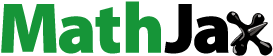
ABSTRACT
This paper presents investigations on the effectiveness of repeated washing in removing heavy metals from contaminated Foundry Sand (FS) sample collected from a recycling company in Melbourne. It is found that after repeated wash-offs, concentrations of heavy metals reduce significantly and these reductions for all the tested metals follow logarithmic trends. Results are compared with other contemporary findings on the physical separation of heavy metals from contaminated soil. Also, it is observed that higher the concentration of metals found in the initial leaching solution, higher the rate of reduction of concentration in subsequent leaching. Using logarithmic relationship, best-fit equations for the reductions of heavy metals’ concentrations through repeated wash-offs were derived. Using such equations, it is possible to work out a number of washing cycles required for a particular heavy metal to wash it up to the requirement of any particular regulatory authority. In regards to the effect of soil depth on accumulated pollutants’ concentrations, it is found that the concentrations of heavy metals in the leachate linearly vary with the depth of FS column used for testing.
1. Introduction
To reduce the world’s ever-increasing ecological footprint, recycling and reusing used materials is becoming a prime focus in the current world (Imteaz, Ali, and Arulrajah Citation2012). Foundry sand is one of such used materials, which needs attention in regards to recycling. Repeated utilisation of high-quality silica sand for casting and moulding in foundries results in the production of Foundry Sand (FS) and is presumably contaminated with different pollutants depending on the industry/site it was being used (Lin et al. Citation2012). However, limited research has been conducted on the reuse options of such waste sand (i.e. industrial/foundry sand), which is emerged from used sands in industrial sites. It was estimated that in 2002, the total world production of FS was 94,900,000 tons (Kelly et al. Citation2004). Dumping this huge amount of contaminated sand is costly; moreover, getting difficult and troublesome due to strict regulations from environmental regulatory authorities (Siddique and Singh Citation2011). As such, different reuse options are being explored and recently FS has been used in applications such as Portland cement manufacturing (FHWA Citation2004), embankments (Fox and Mast Citation1998; Partridge et al. Citation1999) and sub-bases of roads (Guney, Aydilek, and Demirkan Citation2006; Goodhue, Edil, and Benson Citation2001). Arulrajah et al. (Citation2017) have investigated geotechnical properties of FS and concluded that such material can be used for road embankment. They have also presented total energy savings for using such material instead of virgin material will save embodied energy of 81 MJ (per ton); and will reduce carbon emissions of 4.8 kg CO2 and 5.1 kg CO2e. However, as most of these waste materials are contaminated with different pollutants, in some cases reusing such materials result in shifting potential risks from one place to another.
Guney, Aydilek, and Demirkan (Citation2006) through conducting laboratory column tests on soil-foundry sand mixtures amended with cement and lime concluded that leachate coming out from the mixed soil-foundry sand column is safe for the receiving waterbody and/or surrounding environments. For the selected metals (nickel, chromium, lead, copper, zinc, and cadmium), the results of the chemical analyses indicated that the leachate is not significantly contaminated with metals. As per their hypothesis, some leached metals were likely leached from either fresh constituent added to the system sand circuit in the foundry or cooling of the molten metal in the mould. Naik, Singh, and Ramme (Citation2001) performed leachate tests on used FS and presented that the tested leachates were having selected metal concentrations far below the drinking water quality standard. However, as per ground-water quality standards (GWQS) defined by the Wisconsin Department of Natural Resources (USA), iron concentration in the leachate was above enforcement limit; and lead & chromium concentrations were above the preventive action limit. On the other hand, some other researchers (Lee and Benson Citation2002; Coz et al., Citation2004) reported that the concentrations of zinc, lead, chromium, and iron leaching from foundry sand exceeded the US EPA limits; however, they concluded that as the exceedance is only by 10%, these amounts of contaminants leaching can be acceptable.
In regards to reuse options of FS, it is necessary to mention that such FS sample is not a continuous source of pollutants, rather the collected foundry sand was contaminated from the industrial site where it was used before. Guney, Aydilek, and Demirkan (Citation2006) reported that after repeated wash-offs, concentrations of pollutants in the leachates were found to be lower than the concentrations after first leaching. They have experimented reductions of concentrations up to three consecutive wash-offs. However, in some cases it might be necessary to wash such contaminated sand for more than three times, depending on the concentrations of contaminants and required reduction of concentration. Based on field experiments, Partridge et al. (Citation1999) have reported that FS material is safe to be used in some engineering applications including highway embankments. However, if the concentrations of pollutants in the FS sample are high and such FS is intended to be reused, then suitable technique needs to be adopted for removing high concentrations of pollutants.
Soil washing is a traditional practice, widely been used for cleaning/treating typical contaminants from soil. This generally involves washing of soil column/sample using water (with or without additives) through physical separation/chemical extraction (Devgun et al. Citation1993). Physical separation is mainly suitable for soil with metal contaminants, which are under particulate forms (ideally liberated particles accumulated from other sources). The chemical extraction, which requires additions of specific chemical reagent(s), is mainly suitable for metal contaminants which are in ionic forms, i.e. free ions or adsorbed on soil particles/salts (Dermont et al. Citation2008a). Abumaizar and Smith (Citation1999) conducted soil-washing experiments in the laboratory and presented removal efficiencies of heavy metals under both the physical separation with neutral water and chemical extractions using two different reagents; disodium salt of ethylenediaminetetraacetic acid (EDTA) and sodium metabisulfite solution. They have reported that even with the neutral water, it was possible to remove 70% cadmium, 25 ~ 30% zinc, 20 ~ 25% chromium and 10% lead. With the use of reagents, higher amounts of heavy metals were removed depending on the strength and proportion of the reagents.
Wu et al. (Citation2018) investigated the leachability of metals from MgO activated ground granulated blast furnace slag (GGBS) stabilised contaminated kaolin clay exposed to simulated acid rain. In another study, Wu et al. (Citation2017) through conducting desorption test and semi-dynamic leaching test investigated the diffusion of Cadmium (Cd) from a new binder stabilised Cd-contaminated soil. Zhu et al. (Citation2015) conducted laboratory batch experiments on soil-washing efficiency on removing selected heavy metals (Cd, Pb and Zn) using varying concentrations of oxalic acid, citric acid, tartaric acid, acetic acid, hydrochloric acid and ethylenediaminetetra acetic acid (EDTA) added with deionised water. They have reported a maximum 84% removal of Cd, 59% removal of Pb and 76% removal of Zn depending on type and strength of the acids. Moutsatsou et al. (Citation2006) conducted experiments on soil-washing efficiency on removing a range of heavy metals from contaminated soil using different acids (hydrochloric acid, sulfuric acid, nitric acid) and EDTA. They have revealed that depending on strength and type of acid up to 72% removal of Fe, 51% removal of Cu, 97% removal of Zn, 93% removal of Mn, 83% removal of Pb and 92% removal of As are achievable. Dermont et al. (Citation2008b) presented a detailed review of 30 laboratory investigations and 37 field experiments on soil washing using both physical separation and chemical extraction. Due to the cost implications, chemical extraction may not turn out to be feasible for many countries/applications. Moreover, the ultimate carbon footprint of using such chemical reagent(s) is likely to be higher. As such, this study investigates efficiencies of removing different heavy metals from contaminated FS through physical separation using neutral water (i.e. soil washing) applying repeated wash-off process. The objective was to assess the effectiveness of neutral water in removing some of the heavy metals from contaminated soil. Also, as different applications may use different depths of recycled FS, it is necessary to see the effect of depth on pollutants’ concentrations in the leachates. None of the mentioned studies investigated this phenomenon. As such, this study investigated the effect of depth of FS material on concentrations of pollutants in leachate.
2. Materials and methods
The FS used in this research was provided from a recycling construction and demolition facility in Melbourne, Australia. The FS was black in colour, due to the presence of contaminants, during operational works. shows a photo of FS sample. A custom-made column apparatus having 100 mm diameter and 900 mm height was used for the column leaching tests. Leaching tests were performed following Australian Standard Leaching Procedure, ASLP (AS Citation1997) using neutral water (pH = 7) as leaching buffer.
For the repeated wash-off/leaching tests, only four heavy metals (chromium, copper, lead and zinc) were tested/measured, as during preliminary measurements for the collected FS sample all other common heavy metals (barium, nickel, selenium, vanadium and mercury) were found to be below detection limits. Collected leachate was tested for the selected heavy metals’ concentrations. In the collected leachate concentration of copper was the highest (2.33 mg L-1), followed by zinc (1.57 mg L-1), chromium (1.21 mg L-1) and lead (0.143 mg L-1). For the wash-off investigation, leachate samples were tested under repeated 25 cycles (i.e. times of washing) of leaching through an FS column of 20 cm height, placed in three layers with compactions. For the investigation on the effects of depths of FS sample on pollutants’ concentrations in leachate, three different depths (10 cm, 20 cm and 30 cm) were considered. Hach’s DR3900™ Spectrophotometer (RFID LPV440.990012, Hach Company, Colorado, USA) was used to measure the mentioned heavy metal concentrations. Each test was conducted three times and the results are presented for each test (termed as Test 1, Test 2 and Test 3). Copper was measured using USEPA Bicinchoninate method, Chromium was measured using Alkaline Hypobromite Oxidation method, Zinc was measured using USEPA Zincon method and Lead was measured using the PAR method.
3. Results and discussion
3.1 Heavy metals in leachate
After the neutral water drained through the FS column, the leachate was collected from the bottom of the apparatus. As the sample is produced from an industrial activity, it is defined as ‘industrial waste’. For such industrial waste, EPA Victoria controls the disposal in landfills and these can be dumped into solid inert landfills or municipal solid waste landfills licensed by EPA Victoria to accept this type of waste. As per US EPA drinking water standard (US EPA Citation1999), the tested leachates do not fulfil the criteria in regards to copper, chromium and lead, although it is unlikely that leachate from FS will be supplied/used as drinking water.
For this particular sample from a particular site, the heavy metals’ concentrations were found to be not exceeded the limits to be a hazardous waste. However, similar samples from another site may contain higher levels of heavy metals or some other regulatory authorities may impose lower/stringent allowable limits of heavy metals’ concentrations. In such case, a common inexpensive treatment technique is soil washing, which might be effective for contaminants of particular form(s). The following section describes effectiveness and magnitude of such technique for similar FS waste.
3.2 Effects of repeated leaching/washing
show the repeated leaching test results up to 25 cycles (times) of washing for chromium, copper, lead and zinc, respectively. Each figure shows the results of three separate measurements. From the figures, it is clear that for all the metals, differences among the three measurements are very small and can be accepted as consistent. From all the figures, it is clear that after repeated wash-offs, the concentrations of heavy metals in the FS sample significantly drop down, revealing the fact that major portions of the heavy metals for this sample are in ionic form. As such, this sort of inexpensive physical separation (i.e. soil washing) using neutral water is good enough for treating such FS, even if some heavy metals are found to be more than allowable limits. shows the concentrations of tested heavy metals at initial leaching and after 10 and 25 cycles of washing.
Table 1. Concentrations of heavy metals in the leachates of initial washing, and after 10 & 25 cycles of washing
Leachates from FS sample fulfil US EPA drinking water standard in regards to copper and chromium after 9 and 18 wash-offs, respectively. In regards to lead, after 25 cycles of wash-offs, the concentration in the leachate is 0.017 mg L−1, which is slightly higher than the maximum limit (0.015 mg L−1) in drinking water as stipulated by US EPA (Citation1999). It is to be noted that lead is very harmful to human health and as such very strict condition (limit) is enforceable in regards to allowable concentration (0.015 mg L−1) in the drinking water. Nonetheless, it is not expected that the concentrations of heavy metals leachate should be of US EPA drinking water standard, as it is not expected that such leachate will be supplied as drinking water. In regards to leachate/wastewater produced from such soil-washing facility, some sewerage authorities may have regulatory limits which can be disposed of in their sewer systems. As per South Australian Water Authority (Water Citation2012) acceptable concentrations of heavy metals, which can be disposed into the sewer system without treatment are; 20 mg L−1 for Chromium, 10 mg L−1 for Copper, 10 mg L−1 for Lead and 10 mg L−1 for Zinc. As such even leachate from the first cycle of washing can be disposed of (as per South Australian law) to the normal sewer system without any further treatment.
To see the comparison of leaching behaviours among these heavy metals, shows the averages of measurements from all the repeated leaching tests for the four heavy metals having x-axis (number of leaching cycles) in logarithmic scale. From each set of average measurements, best-fit trend lines are drawn for all the four tested metals. From the figure, it is clear that except for copper, all other metals’ leaching characteristics closely follow logarithmic trends. Even for copper, there are minor discrepancies from a pure logarithmic best-fit line. Also, it is found that the higher the concentration of such heavy metal in the FS sample, higher the rate of reductions through subsequent leaching (i.e. repeated wash-offs); copper wash-off rate is the highest and lead wash-off rate is the lowest. From the average measurements of the reductions of heavy metals through repeated washing, the best-fit line was drawn for each of the tested heavy metals. The derived best-fit equations are:
Figure 6. Comparison of best-fit lines of continuous leaching behaviours of chromium, copper, lead and zinc
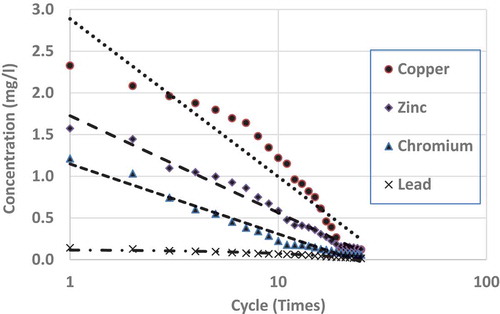
For copper:
For zinc::
For chromium::
For lead::
where ‘y’ is the concentration of heavy metal and ‘x’ is the number of cycle of wash-off. Such equations are helpful to determine the number of cycles of washing required for a particular heavy metal’s concentration to bring it down to a particular level/standard. As it was mentioned earlier wash-off rate is highest for copper, then zinc, chromium and lead (lowest). However, this may be a topic of further research whether these rates are inherent to individual element’s characteristic or the earlier quoted trend, 'higher the concentration of such heavy metal in the FS sample, higher the rate of reductions through subsequent leaching'.
3.3 Effects of contaminated soil depth
One of the major parameters affecting leachate contaminants concentrations is the depth of recycled material (i.e. FS) required/intended to be used in different applications and it is likely that deeper the application depth, higher the contaminants concentrations in the leachate. However, how a particular contaminant’s concentration varying with the depth of contaminated soil (i.e. FS) is a matter of investigation. All the tests described in the previous section were conducted with FS sample of 20 cm depth. To investigate the effect of depth, heavy metals’ concentrations in the leachate samples were tested for FS samples of two more depths; 10 cm and 30 cm. For these tests, only one leaching cycle was used, instead of 25 repeated cycles used in the previous case where the depth of FS sample was 20 cm.
shows the effects of depths on heavy metals’ concentrations in the leachate samples for chromium, copper, lead and zinc. From the figure, it is clear that all the tested metals’ concentrations linearly increase with the increase of sample (FS) depth. Also, it is found that higher the value of the initial concentration of a particular heavy metal in the leachate, higher the rate of increase of same concentrations with depth; i.e. the rate of increase of concentrations with depth is highest for copper and lowest for lead. This finding is quite reasonable, as more the depth of contaminated sand, heavy metal with higher concentration will have a higher rate of increase with the accumulation of higher amounts of heavy metal in the sand.
4. Discussions
Earlier studies revealed achieving very high removals of heavy metals using different types of acidic solutions. However, for the consideration of cost and to avoid ending up with a higher carbon footprint, it is recommended that for moderately contaminated soil, washing with normal (or distilled water) may achieve the goal. Abumaizar and Smith (Citation1999) reported removals of Cr, Pb and Zn as 18.3%, 17.3% and 30.9%, respectively, using deionised water. In the current study using distilled water mean reductions in leachate pollutants’ concentrations are, 10.5% for Cu, 10% for Pb, 15% for Cr and 8% for Zn after one cycle of washing. Findings of Abumaizar and Smith (Citation1999) are slightly higher than the removal efficiencies achieved in the current study. Such higher removal efficiencies are justified, as in the study of Abumaizar and Smith (Citation1999) the reported removal efficiencies were achieved for a soil to solution ratio of 1:5, whereas in the current study the ratio used was 1:1. In contrast, some other studies reported very low reductions in metals’ concentrations using only water; Qi et al. (Citation2018) reported 2% reduction of Pb, Zhu et al. (Citation2015) reported less than 2% removals of Cd and Pb, Moutsatsou et al. (Citation2006) reported less than 1% removals of six different types of metals (Fe, Cu, Zn, Mn, Pb and As) through washing using deionised water. Such wide variations of removal efficiencies occur due to the form of the particular metal present in the soil. Removal efficiency will be very low if the metals are tightly bound with the soil particle as one/more of the following fractions: ion exchangeable, adsorbed, precipitated, organically bound or trapped in an insoluble form with the soil. In regards to experimental results using different depths of contaminated soil, Imteaz et al. (Citation2017) also found the similar trend, where the accumulation of total phosphorus concentration linearly increased with depth while passing through a column of recycled wood chips.
Another important fact needs to be mentioned here that all the findings reported in this study are applicable to the FS samples collected from the industrial sites in Melbourne. It is recommended that similar investigations need to be performed for other FS samples, before it can be reused for any engineering application. Although pollutants in the reused FS are not having continuous source and will wash-off after several natural leaching through rains, even it was not washed off-site before reuse. Moreover, before they reach to a nearby waterbody, pollutants in the leachate will go through diffusion and dispersion processes, through which concentrations of any pollutants will be reduced significantly.
5. Conclusions
With the aim of achieving a sustainable world through increased use of recycled materials, this study presents suitability of reusing foundry sand for different engineering applications. Although there are hundreds of different recycled materials available, due to strict restrictions from the environmental regulatory authorities, not all of those are allowed to be reused. As most of these recycled materials are likely to be contaminated with different pollutants, the major concern is risks of exposure of potentially hazardous material and leaching of pollutants into surrounding environment including waterbodies. Through chemical testing of leachate, FS sample collected from a local recycle company was found to be contaminated with few heavy metals; chromium, copper, lead and zinc. Among these heavy metals, in the leachates passed through an FS column of 20 cm depth, concentrations of copper, chromium and lead were higher than the allowable limits set by US EPA in regards to drinking water. Although it is very unlikely that leachate from any potential engineering application will be used/supplied as drinking water; however to be in the safer side some regulatory authorities may not permit such leaching onsite, lest the leached heavy metals contaminate the surrounding environment or cause harm through physical contacts. As the concentrations of pollutants in the leachate will largely vary with the depth of contaminated soil (i.e. FS), the same leaching tests were conducted with two more depths of the FS samples; 10 cm and 30 cm. As expected, it was found that concentrations of tested heavy metals linearly vary with the depth of the FS sample. Also, the higher the concentration of any particular heavy metal present in the FS sample, the higher the rate of increase of concentrations with the increase of depth.
Thorough chemical testing was conducted to investigate the leaching behaviour of collected FS sample in regards to repeated leaching/wash-offs of heavy metals found in the sample. It is found that through repeated wash-offs, concentrations of heavy metals in the leachate significantly drop down and reductions of heavy metals in the leachates follow logarithmic patterns for all the tested heavy metals. Also, the higher the concentration of particular heavy metal in the leachate, the higher the rate of reduction of concentration through subsequent/repeated wash-offs. Best-fit equations of relationships of heavy metals’ concentrations with the number of washing cycles were derived, which are useful to determine the number of washing required to bring a particular FS sample to a specific required standard. It is to be noted that above-mentioned findings are valid for contaminated sand/soil, where heavy metal(s) present as detachable form, not tightly bound with the soil particles as discussed earlier.
Acknowledgments
The authors wish to thank Alex Fraser Group, Melbourne, Australia for providing the foundry sand sample for this research project.
Disclosure statement
No potential conflict of interest was reported by the authors.
Additional information
Notes on contributors
Monzur Alam Imteaz
Dr Monzur Alam Imteaz is an Associate Professor in the Department of Civil & Construction Engineering at Swinburne University of Technology, Melbourne. He obtained his Ph.D. in 1997 from Saitama University, Japan. Later he has completed his post-doctoral research at The University of Queensland, Brisbane. Before joining at Swinburne he has been involved with several Australian state and local government authorities. He has been actively involved with various researches on sustainability, water recycling, developing decision support tools and seasonal rainfall forecasting. Dr Imteaz is the author of the book, “Urban Water Resources”. He is serving as editorial board member for the international journals, “Resources, Conservation & Recycling”, “Journal of Hydroinformatics”, “World Environment” and “Journal of Water Resource and Hydraulic Engineering”.
Arul Arulrajah
Prof. Arul Arulrajah is the Professor of Geotechnical Engineering at Swinburne University of Technology, Melbourne, Australia. He is currently involved in research topics such as recycled materials in geotechnical applications, geotechnics of pavements and ground improvement. Arulrajah joined Swinburne in 2006 after 14 years of prior industry experience in Australia, Singapore and Malaysia (1992-2006) in the design, site implementation and project management of geotechnical engineering and civil engineering infrastructure projects. Arulrajah is a Fellow of the Institute of Engineers Australia (FIEAust) and a Chartered Professional Engineer (CPEng).
References
- Abumaizar, R. J., and E. H. Smith. 1999. “Heavy Metal Contaminants Removal by Soil Washing.” Journal of Hazardous Materials B70: 71–86. doi:10.1016/S0304-3894(99)00149-1.
- Arulrajah, A., E. Yaghoubi, M. A. Imteaz, and S. Horpibulsukb. 2017. “Recycled Waste Foundry Sand as a Sustainable Subgrade Fill and Pipe-bedding Construction Material: Engineering and Environmental Evaluation.” Sustainable Cities and Society 28: 343–349. doi:10.1016/j.scs.2016.10.009.
- AS. 1997. “Wastes, Sediments and Contaminated Soils, Part 3: Preparation Ofleachates-bottle Leaching Procedure.” In Australian Standards 4439.3. Homebush, NSW, Australia: Standards Australia.
- Coz, A., A. Andres, S. Soriano, and A. Irabien. 2004. “Environmental Behavior of Stabilized Foundry Sludge.” Journal of Hazardous Material 109: 95–104. doi:10.1016/j.jhazmat.2004.03.002.
- Dermont, G., M. Bergeron, G. Mercier, and M. Richer-Laflèche. 2008a. “Soil Washing for Metal Removal: A Review of Physical/chemical Technologies and Field Applications.” Journal of Hazardous Material 152: 1–31. doi:10.1016/j.jhazmat.2007.10.043.
- Dermont, G., M. Bergeron, G. Mercier, and M. Richer-Laflèche. 2008b. “Metal- Contaminated Soils: Remediation Practice Sand Treatment Technologies, ASCE Practice Periodical of Hazardous.” Toxic and Radioactive Waste Management 12 (3): 188–209. doi:10.1061/(ASCE)1090-025X(2008)12:3(188).
- Devgun, J. S., N. J. Beskid, M. E. Natsis, and J. S. Walker (1993). “Soil Washing as a Potential Remediation Technology for Contaminated DOE Sites.” International Waste Management Conference, February–4 March 28 February–4, Tucson, Arizona.
- FHWA (2004) “Foundry Sand Facts for Civil Engineers.” Report No. FHWA-IF-04-004 prepared by American Foundrymen’s Society Inc. for Federal Highway Administration Environmental Protection Agency Washington, DC, USA.
- Fox, P. J., and D. G. Mast. 1998. Geotechnical Performance of a Highway Embankment Constructed Using Waste Foundry Sand. Publication FHWA/IN/JTRP-98/18. Joint Transportation Research Program. West Lafayette, Indiana: Indiana Department of Transportation and Purdue University. doi:10.5703/1288284313231.
- Goodhue, M. J., T. B. Edil, and C. H. Benson. 2001. “Interaction of Foundry Sands with Geosynthetics.” Journal of Geotechnical and Geoenvironmental Engineering 127: 353–362. doi:10.1061/(ASCE)1090-0241(2001)127:4(353).
- Guney, Y., A. H. Aydilek, and M. M. Demirkan. 2006. “Geoenvironmental Behavior of Foundry Sand Amended Mixtures for Highway Subbases.” Waste Management 26: 932–945. doi:10.1016/j.wasman.2005.06.007.
- Imteaz, M. A., M. M. Y. Ali, and A. Arulrajah. 2012. “Possible Environmental Impacts of Recycled Glass Used as a Pavement Base Material.” Waste Management and Research 30 (9): 917–921. doi:10.1177/0734242X12448512.
- Imteaz, M. A., N. Altheeb, A. Arulrajah, S. Horpibulsuk, and A. Ahsan. 2017. “Environmental Benefits and Recycling Options for Wood Chips from Furniture Industries.” Proceedings of ICE - Waste and Resource Management 170 (2): 85–91.
- Kelly, T., D. Buckingham, C. DiFrancesco, K. Porter, T. Goonan, J. Sznopek, C. Berry, and M. Crane (2004). “Historical Statistics for Mineral and Material Commodities in the United States.” U.S. Geological Survey Open-File Report 01–006, http://minerals.usgs.gov/minerals/pubs/of01-006/.Langer
- Lee, T., and C. H. Benson (2002) “Using Waste Foundry Sands as Reactive Media in Permeable Reactive Barriers.” Geo Engineering Report 02-01, University of Wisconsin-Madison, USA.
- Lin, K.-L., C.-J. Cheng, A. Cheng, and S.-J. Chao. 2012. “Study on Recycled Waste Foundry Sand as Raw Materials of Cement Additives.” Sustainable Environmental Research 22: 91–97.
- Moutsatsou, A., M. Gregou, D. Matsas, and V. Protonotarios. 2006. “Washing as a Remediation Technology Applicable in Soils Heavily Polluted by Mining–metallurgical Activities.” Chemosphere 63 (10): 1632–1640. doi:10.1016/j.chemosphere.2005.10.015.
- Naik, T. R., S. S. Singh, and B. W. Ramme. 2001. “Performance and Leaching Assessment of Flowable Slurry.” Journal of Enviromental Engineering 127: 359–368. doi:10.1061/(ASCE)0733-9372(2001)127:4(359).
- Partridge, B., P. Fox, J. Alleman, and D. Mast (1999) “Field Demonstration of Highway Embankment Construction Using Waste Foundry Sand.” Transportation Research Record, Paper No. 99-0612, pp. 98–105.
- Qi, X., X. Xiaoming, Z. Chuanqing, J. Tianyi, W. Wei, and X. Song. 2018. “Removal Of Cadmium and Lead from Contaminated Soils Using Sophorolipids from Fermentation Culture of Starmerella Bombicola Cgmcc 1576 Fermentation.” International Journal of Environmental Research and Public Health 15 (2334): 1–12.
- Siddique, R., and G. Singh. 2011. “Utilization of Waste Foundry Sand (WFS) in Concrete Manufacturing.” Resource, Conservation and Recycling 55: 885–892. doi:10.1016/j.resconrec.2011.05.001.
- US EPA. 1999. National Primary Drinking Water Standards, EPA-F-94-001. Washington, USA: Environment Protection Agency.
- Water, S. A. 2012. Restricted Wastewater Acceptance Standards. Adelaide: South Australia Water.
- Wu, H. L., F. Jin, Y. Bo, Y. J. Du, and J. Zheng. 2018. “Leaching and Microstructural Properties of Lead Contaminated Kaolin Stabilized by GGBS-MgO in Semi-dynamic Leaching Tests.” Construction and Building Materials 172: 626–634. doi:10.1016/j.conbuildmat.2018.03.164.
- Wu, H. L., Y. J. Du, F. Wang, M. L. Wei, and Y. S. Feng (2017). “Study on the Semi-Dynamic Leaching Characteristics of CD Contaminated Soils Solidified/Stabilized with Phosphate under the Condition of Acid Rain.” In Geotechnical Frontiers Conference, pp. 414–422, Orlando, FL. doi:10.4041/kjod.2017.47.6.414.
- Zhu, G., Q. Guo, J. Yang, H. Zhang, R. Wei, C. Wang, M. Peters, X. Zhou, and J. Yang. 2015. “Washing Out Heavy Metals from Contaminated Soils from an Iron and Steel Smelting Site.” Frontier of Environmental Science and Engineering 9 (4): 634–641. doi:10.1007/s11783-014-0713-6.