ABSTRACT
This research deals with replacing proportions of cement and aggregate with Marble Waste Slurry (MWS) as a partial weight in cementitious roofing tiles production. The research is based on an experimental study of roofing tile specimens produced with ordinary Portland cement (OPC) replaced by 5%, 10%, 15%, 20% and 25% (MWS), and roofing tiles produced with sand replaced by 5%, 10%, 20%, 30% and 40% (MWS). The marble waste slurry was dried at a temperature of 200ºC for 6 h.
Physical and mechanical tests were performed to evaluate the performance of the green roofing tile specimens among which were: particle size distribution, water absorption, transverse breaking strength, compressive strength, permeability, freezing and thawing, as well as efflorescence investigations.
For all mechanical tests conducted, roofing tile specimens containing MWS as a partial replacement for cement and sand were within the ASTM standard limits (ASTM C1492/2003 (Reapproved 2016) till 20% replacement ratio.
All tile specimens containing Portland cement resulted in efflorescence.
1. Introduction
At the beginning of the technological development, the marble industry moved from labour-intensive to capital-intensive until it became operated using automated production tools like cranes and diamond cutting wires.
Several attempts had been made to utilise the ornamental stone industry waste products in the construction sector. Using the marble and granite wastes as a replacement for cement and sand in cement-based construction materials, would both reduce economic losses, and prevent environmental pollution. This procedure would lead to minimise the cement production which is responsible for about 5–7% of the global CO2 emissions and would also avoid landfilling the residues improperly. (Kandil and Selim Citation2011; Bacarji et al. Citation2013; Charkha Citation2013; Galetakis and Soultana Citation2016)
Sahan Arel (Citation2016) presented an intensive detailed review () to analyse the studies conducted by several researchers regarding substitution and utilisation of cement by marble residues. The objective of this review was to reach the optimum marble waste substitution ratio in order to cut down the cost per cubic meter of cement while sustaining the compressive strength of the cement-based material produced.
Figure 1. Relation between marble dust-cement substitution ratio and cost-benefit compressive strength [6].
![Figure 1. Relation between marble dust-cement substitution ratio and cost-benefit compressive strength [6].](/cms/asset/71adc94e-7929-450e-917a-a10c0ad1b143/tsue_a_1657981_f0001_oc.jpg)
Consequently, the author developed some formulas that adopted the parameters presented in the reviewed articles which influenced the experimental results of the mechanical and physical properties of marble. The study claimed that substituting cement by 5–10% marble powder improved the mechanical properties of the green concrete produced. This process would also reduce the CO2 emissions of cement production by 12%.
Replacing coarse aggregate by marble waste resulted in the reduction of w/c ratio. The conformity between the theoretical formulas and the experimental compression strength results reached about 99.98%.
Following the same trend, Esra (Citation2019), explored in detail the recycling options of marble waste based on reviewing previous studies. Additionally, the study developed practical equations to calculate both the compressive and splitting tensile strength in concrete containing marble waste. The results for both compressive and splitting tensile strength showed a good fit between the calculated and measured values. This research provided useful equations to determine the compressive and splitting tensile strength of concrete without experimentation. The optimum replacement rate of using waste marble instead of cement was 10%.
Bruna et al. (Citation2018) investigated the results of substituting proportions of cement in the cementitious compounds with waste from the cutting and polishing of marble and granite processing industry in the northern region of Brazil. It was observed that using the wastes as a partial replacement of cement in the proportion of 20% by mass resulted in satisfactory durability performance. The filer effect of the waste improved the particle packing and increased the cohesion of the composites. A small decrease in the compressive and flexural strength was also observed versus adding value to a cementitious product in the market that avoids the impacts caused by the inadequate disposition of the wastes in the environment.
Prasath, Tamilselvan, and Manobala (Citation2019) studied the use of quarry dust as fine aggregate in concrete. The research included five different concrete mixtures with constant water/cement ratio of 0.45.
The study investigated the compressive strength and durability properties of concrete cubes cast with stone powder substituting fine aggregate by 0%, 5%, 10%,15%, 20% and 25%. The cast cubes were allowed for 7and 28 days curing. This study indicated that the compressive strength of the examined cubes with stone powder of 20% replacement as fine aggregates is increased compared with conventional concrete. It should be denoted that this research didn’t clearly indicate the type of stone powder used to substitute the fine aggregates.
In Egypt, several efforts were directed towards studying the characteristics of the ornamental stones industry with an aim to evaluate the size of marble and granite waste problem to reach more sustainable methods of utilising it.
In order to assess the marble industry in Egypt, Kandil and Selim (Citation2011) conducted a microeconomic study. The study revealed that during the past 20 years, the marble industry achieved high-profit margins in the market. Currently, Egypt has around 500 marble and granite factories that dominate the industry. These factories constitute three types; First: factories that are responsible for cutting the blocks into plates and then distributing them to workshops for processing, second: factories for only cutting and finishing the plates, and third: factories that integrate the whole production process in one place. In Shaq Al-Thuban cluster in Cairo, there exists about 70% of the industry (around 400 factories), while the rest are scattered all over Egypt.
Later, Aliabdo, Abd Elmoaty, and Auda (Citation2014) studied the utilisation of marble dust in producing concrete. Marble dust acted as a filler in the concrete mix and showed no significant role in the hydration process. Coinciding with most previous studies, using marble dust as sand replacement in concrete achieved better performance compared to that made with marble dust as cement replacement.
Another attempt to produce self-compacting concrete (SCC) using marble and granite waste was presented by Dina, Mohamed, and Haitham (Citation2016). Three types of waste powders were investigated in this study; marble powder, granite powder and mixed powder. The results indicated that volumes up to 50% by weight of the investigated waste powders were successfully used as mineral additives in the production of SCC. Incorporating granite powder showed the superior performance of the hardened concrete as compared to the minor effect of using marble powder.
Medhat et al. (Citation2018) investigated the effect of adding marble waste in different clay–base mixes using varying percentages of up to 20%. The results demonstrated that the optimum content of marble sludge waste was15% when used as a replacement for hydrated lime in the production of clay bricks.
In a new challenge to make use of marble waste, this research is aiming to test the production of green cementitious roofing tile specimens according to ASTM C 1492/2003 (Reapproved 2016), (Citation2018). Those specs deal with the standards of concrete tiles intended for use as roof covering. The produced tile specimens under these standards are manufactured from Portland cement, water and mineral aggregates with the inclusion of marble waste slurry as partial replacement of cement and sand. The physical and mechanical properties of the tile specimens shall be tested for the compressive strength, freezing and thawing, transverse breaking strength, permeability, and water absorption.
2. Materials and methods
2.1. Raw materials
Three types of raw materials were used in this study:
Ordinary/commercial Portland cement (OPC) [CEM I 42.5 N] from Qena Cement Company, Egypt, was used in this study as a binding material. This cement type is fulfilling both European and Egyptian cement standard (EN 197–1/2011(Citation2011); ES Citation4756–1/2013). Locally available fine aggregates (natural sand from pyramids quarries Giza), and marble industry fine waste which was collected from Shaq Al-Thuban cluster, Egypt were used.
2.2. Characterisation of the raw materials
The particle size distribution for Portland cement was determined by using BT–2001 Laser Particle Size Analyser, which is conforming to ISO 13320 /2009, (Citation2009). Whereas the particle size distribution for marble waste was determined according to the standard sieving procedure described by ASTM D 422/1963 (Reapproved 2007), (Citation2014) using standard sieves complying with ASTM E 11/2017, (Citation2018). The cumulative analyses are reported then represented on a semi-logarithmic graph as processed in Abadir, M.F. (Citation2010).
)) showed that the particle size distribution of cement ranged from 1.73 to 262.38 µm with mean, median and mode size of 28.97, 16.29 and 12.32 µm, respectively. Furthermore, the D10 for cement was 6.25 µm, while D90 for cement was 68.96 µm.
On the other hand, )) presented the cumulative screen analysis of marble waste, which was determined using several sieves according to ASTM D 422/1963 (Reapproved 2007), (Citation2014). The vertical axis represented the fraction passed from each screen diameter. Both volume surface mean diameter (Ds) and D50 values for marble waste were 0.036 mm, and 0.021 mm, respectively.
2.3. Preparation of mixtures
The marble waste slurry used for the cement roofing tile specimens was dried in an oven at a temperature of 200 ºC for 6 h to maintain constant water content. Then, the obtained powder passing through sieve no. 300 was used in the mixes of the tested samples.
Based on the Egyptian Standards, 11 cement mixes of grade 350 () were prepared by partially replacing cement and/or fine aggregate with marble waste. It should be denoted that for each mix, three specimens were cast. The replacement percentages used for cement were 5%, 10%, 15%, 20%, and 25% (C mixes) and 5%, 10%, 20%, 30%, and 40% for sand replacement (S mixes). In addition, a control mix with 0% replacement of marble waste was cast. All (C) mixes had W/Cement plus waste marble powder ratio equals to 0.5. Whereas for the (S) mixes it was observed that its workability decreased as the amount of marble powder used in place of fine aggregate increased. Accordingly, the W/C ratio for these mixes was increased to 0.55.
Table 1. Mixtures components (gm).
2.4. Preparation of roofing tile specimens
For cement roofing tiles, cube specimens of (50 × 50 × 50) mm3 were cast for the compression test and prism specimens (25 × 30 × 150) mm3 were cast for transverse breaking strength test. All physical tests were also performed on prism specimens of same dimensions (25 × 30 × 150) mm3. After mixing process, mixtures were cast in the moulds and kept for 24 h, and then the specimens were cured in a curing basin for 28 days. The specimens were tested using a universal testing machine of 1000 KN (Shematzo).
2.5. Testing of cementitious roofing tile specimens
The physical and mechanical properties of all 11 samples (3 specimen each) of tile mixes including the control mix were prepared to examine the effect of partially replacing cement and fine aggregates by marble waste.
2.5.1. Determination of bulk density and water absorption
Bulk density and cold water absorption were calculated and reported according to ASTM C 67/2017, (Citation2018).
2.5.2. Determination of mechanical strength
In order to determine the compressive and transverse breaking strength of the prepared tile specimens, an apparatus and procedure described by ASTM C 1492/2003 (Reapproved 2016), (Citation2018) were used.
2.5.3. The permeability test
The permeability test was performed in accordance with ASTM C 1492/2003 (Reapproved 2016), (Citation2018).
2.5.4. Freezing and thawing test
The resistance of tile specimens to repeated cycles of freezing and thawing was evaluated in accordance with ASTM C 1492/2003 (Reapproved 2016), (Citation2018). In this test, whole tiles were required for a single test. Test pieces were subjected to alternating freezing and thawing immersed in water for up to 50 cycles. Damage was visually assessed after every five cycles.
2.5.5. Efflorescence test on tiles
The cementitious roofing tile specimens were tested using the apparatus and procedure described by ASTM C 67/2017, (Citation2018).
3. Results and discussions
The effect of cement and sand replacement by marble waste on the physical and mechanical properties of cementitious roofing tiles was studied and demonstrated in this section.
3.1. Bulk density and water absorption
For all cement and sand replacement ratios by marble waste [ and ], the water absorption values were significantly below standard limits. The bulk density showed minor differences for all samples produced with slight fluctuation around the standard value which is 2.0 g/cm3
3.2. The mechanical properties
The mechanical behaviour of cementitious roofing tile specimens presented in and ) demonstrated the relation between compressive strengths and the cement and/or sand replacement proportions of marble waste used in the mixtures.
Figure 5. Effect of cement replacement by marble waste on the mechanical properties of cementitious roofing tiles.
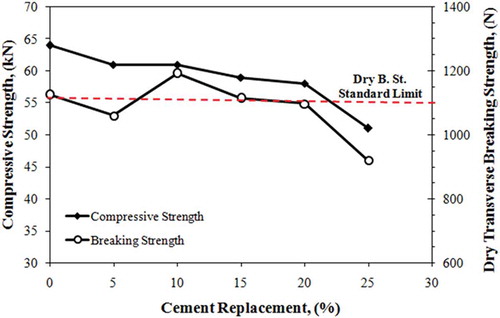
Figure 6. Effect of sand replacement by marble waste on the mechanical properties of cementitious roofing tiles.
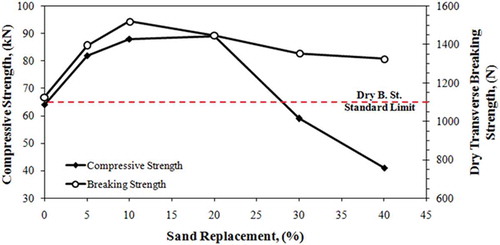
In General, when compared to the control mix, the compressive strength decreased proportionally by increasing the replacement ratio of cement with marble waste (). A significant decrease beyond the standard limits was noticed when 25% replacement ratio was used.
The transverse breaking strength values of replacing cement with marble waste powder fluctuated around the standard limits with 5–10% variance. Exceeding 20% replacement ratio of cement caused steep decrease beyond the transverse breaking strength limit.
Replacing sand with marble waste () caused an increase in compressive strength as compared to both the control mix and the standard limit till a replacement ratio of 20% above which a steep decrease in strength was recorded.
Up to 10% replacement of sand with marble waste caused significant increase about 50% in the transverse breaking strength due to the fact that marble waste particles filled the spaces between particles resulting in minimum number of pores compared to that of the control mix large-sized pores.
Even though all samples of different replacement ratios fulfilled the dry breaking strength standard limit, the recorded results still showed a slight decrease (5–10%) at sand replacement ratios from 10% to 40%.
3.3. Permeability of tiles
After 2-h period, free water was not formed on the underside of all tile specimens. Additionally, after 24 h, no water droplets had fallen from the underside of all tile specimens. Not more than 25% of the visible underside of one tile specimen showed dampness.
3.4. Freezing and thawing on tiles
At the completion of 50 cycles, all specimens remained unbroken and no individual specimen lost more than 1% of its original dry weight as shown in and ).
3.5. Efflorescence on tiles
All tile specimens containing Portland cement resulted in efflorescence. During the hydration reaction of Portland cement, the calcium hydroxide (lime) formed was transported by water to the surface through the capillaries in the concrete. This lime combined with carbon dioxide in the air to produce calcium carbonate (an insoluble material) and water.
4. Conclusions
For all physical and mechanical tests conducted, roofing tile specimens containing MWS as a partial replacement for cement and sand were within the ASTM standard limits till 20% replacement ratio.
The results revealed that:
For all cement and sand replacement ratios by marble waste, the water absorption values of tile specimens ranged between8 and 9% which were significantly below the standard limits.
The bulk density slightly fluctuated around the standard value which is 2.0 g/cm3.
The permeability test showed that after 24 h, no water droplets have fallen from the underside of all tile specimens.
At the completion of 50 cycles of freezing and thawing, all specimens remained unbroken and no individual specimen loses were recorded.
All tile specimens resulted in efflorescence.
Disclosure statement
No potential conflict of interest was reported by the authors.
Additional information
Funding
Notes on contributors
Mostafa E. Allam
Mostafa E. Allam Associate Prof. Civil Engineering Department National Research Centre.
Shereen K. Amin
Shereen K. Amin Associate Prof. Chemical Engineering Department National Research Centre.
Gihan Garas
Gihan Garas Professor of Waste Management Civil Engineering Department National Research Centre.
References
- Abadir, M. F. 2010. Mechanical Unit Operation of Particulate Solids. Cairo University, Faculty of Engineering Press.
- Aliabdo, A. A., A. E. M. Abd Elmoaty, and E. M. Auda. 2014. “Re-use of Waste Marble Dust in the Production of Cement and Concrete.” Construction and Building Materials 50: 28–41. doi:10.1016/j.conbuildmat.2013.09.005.
- ASTM C 1492/2003 (Reapproved 2016). June 2018. Standard Specification for Concrete Roof Tile. Vol. 4. U.S.A.: ASTM Annual Book. 5.
- ASTM C 67/2017. June 2018. Standard Test Methods for Sampling and Testing Brick and Structural Clay Tile. Vol. 4. U.S.A.: Annual book of American Society for Testing of Material (ASTM). 5.
- ASTM D 422/1963 (Reapproved 2007). March 2014. Method for Particle-size Analysis of Soils. Vol. 4. U.S.A.: ASTM Annual Book. 8.
- ASTM E 11/2017. July 2018. Specifications for Wire-cloth Sieves for Testing Purposes. Vol. 14. U.S.A.: ASTM Annual Book. 2.
- Bacarji, E., R. D. TFilho, E. A. B. Koenders, E. P. Figueiredo, and J. L. M. P. Lopes. 2013. “Sustainability Perspective of Marble and Granite Residues as Concrete Fillers.” Construction and Building Materials 45: 1–10. doi:10.1016/j.conbuildmat.2013.03.032.
- Bruna, S. A., G. Fernanda, P. de, S. C. M. Luciane, J. White, S. dos, and T. P. A. Maria. 2018. “Study of Portland Cement Composites Replacing Cement for Waste from the Cutting and Polishing of Ornamental Rocks.” International Journal of Science and Engineering Investigations 7 (76) :120–125. May 2018.
- BS EN 197–1/2011. 2011. Cement, Part (1): Composition, Specifications and Conformity Criteria for Common Cements. British Standards Institution (BSI).
- Charkha, S. D. 2013. “Experimental Investigation of M30 Design Mix Concrete with Partial Replacement of Conventional Ingredients.” International Journal of Research Civil Engineering and Architectural Deaign 1 (2): 38–45.
- Dina, M. S., M. A. Mohamed, and A. A. Haitham. 2016. “Reusing of Marble and Granite Powders in Self-compacting Concrete for Sustainable Development.” Journal of Cleaner Production 121 (2016): 19–32. doi:10.1016/j.jclepro.2016.02.044.
- ES 4756–1/2013. 2013. Cement, Part (1): Composition, Specifications and Conformity Criteria for Common Cements. Cairo, Egypt: Egyptian Organization for Standardization and Quality.
- Esra, T. T. 2019. “Recycling of Marble Waste: A Review Based on Strength of Concrete Containing Marble Waste.” Journal of Environmental Management 231: 86–97. doi: 10.1016/j.jenvman.2018.10.034. Feb2019.
- Galetakis, M., and A. Soultana. 2016. “A Review on the Utilization of Quarry and Ornamental Stone Industry Fine By-products in the Construction Sector.” Construction and Building Materials 102: 769–781. doi:10.1016/j.conbuildmat.2015.10.204.
- ISO 13320/2009. 2009. Particle Size Analysis – Laser Diffraction Methods. Geneva: International Organization for Standardization (ISO.
- Kandil, A. I., and T. H. Selim. 2011. Characteristics of the Marble Industry in Egypt: Structure, Conduct, and Performance. American University in Cairo, Egypt. doi:10.19030/iber.v5i3.3466.
- Medhat, S. M., M. K. Ayman, L. A. L. Mahmoud, and M. N. Abdeen. 2018. “The Feasibility of Using Marble Cutting Waste in a Sustainable Building Clay Industry.” Recycling 3 (39). doi:10.3390/recycling.3030039.
- Prasath, K. S., T. Tamilselvan, and R. Manobala. 2019. “Experimental Investigation On Mechanical And Durability Properties Of Concrete Incorporated With Quarry Dust.” International Research Journal of Engineering and Technology (IRJET) 6 (1) :1610–1614. Jan 2019.
- Sahan Arel, H. 2016. “Recyclability of Waste Marble in Concrete Production.” Journal of Cleaner Production. doi:10.1016/j.jclepro.2016.05.052.