ABSTRACT
Modularity is one of the most useful tools employed in the product development process. Regarding functionality, the use of modules is common to generate flexible platforms to manufacture products and product families that require functional variations. In the current globalized market, the mass individualization or personalization is the preferred production model that delivers cost-effectiveness and satisfaction at the level of the market of one. In this model, the modularity is employed as a powerful concept applied not only for the manufacture but also for the use and final disposal stages, in which the design of modules provides functionalities and features that satisfy a variety of specifications for different market segments. Despite the existence of approaches in modularity and its usefulness in product development, it is possible to identify a lack of analysis of modular and open architecture to enhance the sustainability performance of products regarding strategies to diminish adverse impacts during their lifecycle. This paper provides an analysis of the influence and potential of Modular Architecture Principles – MAPs in the sustainable design of open architecture products. Additionally, lifecycle considerations are analysed to identify and propose strategies that enforce the sustainability performance of products concerning personalization from early design stages
Abbreviations: MAPs: Modular Architecture Principles; FMS: Flexible Manufacturing System; RMS: Reconfigurable Manufacturing System; EOL: End Of Life; LCA: Life Cycle Assessment; QFD: Quality Function Deployment; DFMA: Design For Manufacturing And Assembly
1. Introduction
Nowadays, sustainability is one of the most important considerations in the product design process. Designers and manufacturers are responsible for reducing the negative impacts derived from the manufacturing and further use and final disposal of products (Rusinko Citation2007) (Rizzi et al. Citation2012). Currently, the trend in the market is toward the complete personalization of products. This situation involves the increase of the number of product variants to satisfy a variety of customer in the market. This personalization decreases the volume of product variant but increases the number of product variants (Koren et al. Citation2013), requiring higher consumption of resources and emissions and waste generation in the material production and manufacturing stages. Therefore, the product design and the manufacturing processes should be addressed to minimize adverse impacts on the sustainability of products associated with the personalization model. Main approaches in the literature about product sustainable development are focused on single products and do not consider the product architecture and the implications during the use and final disposal stages. Additionally, the methods related to the enhancement of sustainability do not analyse the requirements associated with the marked product model; therefore, there is a lack of a methodological approach for the improvement of the sustainability of a product taking into account modularity and personalization in the market of one.
Modularity is considered a key concept in the product development in the current globalized market. The use of modularity, especially in the manufacturing stage, provides flexibility for the development of product variants using the same fixed manufacturing resources. Since the last decade, the use of this concept has been extended to all stages of the life cycle of products through the open architecture products, which employs modularity as a transversal tool across the product lifecycle to increase the encompass of many customer niches (Jian et al. Citation2016). Nevertheless, the modularity is included just as a whole strategy for adding, removing and exchanging functions. The approaches that include modularity as a sustainability tool do not consider the possible ways to modularize a product, the different architectures available, and their consequences respect to the overall sustainability performance of products.
This work provides highlights and strategies in the current and potential use of Modular Architecture Principles – MAPs in the design of products based on the personalization production model. In addition, five key strategies to enhance sustainability are proposed taking into account the usefulness of modularity in the open architecture products, which are considered the most competitiveness and cost-effective model to face the changing customer requirements and meet the personalization demanded in the globalized markets. Proposed strategies aim to increase the designer’s sensibility regarding the product architecture from early conceptual design stages.
The sections of this paper have been structured as follows: Section 2 describes the background related to product sustainability issues and the product architecture considerations in the current globalized era. Section 3 describes the MAPs that can be employed to modularize products, and their life cycle attributes including advantages and limitations. Strategies to include sustainability using modular principles are defined in Section 4. Future challenges related to the use of MAPs are included in section 5. Section 6 summarizes the conclusions of this work.
2. Overview
This section summarizes the importance of sustainability in the product development process, the existing relationship between product architecture and sustainability, the current issues concerning product sustainability and open architecture products and the most representative approaches to include sustainability from product architecture.
2.1 Sustainability in product development
The product development process comprises the idea generation, design, and materialization or manufacture of a product that satisfies a specific need within a market segment. These activities are common to all manufacturing companies, which transform raw material and resources in a useful product. This transformation from raw material to the final product is one of the most significant generators of negative impacts in the sustainability during a product development due to depletion of resources, pollution, emissions and consumption of energy among others.
According to some authors (Ramani et al. Citation2010) (Shrivastava and Berger Citation2010), the most important issues related to sustainability in the product development process are resources depletion, uncontrolled consumption of natural resources, high energy consumption, lack of formal supply chains between the final disposer and the manufacturer and waste generation during final disposal stage. Therefore, consideration of sustainability in the product development is currently one of the most important topics in design. The literature provides approaches focused on the enhancement of the manufacturing processes, and on the use and final disposal tasks concerning sustainability. However, the most critical intervention must take place during the design stage because once the product has been designed its sustainability attributes are fixed for further stages (Byggeth, Broman, and Robert Citation2007). It is important to clarify that in this context, supply chain is defined as the group of physical and information resources that facilitates the movement of products, components, material waste and raw material among different lifecycle stages.
2.2 Product architecture and sustainability
Nowadays, the product family development is a widely employed strategy to achieve competitiveness in the globalized market using product architecture models, which employ set of modules or functional elements that carry out specific operations and transformations that contribute to the overall performance of the product (Fiorineschi et al. Citation2015). The evolution of manufacturing systems and the market trends addressed the need of designing robust product platforms to reduce cost, manufacturing time, and customer complaints among others. Currently, the most competitive product architecture model is the open architecture, which is based on the use of commonality and modularization as powerful strategies for hogging the greatest number and variety of customers.
Two models characterize product architecture modularity: modular architecture and open architecture. The modular architecture is traditionally used when the market calls for mass customization. In this case, modular products are developed by adding, substituting or removing one or more functional components within a modular platform (Simpson Citation2004). In the case of hardware, the modular architecture model is implemented to facilitate customization during the manufacturing and assembly process only. On the other hand, software has a wider applicability due to the use of libraries and databases that can be articulated with application software interfaces. It is important to clarify that this article is focused only on modularity for hardware devices.
The open architecture is becoming the new tendency since this approach is focused on the personalization or mass individualization of products. In contrast with the modular architecture, in this case, products can be modified using modular components that can be added, substituted or removed at different stages of the life cycle (manufacturing, assembly, use, or end of life) (Koren et al. Citation2013). Both product architectures are focused on the user-centered design. However, the modular architecture is limited to predefined versions of the product depending on the capabilities of the of the flexible manufacturing system (FMS), while the open architecture is intended to create individualized products for single users or customers who prefer to have a customized and exclusive product. Open architecture is possible through the implementation of reconfigurable manufacturing systems (RMS) (Koren et al. Citation2015).
Both, modular and open architecture are based on modules or common units that provide specific functionalities or ranges of operation. Modular architecture commonly employs set of modules exclusively in the manufacturing stage, offering several options of product variants to face the different requirements from the market. Open architecture goes beyond and considers the use of modules by the user to create a personalized version of the product through his/her participation in the design. This architecture even considers customer personalisation during the use stage, facilitating modifications at function levels or the number of functions that the product can execute. shows the flow between manufacturing and final disposal for mass customisation (Modular Architecture) and personalisation (Open Architecture) models.
Figure 1. Modularization role in mass customization and personalization. Adapted from (Koren et al. Citation2015).
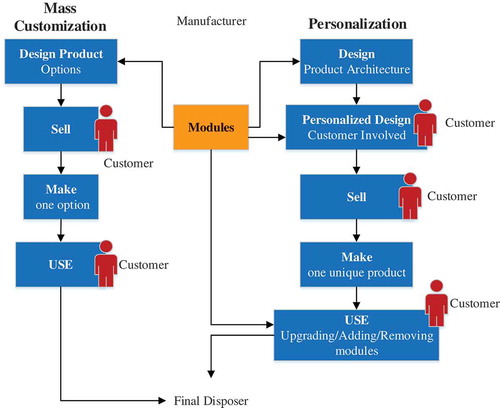
The previous figure shows the modules within the mass customization and personalisation models and the role of the customer to compare them. As it can be seen in , in the mass customization, users can select options for the product from existing designed modules. On the other hand, in the personalization, users can actively be involved in the personalized design. However, it is possible to differentiate the models described regarding other topics. summarizes the key differences between mass customization and personalization, taking into account issues associated with the goals and characteristics of both models.
Table 1. Key differences between, mass customization and mass personalization. Adapted from (Koren et al. Citation2015), (Hu et al. Citation2011) .
2.3 Sustainability issues in modular and open architecture models
Mass customization and personalization models imply the increase of product variety to reach more customers in differentiated market segments. This product variety entails the consumption of more resources (materials, energy, and others) taking into account the population growth and its influence on the rise of individual customers in the market of one. The increase of product variety implies the need for new product manufacturing platforms, tools, and machines among others if the manufacturing system is not designed to reconfigure itself according to the customer requirements. In that case, the use of RMS is a mandatory alternative for developing robust product platforms capable of using a set of resources to achieve competitiveness and a cost-benefit production. FMS can be employed when it is not required a great personalization, and it can be implemented in product development in long series that do not require significant variations among the family of components.
Sustainability impacts derived from the increase of product variety as a response to the population growth are focused in the process where it is required material transformation (material production, manufacturing, and final disposal). The main sustainability impacts identified from the product development process are listed in including tasks associated with the personalization and their sources.
Table 2. Sustainability impacts of personalization.
2.3 Approaches for enhancing sustainability in product development
According to the literature review, several existing methods focused on the enhancement of the sustainability performance of products. The most frequent topic approach used in the product development is the life cycle assessment – LCA, which is oriented to measure the impacts of the product in all lifecycle stages considering their relative importance on specific indicators selected previously. Some authors (Eddy et al. Citation2015), (He et al. Citation2015), (Cor and Zwolinski Citation2014), (Yung et al. Citation2012) have proposed different methods based on LCA to predict the impacts related to environmental, economic and social dimensions. Another approach is the Quality Function Deployment (QFD), which is related to the definition of the relative importance of requirements and design targets from the initial specification stage. This method, used by several authors (Romli et al. Citation2015) (Chang, Wang, and Wang Citation2013) (Tseng, Lin, and Han Citation2012), is also combined with LCA to clarify the need and relevance of requirements and product parameters related to the sustainability performance. Regarding functionality, it is possible to highlight approaches based on the design for manufacturing and assembly – DFMA (Giudice, Balisteri, and Risitano Citation2009) and specifically those related to modular systems. Several authors (Ma and Kremer Citation2016) (Koga and Aoyama Citation2008) (Umeda et al. Citation2008) (Yan and Feng Citation2014) propose methods to reduce the impact derived from the product development through the modification of the product architecture.
Other methods have been proposed taking into account particular lifecycle stages. In the manufacturing stage, it is possible to mention the concepts of lean manufacturing (Bhamu and Sangwan Citation2014), functional impact matrix and LCA (Devanathan et al. Citation2010), and atomic theory (Smith and Yen Citation2010) among others. Ma and Kremer (Ma and Kremer Citation2016) and Koga and Aoyama (Remery, Mascle, and Agard Citation2012) proposed approaches for the design of products taking into account the most suitable end-of-life considerations. Many authors have studied in detail the final disposal stage focusing on the reduction of waste, emissions and cost involved. Concepts of optimization models (Ameli, Mansour, and Ahmadi-Javid Citation2016), design for dismantling (Tian and Chen Citation2014), design for recycling (Subramaniyam, Srinivassan, and Prabaharan Citation2011) and impact assessment through LCA (Vinodh and Rathod Citation2010) can be mentioned as remarkable topics in this stage.
Two main alternatives in the final disposal stage according to the literature are the recycling and reusing of components and products. Recycling is associated with the transformation of used or damaged parts into raw material (Kriwet, Zussman, and Seliger Citation1995). On the other hand, reuse is defined as the re-integration of used components into the lifecycle of a new or existent product that allows continuing its use for the same purpose for which it was conceived (Stevels Citation1997). Concerning sustainability, it is common to employ the concept of reusability, which is defined as the ecologic, economic and social advantageousness of used parts compared to direct product recycling and disposal (Kissling et al. Citation2012). Despite all the methods and approaches founded in literature, just several works consider the modular products, and it is possible to identify a lack of sustainability studies regarding open architecture products. This issue involves the need of developing sustainability enhancement methods that consider the strong relationship between modules in the open architecture products taking into account the impacts of modularity on the manufacture, use, and final disposal.
As conclusions of this review section, it is possible to remark: a) personalization will trend to generate more variety of products; therefore, an effective modularization methodology is required to reduce adverse impacts on sustainability involved with the increase of variety. b) It is possible to identify many approaches focused on the improvement of sustainability in the product development process. Authors commonly analyse the consideration of manufacturing and final disposal stage. The existing strategies are strongly focused in the design stage, where the further impacts in functionality and sustainability should be reduced before starting the manufacturing processes. c) The approaches existent in the literature are focused mainly on single products and do not consider formally open architectures and the relationship between the modularization alternatives and their impact on the lifecycle stages.
3. MAPs in personalisation
Modular architecture principles (MAPs) are strategies to develop the structure of a product based on the needs in design, manufacturing, use and final disposal. According to Mesa et al. (Mesa et al. Citation2015), it is possible to identify 13 modular architecture principles in the literature review. Commonly, most authors refer to modularity as the use of exchangeable hardware sections, but this conception only covers a few of the architecture principles considered in this work. Therefore, the proposed approach is focused on these 13 types of modular architecture principles.
The MAPs can be employed to facilitate the adaptation concerning work ranges and the number of functions required By the type of product or family of products. A classification of the most representative MAPs is proposed in this work taking into account the functionality of each process. The proposed classification is oriented to generate different architecture alternatives for the consideration of the product designer team.
The classification of MAPs is described in detail below, and examples of each MAP are shown in the next section.
3.1 Classification of MAPs
The classification proposed in this paper is oriented to separate the type of MAP according to the main benefits and common uses; this approach was proposed by Mesa et al (Mesa et al. Citation2015). The aim of the proposed classification is to facilitate the identification, selection, and understanding of the MAPs during the early design of products or product families. The selection and implementation of these principles affect the remaining stages of the product lifecycle significantly. MAPs can be classified into two main groups: functional ranging MAPs and functional variety MAPs. In functional ranging MAPs, the products develop a unique function in different working levels; e.g. a set of motors with different horsepower performance (all the products have the same function but at different levels). On another hand, functional variety MAPs are associated with the development of various functionalities in a single specific range; e.g. a Swiss Army knife that can performance different tasks (blades, screwdriver, scissors, can opener, corkscrew, and others.) It is important to clarify that it is possible to combine the MAPs of the two categories described. However, those mixed principles are not considered in this work.
Functional ranging MAPs are related to the variation of a particular function or parameter in size or range. The principles of this category provide different ways to satisfy several levels of capability respect a particular function depending on the levels identified in the customers’ requirements. It is possible to select an adequate principle of functional ranging or to combine them to reach a particular stepping according to the case. The stepping can be by constant steps (stacking, cut to fit, size range), random sizes (cut to fit) and continuous (adjustment – that also can be used in discrete values). The benefits associated with the reach of different levels of work in functional ranging depend on the robust design of the standard interfaces and connectors, especially in stacking and cut to fit principles. describes the principles of this category.
Table 3. Functional ranging MAPs (Mesa et al. Citation2015).
Functional variety MAPs: in this category, the principles are related to the addition, substitution or removal of various functionalities in products. The change of dedicated modules with specific functions are allowed in both single (Component Sharing, Component Swapping) and several functionalities (Widening, Narrowing). This classification also considers the able of generating different configurations from a unique product (Sectional). summarizes the MAPs corresponding to this category.
Table 4. Functional variety MAPs (Mesa et al. Citation2015).
3.2 Examples of modular architecture principles
Instances of each MAP in conventional products are summarized in to illustrate the MAPs implementation, which also includes a brief description of the product functionality and the advantage provided by each principle.
Table 5. Examples of MAP implementation in products.
3.3 Open architecture considerations
The open architecture includes additional considerations related to the lifecycle stages. In this case, products are characterized by the use of common parts and the exchangeability of components during the use stage, and by design for an easy assembly/disassembly that provides the planning of final disposal for each module as independent, facilitating the product modification in the use stage. Even it can include the possibility of upgrading components (new modules associated with specific functions or levels) at the use lifecycle stage. Additionally, the open architecture enables the extension of the useful life of the whole product. This feature is possible because this approach allows designing products to achieve the operation range or the necessary functions using fewer components due to the sharing and swapping of modules through common interfaces. A comparison between the life cycle of modular and open architecture products is summarized in .
3.4 Benefits and limitations of MAPs
The use of MAPs involves important considerations through the lifecycle of the product. The main sustainability enhancements are focused on the reduction of material and energy during the manufacturing stage, which also is related to the reduction of required energy in the final disposal stage to convert the product into raw material. It is possible to identify a benefit cycle between final disposal stage and manufacturing and use stages, the reuse and commonality of components can be seized to extend the product use stage and diminish the roadmap and replacing time of products. The consideration of MAPs from early design stages provides the generation of product architecture alternatives to diminish negative sustainability impacts from manufacturing, use and final disposal stages. In addition, the modularisation also entails the improvement of remanufacturing, reuse and recycle from the perspective of ease of disassembly. summarizes the potential benefit cycle in open architecture products and its comparison respect modular architecture products (mass customization).
Current product development processes are centred in the generation of product families, which involves the use of product platforms, defined as the set of hardware, software, and resources related to the development of products. Conventionally, the product families are designed taking into account a differentiation in market segments, users, special requirements and feedbacks from users, manufacturers and all the actors associated with the development process. Each product variant is not related or linked to the others. Once the product leaves the manufacturing stage and the following stages of the lifecycle (Use and Final Disposal), it is isolated and conceived without any relationship with the origin product platform and the rest of product variants.
Among the attributes of MAPs in open architecture, there are three specific aspects of interest. The first aspect is reusability, related to the reuse of modules between the use, final disposal and manufacturing stages. Regarding this aspect, some MAPs are preferable such as stacking or component swapping, in which the modules are not employed all the time and the interchange of modules implies standby times for those modules that are inactive. MAPs such as adjustment or widening involve the use of the adjustment module for all possible configurations. Due to this, its relative useful life is shorter compared to interchangeable principles. The second aspect is the modular independence, associated with the ability of functional modules of work in another product variant when they are not used in the current product configuration. For instance, modules of widening principle cannot work in another product variant because all the modules are integrated into the same product, whereas the stacking modules can be shared with another product variant simultaneously. The third aspect is the ability of the MAP for replacing a family product. From the perspective of sustainability, this is the most significant benefits. The integration of different functionalities and levels provides the replacement of product families by a single modularized product variant. For example, it is possible to replace different product variants through a unique product with the adjustment. For example, an adjustable wrench replaces a family of wrenches. The widening principle also provides the integration of several products in one, e.g. the Swiss Army knife replaces knife, scissors, and corkscrew among others by a unique integrated product.
The most common limitation of the use of MAPs is the functional centralization of modules, which means dependency of other modules to achieve the desired function and the idle time associated with it. However, the use of MAPs can be improved by increasing the reliability of components and following adequate maintenance tasks. summarizes the benefits, limitations and sustainability impacts of the MAPs implementation in product development. sums up the reusability, modular independence and the ability of family product replacement.
Table 6. Sustainability benefits, impacts, and limitations of MAPs.
Table 7. MAPs respect reusability, modular independence, and family product replacement.
4. Strategies for sustainability based on MAPs
In this section, six main strategies or interventions are proposed considering the benefits/limitations of MAPs to develop products or product families for personalization. It is important to clarify that modularization in this section is only related to modular architecture principles mentioned in previous section, other approaches to modular design are not contemplated in this work.
The proposed strategies are actions that combined with MAPs promote guarantee a reduction of negative impacts regarding the environment, economy, and social dimensions. The focus of the strategies is the use of minimum resources to achieving the desired performance of different product variants through an open architecture platform. It is important to clarity that the proposed strategies need to be considered from early design stages in conjunction with MAP’s to generate substantial impacts in further lifecycle stages of products. The strategies are described in detail below.
4.1 Reuse of modules
Modular products are suitable for reuse modules not only between the final disposal and manufacturing stage but also in use stage or operation. The destination stage involves specific considerations. In the manufacturing stage, the responsible is the manufacturer, which requires a specific supply chain to receive and classify the parts from the final disposer. In the case of the use stage, the responsible of re-incorporate the used part is the customer. In this case, the consciousness and sustainability perspective is strongly needy. Both scenarios are described in detail below.
4.1.1 Reuse by the manufacturer
The reusability in the manufacturing stage provides benefits such as the reduction of raw material, processing energy, manufacturing time and all costs associated with these tasks. In the case of modular and open architecture products, the requirements of supply chains and management of components are the same than regard to conventional products. The used part is commonly used in a refurbished product and cannot be offered as a new part.
4.1.2 Reuse by the customer
Commonly, the disassembly operator and re-manufacturers reuse components, and customers do not have any responsibility (Lu et al. Citation2014). In the case of open architecture products, it is possible to reuse components in both stages manufacturing and use. The modularity of the product should be able to provide an easy addition, removal or modification of modules. In the use stage, the reusability provides savings to the user related to the cost of spare parts and extension of the useful life of products. Reusable parts in the use stage can also be considered as spare or refurbished parts.
4.2 Robust selection and evaluation of MAPs
According to Kimura et al. (Kimura et al. Citation2001) is complex to introduce reused parts based on a conventional product structure. For this reason, it is necessary an appropriate product modularization that provides the enhancement of the product management in its life cycle. The identification and selection of the most suitable MAP according to the design requirements are critical, taking into account that several principles can be employed to modularize a component. For this reason, a systematic and analytic selection process of MAPs is highly recommended. For these tasks, Mesa et al. (Mesa et al. Citation2015) have developed a functional characterization of MAPs; however, it is necessary to consider sustainability issues and benefits of each principle as well. In this field, some works such Seliger et al. (Seliger, Weinert, and Zettl Citation2007) and Erixon et al. (Erixon, von Yxkull, and Arnström Citation1996) propose the consideration of modules in reuse, recycling, landfill and remanufacturing. However, this approach does not cover the use of all modular architecture principles and their different functional attributes and benefits.
4.3 Common interface design
Modular products are designed to be easily assembled and disassembled; therefore, the selection and design of joints and interfaces between modules are critical. An ideal modular product should be designed using a common system of interfaces. This feature facilitates and standardizes the assembly/disassembly tasks and, consequently, provides savings in the lifecycle stages where adding, substituting, and removing modules is required. The interfaces of modular products are critical not only in the manufacturing and final disposal stage but also in the user stage. The tasks related to upgrading, maintenance, and module addition/removal are critical as well. The reusability is highly related to the design of interfaces. A poor interface design entails the excessive use of time and resources in the modularization tasks during the manufacture, use, and final disposal stages.
4.4 Actions for corporate responsibility
The manufacturer and final disposer should develop alliances and formal supply chains to guarantee the reuse and recycling of modules. The recycling provides raw material that can substitute raw material from primary sources, and the reuse minimizes cost in the manufacturing of refurbished products. An ideal model of sustainable product development includes a direct supply chain from the final disposer to the manufacturer or the creation of a productive unit in charge of receiving, classifying and shipping parts to the manufacturing and assembly lines in the case of reusable products.
In the case of recycled material, this should be shipped to the material producer or the manufacturer depending on the primary form required in both stages. The recycled material can substitute the raw material from primary sources, although can represent impacts on the processing costs. In the latter scenario, the use of educational and consciousness campaigns can be implemented to explain to the customers about recycled materials and the reasons for the cost increase associated with the final product.
4.5 Actions for user responsibility
The user in the modular product platform has the most important responsibility concerning sustainability. Once the product passes to the customer, the user is responsible for the correct use of the product and the adequate final disposal when it is necessary. The user consciousness is the main objective of this strategy, and the sustainability success in subsequent lifecycle stages depends in large part on the user decisions.
Since the use stage involves upgrading, maintenance and addition/removal of modules, it is ideal at this stage to receive reused parts, spare parts and to provide an adequate maintenance schedule in the case of MAPs with functional centralization. Besides, the user should carry the product to the final disposer, facilitating the cycling of resources. Another important aspect related to consciousness is the recycling culture and the awareness of acquiring products from companies with sustainability responsibility. For instance, companies with supply chains from the final disposer and material producer that guarantees the cycling of the material and its waste generation.
Implementation of each proposed strategy involves the interaction of many actors along the lifecycle of the product. The five proposed strategies described in this section can be integrated into the lifecycle of products, as is showed in .
From the implementation of the strategies, the following benefits can be expected:
Products designed to be easily disassembled and assembled to facilitate their reuse, repairing, updating, and final disposal.
Products oriented to be updated instead of replaced by a new one completely. The updating process is also improved through the common interface design and the easy assembly and disassembly of products.
Policies oriented to benefit or retribute the user when final disposal involves the manufacturer.
Increase of the sustainability performance of manufacturer companies, indicators associated with environmental and social issues can be improved.
Generation of new business units focused on the management and exploitation of reused components and products. This is the generation of value from old products.
5. Evaluation of proposed strategies for sustainability based on MAPs
The proposed strategies involve different implications not only technical but also behavioral (in the case of the user). summarizes the implications of each strategy and some evaluation tools to measure and determine the degree of success of each one. On the other hand, shows a list of remarkable state-of-the-art approaches that can enable a first approach to each strategy.
Table 8. Evaluation of proposed strategies for sustainability based on MAPs.
Table 9. Some existing approaches to enable the comprehension of the strategies for sustainability.
6. Future challenges
According to the sustainability analysis and the strategies mentioned, it is possible to identify the future challenges in the use of MAPs as a strategy for the establishment of modular and open architecture products
Design methods oriented to generate open architecture products especially focused on the modularization regarding not only manufacture and final disposal but also the use, repair, refurbish, and update scenarios. It is possible to find several works concerning the design of joints and product interfaces, but these are not oriented to improve sustainability as their main objective
The need for policies of corporate sustainability responsibility since the lack of them difficult the integration of manufacturers, material producers, and final disposals companies. The establishment of effective supply chains between these lifecycle stages involves the reduction of costs and better use of resources. The current separation of the companies participating in the product lifecycle generates an increase of new raw material processing, pollution due to products not sent to the final disposer, and emissions, among others.
Policies focused on educating users on sustainability and their responsibility during the use or operation stage. It is important to remark the awareness about reusability, the need to carry the product to the final disposal to continue with the cycle expected, and the importance of acquiring products from companies with sustainable supply chains from final disposal and material production. This challenge is one of the most important to face. Sustainability education should be embedded in the learning culture from pre-K to higher education.
The need for globally accepted metrics for sustainability: it is necessary to establish globally accepted metrics for assessing the lifecycle of modular products, taking into account the specific features involved in modularity (commonality of components, module sharing among others). Currently, it is very complex to develop the assessment of sustainability performance of products due to the variety of companies and the use of appropriate corporate indicators.
7. Conclusions
The work proposed in this paper describes the sustainability issues facing the globalized market and the use of modular and open architecture products to satisfy the customer requirements in the market of one. Additionally, this work provides an analysis of Modular Architecture Principles – MAPs that offer advantages regarding reducing consumption of resources and facilitating the management of modules between the manufacturing, use, and final disposal stages. Six strategies oriented to guarantee sustainability using MAPs are proposed and described. Such strategies simplify the lifecycle relationship and diminish adverse impacts mainly in environmental and economic dimensions.
The framework and strategies proposed in this article contribute to the state of the art regarding sustainable product design. Not only oriented to facilitate the implementation of sustainability in the product design process, but also the consideration of further impacts from early design phases.
The social implications of the strategies depend largely on the education and training of designers, manufacturers, consumers and other stakeholders involved in the product lifecycle to reach the maximum possible positive impact. According to the literature review and analysis of MAPs concerning product sustainability, the following conclusions are listed:
Methods related to product sustainability are mainly focused on conventional or non-modular products. Therefore, the implementation of personalization model implies the need for specific methodologies that consider the lifecycle and the sustainability issues in each one of them. The design of product platforms for open architecture products is still under development and requires sustainability considerations as well.
The open architecture products involve the modularity as a critical topic in the meeting of customer requirements regarding personalization. MAPs in open architecture products also provide the possibility of enhancing the supply chains between the manufacturing, use and final disposal stages. These supply chains between lifecycle stages afford the sustainability improvement concerning reuse, remanufacturing and recycling; involving reduction of materials and energy consumptions.
Educational and training policies for designers, manufacturers, user and all other actors participating in the product lifecycle are urgently needed. The user perspective and awareness of the impacts on sustainability derived from consumption of products are critical and necessary to reach a major sustainability enhancement it is critical to educate first the individual with strong foundations in sustainability related to awareness and behaviors, and then educate the professional who will put into practice principles for sustainable modular design. Sustainability concepts on reusability and recycling should be included early in the schools.
Disclosure statement
No potential conflict of interest was reported by the authors.
Additional information
Funding
Notes on contributors
Jaime Alberto Mesa
Jaime Alberto Mesa Associate Professor at Universidad Tecnológica de Bolivar, Cartagena, Colombia. Received his B.S in Mechanical Engineering from Universidad Pontificia Bolivariana,Montería, Colombia and hisMSc. and Ph.D. (Summa Cum Laude) in Mechanical Engineering from Universidaddel Norte, Barranquilla, Colombia. His research interests include sustainable design, design methods, circular economy, modular design and reconfiguration, Materials Science, additive manufacturing, eco-design and engineering education.
Iván Esparragoza
Iván Esparragoza Professor of Engineering and Director ofEngineering Technology andCommonwealth Engineering at Penn State Brandywine, PA, USA. Received his B.S. in Mechanical Engineering from Universidad del Norte, Barranquilla, Colombia. And his M.Sc.and Ph.D. in Mechanical Engineering from Florida International University, Miami, FL.His research interests include sustainability, global engineering design, and education.
Heriberto Maury
Heriberto Maury Professor ofMechanical Engineering at Universidad del Norte, Barranquilla Colombia. Received his B.S in Mechanical Engineering from Universidad del Norte and hisPh.D. in Industrial Engineering from Universidad Politécnica de Cataluña, Spain. Dr. Maury’s research is focused on the areas of product design, concurrent engineering, material handling systems, sustainability, reconfiguration, and modular design.
References
- Ameli, M., S. Mansour, and A. Ahmadi-Javid. 2016. “A Multi-Objective Model for Selecting Design Alternatives and End-Of-Life Options under Uncertainty: A Sustainable Approach.” Resources, Conservation and Recycling 109: 123–136. doi:10.1016/j.resconrec.2016.01.011.
- Bhamu, J., and K. Sangwan. 2014. “Lean Manufacturing: Literature Review and Research Issues.” International Journal of Operations & Production Management 34 (7): 876–940. doi:10.1108/IJOPM-08-2012-0315.
- Byggeth, S., G. Broman, and K.-H. Robert. 2007. “A Method for Sustainable Product Development Based on A Modular System of Guiding Questions.” Journal of Cleaner Production 15: 1–11. doi:10.1016/j.jclepro.2006.02.007.
- Chang, T.-R., C.-S. Wang, and -C.-C. Wang. 2013. “A Systematic Approach for Green Design in Modular Product Development.” International Journal of Advanced Manufacturing Technology 68: 2729–2741. doi:10.1007/s00170-013-4865-5.
- Chung-Shing, W. 2009. “Web-Based Modular Interface Geometries with Constraints in Assembly Models.” Computers & Industrial Engineering 56 (4): 1675–1686. doi:10.1016/j.cie.2008.10.018.
- Cor, E., and P. Zwolinski. 2014. “A Procedure to Define the Best Design Intervention Strategy on A Product for A Sustainable Behavior of the User.” Procedia CIRP 15: 425–430. doi:10.1016/j.procir.2014.06.075.
- Dahmus, J., J. Gonzalez-Zugasti, and K. Otto. 2001. “Modular Product Architecture.” Design Studies 22 (1): 409–424. doi:10.1016/S0142-694X(01)00004-7.
- Devanathan, S., D. Ramanujan, W. Z. Bernstein, F. Zhao, and K. Ramani. 2010. “Integration of Sustainability into Early Design through the Function Impact Matrix.” Journal of Mechanial Design 132: 081004. doi:10.1115/1.4001890.
- Eddy, D., S. Krishnamurty, I. Grosse, J. Wileden, and K. Lewis. 2015. “A Predictive Modelling-Based Material Selection Method for Sustainable Product Design.” Jorunal of Engineering Design 26 (10–12): 365–390. doi:10.1080/09544828.2015.1070258.
- Erixon, G., A. von Yxkull, and A. Arnström. 1996. “Modularity - the Basis for Product and Factory Reengineering.” CIRP Annals 45: 1–6. doi:10.1016/S0007-8506(07)63005-4.
- Fiorineschi, L., F. Fricilli, P. Rissone, and G. Cascini. 2015. “Product Architecture Definition: Evaluating the Potentiality of TRIZ Tools.” Procedia Engineering 131: 359–371. doi:10.1016/j.proeng.2015.12.415.
- Fujita, K. 2002. “Product Variety Optimization under Modular Architecture.” Computer-Aided Design 34 (12): 953–965. doi:10.1016/S0010-4485(01)00149-X.
- Gifford, R., and A. Nilsson. 2014. “Personal and Social Factors that Influence Pro-Environmental Concern and Behaviour: A Review.” International Journal of Psychology 49 (3): 141–157. doi:10.1002/ijop.12034.
- Giudice, F., F. Balisteri, and G. Risitano. 2009. “A Concurrent Design Method Based on DFMA-FEA Integrated Approach.” Concurrent Engineering 17 (3): 183–202. doi:10.1177/1063293X09343337.
- Gu, P., M. Hashemian, and A. Nee. 2004. “Adaptable Design.” CIRP Annals 53 (2): 539–557. doi:10.1016/S0007-8506(07)60028-6.
- He, B., W. Tang, J. Wang, S. Huang, Z. Deng, and Y. Wang. 2015. “Low-Carbon Conceptual Design Based on Product Lifecycle Assessment.” International Journal of Advanced Manufacturing Technology 81: 863–874. doi:10.1007/s00170-015-7253-5.
- Hu, S., J. Ko, L. Weyland, H. ElMaraghy, T. Lien, Y. Koren, … M. Shpitalni. 2011. “Assembly System Design and Operations for Product Variety.” CIRP Annals-Manufacturing Technology 60 (2): 715–733. doi:10.1016/j.cirp.2011.05.004.
- Jian, P., J. Leng, K. Ding, P. Gu, and Y. Koren. 2016. “Social Manufacturing as a Sustainable Paradigm for Mass Individualization.” Proceedings of the Institution of Mechanical Engineers, Part B: Journal of Engineering Manufacture. doi:10.1177/0954405416666903.
- Kimura, F., S. Kato, T. Hata, and T. Masuda. 2001. “Product Modularization for Parts Reuse in Inverse Manufacturing.” CIRP Annals - Manufacturing Technology 50 (1): 89–92. doi:10.1016/S0007-8506(07)62078-2.
- Kissling, R., C. Fitzpatrick, H. Boeni, C. Luepschen, S. Andrew, and J. Dickenson. 2012. “Definition of Generic Re-Use Operating Models for Electrical and Electronic Equipment.” Resources, Conservation and Recycling 65: 85–99. doi:10.1016/j.resconrec.2012.04.003.
- Koga, T., and K. Aoyama (2008). “Modular Design Method for Sustainable Life-Cycle of Product Family considering Future Market Changes.” Proceedings of the ASME 2008 International Design Engineering Technical Conferences & Computers and Information in Engineering Conference. Brooklyn, New York.
- Kollmuss, A., and J. Agyeman. 2010. “Mind the Gap: Why Do People Act Environmentally and What are the Barriers to Pro-Environmental Behavior?” Environmental Education Research 8 (3): 239–260. doi:10.1080/13504620220145401.
- Koren, Y., S. Hu, P. Gu, and M. Shpitalni. 2013. “Open-Architecture Products.” CIRP Annals - Manufacturing Technology 62: 719–729. doi:10.1016/j.cirp.2013.06.001.
- Koren, Y., M. Shpitalni, P. Gu, and S. Hu. 2015. “Product Design for Mass-Individualization.” Procedia CIRP - 25th Design Conference Innovative Product Creation 36: 64–71.
- Kovács, G. 2008. “Corporate Environmental Responsibility in the Supply Chain.” Journal of Cleaner Production 16 (15): 1571–1578. doi:10.1016/j.jclepro.2008.04.013.
- Kriwet, A., E. Zussman, and G. Seliger. 1995. “Systematic Integration of Design-For-Recycling into Product Design.” International Journal of Production Economics 38 (1): 15–22. doi:10.1016/0925-5273(95)99062-A.
- Kyoung-Yun, K., D. Manley, and H. Yang. 2006. “Ontology-Based Assembly Design and Information Sharing for Collaborative Product Development.” Computer-Aided Design 38 (12): 1233–1250. doi:10.1016/j.cad.2006.08.004.
- Lu, B., B. Li, L. Wang, J. Yang, J. Liu, and X. Wang. 2014. “Reusability Based on Life Cycle Sustainability Assessment: Case Study on WEEE.” Procedia CIRP 15: 473–478. doi:10.1016/j.procir.2014.06.046.
- Ma, J., and G. Kremer. 2016. “A Sustainable Modular Product Design Approach with Key Components and Uncertain End-Of-Life Strategy Consideration.” The International Journal of Advanced Manufacturing Technology 85 (1): 741–763. doi:10.1007/s00170-015-7979-0.
- Mesa, J., H. Maury, R. Arrieta, A. Bula, and C. Riba. 2015. “Characterization of Modular Architecture Principles Towards Reconfiguration: A Firts Approach in Its Selection Process.” International Journal of Advanced Manufacturing Technology 80 (1): 221–232. doi:10.1007/s00170-015-6951-3.
- Mutha, A., and S. Pokharel. 2009. “Strategic Network Design for Reverse Logistics and Remanufacturing Using New and Old Product Modules.” Computers & Industrial Engineering 56 (1): 334–346. doi:10.1016/j.cie.2008.06.006.
- Orlitzky, M., D. Siegel, and D. Waldman. 2011. “Strategic Corporate Social Responsibility and Environmental Sus.” Business & Society 50 (1): 6–27. doi:10.1177/0007650310394323.
- Ramani, K., D. Ramanujan, W. Z. Bernstein, F. Zhao, J. Sutherland, C. Handwerker, … D. Thurson. 2010. “Integrated Sustainable Lifecycle Design: A Review.” Journal of Mechanical Design 136: 091004–1 091004–15. doi:10.1115/1.4002308.
- Remery, M., C. Mascle, and B. Agard. 2012. “A New Method for Evaluating the Best Product End-Of-Life Strategy during the Early Design Phase.” Journal of Engineering Design 23 (6): 419–441. doi:10.1080/09544828.2011.605061.
- Rizzi, F., I. Bartolozzi, A. Borghini, and M. Frey. 2012. “Environmental Management of End-Of-Life Products: Nine Factors of Sustainability in Collaborative Networks.” Business Strategy and the Environment 22 (8): 561–572. doi:10.1002/bse.1766.
- Romli, A., P. Prickett, R. Setchi, and S. Soe. 2015. “Integrated Eco-Design Decision-Making for Sustainable Product Development.” International Journal of Production Research 53 (2): 549–571. doi:10.1080/00207543.2014.958593.
- Rusinko, C. 2007. “Green Manufacturing: An Evaluation of Environmentally Sustainable Manufacturing Practices and Their Impact on Competitive Outcomes.” IEEE Transactions on Engineering Management 54 (3): 445–454. doi:10.1109/TEM.2007.900806.
- Seliger G., Weinert N., Zettl M.. (2007). Module Configurator for the Development of Products for Ease of Remanufacturing. In: Takata S., Umeda Y. (eds) Advances in Life Cycle Engineering for Sustainable Manufacturing Businesses. London: Springer
- Shrivastava, P., and S. Berger. 2010. “Sustainability Principles: A Review and Directions.” Organization Management Journal 7 (4): 246–261. doi:10.1057/omj.2010.35.
- Simpson, T. 2004. “Product Platform Design and Customization: Status and Promise.” Artificial Inteligence for Engineering Design, Analysis and Manufacturing 18: 3–20.
- Smith, S., and -C.-C. Yen. 2010. “Green Product Design through Product Modularization Using Atomic Theory.” Robotics and Computer-Integrated Manufacturing 26 (6): 790–798. doi:10.1016/j.rcim.2010.05.006.
- Stevels, A. 1997. Optimization of the End-Of-Life System. Ecodesign: A promising Approach. UNEP Working Group on sustainable Product Development.
- Subramaniyam, P., K. Srinivassan, and M. Prabaharan. 2011. “Approach for Green Product Design.” International Journal of Innovation, Management and Technology 2 (3): 244–248.
- Tian, J., and M. Chen. 2014. “Sustainable Design for Automotive Products: Dismantling and Recycling of End-Of-Life Vehicles.” Waste Management 34: 458–467. doi:10.1016/j.wasman.2013.11.005.
- Tseng, K., B. Lin, and C.-M. Han. 2012. “An Intelligent System for Sustainable Product Design at the Concept Development Stage.” Computer-Aided Design and Applications 9 (3): 397–408. doi:10.3722/cadaps.2012.397-408.
- Umeda, Y., S. Fukushige, K. Tonoike, and S. Kondoh. 2008. “Product Modularity for Life Cycle Design.” CIRP Annals - Manufacturing Technology 57 (1): 13–16. doi:10.1016/j.cirp.2008.03.115.
- UPSTART. (2016, 05). “News from the Entrepreneurship Community in Singapore.” START-UPS:Singaporean engineer builds the world’s first multi-configuration bicycle with help from ACE Start-up Grant(3). Retrieved 10 May 2017. http://www.upstartnews.sg/archive/03/05.html
- Vinodh, S., and G. Rathod. 2010. “Integration of ECQFD and LCA for Sustainable Product Design.” Journal of Cleaner Production 18: 833–842. doi:10.1016/j.jclepro.2009.12.024.
- Yan, J., and C. Feng. 2014. “Sustainable Design-Oriented Product Modularity Combined with 6R Concept: A Case Study of Rotor Laboratory Bench.” Clean Technologies and Environmental Policiy 16: 95–109. doi:10.1007/s10098-013-0597-3.
- Yung, W., H. Chan, D. Wong, J. So, and A. Choi. 2012. “Eco-Redesign of a Personal Electronic Product Subject to the Energy-Using Product Directive.” International Journal of Production Research 50 (5): 1411–1423. doi:10.1080/00207543.2011.571941.