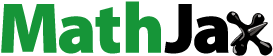
ABSTRACT
This study investigates the effects of various dosages of bacteria on the properties of sustainable bacteria blended fly ash (FA) concrete. Different series of concrete mixtures were designed, with Series C being the plain concrete mix (no FA and bacteria). Series CF contained FA concrete mixes that had 10%, 20% and 30% FA content. Series CB and CBF were prepared with bacteria with varying dosages. Surface deposition of calcium carbonate crystals was found to improve strength and decreased sorptivity by 30–60%, depending on the type of bacteria and FA proportion. The best results in comparison with normal and FA concrete for compressive strength (CS) were obtained to be 31.76 MPa. The bacteria solution to water ratio had a positive effect on strength and sorptivity property but with increasing ratio. This study also applies multiple regression analysisin estimating the CS of concrete that contains FA at a fixed value of bacterial dosage at different curing times. The present paper also shows a mathematical model to predict the CS of concrete with bacteria solution to water ratios. The X-ray diffraction and SEM analysis indicated the formation of calcite crystals in bacterial concrete specimens.
1. Introduction
Concrete the most common building material which is a mixture of cement, water and aggregates (Sathawane, Vairagade, and Kene Citation2013). Concrete is strong and also a low-cost construction material. Since cement being cheap and easily available, it is the first choice of civil engineers as a construction material (Jonkers et al. Citation2010). In forthcoming years, construction sector is expected to grow at a higher rate. Concrete has got a unique position in various construction materials. Apart from the strength of concrete, durability is also an important construction property for providing a better service life. Currently, around 60 billion tons of constructional materials per year are being used. The global construction sector alone contributes to around 3000 Mt/year (50% by weight). In the coming year’s growth in the construction sector will move at a higher rate.
The main raw material of concrete is cement which has to be produced in large quantity. Throughout the world, 5000 million tons of cement was produced in 2014. The main environment threat is in the production of cement. The cement manufacturing process is one of the most energy-intensive processes consuming 12–16% of the total energy consumption in various industries. Another major limitation is the release of around 6% of the global anthropogenic CO2 emissions. From an environmental point of view, cement is not a sustainable material (Jonkers et al. Citation2010). Therefore efforts are underway to reduce the quantity of cement being used. Sustainability in concrete can be brought by partial replacement of cement with supplementing cementing materials (SCM) which are well known and most popular in civil engineering world.
With foreseeable increase in world population, the requirements of building and infrastructure will increase the demand of cement. A rapid growth may be observed in the utilisation of non-renewable materials and generation of waste materials. Hence, the immediate solution to overcome these problems is to develop sustainable construction materials which can contribute to the eco-efficiency of the construction sector and hence a more sustainable development (Pacheco-Torgal and Labrincha Citation2013).
Utilising SCM reduces the consumption of cement, and thereby reduces the energy consumption and greenhouse gas emissions related to cement production. Application of SCM as partial replacement for cement offers a partial solution that helps us to reach targets of increasing demand for concrete (Barbhuiya Citation2009). One of the common SCM used is FA. Recently developing building material which is pollution free is becoming a key factor in the construction sector. Developing environment-friendly building material involves minimising the environmental impact of concrete production by substituting cement partially with material such as FA due to its lightweight and high thermal insulation (Chahal and Siddique Citation2013).
FA, a landfill waste, is commonly being employed in concrete to cut down the quantity of cement being used. Every year million tons of FA are being dumped and only partially amount used in one or other application. In countries like China, the US and India, FA are stockpiled in huge quantities. The annual generation of FA is approximately 500 million tonnes/year. The dumping of huge quantity of FA is a crucial issue as it contributes to a serious environmental problem. Presently, India is among the top 10 countries in power generating and consuming in the world. Presently, around 30% of FA generated is being used in the construction sector (cement manufacture, construction of roads and other application in civil engineering, etc.). Major quantity of FA (which is around 50%) is just being dumped. Storing of FA requires large land area which effects agricultural growth and results in severe environmental degradation which would be disastrous for India. These environmental situations do not allow in dumping large amount of FA (Kwangryul, Noguchi, and Tomosawa Citation2004). To mitigate the impact of FA on the environment, FA has to be reused effectively.
FA, when used as moderate proportions for cement replacement, improves the rheological characteristics of concrete. However, major drawback noted in the utilisation of FA in various proportions in concrete is that it fails to gain early strength. This limitation of FA concrete can be overcome by adapting implementing biomineralisation tool in FA concrete.
Various researchers have discussed biomineralisation tool as a valuable technique that can be implemented in concrete to develop by inducing dormant but viable spores of alkali-resistant urease producing bacteria that convert organic compounds to inorganic mineral precipitates, i.e. calcite. To achieve sustainability in concrete, a biomineralisation mechanism is being adapted in concrete. Biomineralisation is a widespread complex phenomenon where certain organisms form minerals by various biochemical reactions (Ghosh and Mandal Citation2006). Researchers are focussing attention on the production of sustainable concrete. Biological technique when introduced in fresh concrete results in formation calcite in voids and consequently improves overall properties of concrete (Siddique et al. Citation2017).
Bacterial technique when used in fresh concretes results in calcite precipitation in voids and consequently improves the strength and lowers permeability of concrete.
Bacteria have greatest advantage since they do have largest surface to volume ratio. These unique advantages of bacteria provide a large contact area which can interact with the surroundings (Siddique et al. Citation2017). Urease activity is common among different bacteria, but only few specific strains have the ability to produce high levels of urease enzyme. Theoretically, ureolytic bacteria will continue to hydrolyse urea as long as the urease enzyme remains active.
Some of the pathogenic bacteria such as Helicobacter, Proteus vulgaris and Pseudomonas aeruginosa also produce urease. But these bacteria are pathogenic in nature and have proved to produce urinary infection as well as intracellular urinary stones (Periasamy et al. Citation2016). Ramakrishnan, R K, and Bang (Citation2005) and Achal (Citation2010) found that calcite precipitation by Bacillus pasteurii and Bacillus sphaericus was effective in filling the cracks by calcite in concrete. Other bacillus species reported for calcite precipitation are Bacillus pseudofirmus and Bacillus cohnii (Jonkers and Schlangen Citation2009), Bacillus alkalinitrilicus (Kim Citation2013) and other genera such as Shewanellaspecies (Muynck, Debrouwer, and Verstraete Citation2008; Ghosh et al. Citation2009), Cyanobacteria Synechococcus (Zhu and Dittrich Citation2016), P. aeruginosa (Ramachandran et al. Citation2001), Escherichia coli (Ruiyang and Rong Citation2015), Bacillus megaterium (Krishnapriyaa, Venkatesh Babu, and Arul Raj Citation2015) Proteus mirabilis and P. vulgaris (Pacheco-Torgal and Labrincha Citation2013).
Khaliq and Ehsan (Citation2016) found that calcite precipitation by Bacillus subtils was effective in healing cracks of concrete. Halder et al. (Citation2014) showed that mortar prepared with FA up to 5% replacement by weight and bacteria improved compressive strength (CS) and durability of mortar. Research conducted by Talaiekhozani et al. (Citation2014) shows that two indigenous micro-organisms (P. mirabilis and P. Vulgaris) isolated from soil were able to heal the broken concrete. However, moderate improvement in strength was reported. The best environmental pH of P. mirabilis and P. vulgaris to grow is around 7.5 which is less than pH of fresh concrete (Wang, Soens, and De Citation2014) was the reason reported for moderate improvement in strength. Andalib et al. (Citation2016) revealed that the significant increase in the strength was obtained in the case of 30 × 105 cfu/mL at different ages. Biomineralisation is a promising, eco-friendly alternative approach to conventional and current remediation technologies to solve environmental problems in multidisciplinary fields (Seifan, Samani, and Berenjian Citation2017).
Several studies have been reported on the use of varying bacterial dosage in concrete but no information has been reported on the quantity of bacteria solution being used. This study investigates the effects of varying dosages of bacteria and varying ratio of bacteria solution to water ratio on the properties of FA concrete.
Bacterial concrete CS is influenced by many factors such as bacteria solution to water ratio, age, cement content and properties. The secondary objective of this paper is to develop a simple mathematical model for bacterial FA blended concrete that can help to predict the CS at any age.
2. Experimental programme
2.1. Bacterial strains
Biomineralisation process depends on the types of bacteria. Selection of bacteria is a key factor in the biomineralisation process. Bacteria must fulfil some of the requirements for improving the properties of concrete. It must be able to adjust to alkaline atmosphere in concrete for the production of calcium carbonate, it should produce copious amount of calcium carbonate without being affected by calcium ion concentration, it must be able to withstand high pressure and should be oxygen brilliant to consume much oxygen and minimise corrosion of steel (Khaliq and Ehsan Citation2016).
The bacterial strain considered for this research is B. sphaericus which fulfilled the necessary criteria for survival in harsh environment. This strain was procured from National Collection of Industrial Microorganisms, Pune. B. sphaericus bacteria are alkaliphilic (alkali-resistant) spore-forming bacteria (Jianyun et al. Citation2012). Liquid medium solution for B. sphaericus was prepared by using nutrient broth solution supplemented with urea and CaCl2 (Khaliq and Ehsan Citation2016). The medium was sterilised by autoclaving for 20 min at 120°C. The selected strain was introduced in the medium through sterilised loop.
In order to determine cell concentration of the bacterial solution, the optical density (OD) was measured using a spectrometer. The cells of B. sphaericus do not aggregate and thus it ensures a high cell surface to volume ratio that is essential for efficient cementation initiation. Bacteria solution was tested with a wavelength of 600 nm and the OD values were noted.
Therefore, the ability of bacteria to tolerate a high pH and also the capability of CaCO3 precipitation in such conditions are the main challenges to design a sustainable bioconcrete.
2.2. Effects of pH and temperature on growth of bacteria
Biomineralisation process relies on pH and temperature (Jianyun et al. Citation2012). The pH of concrete is higher and bacteria have to survive at higher pH for biomineralisation process. Biomineralisation process is also temperature dependent and it ranges in between 20°C and 30°C (Pacheco-Torgal and Labrincha Citation2013). The pH and temperature are the influential parameters on growth and biomineralisation process. With varying pH and temperature growth of bacteria may be hindered which may effect the biomineralisation process. Hence, selection of bacteria for such conditions is the main challenges to develop a sustainable concrete. In view of this, experiments were conducted to find the suitability of bacteria to tolerate greater pH (8–12) and temperature variations (24–45°C). To adjust the pH of the solution, sodium chloride (1 N solution) and hydrochloric acid (1 N) were used. The prepared bacterial solution was kept in a shaker (120 rpm) at a temperature of 37°C. The growth of all the bacterial strains was studied by noting OD at 600 nm. The OD was noted at regular interval of 1 h by using colorimeter. Survival of bacteria in varying conditions of temperature and pH is essential for effective biochemical process.
2.3. Concrete constituent
The cement employed for the research work was a commercially available ordinary Portland cement. FA used was procured from Raichur power plant. Properties of cement and FA are listed in . Natural sand (size 4.75 mm) and crushed stone (maximum 20 mm) were used as fine and coarse aggregate and were tested for their suitability in concrete as per Indian Standard Specifications and listed in .
Table 1. Composition of Portland cement and fly ash used in this study.
Table 2. Physical properties of materials used in concrete mixture.
2.4. Concrete mixture proportions
The main constituents of FA blended bacterial concrete include cement, FA, coarse aggregate, fine aggregate, water and bacterial solution. Concrete cubes of size 150 mm were cast for CS test and sorptivity test. For the tensile strength, cylindrical specimens of diameter 150 mm and length 300 mm were prepared. Three specimens were produced for each testing age. CS was determined using digital testing machine of 2000 kN. Split tensile strength is an indirect test to determine the tensile strength of the cylindrical specimens of size 150 mm diameter and 300 mm height. Splitting tensile strength was determined in accordance with IS: 5816–1970.
Mixture proportions employed in this experimental work are shown in for M25 grade of concrete. Series C, the plain series, comprised of cement, fine and coarse aggregates. Series CF1, CF2 and CF3 comprised of cement, varying proportion of FA, fine and coarse aggregates. The replacement level of FA is varied for series CF series although the maximum replacement level did not exceed 30% (by weight of cement) for investigations with concrete. The series CB (3), CB (5) and CB (7), the bacterial series were prepared with cement, FA fine and coarse aggregates, bacteria solution of varying dosage. Similarly, the series CBF was prepared for bacterial blended FA concrete with varying proportion of FA and bacterial dosage, respectively.
Table 3. Proportion of concrete constituents present in the mixes.
Cubes and cylinders were cast and compacted on a vibrator machine. The specimens were immersed in water for 7, 28, 56 and 90 days depending upon the tests to be performed. The X-ray diffraction (XRD) spectrum of powdered bacterial and plain concrete was analysed using X-Ray Diffractometer; model Philips-PW3710/PW 1710. The components were identified by comparing with standards established by the International Centre for Diffraction Data. Bacterial and concrete samples were examined by SEM, Model – JEOl – SEM 6360. Twenty-eight day curing samples of both normal and bacteria concrete (cell concentration of 105 cells/mL) were considered for analysis.
2.5. Sorptivity
Sorptivity indicates the rate of penetration of water into the pores of concrete by capillary suction. Low sorptivity is the primary requirement to produce durable concrete. The cubes were supported on small supports. The arrangement was such that only the lowest 10 mm of the prism was submerged. The rise in water level in concrete which manifests itself by dark colour was measured at periodic intervals of 15 min. The procedure was continued till the rise in water level was stopped. The sorptivity was evaluated by using the formula mentioned in the following:
(Abalaka Citation2013)
S = sorptivity in mm/min1/2
m = rise of water level in mm
n = time taken for this rise in min
3. Results and discussions
3. Effects of pH and temperature on the growth of bacteria
The results reported in show the effect of pH on the growth of B. sphaericus. As it is seen in that bacteria B. sphaericus were able to grow between pH 8 and 11. The biomineralisation process involves precipitation of calcite which improves upon the properties of concrete. Upon the introduction of bacterial strain in nutrient broth solution, it takes time for growth which is known as lag phase. Once it adjusts to the environment, population tends to increase with higher growth rate which is known as log phase as it is evidenced in . Log phase is observed between time intervals of 9–17 h at all temperature. During this phase bacteria, active and biomineralisation rate may be high. Bacillus sphaericus showed better growth profile which indicates high to moderate population at all pH. With increase in pH rise in population was observed. After it reaches peak pH 9.5, growth or population decreases sharply. While low to moderate growth was observed at pH in the range 11–12. These types of bacteria which are able to survive with variation in pH are called alkaliphile bacteria which makes suitable for use in concrete. Effects of temperature on the growth of bacteria B.sphaericus can be found in .
Table 4. Test results for the growth of microbes at various pH with temperature as a parameter.
Optimum growth was observed at temperature 37°C compared to that at normal room temperature. At higher and lower temperature, B. sphaericus shows moderate growth making it more suitable for biomineralisation studies.
This can be attributed to enhanced activity of enzyme and rate of urea hydrolysis at this temperature, which increases the rate of growth. Wang et al. (Citation2017) reported that B. sphaericus can grow and germinate in a broad range of alkaline pH. This observation suggests that tropical climate with year-round hot and humid environment has great potential to engage microbial carbonate precipitation.
3.2. Compressive strength
The objective of this experimental work is to highlight the effect of bacteria on the CS of bacteria blended fly ash concrete (BBFC).
The CS test was performed on the specimens at the ages of 7, 28, 60 and 90 days. Bacteria B. sphaericus of different cell concentrations ranging from 103 to 107 cells/mL was introduced during mixing of concrete.
depicts the CS values of the concretes containing different proportions of FA and varying dosages of bacteria dosages under various curing times.
Table 5. Results of compressive strength.
Clearly, the CS of all series increased with curing period (). At all ages, CS of FA concrete displayed lower compressive strength than plain concrete.
Addition of bacteria of various dosage resulted in increment of CS for all types of concrete specimens. CS of plain concrete yielded 25.39, 33.33, 37.28 and 38.79 MPa at 7, 28, 60 and 90 days of curing.
Bacterial concrete specimens cast with B. sphaericus of cell dosage 105 cells/mL have yielded CSs of 31.76, 40.69, 43.74 and 44.90 MPa, respectively, which amount to 25.08%, 22.08, 17.32% and 15.78% greater compared to plain concrete. At bacteria concentration of 105 cells/mL due to higher biochemical reaction rate formation of calcite is high. 105 cells/mL dosage is obtained during exponential phase. During this phase, bacteria are active which hydrolyse more urea resulting in higher pH and also provide more nucleation sites for precipitation of CaCO3 crystals. Both would increase the amount of CaCO3 precipitated.
Incorporation of 103 cells/mL resulted in improvement of 10.90%, 10.41%, 2.54% and 2.5%, compared to control concrete specimens. Results obtained are in good agreement with results reported by Chahal, Siddique, and Rajor (Citation2012) and Siddique et al. (Citation2017). Chahal, Siddique, and Rajor (Citation2012) observed that addition of S. pasteurii significantly increased CS by around 20%, respectively, in comparison with the control specimens at 28 days. Siddique et al. (Citation2016) reported 10% increase in CS of concrete containing bacterial cells due to CaCO3 formations. Increase in strength property in BBFC might be due to the formation of the calcite in the pores, subsequently reduction in pores and compact microstructure obtained (Siddique et al. Citation2016). Incorporation of bacteria has increased the CS of concrete at all dosages. Maximum improvement in CSs was achieved at a cell dosage of 105cells/mL for all type of BBFC.
Concrete made with 10% FA showed the best results at 7, 28, 60 and 90 days of curing among all BBFC specimens. At 7, 28, 60 and 90 days, control series CF1 showed CS of 23.2, 31.84, 36.13 and 37.11 MPa, respectively, whereas BBFC with 10% FA and bacteria dosage of 105 cells/mL showed an improvement of 19.8%,17.46%,14.78% and 3.67% with respect to control concrete. The FA blended concrete showed lower gain than the plain concrete at early age. However, BBFC showed an improvement up to 20% compared to plain concrete. This finding indicates that the limitation of FA concrete in early gain of strength can be over by biomineralisation technique. Biomineralisation tool plays an important role in early gain of strength of FA concrete.
CS of plain concrete is 38.79 MPa at 90 days of curing. CS of BBFC with 30% replacement of cement with FA showed CS of 40.80 MPa, an improvement of around 5.2% in comparison with plain concrete. Utilisation of FA up to 30% replacement level greatly reduces the burden of high cost of cement. Further, it leads to dual environment benefits. Primarily, FA disposal from coal power plant into landfill is greatly reduced and cement replacement by FA lowers cement production thereby reducing greenhouse gases emission from cement plant. Bacterial dosage of 103 and 105 cells/mL shows the same trend in increase of CS.
The rate of increase in the CS of all BBFC specimens at 60 and 90 days was markedly less than that at 7 and 28 days. At young age, i.e. 7 days of curing in tap water highest percentage gain was observed in comparison with plain and control concrete. At young age, CS was found to increase with high gain and at latter age gain in strength tends to slowdown for 60 and 90 days as shown in . Due to biochemical process filling of pores by crystals of calcite near the cells and on the surface would be an important reason for gain in strength. It is due to this reason fraction of opening spaces in the matrix of concrete reduces. At young age, concrete specimen is porous which may lead to movement of oxygen or water into the matrix of specimens. This would have resulted in higher rate of biomineralisation process during initially 7 or 28 days in comparison with 60 and 90 days.
3.3. Ratio of CS to split tensile strength
The ratio of CS to split tensile strength was observed to be in the range8.45–9.81 for concrete blended with B. sphaericus (). The ratio for ordinary concrete is in the range 10.5–15.2 (Shetty Citation2005). This implies that bacterial concrete has higher split tensile strength than ordinary concrete (Thanh, Nguyen, and Ludwig Citation2014). Presence of bacteria may have resulted in improvement of split tensile strength. It is observed from the result that for bacterial concrete as CS increases split tensile increases but at a smaller rate. This implies that the relationship between split tensile and CS is nonlinear. The highest ratio amounted 9.81 at a FA content of 30% as a replacement of cement.
Table 6. Ratio of compressive strength to split tensile strength.
3.4. Prediction of CS
CS is one of the most important and useful properties of concrete. Concrete as a construction material is employed to resist compressive stresses. CS is referred as a quantitative measure for other properties of concrete. Multivariable regression analysis (MRA) was employed to predict the CS of BBFC. Yet so far no equation is available to predict CS for bacterial concrete based on the ratio of bacteria solution to water ratio. depicts CS values with variation in bacteria solution to water ratio.
Table 7. Results of compressive strength.
Several models have been proposed to predict CS based on age, water to cement ratio, etc. The earlier equation developed was Abrams formula which describes the dependence of concrete strength on W/C ratio. In addition to this, the power formula is considered as one of the most useful formulae in the field of concrete technology.
CS of bacterial concrete is influenced by many factors, including bacteria solution to water ratio, cement content, bacterial dosage, aggregate type, age, etc. This paper proposes a simple and direct forward method to predict bacterial concrete CS with variation in bacteria solution to water ratio and curing age.
For the prediction of CS of BBFC, CS is considered as a dependent variable, while the BS/W and curing time are independent variables (). A predictive model of CS, as derived by MRA is given below with R2 value of 0.886. The high value of coefficient of determination (R2 = 0.886) indicates a good correlation between CS and bacteria solution to water ratio. These equations show that CS is a function of bacteria solution to water ratio.
Y = compressive strength (MPa)
X = curing period
B/W = bacterial solution to water ratio.
3.5. Sorptivity test
Sorptivity tests for bacteria blended FA concrete were carried out at the age of 28 and 60 days. The results of the test are shown in . The sorptivity for bacterial concrete was found to be low in comparison with normal concrete. The minimum sorptivity was observed in BBFC containing 10% FA at all ages. For BBFC containing 20% and 30% FA sorptivity was found to be slightly higher than 10% BBFC, but less than normal concrete. Sorptivity in normal concrete was 5.33 and 4.92 mm/min1/2 and in 10% BBFC was 3.18 and 2.76 mm/min1/2,respectively. Capillary water absorption of BBFC was significantly reduced in the range 30–60% at 28 and 60 days. The addition of bacteria of cell concentration 105 cells/mL resulted in a significant decrease in sorptivity compared to normal concrete.
Table 8. Sorptivity values for of FA concrete with and without bacteria.
The reduction in sorptivity of BBFC may be attributed to porosity and micro-cracking plugged by CaCO3 precipitation (Jonkers et al. Citation2010) presence of organic matters in bacteria-induced CaCO3 reduce wettability of BBFC surface. This bacterial action seals the pores and voids in the matrix of concrete which reduces sorptivity property. Hence, from this experiment, it shows that the presence of calcium carbonate crystals in the pores and void has the ability to improve the resistance of cementitious materials towards degradation.
3.6. Relationship between CS and sorptivity
The multiple linear equation expressing the relationship between sorptivity, CS, FA in per cent and curing period together with the coefficients of determination (R2) is given in the following:
where
δ – sorptivity in mm/min1/2,
β = compressive strength in MPa
γ = curing period, h
α = %FA.
A high value of coefficient of determination (R2 = 0.783) indicates good relevance between the data points and regression curve. The above equation helps to predict sorptivity with variation in strength, curing period and FA composition. It is evident from the above equation that the higher is the CS of the concrete, lower is the sorptivity.
3.7. SEM analysis
shows the microstructure and surface morphology of plain concrete, BC and BBFC of 28 days samples. As can be noted in ), the surface of plain concrete is porous with high irregularity. As shown in ,), the surface of BC is composed of crystals of spherical shapes. ) the surface of BBFC is covered with calcite layer. Calcite crystals observed in all bacterial blended concrete actas a barrier to harmful substances and thus are responsible for overall improved property of concrete. Similar observations were noticed by Siddique et al. (Citation2016) and Kirti et al.(Citation2016).
3.8. X-ray diffraction (XRD)
X-ray analyses of the samples () with and without bacteria show that there were some extra peaks in the XRD pattern of the bacteria specimens, which are absent in the specimens without bacteria. In ) as shown on the XRD pattern, the highest peak was observed at 2 theta (2 h) value of 29.37° for bacterial concrete (both the bacteria) at cell concentration of 105 cells/mL which is close to 2 theta of 29.455° of pure calcite which was also observed by Harrington (Citation1927), Khaliq (2010) and Kirti (2012). This approves the presence of calcite crystals in bacteria concrete immersed in tap water in the absence of nutrients. The components of concrete specimens were recognised by comparing with standards developed by the International Centre for Diffraction Data (Chahal, Siddique, and Rajor Citation2012). XRD spectra of normal samples showed no extra peaks. Calcite formation as confirmed by XRD analysis is considered responsible for improving the property of concrete specimens.
4. Conclusion
The following conclusions may be drawn from the experimental investigation of the present study:
Strength gain in case of bacteria incorporated specimens of various cell concentrations were found to be predominant at early age of curing.
The results presented herein demonstrate the feasibility of using the biomineralisation process for producing a durable sustainable FA concrete.
Bacterial cells addition in FA concrete further improved its CS and durability properties. Optimum dosage of FA as cement replacement in concrete was 10%.
SEM and XRD results showed that the addition of bacteria B. spahericus of various dosages densified the matrix of concrete.
Application of microbiology along with FAup to 30% replacement level shows a promising combination in the construction sector which thereby greatly enhances the service life of concrete. Development of sustainable bacteria embedded FA concrete by novel biotechnology method provides greener and economic options.
Acknowledgments
The authors would like to thank the management authorities of KLE Dr. M.S. Sheshgiri College of Engineering and Technology, Belgaum for their kind support. The authors are grateful to Dr. Basavaraj G. Katageri for giving all the encouragement needed which kept the enthusiasm alive.
Disclosure statement
No potential conflict of interest was reported by the authors.
Additional information
Notes on contributors
Santosh A. Kadapure
Dr. Santosh A. Kadapure is working as an associate professor in the department of chemical engineering at KLE Dr. M.S. Sheshgiri College of Engineering and Technology, Belagavi. He has widely published research papers on bacterial concrete and biodiesel production in various national and international journals.
Girish Kulkarni
Dr Girish kulkarni has graduated in Civil Engineering, post graduated in Environmental Engineering and pursued PhD from Shivaji University Kolhapur. He is working as Deputy Registrar at Shivaji University Kolhapur. He has published and presented many papers in international and national conferences.
K.B Prakash
K.B Prakash is currently working as Principal at Government Engineering College, Haveri, and Karnataka. He has published and presented many papers in international and national conferences.
Poonam S. Kadapure
Poonam S. Kadapure is working as lecturer in the Department of BBA, Rani Parvati Devi College of Arts and Commerce, Belagavi, India. She has published and presented papers international and national conferences and has a experience of 6 years.
References
- Abalaka, A. E. 2013. “Strength and Some Durability Properties of Concrete Containing Rice Husk Ash Produced in a Charcoal Incinerator at Low Specific Surface.” International Journal of Concrete Structures and Materials 7 (4): 287–293. doi:10.1007/s40069-013-0058-8.
- Achal, V., A. Mukherjee, and S. Reddy. 2010. “Microbial Concrete: A Way to Enhance Durability of Building Structures.” Journal of Materials in Civil Engineering. Jan.38: 1229–1234.
- Andalib, R., M. Z. Majid, M. W. Hussin, M. Ponraj, M. Jahangir, A. K. H. S. Mirza, H. S. P. Lee, et al. 2016. “Formations of Calcium Carbonate Minerals by Bacteria and Its Multiple Applications.” SpringerPlus 5: 250. doi:10.1186/s40064-016-1869-2.
- Barbhuiya, S. A. 2009. “Properties of Fly Ash Concrete Modified with Hydrated Lime and Silica Fume.” Construction and Building Materials 23 (10): 3233–3239. doi:10.1016/j.conbuildmat.2009.06.001.
- Chahal, N., and R. Siddique. 2013. “Permeation Properties of Concrete Made with Fly Ash and Silica Fume: Influence of Ureolytic Bacteria.” Construction and Building Materials 49: 161–174. doi:10.1016/j.conbuildmat.2013.08.023.
- Chahal, N., R. Siddique, and A. Rajor. 2012. “Influence of Bacteria on the Compressive Strength, Water Absorption and Rapid Chloride Permeability of Concrete Incorporating Silica Fume.” Construction and Building Materials 37: 645–651. doi:10.1016/j.conbuildmat.2012.07.029.
- Ghosh, P., and S. Mandal. 2006. “Development of Bio-concrete Material Using an Enrichment Culture of Novel Thermophilic Anaerobic Bacteria.” Indian Journal of Experimental Biology 44 (4): 336.
- Ghosh, S., M. Biswas, C. B D, and S. Mandal. 2009. “Microbial Activity on the Microstructure of Bacteria Modified Mortar.” Cement & Concrete Composites 31: 93–98. doi:10.1016/j.cemconcomp.2009.01.001.
- Halder, B., D. Roy, V. Tandon, C. Ramana, and A. Tarquin. 2014. “Use of Mutated Micro-Organism to Produce Sustainable Mortar.”ACI Materials Journal 111 October. doi:10.14359/51686597.
- Harrington, E. A. 1927. “X-ray Diffraction Measurements on Some of the Pure Compounds Concerned in the Study of Portland Cement.” American Journal of Science 78: 467–479. doi:10.2475/ajs.s5-13.78.467.
- Jianyun, W., K. V. Tittelboom, W. Belie, and D. N. Verstraete. 2012. “Use of Silica Gel or Polyurethane Immobilized Bacteria for Self-healing Concrete.” Construction and Building Materials 26: 532–540. doi:10.1016/j.conbuildmat.2011.06.054.
- Jonkers, H., and E. Schlangen. 2009. “A Two Component Bacteria-based Self-healing Concrete.” In Mark G. A (ed.) Concrete Repair, Rehabilitation and Retrofitting II. London: Taylor & Francis Group. Alexander et al 2009. ISBN 978-0-415-46850-3.
- Jonkers, H. M. A., A. Thijssen, G. Muyzer, O. Copuroglu, and E. Schlangen. 2010. “Application of Bacteria as Self-healing Agent for the Development of Sustainable Concrete.” Ecological Engineering 36 (2): 230–235. doi:10.1016/j.ecoleng.2008.12.036.
- Ramachandran, K. S., K. Santhosh, V. Ramakrishnan, and S. Bang. 2001. “Remediation of Concrete Using Microorganisms.” ACI Materials Journal 98 (1): 3–9.
- Khaliq, W., and M. Ehsan. 2016. “Crack Healing in Concrete Using Various Bioinfluenced Self-healing Techniques.” Construction and Building Materials 102: 349–357. doi:10.1016/j.conbuildmat.2015.11.006.
- Kim, H. K. 2013. “Microbially Mediated Calcium Carbonate Precipitation on Normal and Lightweight Concrete.” Construction and Building Materials 38: 1073–1082. doi:10.1016/j.conbuildmat.2012.07.040.
- Kirti, K., M. Arakha, P. Sarkar, R. P. Davis, and S. Jha. 2016. “Enhancement of Properties of Recycled Coarse Aggregate Concrete Using Bacteria.” International Journal of Smart and Nano Materials 47 (1): 22–38.
- Krishnapriyaa, S., D. L. Venkatesh Babu, and P. G. Arul Raj. 2015. “Isolation and Identification of Bacteria to Improve the Strength of Concrete. Isolation and Identification of Bacteria to Improve the Strength of Concrete.” Microbiological Research 174: 48–55. doi:10.1016/j.micres.2015.03.009.
- Kwangryul, H., T. Noguchi, and F. Tomosawa. 2004. “Prediction Model of Compressive Strength Development of Flyash Concrete.” Cement and Concrete Research 34 (12): 2269–2276. doi:10.1016/j.cemconres.2004.04.009.
- Muynck, W. D., N. D. Debrouwer, and W. Verstraete. 2008. “Bacterial Carbonate Precipitation Improves the Durability of Cementitious Materials.” Cement and Concrete Research 38 (7, July): 1005–1014. doi:10.1016/j.cemconres.2008.03.005.
- Pacheco-Torgal, F., and J. A. Labrincha. 2013. “Biotech Cementitious Materials: Some Aspects of an Innovative Approach for Concrete with Enhanced Durability.” Construction and Building Materials 40: 1136–1141. doi:10.1016/j.conbuildmat.2012.09.080.
- Periasamy, A., K. C H, Y. Shin, and J. Seong So. 2016. “Formations of Calcium Carbonate Minerals by Bacteria and Its Multiple Applications.” SpringerPlus 5 (1).
- Ramakrishnan, V., P. R K, and S. S. Bang 2005. “Improvement of Concrete Durability by Bacterial Mineral Precipitation.” ICF11, Italy. 2013.
- Ruiyang, L., and H. Rong. 2015. “Efficiency of Concrete Crack-healing Based on Biological Carbonate Precipitation.” Journal of Wuhan University of Technology-Mater. Sci. Ed 30 (6): 1255–1259. doi:10.1007/s11595-015-1304-5.
- Sathawane, S. H., V.S. Vairagade, and K. S. Kene. 2013. “Combine Effect of Rice Husk Ash and Fly Ash on Concrete by 30% Cement Replacement.” Procedia Engineering 51: 35–44. doi:10.1016/j.proeng.2013.01.009.
- Seifan, M., A.K. Samani, and A. Berenjian. 2017. “New Insights into the Role of pH and Aeration in the Bacterial Production of Calcium Carbonate (caco3).” Applied Microbiology and Biotechnology 101: 3131–3142. doi:10.1007/s00253-017-8109-8.
- Shetty, M. S. 2005. Concrete Technology, 420–453. S. Newdelhi, India: chand & company .
- Siddique, R., A. Jameel, M. Singh, D. Barnat-Hunek, D. Kunal, A. Mokhtar, B. Rafik, and A. Rajor. 2017. “Effect of Bacteria on Strength, Permeation Characteristics and Micro-structure of Silica Fume Concrete.” Construction and Building Materials 142: 92–100. doi:10.1016/j.conbuildmat.2017.03.057.
- Siddique, R., K. Singh, Kunal, M. Singh, V. Corinaldesi, and A. Rajor. 2016. “Properties of Bacterial Rice Husk Ash Concrete.” Construction and Building Materials 121: 112–119. doi:10.1016/j.conbuildmat.2016.05.146.
- Talaiekhozani, A., A. Keyvanfar, R. Andalib, M. Samadi, A. Shafaghat, H. Kamyab, A. M. M Z, et al. 2014. “Application of Proteus Mirabilis and Proteus Vulgaris Mixture to Design Self-healing Concrete.” Desalination and Water Treatment 52: 3623–3630. doi:10.1080/19443994.2013.854092.
- Thanh, L. H., T. Nguyen, and H. M. Ludwig. 2014. “A Study on High Performance Fine- Grained Concrete Containing Rice Husk Ash.” International Journal of Concrete Structures and Materials 8 (4): 301–307. doi:10.1007/s40069-014-0078-z.
- Wang, J., J. H M, N. Boon, and N. D. Belie. 2017. “Bacillus Sphaericus LMG 22257 Is Physiologically Suitable for Self-healing Concrete.” Applied Microbiology and Biotechnology 56: 1–14.
- Wang J Y, Verstraete W Soens, and Belie N De. 2014. “Self-healing Concrete by Use of Microencapsulated Bacterial Spores.” Cement and Concrete Research 56: 139–152. doi:10.1016/j.cemconres.2013.11.009.
- Zhu, T., and M. Dittrich. 2016. “Carbonate Precipitation through Microbial Activities in Natural, Environment, and Their Potential in Biotechnology: A Review.” Frontiers in Bioengineering and Biotechnology 4. doi:10.3389/fbioe.2016.00004.