ABSTRACT
The low freshwater output is always a matter of concern regarding a solar still. It is observed from the current literature that evacuated tubes are directly connected to the still basin for productivity enhancement. The main limitation of integrating evacuated tubes directly to solar still is rapid scaling on the inner surfaces of tubes due to the presence of dissolved impurities in water. This increases thermal resistance and decreases the overall performance of the still. In the present experimental study, a copper tube heat exchanger is installed in still basin and waste engine oil is utilised as a working fluid in the evacuated tube collector (ETC) and heat exchanger circuit. This modification increases the evaporation rate of basin water and boosts freshwater output. The modified still is experimentally investigated at distinct water depths of 4 cm, 5 cm and 6 cm, and the performance is simultaneously compared with a conventional still. Experimental results showed maximum daily productivity of 7.38 L/m2 day and daily efficiency of 30.5% achieved for modified still at 4 cm water depth. These values are 138.9% and 2.1% higher than that of conventional still respectively at same water depth.
1. Introduction
Water is very precious resource on earth that should be used wisely and managed accordingly for sustainable development. In India, water shortage is due to increasing population, large scale water consumption, inadequate planning and infrastructure. Moreover, people are using water recklessly to fulfil their daily requirements. Water desalination becomes extremely important in mitigating this potable water crisis and satisfying drinking water requirements of people of this country. Water distillation using solar still is one of the simplest and low-cost techniques. Major drawback of solar still is the low potable water productivity. A simple basin type solar still has a productivity of 3–4 kg/m2 day, which is scant to meet the existing demands. Various modifications are employed in a solar still by different researchers to enhance its productivity. These modifications include use of fins, wicks, thermal energy storage materials and internal external reflectors in the still. Utilising nano-fluids and different absorber configurations in the still basin also resulted in productivity enhancement. Solar still integrated with different solar collectors also resulted in productivity enhancement to a considerable extent. Solar collectors preheat the saline water thereby enhancing the evaporation rate. Singh and Yadav et al. (Citation2016) integrated two photo-voltaic thermal (PVT) collectors to a simple basin type solar still. The PVT collector consists of PV module to generate electricity and copper pipes to preheat feed water entering the still. This system generates electricity and increases the potable water productivity from the still. Gaur and Tiwari (Citation2010) optimised the total PVT collectors used and achieved maximum productivity using four collectors. Kumar and Tiwari (Citation2010) performed a comparative study of a simple basin type and PVT integrated still working under forced circulation mode. The experimental results showed the productivity of 7.22 kg/m2 day for a PVT integrated still which was 313% more than basin type still. Kabeel and Abdelgaied (Citation2017) designed a parabolic trough concentrator (PTC) integrated solar still and experimentally compared its performance with a basin type still. The results showed 140.4% increase in freshwater productivity of modified still. Appadurai and Velmurugan (Citation2015) enhanced the still productivity by 50% on integrating it with a solar pond. Eltawil and Omara (Citation2014) integrated a flat plate collector (FPC), solar air heater and an externally applied condenser to a basin type still. Still is modified with water spraying unit powered by PV panels. The maximum productivity increase of 148% is observed in the experimental results. Kabeel and Abdelgaied et al. (Citation2016) developed a still with an arrangement of hot air injection in basin water. The air is heated using a solar air collector with double pass arrangement. Phase change material (PCM) is also used to enhance the productivity during evening hours. The maximum freshwater productivity reached to 9.36 L/m2 day using both these modifications. Shafii and Shahmohamadi et al. (Citation2016) developed a still in which latent heat of condensing vapours is utilised in thermoelectric modules integrated to the still for electricity generation. The electricity produced is used in operating a propeller fan. The maximum hourly efficiency of 68% is achieved during the experiments conducted at different water depths. The latent heat of condensing vapours is used in charging PCM resulting in 86% productivity enhancement (Faegh and Shafii Citation2017). Black granite pebbles are used as heat storage material in a double slope solar still integrated with evacuated tube collector (ETC) (Panchal Citation2015). The results showed 65% increase in productivity. Shafii and Mamouri et al. (Citation2016) used evacuated tubes as solar collector and basin to dispel the thermal resistance between basin and collector. The results showed maximum productivity of 0.83 kg/m2 h. The still productivity increased further by 21.6% when evacuated tubes are filled with stainless steel wool. Badran and Al-Tahaineh (Citation2005) experimentally investigated the impact of integrating an FPC on still productivity at different water depths. Mirrors are used as internal reflectors fixed on the inner walls of the still. The results showed 36% increase in still productivity when augmented with an FPC. Arunkumar and Denkenberger et al. (Citation2013) made a solar still with hemispherical basin by integrating it with a parabolic concentrator. PCM filled balls made of copper are placed inside the hemispherical basin to enhance the still performance. Using PCM filled balls and parabolic concentrator increased the productivity up to 26%. Singh (Citation2018) compared ETC integrated solar still with PVT and compound parabolic concentrator integrated solar still. It was observed that ETC integrated solar still performed better in comparison to others in terms of payback time and cycle conversion efficiency. Same result trend was observed when the above mentioned collectors are used with a double slope still (Singh and Al-Helal Citation2018). Singh and Kumar et al. (Citation2013) analysed the effect of using ETC on single slope still performance at 3 cm water depth. Maximum productivity of 3.8 kg/m2 is observed using 10 evacuated tubes working on thermosyphon.
In the existing literature, evacuated tubes are mostly directly attached to the still basin. The saline water is heated and circulated from the tubes to basin by natural convection. This leads to the rapid scale formation on the inner surface of tubes due to the presence of dissolved salts in saline water. The heat transfer rate and still output is thus reduced. Frequent cleaning of evacuated tubes from inside is also required for effective functioning. Therefore, the prime objective of this study is to increase the productivity of still by enhancing the heat transfer rate to the basin water using a heat exchanger. In the present experimental study, a tube type heat exchanger is installed in the still basin and oil is used as a working fluid in the circuit to overcome this problem. Also, restricted studies were conducted on ETC integrated solar still operating on natural convection with a heat exchanger installed in the still basin. Moreover, the experimental performance of this heat exchanger modified active solar still is compared with conventional still. All experiments were performed under same meteorological conditions at 4cm, 5 cm and 6 cm water depths. The real application of this ETC integrated active solar still lies in remote areas of the world. It is meant for the people living in remote areas where access to electricity is very limited. Most of the desalination devices are electrically driven and also uneconomical to the people living in remote areas. Therefore, solar still is mostly well suited in the remote areas having minimal electricity supply and abundant thermal energy.
2. Experimental set-up
In the present work, two single slope stills of same bottom plate area and material are designed and fabricated for the experimental testing. One still is modified by integrating with ETC and tubular heat exchanger installed in still basin. Experiments are performed in the month of June, 2018 on the terrace of AB block, NIT Kurukshetra having coordinates (29.9490° N, 76.8173° E). Tubular heat exchanger and basins of both stills are painted matt black for utmost solar radiations absorption. Glass wool insulation is used to minimise the energy losses from bottom plate and side walls of still to surroundings. Glass condensing cover is inclined at the latitude angle of location. A storage tank of capacity 50 L is utilised to maintain the desired water depth throughout the experiments. Separate openings are provided in both the stills for saline water inlet, hot brine outlet and collecting freshwater. To prevent any vapour leakage from the still, silicon is used as a sealant. In the modified still, header of ETC is connected to the still by suitable piping arrangement forming a loop consisting of header inlet, outlet pipes and copper tube heat exchanger. Due to good thermo-physical properties and easy availability, waste engine oil from automobiles is used as HTF. The HTF is heated and circulated in the loop by thermosyphon principle. The HTF at high temperature flows through the heat exchanger by natural circulation and exchanges heat with basin water. An expansion tank is fixed at heat exchanger inlet to release extra pressure developed in the pipes. Iron stands are used to hold and support solar still and ETC. The schematic diagram of set-up is shown in and photograph is shown .
The experimental data is recorded and noted down from 9am to 7pm. The heat exchanger is inclined at 10° for the smooth and efficient functioning of thermosyphon. A minimum water depth of 4 cm is required to ensure complete immersion of heat exchanger in basin water. The experiments are conducted at distinct water depths of 4 cm, 5 cm and 6 cm. The results are obtained in the form of water temperature, hourly and accumulative productivity. Different specifications of the experiment set-up are elaborately mentioned in .
Table 1. Various specifications of experiment set-up.
3. Thermal performance
The four main components of modified still are basin bottom plate, basin water, inclined glass cover and ETC. In this part, the energy balance equations for all modified still components are formulated. Following are the assumptions made before formulating the equations and simplification of analysis:
Leakage of vapours from the still is negligible.
Heat capacity of condensing cover, bottom plate, side walls and insulation is negligible.
Only film condensation occurs at glass inner surface.
Basin water depth or water mass is constant.
No temperature gradient exists in the inclined glass cover.
Energy losses from side walls of still are negligible.
The temperature of water inside ETC is an average of outlet and inlet water temperatures.
The equations for main components of modified still are presented hereunder (Badran and Abu-Khader Citation2007):
Bottom plate of still basin:
The energy balance equation for the basin liner is presented as:
(1)
Mass of water inside still:
The energy balance equation on water mass in solar still presented by Duffie and Beckman (Citation2013) is as follows:
(2)
where subscripts g, c, t, b, w and e represent glass, cover, time, basin, water and evaporation, respectively. The subscripts cond, rad and conv represent conduction, radiation and convection, respectively. Expanding EquationEq. (2
(2) ) gives the following expression:
(3)
Where, h is heat loss coefficient, hwe is heat loss coefficient of evaporation for water surface and determined by the following expression:
(4)
Where, Psw is the partial pressure of saturated water and is calculated using following formula:
(5)
and Psg is the partial pressure of saturated glass cover and is calculated by the following formula:
(6)
hwr is heat loss coefficient of radiation for basin water and its value can be calculated by the following expression:
(7)
Where,
is the total effective emissivity and is calculated by the following formula:
(8)
hwc is heat loss coefficient of convection for water surface and can be determined by using the following formula:
(9)
Inclined glass cover:
Energy equation for glass cover is presented as:
(10)
Where, hgr is radiative heat loss coefficient for glass surface and calculated using following formula:
(11)
Water present in ETC:
Energy equation for water inside ETC is presented by Singh and Kumar et al. (Citation2013) as:
(12)
To solve these equations, values of Tsb from EquationEq. (1(1) ) and Tgc from EquationEq. (3
(3) ) and putting these values in EquationEq. (10
(10) ), we get:
Where,
is the total effective fraction heat absorbed in given time interval and is represented by the following expression:
UT is total overall heat loss coefficient represented as the sum of overall heat loss coefficient for still basin (Usb) and glass cover (Ug).
Where, Ug is calculated using the mentioned formula:
Where, hwt total heat loss coefficient of water surface and is represented as:
and hgt is total heat loss coefficient of glass surface and is represented as:.
The analytical solution to these equations gives following result in the form of instantaneous efficiency of single slope solar still as:
Tubular heat exchanger analysis:
Rate of heat exchange,
Neglecting fouling factor, the value of U is calculated using following formula:
Where Aie, Aoe and A are inner, outer area of heat exchanger and total heat exchange area, respectively.
4. Instrumentation and uncertainty analysis
Thermocouples of PT-100 type are installed at different locations of both the stills to measure the temperature values. These include temperatures of header inlet, header outlet, heat exchanger inlet, outlet, basin water and glass cover. The accuracy of PT-100 thermocouples used is ±0.1°C with the capacity to measure temperatures up to 200°C. A digital temperature indicator is used to indicate the temperatures measured at respective points. Pyranometer with ±2% accuracy and 1 W/m2 resolution is used to measure the solar intensity up to 5000 W/m2. Hourly distillate output is measured with a calibrated cylindrical flask of ±1 mL accuracy and 2000 mL capacity. The uncertainty in experimental measurements is contributed by fixed errors and random errors. Bell (Citation1999) and Nakra and Chaudhry (Citation2003) presented an expression to calculate the standard uncertainty in measurements. The expression is presented as follows:
Where, is the deviation from mean, Sn is number of sets, So is total observations in a set. Total uncertainty or total error of ±3.9% is calculated during the experiments using the formula presented by Singh and Yadav et al. Citation2016) as:
The total uncertainty in the measurement of freshwater productivity (mh) is ±0.75% and the total uncertainty or percentage error in the measurement of solar radiation intensity (I) using Kipp and Zonen Pyranometer with Model no. CM11 is ±2%. Using equations of uncertainty analysis the total uncertainty in the efficiencies of conventional and modified solar stills are calculated as ±0.9% and ±1.3%, respectively.
5. Results and discussions
5.1 Solar intensity
The intensity of solar radiations had an appreciable effect on still performance. The glass cover and ETC in the experiment set-up are inclined at same angle (30°). Therefore, the intensity of solar radiations received by the ETC and glass cover is also equal. To measure the global horizontal irradiation (GHI) accurately, the pyranometer is also aligned with the ETC and glass cover of still. Hence, solar intensity variation as shown in is same for the both ETC and glass cover. The maximum values of solar intensity are observed at 2pm on all testing days as shown in . The ambient temperature varies accordingly with solar intensity attaining maximum value at time of maximum intensity.
Intensity increases rapidly in the morning reaching maximum value in the afternoon and gradually decreases till the evening. The water and glass cover temperature attains maximum values at around 2pm in all cases as shown in and . This is due to the availability of maximum solar intensity and heat absorption. Basin water in the modified still reached highest temperature of 87.5°C at 4 cm water depth which is 17.7°C higher than the conventional still. This result is in accordance with the maximum water temperature of 84°C as obtained using evacuated tubes by Sampathkumar and Senthilkumar (Citation2012).
Water temperature in both the stills observed a steep rise in the morning and afternoon hours. It is also observed that the water temperature in the modified still decreases at a lower rate till the evening. This is due to high heat storage capacity of HTF to maintain high temperatures even in the hours of low intensity.
5.2 Water depth
Water depth influences the freshwater productivity to a good extent. Lower water depth results in high water temperature with increased evaporation due to speedy heating of less amount of water. The results from experiments showed maximum values of different parameters obtained at 4 cm water depth for both stills. These parameters include water temperature, hourly productivity, daily productivity, hourly efficiency and daily efficiency. This result is in accordance with the results obtained by Taghvaei and Taghvaei et al. (Citation2014) and Elango and Murugavel (Citation2015) as they have also achieved maximum productivity at minimum possible water depth.
5.3 Hourly productivity and efficiency
The hourly productivity variation with time for all cases is shown in and variation of accumulative productivity with time is shown in . The hourly productivity for a modified still at all water depths is low in the morning but increases to high values in the afternoon and afterwards. This is because effective working of thermosyphon in the loop starts after 11am and continued till the evening hours.
The variation of hourly efficiency with time for all water depths as shown in also follows the same trend as productivity is low during morning. also shows higher hourly efficiency values in afternoon and evening hours due to high productivity values. The highest hourly productivity and hourly efficiency for all cases are obtained at 2pm due to the absorption of maximum solar radiations by the basin water and ETC. The maximum hourly productivity of 487 mL is achieved for a modified still at 4 cm water depth. This value is 20.2% (405 mL), 26.4% (385 mL), greater than the hourly productivity at 5 cm and 6 cm water depths. The maximum accumulative productivity of 2659 mL (7.38 L/m2 day) is obtained from the modified still. This is 18.2% (2249 mL), and 32.2% (2010 mL) greater than accumulative productivity at 5 cm, and 6 cm water depths. The efficiencies of conventional and active still are calculated using following formulas:
The area of ETC is calculated as follows:
Where, is the outer diameter of evacuated tube and,
is the length of evacuated tube.
The maximum hourly efficiency of 41.2% is achieved for a modified still at 4 cm water depth which is 9.6%, and 11% greater than modified still operating at 5 cm, and 6 cm water depths. The highest values of 49.9%, 40.5% and 37.2% is achieved for a conventional still at 4 cm, 5 cm and 6 cm water depths, respectively. Maximum daily efficiency of 30.5% is achieved for modified still at 4 cm depth which is 3.6%, and 6.9% greater than 5 cm, and 6 cm water depth. In case of conventional still, maximum daily efficiency of 28.4% is observed at 4 cm water depth. The values of daily overall efficiencies of modified and conventional still at all water depths are shown in .
5.4 Heat exchanger
HTF is heated to high temperatures in ETC and circulated by natural convection from the heat exchanger installed in still basin. HTF at high temperature increases the basin water temperature and evaporation rate resulting in higher productivity and efficiency. The hourly inlet outlet temperature variations of heat exchanger for modified still is shown in . The highest temperatures observed at heat exchanger inlet are almost the same at all depths. This is because of nearly similar solar radiation intensities received by ETC on different testing days. The effectiveness of heat exchanger () for all different cases is calculated by the following formulae:
Figure 10. Oil inlet and outlet temperature variations in heat exchanger with time for modified still on different testing days.
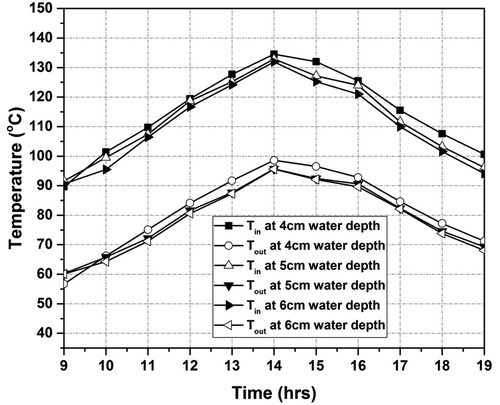
where, is the mass flow rate of oil in heat exchanger pipe,
is oil specific heat,
is oil temperature at heat exchanger inlet, and
is oil temperature at heat exchanger outlet. shows the variation of heat exchanger effectiveness with time in case of modified still at all water depths.
The effectiveness mainly depends on oil temperature at heat exchanger outlet and basin water temperature as the oil temperature at heat exchanger inlet is nearly the same in all cases. In case of modified still at 4 cm depth, the temperatures of oil and water at exchanger outlet are higher due to efficient heat transfer at low depth. Therefore, heat exchanger effectiveness values are maximum at 4 cm water depths.
5.5 Comparison of modified and conventional still
Modified still utilises heat from ETC as well as solar radiations, therefore resulting in better freshwater productivity in comparison to a conventional still. Also, the rate of increase in distillate output is more in proportion to rate of increase in heat input in case of modified still. This leads to the higher efficiency of modified still than conventional still. Due to higher input energy available, a meagre rise in daily efficiency is observed for a modified still at all depths. The maximum hourly and accumulative productivity of modified still is 107.2% and 138.9% greater than conventional still at 4 cm depth. The maximum hourly efficiency of conventional still is 8.7% higher than modified still at 4 cm depth. But, the daily efficiency of modified still is 2.1% greater than conventional still at 4 cm water depth.
6. Cost analysis
In this section, a cost analysis of both solar stills is performed to calculate the cost of freshwater output per litre. The days of full sunshine are assumed to be 305 days in a year. The approximated costs of items used in the construction and installation of modified still are mentioned in . The costs of different items used in construction of conventional still are mentioned in . The increased initial cost of modified still is mainly due to the use of ETC.
Table 2. The costs of distinct items used in fabrication of the modified still.
Table 3. The costs of distinct items used in fabrication of the conventional still.
The first annual cost of a still (Z) is calculated by Goosen and Sablani et al. (Citation2000) as follows:
Where, P is capital cost of the still and CRF is the capital recovery factor, which is expressed by Goosen and Sablani et al. (Citation2000) as follows:
Where, r is the interest rate in (%) of lending banks which varies with location and n is the average life (in years) of the still. The average life of both stills is 15 years. Salvage value is defined as the approximated value of an item after the end of its utility life and it is usually 50% of the usable item (Goosen and Sablani et al. Citation2000). The salvage value of the still is calculated annually using the formula given by Goosen and Sablani et al. (Citation2000) and is expressed as follows:
Where, SVannual is the annual salvage value of the still, SFF is the sinking fund factor which is determined by using a relation given by Goosen and Sablani et al. (Citation2000) as:
and Sv is salvage value of the still which is calculated by using the formula given by Rahbar and Esfahani (Citation2012) as:
The total annual cost of the still Cannual comprises of the first annual cost (Z), the annual maintenance cost (MCannual) and the annual salvage value (SVannual) of the system. The relationship between these parameters was established by Goosen and Sablani et al. (Citation2000) and is expressed as follows:
The annual maintenance cost (MCannual) of the still is determined by Rahbar and Esfahani (Citation2012) as follows:
Now, the cost of freshwater produced per litre (CPL) from the solar still was calculated by Rahbar and Esfahani (Citation2012) as follows:
Where, Paverage is the average annual freshwater production. shows the calculated values of all the parameters mention in cost analysis. The cost per litre of conventional still is calculated as 0.0084$ and that of modified still as 0.0136$, respectively using above analysis.
Table 4. Different parameter values obtained in the cost analysis of conventional still and modified still.
7. Conclusions
In this experimental study, the impact of using heat exchanger in ETC integrated active solar still is experimentally investigated and compared with a passive single slope still at 4 cm, 5 cm and 6 cm water depths. The experimental comparison on the basis of hourly productivity, water temperature, hourly and daily efficiency has been performed for same meteorological conditions. The important outcomes and conclusions of this experimental study are expressed hereunder:
The use of tube heat exchanger in still basin decreases the scale deposition inside evacuated tubes. This further decreases the thermal resistance and enhances the evaporation rate and productivity.
The solar intensity and ambient temperature values are observed to be maximum at 2pm on all testing days.
The water temperature, hourly productivity, accumulative productivity, hourly efficiency and heat exchanger effectiveness attained maximum values at 2pm and in case of 4 cm water depth.
A direct dependence of these parameters with solar intensity is observed during the experimentation. These parameters attained maximum values at the time of around maximum solar intensity.
The maximum hourly productivity of 487 mL is observed in the case of modified still at 4 cm water depth. It is 20.2%, 26.4% and 107.2% higher than modified still at 5 cm, 6 cm and conventional still, respectively.
The maximum accumulative productivity of 2659 mL is obtained for modified still at 4 cm depth. It is 18.2%, 32.2% and 138.9% higher than modified still at 5 cm depth, 6 cm depth and conventional still, respectively.
The daily efficiencies of 30.5%, 26.9% and 23.6% is calculated in case of a modified still at 4 cm, 5 cm and 6 cm water depths.
The hourly efficiency of conventional still is observed to be 8.7%, 8.1% and 7% higher than modified still at 4 cm water depth.
Heat exchanger effectiveness for a modified still at 4 cm water depth is calculated as 0.58, which is highest in comparison to other cases.
The cost per litre of conventional still is 0.0084$ and that of modified still as 0.0136$, respectively.
Nomenclature
= | Coefficient of evacuated tube collector efficiency. | |
= | Still basin area (m2). | |
A | = | Heat exchanger area (m2). |
= | Area of evacuated tube collector (m2). | |
= | Inner area of ETC (m2). | |
= | Outer area of ETC (m2). | |
= | Water specific heat (J/kg K). | |
= | Oil specific heat (J/kg K). | |
dt | = | Time interval, s. |
= | Heat exchanger outer dia (m). | |
= | Heat exchanger inner dia (m). | |
= | Overall heat loss coefficient of still basin (W/m2K). | |
= | Total heat loss coefficient of water surface (W/m2 K). | |
= | Total heat loss coefficient of glass surface (W/m2 K). | |
= | Latent heat of water vapour (J/kg). | |
= | Solar radiation intensity falling on evacuated tube collector (W/m2). | |
= | Solar radiation intensity at a given time (W/m2). | |
K | = | Thermal conductivity of heat exchanger (W/mK). |
L | = | Length of heat exchanger (m). |
= | Water mass in still basin (kg). | |
= | Rate of water circulation by free convection trough one evacuated tube (kg/s). | |
= | Oil flow rate in heat exchanger pipe (kg/s). | |
= | Water mass present in evacuated tubes (kg). | |
= | Hourly freshwater productivity (kg/h). | |
= | Total number of evacuated tubes used. | |
= | Partial pressure of saturated water (N/m2). | |
= | Partial pressure of saturated glass cover (N/m2). | |
= | Conduction heat loss (W/m2). | |
= | Convection heat loss from basin water to glass cover (W/m2). | |
= | Evaporation heat loss from basin water to glass cover (W/m2). | |
= | Radiation heat loss from basin water to glass cover (W/m2) | |
= | Evaporation loss rate from water surface (W/m2). | |
= | Ambient temperature (K). | |
= | Time (s). | |
= | Temperature of glass cover (K). | |
= | Heat loss coefficient inside heat exchanger (W/m2 K). | |
= | Heat loss coefficient for water surface (W/m2 K). | |
= | Heat loss coefficient outside heat exchanger (W/m2 K). | |
= | Evaporative heat loss coefficient for water surface (W/m2 K) | |
= | Radiative heat loss coefficient for glass surface (W/m2 K). | |
= | Convective heat loss coefficient for glass surface (W/m2 K). | |
= | Convective heat loss coefficient of still basin to water (W/m2 K). | |
= | Radiative heat loss coefficient of basin water (W/m2 K). | |
= | Temperature of sky (K). | |
= | Overall energy coefficient (W/m2 K). | |
U | = | Overall heat loss coefficient of heat exchanger (W/m2 K). |
= | Overall heat loss coefficient of top glass cover (W/m2 K). | |
= | Standard uncertainty. | |
= | Overall heat loss coefficient (W/m2 K). | |
= | Standard uncertainty. |
Greek symbols
= | Standard deviation. | |
= | Stefan Boltzmann constant, W/m2 K4. | |
= | Standard deviation. | |
= | Overall daily efficiency. | |
= | Still basin absorptivity. | |
= | Basin water absorptivity. | |
= | Glass cover absorptivity. | |
= | Glass cover transmissivity. | |
= | Basin water emissivity. | |
= | Instantaneous efficiency of solar still. | |
= | Daily efficiency of passive still. | |
= | Daily efficiency of active still. | |
= | Emissivity of glass cover. | |
= | Total effective emissivity. |
Disclosure statement
No potential conflict of interest was reported by the authors.
Additional information
Notes on contributors
Mohit Bhargva
Mohit Bhargva, Corresponding Author, is a Ph.D. research scholar in Mechanical Engineering Department, National Institute of Technology Kurukshetra, India. His current interests are in solar water desalination using solar still. His research work mainly concentrates on the performance enhancement of a solar still using evacuated tube collector and different heat exchangers. His previous publications have appeared in reputed International Journals.
Avadhesh Yadav
Dr. Avadhesh Yadav, Co-Author, is an Assistant Professor in Mechanical Engineering Department, National Institute of Technology Kurukshetra, India. His primary research interests include solar cooking (both indoors and outdoors), solar distillation, solar drying and water extraction from atmospheric air using solar energy. He has published more than 50 research papers in reputed International Journals in the field of solar energy and others.
References
- Appadurai, M., and V. Velmurugan. 2015. “Performance Analysis of Fin Type Solar Still Integrated with Fin Type Mini Solar Pond.” Sustainable Energy Technologies and Assessments 9: 30–36. doi:10.1016/j.seta.2014.11.001.
- Arunkumar, T., D. Denkenberger, Amimul Ahsan, and R. Jayaprakash.. 2013. “The Augmentation of Distillate Yield by Using Concentrator Coupled Solar Still with Phase Change Material.” Desalination 314: 189–192.doi:10.1016/j.desal.2013.01.018.
- Badran, O., and H. Al-Tahaineh. 2005. “The Effect of Coupling a Flat-plate Collector on the Solar Still Productivity.” Desalination 183 (1–3): 137–142. doi:10.1016/j.desal.2005.02.046.
- Badran, O. O., and M. M. Abu-Khader. 2007. “Evaluating Thermal Performance of a Single Slope Solar Still.” Heat and Mass Transfer 43 (10): 985–995. doi:10.1007/s00231-006-0180-0.
- Bell, S. 1999. “A Beginner’s Guide to Uncertainty of Measurement.” Measurement Good Practice Guide 11: 1.
- Duffie, J. A., and W. A. Beckman. 2013. Solar Engineering of Thermal Processes. John Wiley & Sons, Hoboken, New Jersey.
- Elango, T., and K. K. Murugavel. 2015. “The Effect of the Water Depth on the Productivity for Single and Double Basin Double Slope Glass Solar Stills.” Desalination 359: 82–91. doi:10.1016/j.desal.2014.12.036.
- Eltawil, M. A., and Z. Omara. 2014. “Enhancing the Solar Still Performance Using Solar Photovoltaic, Flat Plate Collector and Hot Air.” Desalination 349: 1–9. doi:10.1016/j.desal.2014.06.021.
- Faegh, M., and M. B. Shafii. 2017. “Experimental Investigation of a Solar Still Equipped with an External Heat Storage System Using Phase Change Materials and Heat Pipes.” Desalination 409: 128–135. doi:10.1016/j.desal.2017.01.023.
- Gaur, M., and G. Tiwari. 2010. “Optimization of Number of Collectors for Integrated PV/T Hybrid Active Solar Still.” Applied Energy 87 (5): 1763–1772. doi:10.1016/j.apenergy.2009.10.019.
- Goosen, M. F., S. S. Sablani, Walid H. Shayya, Charles Paton, and Hilal Al-Hinai. 2000. “Thermodynamic and Economic Considerations in Solar Desalination.” Desalination 129 (1): 63–89. DOI:10.1016/S0011-9164(00)00052-7.
- Kabeel, A., M. Abdelgaied, and M. Mahgoub. 2016. “The Performance of a Modified Solar Still Using Hot Air Injection and PCM.” Desalination 379: 102–107.doi:10.1016/j.desal.2015.11.007.
- Kabeel, A., and M. Abdelgaied. 2017. “Observational Study of Modified Solar Still Coupled with Oil Serpentine Loop from Cylindrical Parabolic Concentrator and Phase Changing Material under Basin.” Solar Energy 144: 71–78. doi:10.1016/j.solener.2017.01.007.
- Kumar, S., and A. Tiwari. 2010. “Design, Fabrication and Performance of a Hybrid Photovoltaic/thermal (PV/T) Active Solar Still.” Energy Conversion and Management 51 (6): 1219–1229. doi:10.1016/j.enconman.2009.12.033.
- Nakra, B., and K. Chaudhry. 2003. Instrumentation. Measurement and analysis. Tata McGraw-Hill Education, New Delhi.
- Panchal, H. N. 2015. “Enhancement of Distillate Output of Double Basin Solar Still with Vacuum Tubes.” Journal of King Saud University-Engineering Sciences 27 (2): 170–175. doi:10.1016/j.jksues.2013.06.007.
- Rahbar, N., and J. A. Esfahani. 2012. “Experimental Study of a Novel Portable Solar Still by Utilizing the Heatpipe and Thermoelectric Module.” Desalination 284: 55–61. doi:10.1016/j.desal.2011.08.036.
- Sampathkumar, K., and P. Senthilkumar. 2012. “Utilization of Solar Water Heater in a Single Basin Solar Still—an Experimental Study.” Desalination 297: 8–19. doi:10.1016/j.desal.2012.04.012.
- Shafii, M., S. J. Mamouri, M.M. Lotfi, and H. Jafari Mosleh. 2016. “A Modified Solar Desalination System Using Evacuated Tube Collector.” Desalination 396: 30–38.doi:10.1016/j.desal.2016.05.030.
- Shafii, M. B., M. Shahmohamadi, Meysam Faegh, and Hani Sadrhosseini. 2016. “Examination of a Novel Solar Still Equipped with Evacuated Tube Collectors and Thermoelectric Modules.” Desalination 382: 21–27.doi:10.1016/j.desal.2015.12.019.
- Singh, D. 2018. “Energy Metrics Analysis of N Identical Evacuated Tubular Collectors Integrated Single Slope Solar Still.” Energy 148: 546–560. doi:10.1016/j.energy.2018.01.130.
- Singh, D., and I. Al-Helal. 2018. “Energy Metrics Analysis of N Identical Evacuated Tubular Collectors Integrated Double Slope Solar Still.” Desalination 432: 10–22. doi:10.1016/j.desal.2017.12.053.
- Singh, D., J. Yadav, V.K. Dwivedi, S. Kumar, G.N. Tiwari, and I.M. Al-Helal. 2016. “Experimental Studies of Active Solar Still Integrated with Two Hybrid PVT Collectors.” Solar Energy 130: 207–223.doi:10.1016/j.solener.2016.02.024.
- Singh, R. V., S. Kumar, M.M. Hasan, M. Emran Khan, and G.N. Tiwari. 2013. “Performance of a Solar Still Integrated with Evacuated Tube Collector in Natural Mode.” Desalination 318: 25–33.doi:10.1016/j.desal.2013.03.012.
- Taghvaei, H., H. Taghvaei, Khosrow Jafarpur, M.R. Karimi Estahbanati, Mehrzad Feilizadeh, Mansoor Feilizadeh, and A. Seddigh Ardekani. 2014. “A Thorough Investigation of the Effects of Water Depth on the Performance of Active Solar Stills.” Desalination 347: 77–85.doi:10.1016/j.desal.2014.05.038.