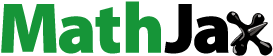
ABSTRACT
The growing attention to environmental sustainability is not reflected in the effective implementation of ecodesign principles in the industrial context. The main barriers are related to the propensity of companies to optimise only specific aspects directly affected by legislation and to the higher priority given to other drivers such as performance, cost and aesthetics. The present paper faces this issue by proposing an approach to support designers to concurrently deal with environmental, economic and technical performance in all the key phases of the product development process (selection of drivers, evaluation of design alternatives, preservation, sharing and reuse of knowledge, etc.). The approach is grounded on a multi-criteria index, called Product Impact Index (PII), which allows weighting different drivers, verifying the satisfaction of the company’s goals, and comparing different design solutions. The method has been successfully applied in two case studies to support the redesign of a cooker hood and a freestanding cooker. Results confirmed its usefulness and effectiveness in overcoming the common barriers to ecodesign implementation in the industrial context.
Introduction
During the last years, environmental sustainability has acquired a growing importance both in academia and policy worlds, stimulating the development of many different design methodologies and tools, and the emanation of several standards and regulations to promote its consideration inside industrial companies.
Among them, ecodesign represents one of the most promising approaches to integrate environmental aspects in the product development process (ISO Citation2011), through the application of strategies aimed at reducing the negative environmental impacts along the product life cycle. Due to the high number of methods and tools developed, several authors analysed, classified and collected them (Baumann, Boons, and Bragd Citation2002; Gómez-Navarro et al. Citation2005; McAloone and Pigosso Citation2017), while others reflected on the barriers that limit their real and effective implementation inside industrial companies (Bey, Hauschild, and McAloone Citation2013; Rossi, Germani, and Zamagni Citation2016). Recent initiatives at the European level, such as the Single Market for Green Products (European Commission Citation2013), are pushing the demand for green products, thus making the environment, not just a cost, but a competitive factor for all organizations. Moreover, several studies (van Birgelen, Semeijn, and Keicher Citation2009; Dono, Webb, and Richardson Citation2010; Schumacher Citation2010; Gadenne et al. Citation2011) demonstrate the increasing awareness of consumers who prefer to buy green products, even if they have a higher price. But usually, the implementation of ecodesign approaches is limited to the optimization of those life cycle phases affected by legislation (e.g. the material and use phase). Other constraints, such as performance, production cost, aesthetics and standardization (Bonvoisin Citation2017) have a higher priority and still limit the implementation of ecodesign inside industrial contexts. Furthermore, when companies decide to face the implementation of ecodesign approaches in their design departments, other specific barriers, directly related to tools and methods, arise. Among them: need for specific knowledge, time-consuming efforts for performing activities to promote sustainability (Ritzén Citation2000; van Hemel and Cramer Citation2002), scarcity of financial and human resources (Hillary Citation2004), over-formalization of methods and tools (Cross Citation2000; Tukker et al. Citation2001; Mathieux et al. Citation2002; Stempfle and Badke Schaub Citation2002; Blessing Citation2003). Moreover, companies rarely consider sustainability as a functional requirement, rather than just a design criterion, limiting the possibilities for radical environmental innovation (Deutz, McGuire, and Neighbour Citation2013).
Based on these issues, ecodesign tools should be:
able to carry out several but integrated analyses with life cycle perspective (Lindahl Citation2005);
linked to economic aspects to allow companies to consider also cost drivers (Schmidt Citation2002);
able to favour an efficient sharing and reusing of company knowledge (Hallstedt, Thompson, and Lindahl Citation2013);
customised on the company’s needs and able to support the definition of environmental objectives, checkpoints, reviews, milestones and roadmaps (Boks Citation2006).
Starting from these lacks, this paper proposes an integrated approach to foster ecodesign implementation in industrial companies. The method, customised on the company’s needs and easy to apply, supports the actors involved in the product development process during the implementation of ecodesign strategies. It allows the integration, during the design/redesign process, of environmental and economic sustainability, taking also into account product technical performance. The multi-criteria analysis is based on a unique indicator, called Product Impact Index (PII), which appears to be useful to compare alternative design alternatives, jointly considering different viewpoints. The index is expressed in Euro, which maximise the ease of interpretation by designers and the other involved stakeholders. Furthermore, the method foresees the creation of knowledge, supporting companies in sharing and collecting past experiences in a structured way.
The paper is organised as follows: After the Introduction, the State of the art and research context section presents a review of the scientific literature about the integration of ecodesign methods with other design tools and methods. The integrated approach section describes the proposed approach, based on the Product Impact Index, aiming to support the concurrent consideration of environmental, economic and performance drivers. The Approach testing section details the experimentation of the approach to redesign a domestic hood and a freestanding cooker. The Results discussion section discusses the obtained results to understand the main strengths and weaknesses of the approach. Finally, the Conclusions section reports conclusions and some proposals for future work.
State of the art and research context
Several ecodesign methods and tools are available in literature (Baumann, Boons, and Bragd Citation2002; Stevels Citation2007; Arana-Landin and Heras-Saizarbitoria Citation2011), and recently also aspects related to their implementation in industrial context, the so-called ecodesign management issue, are widely investigated (Pigosso Citation2012; Verhulst and Boks Citation2012; Pigosso et al. Citation2013). In the ecodesign context, the effective incorporation of environmental aspects in product development process plays a key role (Brones and de Carvalho Citation2015).
During last years, many methods aiming to integrate existing ecodesign tools have been developed as a possible answer to improve their usability and to favour their real and effective implementation by industries. Among them, Tingström and Karlsson (Citation2006) proposed a combined use of Environmental Effect Analysis (EEA) and Life Cycle Assessment (LCA) to facilitate the effective consideration of environmental aspects during the design process. Cappelli, Delogu, and Pierini (Citation2006) presented an integration of LCA, CAD and ecodesign guidelines. Yang and Chen (Citation2012) proposed an approach that integrates the Case Based Reasoning (CBR) and TRIZ methods, with Simplified LCA (S-LCA). Russo et al. (Citation2011) proposed an approach based on the integration of LCA and TRIZ eco-guidelines to foster the implementation of the ecodesign approach in European small and medium enterprises (SMEs). Sakao (Citation2007) proposed a design methodology to support environmentally conscious design of products by integrating LCA, QFDE (Quality Function Deployment for Environment), and TRIZ. MacDonald (Citation2005) proposed a strategic planning section for ISO 14001 integrated with the ‘back casting from principles’ method, with the objective to help companies in moving toward the effective implementation of sustainable strategies.
Enlarging the concept of tool integration, some authors proposed frameworks and related tool platforms for a wider consideration of environmental aspects. Some of them are related to a specific product life cycle phase (Um, Yoon, and Suh Citation2008), while other ones to the entire product life cycle (Khan, Natrajan, and Revathi Citation2001; Dufrene, Zwolinski, and Brissaud Citation2013; Bonou, Skelton, and Olsen Citation2016; Favi et al. Citation2018). What emerges is that both tools and platforms proposed in the literature are usually academic methods or approaches, focused on specific product or product categories. As a consequence, the application of these concepts in industrial practices remains difficult (Raluy, Serra, and Uche Citation2005; Bonton et al. Citation2012).
Other studies proposed integrated multi-objective ecodesign approaches (Guillen-Gosalbez and Grossmann Citation2010; Gerber, Gassner, and François Citation2011; Rerat, Papadokonstantakis, and Konrad Citation2013; Capon-Garcia et al. Citation2014; Ahmadi and Tiruta-Barna Citation2015), but they are often related to specific fields (e.g. chemical, energy, water and waste management). Popoff and Millet (Citation2017) proposed a method using QFD (Quality Function Deployment) optimisation to design an innovative inflatable dinghy. However, the authors recognise the high complexity of the model which requires a lot of work and computing time to be solved. High level of complexity can also be found in all those multi-objective optimisation methods available in the literature, where environmental sustainability is one of the parameters included in the analysis (Honda et al., Citation2013; Correia et al. Citation2017; Delgado et al. Citation2017; Kanbur et al. Citation2017). They require knowledge on specific software (e.g. MATLAB), high time and related costs. Therefore, they appear far from a fluid and easy implementation inside traditional company design departments.
In summary, when a company faces the implementation of ecodesign, some problems arise, from the high complexity of approaches to their limited integration with other design methods. Considering this situation, the present paper proposes an integrated approach, easy to be used and customised, which supports the effective implementation of ecodesign in industrial companies, allowing also the consideration of traditional drivers, as costs and technical aspects.
The integrated approach
The proposed approach aims to integrate different analyses into the traditional product development process, allowing designers to satisfy several criteria and be supported during redesign processes of manufacturing products, with a focus on the mechatronic sector. In particular, environmental impacts, technical performance and costs are the three product criteria considered by the Product Impact Index, which allows having a holistic view about the overall performance of a design alternative.
The approach has been developed involving two Italian mechatronic companies, which produce household appliances. Their traditional design process has been analysed and their typical redesign drivers have been investigated to develop an effective, but easy to implement approach. It consists of four steps, which are shown in and detailed in the following paragraphs.
Step 1: identification of company objectives
In this phase, the company’s management team identifies in a clear way what are the objective of the analysis, the targets to reach, and the drivers that will guide the project. The product development often addresses conflicting needs of different stakeholders and its drivers differ significantly between projects, companies, and even individuals. Based on these evidences, companies should clarify their product development drivers, understand how their importance is perceived and align them among the relevant stakeholders to enhance the decision-making and focus the product development efforts (Majava Citation2014). Moreover, driver’s prioritisation is essential to lead the product innovation because of the limited financial and other resources. The review of extant literature suggests that there is hardly any paper that addresses this issue (Mittal and Sangwan Citation2014).
For this aim, a simplified checklist able to support the management team in this activity has been defined (). It consists in a set of drivers classified according to a three-levels priority scale:
‘Mandatory’ requirements (red bullets), which are necessary to stay on the market (e.g. compliance to standards, market-access barriers);
‘Recommended’ requirements (yellow bullets), which are concrete opportunities to increase the company competitiveness from different points of view;
‘Optional’ requirements (green bullets), which enable the firm to initiate innovative competence to acquire competitive advantage or improve its image.
In order to create an effective checklist, the literature has been analysed to identify the main drivers and motivations that boost companies’ adoption of eco-innovation. Once identified, they have been validated by the two involved companies. From a general overview, regulations and market pull factors clearly dominate the rest of the factors (Hojnik and Ruzzier Citation2016). Other drivers have been identified, analysed and categorised starting from systematic literature reviews (Bossle et al. Citation2016; Pacheco et al. Citation2017; Peñasco, Del Río, and Desiderio Citation2017) and considering different perspectives, for instance, technology-push vs. market-pull, internal vs. external, reactive vs. preventive, etc. (Tariq et al. Citation2017).
For each of the main design drivers of the mechatronic sector, a possible correlation with the environmental, economic and technical performance of the product has been defined. The strength of the correlation is expressed according to the following three intensity levels:
High (3-value);
Medium (2-value);
Low (1-value).
Once selected the main innovation drivers, the management team can vary the relative priorities and correlations if necessary (e.g. compliance with ISO 14000 series is only related to the environmental performances) or use the default ones. In this way, the weight of each performance category can be calculated as the weighted sum of all contributions obtained from all selected drivers, according to Equation (1). It allows evaluating the capacity of the innovation to fulfil the desired objectives.
Step 2: product multi-objective analysis
Modular analysis
To help the design team to have a clear vision of the product a modular analysis could be performed. It allows subdividing the product into independent modules, which contains components that concur to realise a specific product function (Pahl et al. Citation2007). More the modules are independent of each other’s, more the product can be optimised with minimum impacts. Such preliminary analysis should enable the following analyses, allowing to focus the attention on the more critical modules.
Product impact index
This step represents the main core of the approach. Each analysis allows examining a specific aspect of the product and guiding its optimisation based on the identification of the main criticalities. It is conducted by the design team supported, if necessary, by an environmental expert able to realise environmental analyses and to correctly interpret results. In particular, the proposed approach foresees the integration of:
Environmental analysis;
Economic analysis;
Technical Performance analysis.
The results of these evaluations converge into the proposed PII, defined as in Equation (2):
where, Ienv, Ieco, and Iper are the Environmental, Economic and Technical Performance Indices, wenv, weco and wper the weights derived according to procedure described in Step 1, to be used for the environmental, economic and technical product impacts.
The PII is calculated, at first, for the reference product to understand its performances and/or criticalities, and then, iteratively, for all the successive redesigned versions to evaluate the possible achievable benefits and to identify the best design solution. The integration of several analyses’ results into a unique index permits to have a clear indication of product criticalities, easy to be managed and interpreted by company designers and managers. In the following paragraphs, details of each analysis are provided.
Environmental analysis
The product is analysed by a life cycle perspective in order to calculate the environmental impact of its modules and components. This analysis is based on the LCA methodology (ISO Citation2006), which allows quantifying the environmental load of a product along with all its life cycle phases. In case detailed data on product are not available, S-LCA methods and tools can be used (e.g. Solid Works Sustainability).
Based on Guenther, Saunders, and Tait (Citation2012), it appears that there is more familiarity among consumers with the concept of carbon footprint rather than other ones. Therefore, this approach foresees the assessment of the environmental impact through the use of the Global Warming indicator [kgCO2eq.], calculated by using the IPCC 2007 GWP 100a method. Successively, by using the damage characterisation conversion factor (8.32e-2 € per kgCO2eq.), defined in the impact assessment method ‘Stepwise2006ʹ (Weidema Citation2009), the Environmental Index (Ienv) expressed in Euro could be derived through the Equation (3):
Economic analysis
The economic analysis aims to determine the product ‘price’ based on the cost of raw material, manufacturing and other overheads (e.g. management costs, maintenance costs, recycling costs). It is a means to be used during the product design process, when the assessment of different cost items allows achieving a wider perspective and validly supporting managers’ decisions. Indeed, the final aim is creating alternative scenarios, evaluating the consumed resources and choosing the less-economic-impact solution (Castella et al. Citation2009). Currently, a standardised methodology for such analysis has not been defined yet and in literature many different viewpoints are adopted (Swarr et al. Citation2011; Peruzzini, Germani, and Marilungo Citation2013). In particular, the following items are considered in this analysis:
Raw materials;
Commercial components (e.g. sensors, fans, heat exchangers, etc.);
Capex, i.e. funds used by the company to acquire or upgrade physical assets such as machinery and equipment that are allocated to the product according to the production lot size and the amortisation period;
Manufacturing process (e.g. machining, assembly, quality control).
The Economic Index (Ieco) is derived as the sum of all the cost items, according to Equation (4):
Technical performance analysis
A check of the product technical and functional performance is needed to verify the global product conformity to specific parameters. The redesign process, in fact, foresees the modification of product characteristics to reach a specific objective (e.g. increase product recyclability, reduce the use phase impact). These modifications can potentially influence other product performances, which need to be checked and verified. In the case of mechatronic products for which specific directives are available (i.e. Energy-related Products – ErP), the Energy Efficiency Index (EEI) is the most common indicator. However, other performance indicators can be considered, such as insulation thermal conductivity, noise, fluid dynamic efficiency, time to boil. Another performance to evaluate may be the disassembly time of the product, an important factor that significantly influences its End of Life (EoL) behaviour (European Parliament and Council Citation2012; European Commission Citation2014b). As an example, some technical performance indicators and the relative test methods are shown in .
Table 1. Examples of technical performance indicators.
Such indicators can be considered as simple checks (e.g. emission limit for domestic gas burners that is COn <0.1) or determinants of the product value (e.g. A+++ instead of A++ for the EEI class). In the first case, they must be satisfied without affecting the final price of the product. In the second case, they could be monetised by the company market department to calculate the mark-up, expressed as a percentage of the product total cost [€]. This represents the Technical Performance Index (Iper).
Step 3: product redesign
Based on the key product criticalities identified in the previous step, and considering the company’s objectives and drivers, the possible redesign hypotheses are defined by the design team. The available methods to support designers in this phase are numerous: the well-known brainstorming (Osborn Citation1953), analogical thinking processes (e.g. Kim Citation2017), mapping methods (e.g. Jones and Bowes Citation2017), knowledge-based methods (e.g. Rossi et al. Citation2013). The influence of each design choice on the product overall performance is iteratively checked through the calculation of the PII. Thanks to the proposed index, the possible benefits can be quantified and the fulfilment of the company’s objective can be verified.
Step 4: long-term strategy definition
During the previous stages, the design team produced a huge quantity of information (e.g. analyses performed on different product alternatives, decisions taken on the basis of analyses results). These documents represent specific company knowledge that is important to preserve and to share, supporting and facilitating future projects. Knowledge is a crucial element for the survival of industrial organisations (Asrar-ul-Haq and Anwar Citation2016) and its sharing (i.e. its transference among individuals, groups, teams, and departments) represents a key element for their success (Crossan et al. Citation1999; Ipe Citation2003; Witherspoon et al. Citation2013). The reuse of knowledge is particularly useful for companies operating in mature domains, due to the high quality of produced knowledge, the similarity between different product generations and the possibility to dedicate more time to innovations (Baxter et al. Citation2008).
Step 4 aims to support the design team in the generation of structured knowledge, which can be stored, shared and reused in the proper way. The design team is invited to compile a quick and simple questionnaire () able to catalogue the most important documents and information generated during a project and to populate a dedicated repository. The proposed questionnaire foresees seven levels of information:
Keyword, in which some representative words are identified;
Type of Information, Status of information and Level of Information, in which general information related to the project are filled in to characterise it;
Source of information, in which information on the level of expertise of the user directly involved in the project are provided;
Classification of information and Description of information, in which specific data on the project, product involved, and results obtained are provided.
Table 2. Company knowledge questionnaire.
Approach testing
In order to validate the proposed approach, verify its usefulness within companies and quantify the benefits achievable, two industrial test cases have been carried out. The former faces the analysis of a cooker hood, produced and delivered by an Italian large company. The model selected is a standard low-price built-in hood. The simplicity of the product makes its redesign a hard challenge for engineers, which have few chances to improve it. The latter involves an Italian company that produces and distributes major household appliances. The product chosen for this study is a freestanding cooker. In the following paragraphs, steps realised, analyses performed, and results obtained by each company are presented.
Objectives definition
The first company would redesign the current product version in order to make the hood more sustainable in terms of recyclability and energy efficiency. This requirement derives from the observation of the target market that is rapidly increasing the attention to ecological issues.
Concerning the second product, from the analysis of cooker sector, it emerged that the innovation is pushed by the market pressure towards green products. Therefore, the main drivers behind the development of a new cooker are the improvement of performances, the reduction of energy consumptions and the reduction of the environmental impact. At the same time, nowadays, consumers are more demanding about the product versatility, leading the company to differentiate their products by offering more cooking programs and modalities. For this aim, patented technologies could be exploited by the company.
As shown in , different drivers (in bold) has been selected by the two companies. In some cases, the management teams adjusted the basic correlation level with the environmental, economic and technical performance (in bold and red) to better fit the company objectives.
Products multi-objective analysis and PII calculation
In both cases, designers and engineers have conducted the functional and modular analysis of the products, in order to identify the main modules ().
Table 3. Cooker hood and freestanding cooker modules.
Both environmental analyses have been carried out using the LCA Software SimaPro 7 and the database EcoInvent v2.2. For the economic analysis, the main cost items have been considered, such as raw materials, commercial components, manufacturing process. As far as the performance analysis is concerned, different parameters have been considered according to the product and the selected drivers. The results are summarised in the following paragraphs.
Cooker hood PII
Concerning the environmental analysis, the considered reference flow was a standard cooker hood, with a power of 128 W, with a lifetime of 8 years, exploiting energy for 7 h per week at the medium speed. The LCA boundaries included all the phases of product lifecycle (i.e. material extraction, production process, transportation, use, EoL). At the EoL, it was considered that the cooker hood is delivered to an authorised Waste Electrical and Electronic Equipment (WEEE) treatment center (European Parliament and Council Citation2012). Based on this, the disposal scenario has been modelled by considering the recycling of plastics, metals, etc. (IEC Citation2012), the shredding of non-recyclable materials and a dedicated treatment for specific components, such as the electronic devices.
The manufacturing costs related to material extraction, production processes and transportation were defined according to the available bill of materials.
The performances analysis has been conducted by evaluating the electric motor efficiency η (directly influencing the aspiration efficiency of the hood), the lighting efficiency, and the disassembly time and cost. The disassembly analysis has been carried out on each hood’s module using the Lean DfD approach (Favi et al. Citation2018). It is worth to notice that the disassembly cost is not in charge of the cooker hood producer, but the disassemblability significantly influences the product recyclability. For this reason, it was important to consider also this aspect in order to improve the product environmental performances related to the EoL phase.
Analysing the results obtained (), Aspiration and Lighting modules represent the most critical parts. These are the modules where the energy using components (motor and lamps) are located, thus they are responsible for the entire energy consumption. They contribute, respectively, to 74% and 27% of the total environmental impact and to 41% and 1% of production costs. This means that the design team should redesign these main modules’ components to make the final product cheaper and more environmental friendly. Concerning the Iper, its value has been set by the marketing department on the basis of the production cost (Ieco), considering a price mark-up of 75%, which can be considered an appropriate value for the low-end market. Finally, a PII of 37,30 € has been calculated by using equation (2), which represented the target to minimise during the redesign process.
Table 4. Cooker hood PII.
Freestanding cooker PII
The reference flow considered is a 60 × 60 freestanding cooker (four gas burners and a pyrolytic electric oven) with a lifetime of 10 years. The boundaries of the environmental analysis included the entire product lifecycle, from the raw material recruitment to the product dismantling. All materials, resources and processes were considered to model the manufacturing phase. Only components with a mass lower than 0.5 g and the transportation to the final user were neglected. For the use phase, the cooking cycles for each burner and for the oven, as well as the cleaning cycles, have been supposed. At the EoL, the same modelling hypotheses explained in the hood case study have been used.
Results () of the environmental analysis show a predominance of the impact related to the use phase because of the gas consumption during the use of the hob. Focusing on the manufacturing phase, the oven, the structure and the hob resulted in the less sustainable modules. EoL could be neglected considering the low impact (about 2% of the total impact).
Table 5. Freestanding cooker PII.
The results of the economic analysis show a higher impact of the following modules: Oven (27%), Oven door (26%), and Hob (19%).
As well-known, mechatronic products have a high impact especially during the use phase; this pushed the company to focus its efforts on product performance. Therefore, the performance analysis was executed by evaluating: (i) the efficiency of the burners (η), according to standard (CEN Citation2008) and internal procedures (e.g. time to boil calculation), (ii) the efficiency of the oven (European Commission Citation2014a), and (iii) the insulation performance. In this case, Iper was set to 168.36 €, which equals to a mark-up of 60% of production costs.
From the results obtained, it emerged that the efficiency of the hob could be improved and new cooking modalities for the oven could be studied, in order to reduce the value obtained for the PII (95.39 €).
Products redesign
Cooker hood redesign and final PII
Based on the results of the first analyses, designers decided to modify first of all the motor, by adopting an asynchronous single-phase motor instead of the original shaded-poles motor (+16% in terms of efficiency). Moreover, led lamps were adopted to improve also the lighting system. shows how these changes positively influenced the overall PII, with a reduction of 2,6 €. Despite an acceptable increase in costs (about +11%), they reduced the environmental impact and significantly increased technical performance (i.e. motor efficiency and lighting efficiency) associated to Aspiration and Lighting modules. Concerning the product disassemblability, the adopted components allowed maintaining the same disassembly times for both the electric motor and the lamps, since the mechanical liaisons and interfaces used to fix the new components with the hood assembly remained unchanged. Thanks to the improved technical performance also the cost mark-up was increased to +90% of the manufacturing costs.
Table 6. Redesigned cooker hood PII.
Freestanding cooker redesign and final PII
According to the outcomes of previous steps, the company efforts focused on new solutions to increase the efficiency of burners and the oven, satisfying consumers’ expectations. The redesign activities consisted in the study and optimisation of product thermo-fluid-dynamic, ergonomics and usability performance, as well as in the improvement of the control system. For this aim, the company involved heterogeneous resources on the project such as the product manager, the marketing manager and engineers of different technical departments (i.e. mechanical, electrical and automation). In particular, the stoichiometric fuel/air mixture was improved by developing a new typology of forced air burners equipped with a dedicated fan. In this way, the flame distribution resulted in flatter and allowed approaching the pan to the burner and increasing the efficiency. About the oven module, two innovations have been implemented:
The installation of a gas burner in the lower part of the oven muffle, which has to operate in combination with the electric components to improve the calorific value and the heat distribution. In this way, it was possible to reduce the pre-heating time, increasing cooking performance and offering to the user a new modality of cooking;
The substitution of the actual insulation (i.e. glass wool) with the Solimide® (i.e. polyimide foam certified as a fire-restricting material), which is more sustainable and able to ensure the required insulation performance.
As shown by results (), the new cooker is more efficient than the reference model. In particular, the insertion of a gas burner in the oven muffle allowed improving the energy class of the product from A to A+ and increasing the cooking functions. The new typology of hob burners allowed increasing their efficiency of approximately 3%. Such results, as well the identification of a new insulation, satisfied the company objectives and allowed them to obtain benefits in terms of both environmental and economic impacts. The final PII demonstrates the success of the redesign process.
Table 7. Redesigned freestanding cooker PII.
Long-term strategy definition
From the mentioned analyses, the two involved companies derived a set of generic and specific guidelines that could be exploited in future projects.
About the hood, the main results obtained refer to the analysis of different typologies of motors and their relative environmental and economic impacts. Also, different lighting systems were assessed in the same way. These analyses produced a historical dataset of information that is now consultable by different company departments.
The main guidelines concerning the freestanding cooker refer to the geometrical and functional characteristics of hob and oven burners, hob layout, fan settings and insulation proprieties. In particular, the results of the experimental tests have been stored in a structured database to share the acquired knowledge between the different technical departments and to exploit the transferability of the proposed solutions. For example, in the next future, the company will focus on redesigning the traditional gas hob by implementing a new typology of burners, thus designers will be supported by the results of the past project.
To ensure a proper storing, sharing and reusing of such knowledge both companies filled in the proposed questionnaire. shows an extract of the questionnaire filled for the freestanding cooker.
Results discussion
Both case studies highlight the usefulness of the proposed approach that allows translating results of different analyses in a unique indicator, simple to be interpreted. The consideration of several aspects related to sustainable development in the design/redesign process supports designers in developing an integrated design. In terms of overall product performance, the companies’ goals were fully achieved: the environmental and economic impacts of the two products have been reduced, increasing also their technical performances. At the end of the redesign phase, the PII values have been reduced of about 7% and 98%, respectively for the hood and the freestanding cooker ().
Table 8. Comparison among the original and the redesigned versions of the two analysed products.
The main strength of the proposed approach and design index is certainly their multi-criteria nature. The most important factors that influence the design process, such as environmental, economic and technical performance are integrated to identify the optimal solution. Standard approaches usually treat these aspects in a separate way, in different departments and at different steps of the design process, sometimes generating conflicted situations. The definition of the PII, based on product performance, as well as on weighed design drivers, allows overcoming such problem by suggesting the solution that simultaneously satisfies the most relevant company priorities. In the presented approach, the strategy and the real needs of the company are the core of the design process because they are taken into account to proper contextualise, interpret and elaborate all the results.
From results shown in , it emerges that if the three analyses (i.e. environmental, economic and technical performance) had been carried out separately, it would be very difficult to establish if the redesigned products were acceptable considering the company strategy. Indeed, both for the hood and the cooker, from the economic viewpoint an increase of impacts is observed, while the environmental and technical performance were significantly improved. The use of an integrated index allowed having a quantitative and univocal value to guide the decision-making process.
The implementation of the method in two different industrial realities also provided preliminary and qualitative feedbacks about the approach usability. At first, companies did not need to involve additional resources than in their traditional design process. Furthermore, the approach allowed carrying out a joint analysis, reducing the inefficiencies of the typical internal procedures which occur when different functional areas and stakeholders have conflicting needs. In the context of ecodesign methods and tools, this integration of environmental aspects with other traditional drivers (i.e. production cost and product performance) represents also an essential means to mitigate the common barriers that limit their diffusion in real design departments.
A limit of this work is due to the choice of energy-consuming products as test cases, for which the use phase is the most impacting from all the perspectives. As a consequence, the redesign process focused only on those modules responsible of the product operation (e.g. aspiration, hob, oven). The obtained results did not allow to fully appreciate the potentialities of the design index to identify the best trade-off between conflicting solutions because of the high preponderance of the use phase. For example, the development of a more efficient burner requires a more complex configuration that entails additional components and investments in the manufacturing process, therefore, an increase in costs. However, it could be neglected if compared to the reduction of the gas consumption and the relative environmental benefits observed in the use phase.
Another potential weak point related to the approach implementation is the need to apply the concurrent design paradigm, where different company stakeholders and areas strictly collaborates to reach the final objectives. On one hand, this can be viewed as an added value to improve the company workflows toward the internal process optimisation. However, on the other hand, this represents an additional complexity to manage and requires a deep ‘change of mentality’ and a long-term view, which is difficult to have in the industrial sector, especially in case of SMEs.
Conclusions
This paper presents an innovative structured approach, based on a multi-criteria index (i.e. PII), to jointly consider different aspects (environmental, economic and technical) during the entire product design/redesign process (from the selection of innovation drivers to the performance evaluation). The proposed approach can be viewed as an extension of the traditional Design for X approaches, which generally focus on the optimisation of single drivers (e.g. assembly, cost, and environment) and potentially lead to difficulties in finding trade-off solutions. The evaluation of the PII during design activities represents an effective feedback to verify if a design solution satisfies all the design constraints, to quantitatively compare different alternatives, and to guide the decision-making process toward the most suitable product improvement strategy. Moreover, the proposed approach leads to significant practical implications: (i) it allows overcoming the current barriers that limit the implementation of ecodesign principles and tools in the industrial context, and (ii) it positively contributes to the implementation of a participatory and concurrent approach, requiring the involvement of different company’s figures and departments.
The approach testing in two cases studies led to promising results. A significant reduction of PII values was obtained in both cases. The use of the proposed approach and index allowed the involved companies to fully satisfy the redesign objectives, configuring a new hood and a new cooker with reduced environmental impacts and higher performances, against an acceptable cost increase.
However, these case studies did not allow highlighting the real potentialities of the approach because of the selection of two energy-consuming products, where the use phase plays a preponderant role. Therefore, in the next future, the method should be applied to other products categories to better investigate potentialities and drawbacks. Moreover, this method currently requires a higher and more complex level of detail to perform the different analyses than in case of traditional design approaches. For this reason, the need to develop a tool that supports the method adoption emerged. By integrating the analyses in a single tool allows obtaining several benefits: (i) to increase the results accuracy by correlating inputs and outputs of several analyses, (ii) to automate the process and, consequently, reduce resources, time and costs to perform the analyses, and (iii) to carry out the simulation of several scenarios, easily compare their results, and select the best configuration.
Acknowledgments
This study has been developed in the context of the agreement “Study and development of methodologies and tools for the life cycle design and end of life management of products and processes” between Università Politecnica delle Marche and Università degli Studi della Tuscia.
Disclosure statement
No potential conflict of interest was reported by the authors.
Additional information
Notes on contributors
Marta Rossi
Marta Rossi, PhD research fellow in Design Tools and Methods for Industrial Engineering. Expertise: ecodesign, life cycle assessment, eco-sustainability.
Alessandra Papetti
Alessandra Papetti, PhD research fellow in Design Tools and Methods for Industrial Engineering. Expertise: Energy management, sustainable manufacturing, ecodesign.
Marco Marconi
Marco Marconi, PhD Researcher in Design Tools and Methods for Industrial Engineering. Expertise: eco-design, life cycle assessment, design for disassembly, tools and methods for end-of-life management, sustainable manufacturing.
Michele Germani
Michele Germani, PhD, full professor in Design Tools and Methods for Industrial Engineering. Expertise: Virtual Prototyping, configuration ecodesign, sustainable manufacturing.
References
- Ahmadi, A., and L. Tiruta-Barnaabc. 2015. “A Process Modelling-Life Cycle Assessment-MultiObjective Optimization Tool for the Eco-Design of Conventional Treatment Processes of Potable Water.” Journal of Cleaner Production 100: 116–125. doi:10.1016/j.jclepro.2015.03.045.
- Arana-Landin, G., and I. Heras-Saizarbitoria. 2011. “Paving the Way for the ISO 14006 Ecodesign Standard: An Exploratory Study in Spanish Companies.” Journal of Cleaner Production 19: 1007–1015. doi:10.1016/j.jclepro.2011.01.013.
- Ardente, F., O. Wolf, F. Mathieux, and D. Pennington. 2011. In-depth analysis of the measurement and verification approaches, identification of the possible gaps and recommendations. Deliverable 2 of the project “Integration of resource efficiency and waste management criteria in the implementing measures under the Ecodesign Directive” 1. July 2011. European Commission. Joint Research Centre. Institute for Environment and Sustainability, European Union, 2011.
- Asrar-ul-Haq, M., and S. Anwar. 2016. “A Systematic Review of Knowledge Management and Knowledge Sharing: Trends, Issues, and Challenges.” Cogent Business & Management 3: 1127744. doi:10.1080/23311975.2015.1127744.
- Baumann, H., F. Boons, and A. Bragd. 2002. “Mapping the Green Product Development Field: Engineering, Policy and Business Perspectives.” Journal of Cleaner Production 10: 409–425. doi:10.1016/S0959-6526(02)00015-X.
- Baxter, D., J. Gao, K. Case, H. Jenny, Y. Bob, S. Cochrane, and S. Dani. 2008. “A Framework to Integrate Design Knowledge Reuse and Requirements Management in Engineering Design.” Robotics and Computer-Integrated Manufacturing 24: 585–593. doi:10.1016/j.rcim.2007.07.010.
- Bey, N., M. Z. Hauschild, and T. C. McAloone. 2013. “Drivers and Barriers for Implementation of Environmental Strategies in Manufacturing Companies.” CIRP Annals 62 (1): 43–46. doi:10.1016/j.cirp.2013.03.001.
- Blessing, L. 2003. “What Is This Thing Called Design Research?” In Proceedings of 14th International Conference on Engineering Design, ICED ‘ 03, Stockholm, August 19–21.
- Boks, C. 2006. “The Soft Side of Ecodesign.” Journal of Cleaner Production 14 (15–16): 1346–1356. doi:10.1016/j.jclepro.2005.11.015.
- Bonou, A., K. Skelton, and S. I. Olsen. 2016. “Ecodesign Framework for Developing Wind Turbines.” Journal of Cleaner Production 126: 643–653. doi:10.1016/j.jclepro.2016.02.093.
- Bonton, A., C. Bouchard, B. Barbeau, and S. Jedrzejak. 2012. “Comparative Life Cycle Assessment of Water Treatment Plants.” Desalination 284: 42–54. doi:10.1016/j.desal.2011.08.035.
- Bonvoisin, J. 2017. “Limits of Ecodesign: The Case for Open Source Product Development.” International Journal of Sustainable Engineering 10: 198–206. doi:10.1080/19397038.2017.1317875.
- Bossle, M. B., M. D. de Barcellos, L. M. Vieira, and S. Loïc. 2016. “The Drivers for Adoption of Eco-Innovation.” Journal of Cleaner Production 113: 861–872. doi:10.1016/j.jclepro.2015.11.033.
- Brones, F., and M. M. de Carvalho. 2015. “From 50 to 1: Integrating Literature toward a Systemic Ecodesign Model.” Journal of Cleaner Production 96: 44–57. doi:10.1016/j.jclepro.2014.07.036.
- Capón-García, E., S. Papadokonstantakis, and H. Konrad. 2014. “Multi-Objective Optimization of Industrial Waste Management in Chemical Sites Coupled with Heat Integration Issues.” Computers & Chemical Engineering 62: 21–36. doi:10.1016/j.compchemeng.2013.11.012.
- Cappelli, F., M. Delogu, and M. Pierini. 2006. “Integration of LCA and EcoDesign Guideline in a Virtual Cad Framework.” Proceedings Of LCE2006: 185–188.
- Castella, P. S., I. Blanc, M. G. Ferrer, B. Ecabert, M. Wakeman, J.-A. Manson, D. Emery, S.-H. Han, J. Hong, and O. Jolliet. 2009. “Integrating Life Cycle Costs and Environmental Impacts of Composite Rail Car-Bodies for a Korean Train.” The International Journal of Life Cycle Assessment 14 (5): 429–442. doi:10.1007/s11367-009-0096-2.
- CEN. 2008. “Domestic Cooking Appliances Burning Gas - Part 1-1: Safety – General. EN 30-1-1:2008+A3:2013.” Bruselles: CEN.
- Correia, V. M., J. F. Franco, A. L. Aguillar Madeira, C. M. Araújo, and M. Soares. 2017. “Multiobjective Design Optimization of Laminated Composite Plates with Piezoelectric Layers.” Composite Structures 169: 10–20. doi:10.1016/j.compstruct.2016.09.052.
- Cross, N. 2000. Engineering Design Methods: Strategies for Product Design. Chichester, West Sussex: John Wiley & Sons, Litd.
- Crossan, M., M. Henry, W. Lane, and E. W. Roderick. 1999. “An Organizational Learning Framework: From Intuition to Institution.” Academy of Management Review 24: 522–537. doi:10.5465/amr.1999.2202135.
- Delgado, B. M., S. Cao, A. Hasan, and S. Kai. 2017. “Multiobjective Optimization for Lifecycle Cost, Carbon Dioxide Emissions and Exergy of Residential Heat and Electricity Prosumers.” Energy Conversion and Management 154: 455–469. doi:10.1016/j.enconman.2017.11.037.
- Deutz, P., M. McGuire, and G. Neighbour. 2013. “Eco-Design Practice in the Context of a Structured Design Process: An Interdisciplinary Empirical Study of UK Manufacturers.” Journal of Cleaner Production 39: 117–128. doi:10.1016/j.jclepro.2012.08.035.
- Dono, J., J. Webb, and B. Richardson. 2010. “The Relationship between Environmental Activism, Pro-Environmental Behaviour and Social Identity.” Journal of Environmental Psychology 30 (2): 178–186. doi:10.1016/j.jenvp.2009.11.006.
- Dufrene, M., P. Zwolinski, and D. Brissaud. 2013. “An Engineering Platform to Support a Practical Integrated Eco-Design Methodology.” CIRP Annals 62 (1): 131–134. doi:10.1016/j.cirp.2013.03.065.
- European Commission. 2010a. COMMISSION DELEGATED REGULATION (EU) No 1060/2010 of 28 September 2010 Supplementing Directive 2010/30/EU of the European Parliament and of the Council with Regard to Energy Labelling of Household Refrigerating Appliances.
- European Commission. 2010b. COMMISSION DELEGATED REGULATION (EU) No 1061/2010 of 28 September 2010 Supplementing Directive 2010/30/EU of the European Parliament and of the Council with Regard to Energy Labelling of Household Washing Machines.
- European Commission. 2013. Communication from the Commission to the European Parliament and the Council - Building the Single Market for Green Products.
- European Commission. 2014a. COMMISSION DELEGATED REGULATION (EU) No 65/2014 of 1 October 2013 Supplementing Directive 2010/30/EU of the European Parliament and of the Council with Regard to the Energy Labelling of Domestic Ovens and Range Hoods.
- European Commission. 2014b. Regulation N.66/2014 of 14 January 2014 Implementing Directive 2009/125/EC of the European Parliament and of the Council with Regard to Ecodesign Requirements for Domestic Ovens, Hobs and Range Hoods.
- European Parliament and Council. 2012. DIRECTIVE 2012/19/EU OF THE EUROPEAN PARLIAMENT AND OF THE COUNCIL OF 4 July 2012 on Waste Electrical AND Electronic Equipment (WEEE).
- Favi, C., M. Germani, M. Mandolini, and M. Marconi. 2018. “Implementation of a Software Platform to Support an Eco-Design Methodology within a Manufacturing Firm.” International Journal of Sustainable Engineering 11: 79–96. doi:10.1080/19397038.2018.1439121.
- Gadenne, D., B. Sharma, D. Kerr, and T. Smith. 2011. “The Influence of Consumers’ Environmental Beliefs and Attitudes on Energy Saving Behaviours.” Energy Policy 39 (12): 7684–7694. doi:10.1016/j.enpol.2011.09.002.
- Gerber, L., M. Gassner, and M. François. 2011. “Systematic Integration of LCA in Process Systems Design: Application to Combined Fuel and Electricity Production from Lignocellulosic Biomass.” Computers & Chemical Engineering 35 (7): 1265–1280. doi:10.1016/j.compchemeng.2010.11.012.
- Germani, M., M. Mandolini, M. Marconi, and M. Rossi. 2014. “An Approach to Analytically Evaluate the Product Disassemblability during the Design Process.” Procedia CIRP 21: 336–341. doi:10.1016/j.procir.2014.03.153.
- Gómez-Navarro, T., S. F. Capuz-Rizo, S. F. Capuz-Rizo, M. J. Bastante-Ceca, and D. Collado-Ruiz. 2005. “Ecodesign Function and Form. Classification of Ecodesign Tools according to Their Functional Aspects.” In Procedia ICED 2005, Melbourne.
- Guenther, M., C. M. Saunders, and P. Tait. 2012. “Carbon Labeling and Consumer Attitudes.” Carbon Management 3 (5): 445–455. doi:10.4155/cmt.12.50.
- Guillén-Gosálbez, G., and I. Grossmann. 2010. “A Global Optimization Strategy for the Environmentally Conscious Design of Chemical Supply Chains under Uncertainty in the Damage Assessment Model.” Computers & Chemical Engineering 34 (1): 42–58. doi:10.1016/j.compchemeng.2009.09.003.
- Hallstedt, S. I., A. W. Thompson, and P. Lindahl. 2013. “Key Elements for Implementing a Strategic Sustainability Perspective in the Product Innovation Process.” Journal of Cleaner Production 51: 277–288. doi:10.1016/j.jclepro.2013.01.043.
- Hillary, R. 2004. “Environmental Management Systems and the Smaller Enterprise.” Journal of Cleaner Production 12: 561–569. doi:10.1016/j.jclepro.2003.08.006.
- Hojnik, J., and M. Ruzzier. 2016. “What Drives Eco-Innovation? A Review of an Emerging Literature.” Environmental Innovation and Societal Transitions 19: 31–41. doi:10.1016/j.eist.2015.09.006.
- Honda, S., T. Igarashi, and Y. Narita. 2013. “Multi-Objective Optimization of Curvilinear Fiber Shapes for Laminated Composite Plates by Using NSGA-II.” Composites Part B: Engineering 45 (1): 1071–1078. doi:10.1016/j.compositesb.2012.07.056.
- IEC. 2012. Guidelines for End-Of-Life Information Provided by Manufacturers and Recyclers and for Recyclability Rate Calculation of Electrical and Electronic Equipment. IEC TR 62635: 2012. Geneva: IEC.
- Ipe, M. 2003. “Knowledge Sharing in Organizations: A Conceptual Framework.” Human Resource Development Review 2: 337–359. doi:10.1177/1534484303257985.
- ISO. 2006. Environmental Management - Lifecycle Assessment: Principles and Framework. ISO 14040: 2006. Geneva: ISO.
- ISO. 2011. Environmental Management Systems - Guidelines for Incorporating Ecodesign. ISO 14006: 2011. Geneva: ISO.
- Jones, P., and J. Bowes. 2017. “Rendering Systems Visible for Design: Synthesis Maps as Constructivist Design Narratives.” She Ji: the Journal of Design, Economics, and Innovation 3: 229–248.
- Kanbur, B. B., X. Liming, S. Dubey, C. F. Hoong, and F. Duan. 2017. “Multiobjective Thermodynamic and Environmental Optimization of the Small Scale LNG Cold Utilization System.” Energy Procedia 142: 997–1002. doi:10.1016/j.egypro.2017.12.345.
- Khan, F. B., R. Natrajan, and P. Revathi. 2001. “GreenPro: A New Methodology for Cleaner and Greener Process Design.” Journal of Loss Prevention in the Process Industries 14 (4): 307–328. doi:10.1016/S0950-4230(00)00047-4.
- Kim, E. 2017. “Workshop Design for Enhancing the Appropriateness of Idea Generation Using Analogical Thinking.” International Journal of Innovation Studies 1: 134–143.
- Lindahl, M. 2005. “Designers’ Utilization of and Requirements on Design for Environment (Dfe) Methods and Tools.” Procedia 4th International Symposium on Environmentally Conscious Design and Inverse Manufacturing, Tokyo, Japan.
- MacDonald, J. P. 2005. “Strategic Sustainable Development Using the ISO 14001 Standard.” Journal of Cleaner Production 13 (6): 631–643. doi:10.1016/j.jclepro.2003.06.001.
- Majava, J. 2014. Product Development. Drivers, Stakeholders, and Customer Representation during Early Development. C, Technica.
- Mandolini, M., C. Favi, M. Germani, and M. Marconi. 2018. “Time-Based Disassembly Method: How to Assess the Best Disassembly Sequence and Time of Target Components in Complex Products.” The International Journal of Advanced Manufacturing Technology 95 (1–4): 409–430. doi:10.1007/s00170-017-1201-5.
- Mathieux, F., G. Rebitzer, S. Ferrendier, M. Simon, and D. mFroelich. 2002. “Implementation of Ecodesign in the European Electr(On)Ics Industry.” The Journal of Sustainable Product Design 1 (4): 233–245. doi:10.1023/A:1024670630297.
- McAloone, T. C., and D. C. A. Pigosso. 2017. “From Ecodesign to Sustainable Product/Service-Systems: A Journey through Research Contributions over Recent Decades.” In Sustainable Manufacturing. Sustainable Production, Life Cycle Engineering and Management, edited by R. Stark, G. Seliger, and J. Bonvoisin, 99–111. Cham: Springer, doi: https://doi.org/10.1007/978-3-319-48514-0_7
- Mittal, V. K., and K. S. Sangwan. 2014. “Prioritizing Drivers for Green Manufacturing: Environmental, Social and Economic Perspectives.” Procedia CIRP 15: 135–140. doi:10.1016/j.procir.2014.06.038.
- Osborn, A. 1953. Applied Imagination; Principles and Procedures of Creative Thinking. New York: Scribner.
- Pacheco, D., A. de Jesus, C. S. Ten Caten, C. F. Jung, D. José Luis, H. Ribeiro, V. G. Navas, and V. A. Cruz-Machado. 2017. “Eco-Innovation Determinants in Manufacturing SMEs: Systematic Review and Research Directions.” Journal of Cleaner Production 142: 2277–2287. doi:10.1016/j.jclepro.2016.11.049.
- Pahl, G., W. Beitz, J. Feldhusen, and K.-H. Grote. 2007. Engineering Design: A Systematic Approach. Springer. doi:10.1007/978-1-84628-319-2.
- Peñasco, C., P. Del Río, and R.-J. Desiderio. 2017. “Analysing the Role of International Drivers for Eco-Innovators.” Journal of International Management 23: 56–71. doi:10.1016/j.intman.2016.09.001.
- Peruzzini, M., M. Germani, and E. Marilungo. 2013. “A Sustainability Lifecycle Assessment of Products and Services for the Extended Enterprise Evolution.”In Product Lifecycle Management for Society. PLM 2013. IFIP Advances in Information and Communication Technology, vol 409, Edited by A. Bernard, L. Rivest, and D. Dutta, 100–109. Berlin: Springer., doi https://doi.org/10.1007/978-3-642-41501-2_11
- Pigosso, D., C. Antelmi, H. Rozenfeld, and T. C. McAloone. 2013. “Ecodesign Maturity Model: A Management Framework to Support Ecodesign Implementation into Manufacturing Companies.” Journal of Cleaner Production 59: 160–173. doi:10.1016/j.jclepro.2013.06.040.
- Pigosso, D. C. A. 2012. “Ecodesign Maturity Model: a Framework to Support Companies in the Selection and Implementation of Ecodesign Practices” PhD diss., Technical University of Denmark.
- Popoff, A., and D. Millet. 2017. “Sustainable Life Cycle Design Using Constraint Satisfaction Problems and Quality Function Deployment.” Procedia CIRP 61: 75–80. doi:10.1016/j.procir.2016.11.147.
- Raluy, R. G., L. Serra, and J. Uche. 2005. “Life Cycle Assessment of Desalination Technologies Integrated with Renewable Energies.” Desalination 183 (1–3): 81–93. doi:10.1016/j.desal.2005.04.023.
- Rerat, C., S. Papadokonstantakis, and H. Konrad. 2013. “Integrated Liquid Waste Management in Batch Chemical Industry Based on Multiobjective Optimization.” Journal of the Air & Waste Management Association 63: 349–366. doi:10.1080/10962247.2012.750252.
- Ritzén, S. 2000. “Integrating Environmental Aspects into Product Development – Proactive Measures” PhD diss., Royal Institute of Technology, Stockholm, Sweden.
- Rossi, M., M. Germani, M. Mandolini, M. Marconi, M. Mengoni, and A. Morbidoni. 2013. “Eco-Design Guidelines and Eco-Knowledge Integration in Product Development Process” Proceedings of 19th International Conference on Engineering Design, ICED ‘ 13, Seoul, August 19–22.
- Rossi, M., M. Germani, and A. Zamagni. 2016. “Review of Ecodesign Methods and Tools. Barriers and Strategies for an Effective Implementation in Industrial Companies.” Journal of Cleaner Production 129: 361–373. doi:10.1016/j.jclepro.2016.04.051.
- Russo, D., G. Bersano, V. Birolini, and R. Uhl. 2011. “European Testing of the Efficiency of TRIZ in Eco Innovation Projects for Manufacturing SMEs.” Procedia Engineering 9: 157–171. doi:10.1016/j.proeng.2011.03.109.
- Sakao, T. 2007. “A QFD-centred Design Methodology for Environmentally Conscious Product Design.” International Journal of Production Research 45: 4143–4162. doi:10.1080/00207540701450179.
- Schmidt, W. P., 2002. “LCAs in Eu Infringements so Far.” Paper presented at “Use of life cycle assessment in policy making in the context of Directive 94/62/EC, DG Environment/EUROPEAN Workshop, 2002-06-20, Brussels. doi:10.1044/1059-0889(2002/er01).
- Schumacher, I. 2010. “Ecolabeling, Consumers’ Preferences and Taxation.” Ecological Economics 69: 2202–2212. doi:10.1016/j.ecolecon.2010.06.005.
- Stempfle, J., and P. Badke-Schaub. 2002. “Thinking in Design Teams an Analysis of Team Communication.” Design Studies 23: 473–496. doi:10.1016/S0142-694X(02)00004-2.
- Stevels, A. L. N. 2007. Adventures in Ecodesign of Electronic Products E 1993e2007. The Netherlands: Delft University of Technology.
- Swarr, T. E., D. Hunkeler, W. Klöpffer, H.-L. Pesonen, A. Ciroth, A. C. Brent, and R. Pagan. 2011. “Environmental Life Cycle Costing: A Code of Practice.” The International Journal of Life Cycle Assessment 16 (5): 389–391. doi:10.1007/s11367-011-0287-5.
- Tariq, A., Y. F. Badir, W. Tariq, and U. S. Bhutta. 2017. “Drivers and Consequences of Green Product and Process Innovation: A Systematic Review, Conceptual Framework and Future Outlook.” Technology in Society 51: 8–23. doi:10.1016/j.techsoc.2017.06.002.
- Tingström, J., and R. Karlsson. 2006. “The Relationship between Environmental Analyses and the Dialogue Process in Product Development.” Journal of Cleaner Production 14: 1409–1419. doi:10.1016/j.jclepro.2005.11.012.
- Tukker, A., P. Eder, M. Charter, E. Haag, A. Vercalsteren, and T. Wiedmann. 2001. “Eco-Design: The State of Implementation in Europe - Conclusions of a State of the Art Study for IPTS.” The Journal of Sustainable Product Design 1 (3): 147–161. doi:10.1023/A:1020564820675.
- Um, J., J.-S. Yoon, and S.-H. Suh. 2008. “An Architecture Design with Data Model for Product Recovery Management Systems.” Resources, Conservation and Recycling 52 (10): 1175–1184. doi:10.1016/j.resconrec.2008.06.001.
- van Birgelen, M., J. Semeijn, and M. Keicher. 2009. “Packaging and Proenvironmental Consumption Behavior: Investigating Purchase and Disposal Decisions for Beverages.” Environment and Behavior 41 (1): 125–146. doi:10.1177/0013916507311140.
- van Hemel, C., and J. Cramer. 2002. “Barriers and Stimuli for Ecodesign in SMEs.” Journal of Cleaner Production 10 (5): 439–453. doi:10.1016/S0959-6526(02)00013-6.
- Verhulst, E., and C. Boks. 2012. “Bringing about Sustainable Change in Product Development: Theory Versus Practice.” In Advances in Production Management Systems. Value Networks: Innovation, Technologies, and Management. APMS 2011. IFIP Advances in Information and Communication Technology, edited by J. Frick, and B.T. Laugen vol 384. Berlin : Springer, 448–457 . doi https://doi.org/10.1007/978-3-642-33980-6_49
- Weidema, B. P. 2009. “Using the Budget Constraint to Monetarise Impact Assessment Results.” Ecological Economics 68 (6): 1591–1598. doi:10.1016/j.ecolecon.2008.01.019.
- Witherspoon, C. L., B. Jason, C. Cam, and N. S. Dan. 2013. “Antecedents of Organizational Knowledge Sharing: A Meta-Analysis and Critique.” Journal of Knowledge Management 17: 250–277. doi:10.1108/13673271311315204.
- Yang, C. J., and J. L. Chen. 2012. “Forecasting the Design of Eco Products by Integrating TRIZ Evolution Patterns with CBR and Simple LCA Methods.” Expert Systems with Applications 39 (3): 2884–2892. doi:10.1016/j.eswa.2011.08.150.