ISO 14051. EMFA qualifies and quantifies using multiple scales, providing both research opportunities and rationale for policymaking. EMFA comprises a whole family of tools, such as industrial or urban metabolism; input-output analysis; economy-wide material flow accounting; socio-economic metabolism; and material flow cost accounting (Moreau and Massard Citation2017). In general, the target of these methods is to qualify and quantify the consumption of energy, raw material, water, the discharge of effluent, and labour required to transform materials into products (see examples: [Li et al. Citation2017; Blume et al. Citation2017; Torres et al. Citation2008]).
Because of its simplicity and method of presentation, this paper is focused on input-output analysis to qualify the input and output information for conventional and sustainable PPC systems. Input-output models are usually used to simplify real systems. The simplified model can be used to investigate the behaviour of the system in specific situations and to solve a research question. An input-output model consists of two basic elements: a system and input/output streams (Günther and Velten Citation2014).
No general guideline exists for developing an input-output model (Moreau and Massard Citation2017). To describe and investigate the behaviour of a system, Günther and Velten (Citation2014) defined the following general four steps:
System analysis;
Modelling;
Simulation;
Validation.
In step one, system analysis, all required input information to describe the behaviour of the considered system is collected. Based on the collected information from step one, a model (e.g. analytical, numerical, probabilistically) is modelled in step two. The model describes the relationship between the input and output information from the system. In step three, the model is transferred into a simulation. Based on the information collected in step one, the first experiments are carried out to present the real system. In step four, validation, the simulation results are tested in the real system to solve the research questions.
This paper focuses on the first step: system analysis. With the aid of an input-output model, the behaviour of the system production is described from a PPC perspective in an enterprise. The main research question is how a PPC must be modified to plan the production considering aspects of sustainability.
Selection of indicators for sustainable manufacturing
Indicators can be defined to describe the status of a system. The selection of the right indicators is a process that needs scientific and technical knowledge about how the system works and what informations are required (Rodrigues Citation2016). For this selection process, several sources exist for sustainability indicators, which can be used for the evaluation of sustainability performances of manufacturing systems. Joung et al. (Citation2013) provided an overview of the common list of indicators for the sustainable evaluation:
Global Report Initiative (GRI)
Dow Jones Sustainability Indexes (DJSI)
Environmental Sustainability Indicators (ESI)
Environment Performance Index (EPfI)
United Nations-Indicators of Sustainable Development (UN-CSD)
International Organization for Standardization (ISO) Environment Performance Evaluation (EPE) standard (ISO 14031)
Environmental Pressure Indicators for European Union (EPrI)
European Environmental Agency Core Set of Indicators (EEA-CSI)
The sets of indicators from ESI, EPfI, EPrI, EEA-CSI, DJSI, ISO 14031, and UN-CSD focus on the sustainable development of a region or country. The GRI standard is more focused on the process/product level for sustainable manufacturing (Joung et al. Citation2013). For PPC processes, indicators are needed which consider manufacturing systems. For this reason, this paper is focused on the indicators presented by GRI.
The GRI is a provider for CSR reporting indicator. The GRI is an international, independent organisation that helps businesses, governments, and other organisations understand and communicate the impact of business on critical sustainability issues such as climate change, human rights, corruption and many others. The published GRI standards for sustainability reporting are widely used in industry; in fact, 92% of the world’s largest 250 corporations report on their sustainability performance. The free available standard provides a list of indicators including definitions, requirements, and descriptions to use the indicator (GRI Citation2018).
Through a text review of the GRI standards, applicable indicators are identified for PPC processes according to sustainability aspects. A set of requirements has been defined for the selection of an applicable indicator for PPC processes:
The indicator must have a predictable quantitative impact on short and mid-term production planning and/or controlling.
The indicator must be relevant regarding sustainable manufacturing.
The indicator must be understandable and easy to interpret by the decision maker in the company.
The results of the analysis are presented as an input-output diagram and described more detailed in the text.
Input-output model for conventional PPC systems
In general, production facilities serve to transform raw materials into products, usually with the goal of achieving a designated output (in terms of quantity and quality) at minimum cost (Stark, Seliger, and Bonvoisin Citation2017). PPC systems have two main functions for this transformation process. First, with the aid of PPC systems, raw material input, labour, and costs are planned to achieve a determined output of products. Secondly, PPC systems control the order management from order acceptance to delivery of the products. For both functions, the data bill of materials (BOM) and production plans are necessary (Gronau Citation2014). BOM is a formal list of raw materials, which presents material inputs required to produce a specific product. Work plans describe the required production steps to produce a specific product. The description contains information on dependencies to other production steps (subsequent-steps and simultaneously steps); required machines and workstations; transport-time between two subsequent-steps; production times divided into waiting time, setup time, processing time, and clearing time; and required raw materials. (Wieneke and Schmidt Citation2012)
Based on this short description, shows an overview of basic indicators for conventional PPC systems, and presents the indicators in an input-output model. The descriptions of the indicators follow the standards provided by the GRI. Depending on the industry sector, products or business models, the importance of indicators can be varied.
Table 1. Indicators for conventional PPC systems.
The indicators for conventional PPC systems can be categorised into four groups followed by the GRI standard framework:
Costs
Materials
Labour
Products
The group of indicators ‘Costs’ considers the incurred and predictable costs from production. The following indicators are defined according to GRI 201: The total economic value is the sum of operation costs, employee wages, and benefits. Operation costs are cash payments for materials, product components, facilities, energy and services purchased. Services purchased includes payments to self-employed persons, temporary placement agencies and other organisations providing services (e.g. maintaining a machine). Employee wages and benefits are the total payroll (including employee salaries, employee taxes, levies, and unemployment funds) and total benefits (pensions, insurance, company vehicles, and health and safety).
The group of indicators ‘Materials’ considers the predictable material consumption from production. The following indicator is defined according to GRI 301: The total weight or volume of materials is the amount of materials that are used to produce and package the primary products produced. This includes raw materials, materials that are needed for the manufacturing process but are not part of the final product (e.g. lubricants for manufacturing machinery), and materials for packaging purposes (e.g. paper, cardboard and plastics).
The group of indicators ‘Labour’ considers the predictable worktime of employees in the production facility. For the following two indicators, no specific GRI standard exists. The following indicators are assumed according to GRI 403 and 404. The total time of working hours in the production defines the total time of employees working in production to produce products. The total number of employees in the production gives the number of employees working in production.
The group of indicators ‘Product’ considers the product output from production. For this group, no specific GRI standard exists. It is assumed that the indicator defined for materials inputs (GRI 301-1) can also be used to define the product output. The weight or volume of products is the outcome of products, which are produced in production with the input materials.
In addition to the indicators, contains the information stream ‘Production Task/Order/Time’. Through this information, it is possible to normalise the indicators. The input indicators for ‘Costs’ and ‘Materials’ can be expressed as material consumption or costs per production order, production tasks, product output, and time. The normalised indicator can be used to increase the efficiency of the production through, e.g. monitoring production, comparison of equal production orders, and for benchmarking processes.
Input-output model for sustainable PPC systems
This section presents additional indicators for the groups ‘Costs’, ‘Materials’, and ‘Labour’ from Section 4 and new groups of indicators for sustainable PPC systems provided by the GRI standards. provides an overview of additional indicators for sustainable PPC systems, and presents the indicators in an input-output model as an integrated relationship between economic, social, and environmental considerations. The descriptions of the indicators are organised according to their sustainable aspect and group of indicators. In addition to the descriptions of the indicator, requirements for PPC systems are presented.
Table 2. Indicators for sustainable PPC systems.
Figure 4. Input-output model for sustainable PPC systems as an integrated relationship between economic, social and environment.
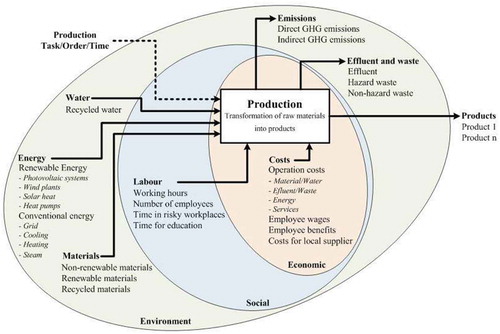
Sustainable aspect: economic
The group of indicators ‘Costs’ (see Section 4) is assigned to the sustainable aspect economic. The GRI proposed in GRI 204-1 to differentiate between costs for the local and non-local supplier. The indicator total economic value can be divided into costs for local and non-local suppliers. With this separation, the input indicator costs spent on local suppliers is calculated.
For these sustainable PPC systems, it is necessary to specify materials and services as local and non-local. For sustainable production planning, the PPC systems should prefer materials from local suppliers in the planning process. Supporting local suppliers can be a strategy to help ensure supply, support a stable local economy, and maintain community relations.
Sustainable aspect: social
The group of indicators ‘Labour’ is assigned to the sustainable aspect social. This group of indicators considers predictable working conditions in the workplace, such as risks for work accidents and effects on the health of employees. The indicators provided by the GRI consider social aspects such as human rights, customer privacy, and discrimination. Most of these indicators are not applicable to sustainable PPC systems according to the defined criteria in Section 3.3, because the indicators are only qualitatively measurable and not related. Only the following indicators defined according to GRI 403 and 404 are applicable:
The time of employees working in dangerous workplaces describes how many employees work in environments which influence the health of the employee, such as working in workspaces with toxic chemicals, but also shift work during night-times which impacts the social life of the employee. This includes working with hazardous materials, non-ergonomic workplaces, and working with heavy machines. The time of training for employees describes the time for training in terms of safety at the workplace, for example, and future training for the employees using current or new machines.
For these indicators, it is necessary to specify dangerous workplaces in the work plan and to specify hazardous materials in the BOM.
Sustainable aspect: environment
The following groups of indicators are assigned to the sustainable aspect environment:
Materials
Energy
Emissions
Water
Effluent and waste
For sustainable PPC systems, the group of indicators ‘Materials’ (see Section 4) considers the environmental impacts of the materials used in production. For this approach, the GRI provided the following indicators according to GRI 301-1: The raw materials are divided into weight or volume of non-renewable materials; weight or volume of renewable materials; and weight or volume of recycled input materials.
The group of indicators ‘Energy’ considers the energy consumption of production. The following indicators are defined according to GRI 302: The total energy consumption is the sum of the consumption of conventional and renewable energies. The consumption of conventional energies includes the energy demand from the electricity grid, cooling, heating, and steam. The consumption of renewable energies includes all energies, which are produced by renewable energy producers (e.g. photovoltaic systems, wind plants, solar heat and heat pumps.
The group of indicators ‘Emissions’ considers the emission of CO2 caused by energy consumption and is divided into direct and indirect emissions. The following indicators are defined according to GRI 305: Direct GHG emissions include the CO2 emissions from fuel consumption and can arise from the following sources owned or controlled by the enterprise: generation of electricity, heating-, cooling- and steam energy; chemical processes; and transportation of materials. Indirect GHG emissions include the CO2 emissions from the generation of purchased or acquired energy, such as electricity, heating, cooling, and steam consumed by a supplier. For many enterprises, the indirect GHG emissions that result from the generation of purchased electricity can be much greater than their direct GHG emissions.
The group of indicators ‘Water’ considers the water consumption used in/required for production. The following indicators are defined according to GRI 303: The total volume of water includes water withdrawn directly in production, such as cooling water. The volume of recycled water is the volume, which is reused in production; for example, production has a cycle that requires 20 m3 of water per cycle. It is possible to recycle the 20 m3 of water in an additional three cycles; then the total volume of water is 80 m3 and the recycled water for that process is 60 m3.
The group of indicators ‘Effluent and Waste’ considers the discharge of water and waste from production. The following indicators are defined according to GRI 306 for the discharge of water and waste:
The total volume of water discharge includes water effluent discharged to subsurface water, surface water, sewers that lead to rivers, oceans, lakes, wetlands, treatment facilities, and groundwater. The total weight of waste can be divided into hazardous and non-hazardous waste. Additionally, both can be divided into the following subcategories: reuse, recycling, and composting.
To plan the presented indicators in a PPC system, it is required to extend current BOM to include the resources energy and water, and the by-products emission, effluent, and waste. Additionally, it is necessary to classify the materials and energies into local/non-local supplier; conventional/renewable energy; and non-renewable/renewable/recycled materials. The new resources and by-products must also be connected to the work plans to plan production.
Conclusion
This paper presents an overview of additional information for sustainable PPC systems. Based on the provided indicator from the GRI for CSR reporting, a set of indicators is defined for sustainable PPC systems.
The economic aspect (production costs) is widely considered by conventional PPC systems. For sustainable production, supplier selection must be considered. For this approach, the GRI provides indicators to specify local and non-local suppliers.
In addition to economic impacts, social aspects, such as working conditions, health, and safety for employees must be considered in the planning phase. For this approach, the provided indicators by the GRI are limited. In the literature, no comprehensive guideline or applicable indicators exist for sustainable PPC systems. Integrating the working conditions as an indicator of production planning can be an interesting approach for future work.
For sustainable production planning considering environmental aspects, additional information is required. Resources must be classified as non-renewable, renewable, or recycled materials. Through the classification, it is possible to recognise the composition of the product output already in the planning phase, and adjustments can be made when renewable and recycled materials are preferred. In addition to the classification, new resources and by-products must be added to the BOMs: these include energy, water, emissions, and effluent and waste. Through this additional information, a comprehensive overview can be developed that considers the environmental aspects of production.
To plan production according to sustainable aspects, a uniform acquisition, and processing of the data is required. This assumes the use of information and communication technologies in the enterprises. The data must be assigned to machines, workplaces, products, production tasks, and production orders. For enterprises, it must individually be determined whether all presented indicators are required for planning processes. For example, when an enterprise uses no water for production, it makes no sense to consider water consumption and the effluent caused by the production.
The indicators provided by the GRI provide a basis for sustainable production planning under economic and environmental aspects. For social aspects, the indicators provided by the GRI are not applicable to sustainable PPC systems, because they are only qualitative measurable. The integration of social aspects in the economic and environment is not possible with the provided indicators. For example, the indicators for energy demand have a direct influence on the indicators for the production costs. A reduction in energy demand also reduces energy costs. The selection of suppliers for renewable materials has an influence on both cost indicators and environmental indicators. A link between social GRI indicators and economic/environment GRI indicators is not possible. To connect social indicators with economic and environmental indicators, new indicators and methods must be defined in the future work.
The results of the analysis can be used as the basis to support and improve the outcome of the PPC process discussions with company managers, experts and employees’ representatives. The integration of the sustainable aspects ensures that the sustainable PPC process is guided by principles of social responsibility (human well-being), green system design (environmental cleanness), and economic success (system performance). In the future work, surveys with decision makers in enterprises can be performed to investigate the relevance of the identified sustainable indicators related to, e.g. industry sector, kind of products. The most relevant indicators must be implemented in PPC systems to test the feasibility and practicability in manufacturing enterprises.
Disclosure statement
No potential conflict of interest was reported by the authors.
Additional information
Notes on contributors
Maximilian Zarte
Maximilian Zarte completed a Master of Engineering degree in ‘Sustainable Chemical Engineering and Biotechnology’. After his master’s degree, Maximilian started a job as a Research Assistant at the University of Applied Sciences Emden/Leer to work on different projects. One of these projects is the EU HORIZON 2020 project ‘PERFoRM’ (www.horizon2020-perform.eu), which searches and validates industrially accepted connectivity and re-configurability solutions. Another project is WeEmU (www.e-pps.de), which investigates the optimal design of a decentralized renewable energy system for supplying an industrial consumer, fitting its energy demand and profile within the restrictions of the German Renewable Energy Sources Act. In April, Maximilian started the PhD program in Industrial Engineering at the Universidae Nova de Lisboa. The proposed PhD topic is an intelligent fuzzy decision model for sustainable production planning and monitoring.
Agnes Pechmann
Prof. Dr.-Ing. Agnes Pechmann joined the Department of Mechanical Engineering at the University of Applied Sciences Emden-Leer and became Professor of Production Planning in 2008. She received the Doctorate degree from the University of Freiburg, Germany, in 2004. During her professorship, she founded the research group ‘Energy Efficiency in Production’. She has led several energy efficiency projects funded by German ministries (e-PPS, REN ProV and PREmdeK). There, one aspect is the increase of direct (local) use of renewable energies in production and the technical and economic feasibility of demand-side management by non-energy intensive production companies. Prof. Pechmann is a regular member of scientific conference committees and an evaluator of research proposals for various funding agencies.
Isabel L. Nunes
Prof. Dr.Isabel L. Nunes has a PhD in Industrial Engineering - Specialty in Human Factors/Ergonomics from the Universidade Nova de Lisboa, Portugal. Currently she is Vice Dean for Planning and Quality Management at Faculty of Science and Technology (FCT). She is Assistant Professor at the Mechanical and Industrial Engineering Department, where she teaches, in MSc and PhD programs of Industrial & Management Engineering, in the following courses: Systems Ergonomic Design; Occupational Safety and Health; Quality Management; Intelligent Systems for Decision Support; Entrepreneurship. She is member of the Research Centre UNIDEMI. Her main research interests include Ergonomics, Occupational Health & Safety and Quality (in Higher Education). She is author of scientific papers and book chapters, is a patent owner, and has been member of several Scientific Conference Committees. She is Chair of the Human Factors and Systems Interaction International Conference. She coordinated and participated in several national and international research projects and supervises PhD and MSc thesis.
References
- Alasseri, R., T. Ashish Tripathi, J. Rao, and K. J. Sreekanth. 2017. “A Review on Implementation Strategies for Demand Side Management (DSM) in Kuwait through Incentive-Based Demand Response Programs.” Renewable and Sustainable Energy Reviews 77: 617–635. doi:10.1016/j.rser.2017.04.023.
- Azadnia, A. H., M. Z. M. Saman, and K. Y. Wong. 2014. “Sustainable Supplier Selection and Order Lot-Sizing: An Integrated Multi-Objective Decision-Making Process.” International Journal of Production Research 53 (2): 383–408. doi:10.1080/00207543.2014.935827.
- Balachandran, K., A. R. Clarke‐Sather, M. J. Hutchins, J. K. G. Qiong Zhang, and J. W. Sutherland. 2011. “Development of Social, Environmental, and Economic Indicators for a Small/Medium Enterprise.” International Journal of Accounting and Information Management 19 (3): 247–266. doi:10.1108/18347641111169250.
- Benoît, C., B. Mazijn, and E. S. Andrews. 2009. Guidelines for Social Life Cycle Assessment of Products: Social and Socio-Economic LCA Guidelines Complementing Environmental LCA and Life Cycle Costing, Contributing to the Full Assessment of Goods and Services within the Context of Sustainable Development. Paris, France: United Nations Environment Programme.
- Bhanot, N. P., V. Rao, and S. G. Deshmukh. 2017. “An Integrated Approach for Analysing the Enablers and Barriers of Sustainable Manufacturing.” Journal of Cleaner Production 142: 4412–4439. doi:10.1016/j.jclepro.2016.11.123.
- Biel, K., and C. H. Glock. 2016. “Systematic Literature Review of Decision Support Models for Energy-Efficient Production Planning.” Computers & Industrial Engineering 101: 243–259. doi:10.1016/j.cie.2016.08.021.
- Blume, S., D. Kurle, C. Herrmann, and S. Thiede. 2017. “Toolbox for Increasing Resource Efficiency in the European Metal Mechanic Sector.” Procedia CIRP 61: 40–45. doi:10.1016/j.procir.2016.11.247.
- Boosothonsatit, K., S. Kara, and S. Ibbotson. 2012. “A Generic Simulation Model for Green Supplier Selection.” In 19th CIRP International Conference on Life Cycle Engineering, 587–592. doi:10.1007/978-3-642-29069-5_99.
- Bossink, B. A. G. 2017. “Demonstrating Sustainable Energy: A Review Based Model of Sustainable Energy Demonstration Projects.” Renewable and Sustainable Energy Reviews 77: 1349–1362. doi:10.1016/j.rser.2017.02.002.
- Cavaco, S., and P. Crifo. 2014. “CSR and Financial Performance: Complementarity between Environmental, Social and Business Behaviours.” Applied Economics 46 (27): 3323–3338. doi:10.1080/00036846.2014.927572.
- Chang, Y.-J., S. Neugebauer, A. Lehmann, R. Scheumann, and M. Finkbeiner. 2017. “Life Cycle Sustainability Assessment Approaches for Manufacturing.” Sustainable Manufacturing 221–237. doi:10.1007/978-3-319-48514-0_14.
- Chen, L., B. Shen, W. Xiongying, and X. Ding. 2017. “Development of a Cost-Effective Energy and Water Management System for Small and Medium-Sized Manufacturers.” Journal of Cleaner Production 153: 264–274. doi:10.1016/j.jclepro.2017.03.165.
- Dahlsrud, A. 2008. “How Corporate Social Responsibility Is Defined: An Analysis of 37 Definitions.” Corporate Social Responsibility and Environmental Management 15 (1): 1–13. doi:10.1002/csr.132.
- Elkington, J. 1997. Cannibals with Forks: The Triple Bottom Line of 21st Century Business. Oxford: Capstone.
- Gahm, C., F. Denz, M. Dirr, and A. Tuma. 2016. “Energy-Efficient Scheduling in Manufacturing Companies: A Review and Research Framework.” European Journal of Operational Research 248 (3): 744–757. doi:10.1016/j.ejor.2015.07.017.
- Gbededo, M. A., K. Liyanage, and J. A. Garza-Reyes. 2018. “Towards A Life Cycle Sustainability Analysis: A Systematic Review of Approaches to Sustainable Manufacturing.” Journal of Cleaner Production 184: 1002–1015. doi:10.1016/j.jclepro.2018.02.310.
- Ghadimi, P., A. Dargi, and C. Heavey. 2017. “Sustainable Supplier Performance Scoring Using Audition Check-List Based Fuzzy Inference System: A Case Application in Automotive Spare Part Industry.” Computers & Industrial Engineering 105: 12–27. doi:10.1016/j.cie.2017.01.002.
- Giret, A., D. Trentesaux, and V. Prabhu. 2015. “Sustainability in Manufacturing Operations Scheduling: A State of the Art Review.” Journal of Manufacturing Systems 37: 126–140. doi:10.1016/j.jmsy.2015.08.002.
- GRI. 2018. “GRI standards.” Accessed September 4 2018. https://www.globalreporting.org/standards
- Gronau, N. 2014. Enterprise Resource Planning: Architektur, Funktionen Und Management Von ERP-Systemen. 3. [überarbeitete] Auflage. Studium. München: De Gruyter Oldenbourg.
- Gunasekaran, A., and N. Subramanian. 2018. “Sustainable Operations Modeling and Data Analytics.” Computers & Operations Research 89: 163–167. doi:10.1016/j.cor.2017.09.009.
- Günther, M., and K. Velten. 2014. Mathematische Modellbildung und Simulation. Weinheim: Wiley-VCH.
- Hanson, M. A. 2013. “Green Ergonomics: Challenges and Opportunities.” Ergonomics 56 (3): 399–408. doi:10.1080/00140139.2012.751457.
- Hashemi, H., H. Pourzamani, and B. Rahmani Samani. 2014. “Comprehensive Planning for Classification and Disposal of Solid Waste at the Industrial Parks regarding Health and Environmental Impacts.” Journal of Environmental and Public Health 2014: 230163. doi:10.1155/2014/230163.
- Hauschild, M. Z., C. Herrmann, and S. Kara. 2017. “An Integrated Framework for Life Cycle Engineering.” Procedia CIRP 61: 2–9. doi:10.1016/j.procir.2016.11.257.
- Husgafvel, R., N. Pajunen, M. Päällysaho, I.-L. Paavola, V. Inkinen, K. Heiskanen, O. Dahl, and A. Ekroos. 2014. “Social Metrics in the Process Industry: Background, Theory and Development Work.” International Journal of Sustainable Engineering 7 (2): 171–182. doi:10.1080/19397038.2013.800166.
- Hutchins, M. J., and J. W. Sutherland. 2008. “An Exploration of Measures of Social Sustainability and Their Application to Supply Chain Decisions.” Journal of Cleaner Production 16 (15): 1688–1698. doi:10.1016/j.jclepro.2008.06.001.
- Imada, A. S. 2008. “Achieving Sustainability through Macroergonomic Change Management and Participation.” Corporate Sustainability as a Challenge for Comprehensive Management 129–138. doi:10.1007/978-3-7908-2046-1_8.
- Inoue, M., K. Lindow, R. Stark, K. Tanaka, Y.-E. Nahm, and H. Ishikawa. 2012. “Decision-Making Support for Sustainable Product Creation.” Advanced Engineering Informatics 26 (4): 782–792. doi:10.1016/j.aei.2012.07.002.
- Joung, C. B., J. Carrell, P. Sarkar, and S. C. Feng. 2013. “Categorization of Indicators for Sustainable Manufacturing.” Ecological Indicators 24: 148–157. doi:10.1016/j.ecolind.2012.05.030.
- Kloepffer, W. 2008. “Life Cycle Sustainability Assessment of Products.” The International Journal of Life Cycle Assessment 13 (2): 89–95. doi:10.1065/lca2008.02.376.
- Kreimeier, D., ed. 2012. Ressourcenorientierte Bewertung und Optimierung von Prozessketten. Frankfurt am Main: VDMA-Verl.
- Li, W., S. Thiede, S. Kara, and C. Herrmann. 2017. “A Generic Sankey Tool for Evaluating Energy Value Stream in Manufacturing Systems.” Procedia CIRP 61: 475–480. doi:10.1016/j.procir.2016.11.174.
- Martin, K., S. Legg, and C. Brown. 2013. “Designing for Sustainability: Ergonomics–Carpe Diem.” Ergonomics 56 (3): 365–388. doi:10.1080/00140139.2012.718368.
- May, G., B. Stahl, M. Taisch, and D. Kiritsis. 2016. “Energy Management in Manufacturing: From Literature Review to a Conceptual Framework.” Journal of Cleaner Production. doi:10.1016/j.jclepro.2016.10.191.
- Moreau, V., and G. Massard. 2017. “Material and Energy Flow Analysis.” Oxford University Press 1. doi:10.1093/acrefore/9780199389414.013.109.
- Neugebauer, S., J. Martinez-Blanco, R. Scheumann, and M. Finkbeiner. 2015. “Enhancing the Practical Implementation of Life Cycle Sustainability Assessment – Proposal of a Tiered Approach.” Journal of Cleaner Production 102: 165–176. doi:10.1016/j.jclepro.2015.04.053.
- Nurjanni, K. P., M. S. Carvalho, and L. Costa. 2017. “Green Supply Chain Design: A Mathematical Modeling Approach Based on A Multi-Objective Optimization Model.” International Journal of Production Economics 183: 421–432. doi:10.1016/j.ijpe.2016.08.028.
- Rockström, J. 2015. “Bounding the Planetary Future: Why We Need a Great Transition.” Great Transition Initiative.
- Rockström, J., W. Steffen, K. Noone, A. Persson, F. S. Chapin, E. F. Lambin, T. M. Lenton, et al. 2009. “A Safe Operating Space for Humanity.” Nature 461 (7263): 472–475. doi:10.1038/461472a.
- Rodrigues, P., and M. V. Bila. 2016. “Developing an Input-Output Model for Benchmarking Company Resource Usage.” Master Thesis, Universidade Nova de Lisboa.
- Sang-Oh, S., P. K. Bae, C. S. Yong, S.-O. Shim, K. Park, and S. Choi. 2017. “Innovative Production Scheduling with Customer Satisfaction Based Measurement for the Sustainability of Manufacturing Firms.” Sustainability 9 (12): 2249. doi:10.3390/su9122249.
- Schau, E. M., M. Traverso, and M. Finkbeiner. 2012. “Life Cycle Approach to Sustainability Assessment: A Case Study of Remanufactured Alternators.” Journal of Remanufacturing 2 (1): 5. doi:10.1186/2210-4690-2-5.
- Schmidt, I. A., M. B. Meurer, P. C. Saling, A. C. Kicherer, W. D. Reuter, and C.-O. D. Gensch. 2014. “SEEbalance ®: Managing Sustainability of Products and Processes with the Socio-Eco-Efficiency Analysis by BASF.” Greener Management International (45): 79–94. https://www.scopus.com/inward/record.uri?eid=2-s2.0-23844453064&partnerID=40&md5=71adb7cc73c61865f878635a602e27fd
- Singh, B., and J. Sharma. 2017. “A Review on Distributed Generation Planning.” Renewable and Sustainable Energy Reviews 76: 529–544. doi:10.1016/j.rser.2017.03.034.
- Speziale, M.-T., and L. Klovienė. 2014. “The Relationship between Performance Measurement and Sustainability Reporting: A Literature Review.” Procedia - Social and Behavioral Sciences 156: 633–638. doi:10.1016/j.sbspro.2014.11.254.
- Stark, R., G. Seliger, and J. Bonvoisin, eds. 2017. Sustainable Manufacturing: Challenges, Solutions and Implementation Perspectives. Cham, Switzerland. Springer.
- Steingrimsson, J. G., S. Heyer, and G. Seliger. 2012. “Rapid Sustainable Plant Assessment.” In Towards Implementing Sustainable Manufacturing: Proceedings, edited by G. Seliger and S. E. Kiliç, 588–593. Istanbul: CIRP.
- Straub, T. 2015. Einführung in die allgemeine Betriebswirtschaftslehre. 2. aktualisierte und erw. Aufl. wi - Wirtschaft. Hallbergmoos: Pearson.
- Summerbell, D. L., D. Khripko, C. Barlow, and J. Hesselbach. 2017. “Cost and Carbon Reductions from Industrial Demand-Side Management: Study of Potential Savings at a Cement Plant.” Applied Energy 197: 100–113. doi:10.1016/j.apenergy.2017.03.083.
- Sutherland, J. W., J. S. Richter, M. J. Hutchins, D. Dornfeld, R. Dzombak, J. Mangold, S. Robinson, et al. 2016. “The Role of Manufacturing in Affecting the Social Dimension of Sustainability.” CIRP Annals 65 (2): 689–712. doi:10.1016/j.cirp.2016.05.003.
- Thomas, L. E. 2010. “Evaluating Design Strategies, Performance and Occupant Satisfaction: A Low Carbon Office Refurbishment.” Building Research & Information 38 (6): 610–624. doi:10.1080/09613218.2010.501654.
- Torabi, S. A., and E. Hassini. 2009. “Multi-Site Production Planning Integrating Procurement and Distribution Plans in Multi-Echelon Supply Chains: An Interactive Fuzzy Goal Programming Approach.” International Journal of Production Research 47 (19): 5475–5499. doi:10.1080/00207540801905460.
- Torres, T. M., C. M. Barros, P. M. Bello, J. J. Casares, and M. J. Rodrı´guez-Blas. 2008. “Energy and Material Flow Analysis: Application to the Storage Stage of Clay in the Roof-Tile Manufacture.” Energy 33 (6): 963–973. doi:10.1016/j.energy.2007.09.008.
- Traverso, M., F. Asdrubali, A. Francia, and M. Finkbeiner. 2012a. “Towards Life Cycle Sustainability Assessment: An Implementation to Photovoltaic Modules.” The International Journal of Life Cycle Assessment 17 (8): 1068–1079. doi:10.1007/s11367-012-0433-8.
- Traverso, M., M. Finkbeiner, A. Jørgensen, and L. Schneider. 2012b. “Life Cycle Sustainability Dashboard.” Journal of Industrial Ecology 16 (5): 680–688. doi:10.1111/j.1530-9290.2012.00497.x.
- Trost, M., T. Claus, and F. Herrmann. 2017. “Master Production Scheduling and the Relevance of Included Social Criteria.” ACC 23 (2): 146–154. doi:10.15240/tul/004/2017-2-011.
- United Nations. 2015. “Sustainable development goals: 17 Goals to transform our world.” Accessed April 6 2017. http://www.un.org/sustainabledevelopment/sustainable-development-goals/
- Vimal, K. E. K., S. Vinodh, and R. Muralidharan. 2014. “An Approach for Evaluation of Process Sustainability Using Multi-Grade Fuzzy Method.” International Journal of Sustainable Engineering 8 (1): 40–54. doi:10.1080/19397038.2014.912254.
- Vorderwinkler, M., and H. Helga 2011. “Nachhaltige Produktionsregelung.” Bundesministerium für Verkehr, Innovation und Technologie 40. http://www.nachhaltigwirtschaften.at
- WCED. 1987. Report of the World Commission on Environment and Development: Our Common Future. Oxford, UK: Oxford University Press.
- Weidema, B. P. 2006. “The Integration of Economic and Social Aspects in Life Cycle Impact Assessment.” The International Journal of Life Cycle Assessment 11 (S1): 89–96. doi:10.1065/lca2006.04.016.
- Wieneke, F., and J. Schmidt. 2012. Produktionsmanagement. 4. Aufl. Bibliothek des technischen Wissens. Haan-Gruiten: Verl. Europa-Lehrmittel.
- Yawar, S. A., and S. Seuring. 2017. “Management of Social Issues in Supply Chains: A Literature Review Exploring Social Issues, Actions and Performance Outcomes.” Journal of Business Ethics : JBE 141 (3): 621–643. doi:10.1007/s10551-015-2719-9.