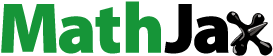
ABSTRACT
Process sustainability indicates the proper utilisation of technology to improve productivity, reduce cost as well as environmental impact in the industry. These attributes are vital for an industry to be continuously monitored in order to stand in the market for the long run. In this context, a timber mill has been chosen for a case study in the present investigation. The most produced product is targeted, and a complete audit is made from the product start to end in order to compile the data, which are then used for sustainability study. The sustainable analysis is made with respect to carbon footprint, energy consumption, water, and air impact. This study enables to know the target area to address; accordingly, the process design has been modified using the principles of design for manufacturability/sustainability. The proposed process model ensures production not only for the target product but also versatile for other different products that are produced in the industry. In addition, the proposed model reduces the energy required to produce the target product thereby reduces environmental impact and cost; on the other hand, it improves productivity as well.
1. Introduction
Sustainability emphasises on social, economic and the environment in order for a business to sustain in the competing market (Dyllick and Rost Citation2017). In the context of manufacturing, sustainability is brought in the initial phase of the product design itself (Salari and Bhuiyan Citation2016). The process is then designed to optimise the sequencing of operations that required minimum resource utilisation for sustainable production. In majority of the developed countries, the design for manufacturability and/or sustainability (DFM/S) are strictly linked to broader perceptions that include: ‘sustainable product-service system, systems innovation and other life cycle based efforts’ where industries are forced to maintain environmental safety and focus towards practices that are planned in a manner to reduce energy utilisation (Sutherland et al. Citation2008). Sustainable practices are implemented to reduce production cost and prevent environmental problems, which helps to maintain a clean and green atmosphere. The practical aspect of the eco-conscious product or process design involves with minimum material wastage, improve material choices, the design for ease of disassembly, product reuse, minimal energy consumption, manufacture without hazardous waste and use of clean technology (Jan Schoormans Citation2018). Thus, the choice of the manufacturing process is very crucial as it controls ‘the usage of the product with the amount of energy used for the process of production’ as well as ‘the use of natural resources’. However, industries in developing countries such as Fiji, the lack of awareness about the eco-conscious process or product design is one of the prime concern.
In this context, a case study is conducted to ensure some aspects of sustainable design for a 6x2 (inch) timber manufactured in a sawmill, situated in Pacific Harbour, Fiji, using DFM/S. The case study begins with the identification of a product, which had been manufactured by a company over five decades. The study then engages towards CAD modelling of the product (timber) in SOLIDWORKS followed by sustainability analysis (sustainability Xpress) to determine the environmental impacts in terms of; energy consumption, carbon footprint, water eutrophication, and air acidification. Then the production processes are redesigned using DFM/S, after which the environmental impact is compared, and finally, the cost analysis is carried out for the proposed solution to check its affordability. The purpose of this study is to bring awareness about environmental conscious production process that not only reduces environmental impact but also improves productivity and reduces the production cost and thereby successfully meets the consumer requirement.
2. Literature research
The first phase of the literature review is focused on the tools and techniques that are adopted in carrying out sustainability analysis of a product. Then, the review is extended to examine the sustainability study with respect to the product as well as the process.
2.1 Sustainable product development
Suresh, Ramabalan, and Natarajan (Citation2016) reported that design for environment (DFE) and design for manufacturing and assembly (DFMA) principles can be used to develop a sustainable product, for which a case study was conducted on the ‘charge alternator pulley’. In this investigation, the ANSYS software is used to remodel the product and perform the stress analysis. Eventually, SOLIDWORKS software was used to determine the environmental impact of the CAD model; by which the remodelled product was proven to have a less environmental impact as compared to the existing design. Likewise, Vinodh (Citation2011) performed an investigation on ‘rotary switch’ using computer-aided design and engineering. The existing design is remodelled using the ProE software and is subjected to sustainability analysis to reveal the environmental impact for both before and after the design; the redesign in software was proven to have a less environmental impact as compared to the existing design. Almost in a very similar context, Vinodh and Rajanayagam (Citation2010) and Vinodh (Citation2010) were performed the studies on sustainable product development. It is reported that in the ‘former case’, design for manufacturability (DFM) is used and in the ‘latter case’, the DFE is used. To summarise, the SOLIDWORKS software appears to be highly used to perform the sustainability analysis of a product. This reveals the environmental impact, in terms of carbon footprint (CFP), energy consumption (EC), air acidification (AA), and water eutrophication (WE) for the product’s initial to the final phases, such as materials, manufacturing, usage, end of life and transportation. All the literature above is focused on either modifying the geometry or the material or the manufacturing just in the software to develop a sustainable product. Neither in the software nor the real industry environment, the whole or the part of the processes were considered in developing the sustainable product.
2.2 Environmental conscious quality function deployment
On the other hand, the literature study attests that the quality function deployment (QFD) is widely employed in developing environmentally conscious product design (ECPD). Romli et al. (Citation2018) proposed a methodology to combine ecological QFD with eco-design case-based reasoning to produce the product with the consideration both environmental as well as economical. This paper deals with a case study that takes advantage of environmental conscious QFD with economic costing during the conceptual design phase itself. Alemam and Li (Citation2016) believed that the quality management system (QMS) is not only useful in achieving process improvement but also to address the environmental concerns of the product. Therefore, an attempt has been made to combine QFD and functional analysis using relational matrices in order to develop a framework to ensure the eco-design; which was eventually proved using a case study, ‘hairdryer’. Likewise, many researchers (Sakao Citation2009; Vinodh and Jayakrishna Citation2014) had reported that QFD is a prominent tool for ECPD; moreover, the authors developed a framework for the successful implementation of environmental conscious quality function deployment (ECQFD). However, this tool is reported to have utilised effectively in the literature for ‘early product design’.
2.3 Sustainable manufacturing process study
If the process is taken into consideration for sustainable analysis then the energy reduction or waste reduction or the combination of both is inevitable to observe in the literature. The application of lean tools such as value stream mapping is widely found to be utilised in achieving the aforementioned reductions (Henao, Sarache, and Gomez Citation2019; Reyes et al. Citation2018). Seow et al. (Citation2016) designed an energy minimisation approach to reducing energy consumption during the manufacturing phase; this approach was eventually demonstrated using a simple and complex product. Salonitis (Citation2015) proposed a framework to measure the energy performance of a grinding process. He also suggested that the proper formulation of ‘process strategy’ significantly reduces energy consumption. UzZaman (Citation2015) has provided a comprehensive review of waste management; the study not only focused on the manufacturing sector but also on the service sectors. Particularly, the study emphasises on the scope of pursuing research in ‘zero waste design’ for the industries and even for the nations in terms of strategic policymaking for the implementation of zero waste concept in a holistic approach.
Therefore, from the literature study, it was clearly understood that the sustainability study had been widely conducted on the product design phase using appropriate tools. In other words, there are several tools available, and the frameworks are developed to address the sustainability of the product under design phase. On the other hand, manufacturing processes based sustainability study is very limited in the literature. Even if it is there, the process-based is predominantly addressing the energy minimisation during the design phase of the product or to a particular machining process by more focusing on the process parameter optimisation. On the other hand, the managerial concepts such as lean approach and zero waste approach are widely seen in the literature to address the process side sustainability in terms of waste reduction or waste management strategy. The entire manufacturing processes considered against the sustainability index using appropriate software and the mitigating procedures are sequentially followed in line with the concept of design for sustainability/manufacturability to ensure the technical, economic and environmental aspect is the novelty of the present work.
2.4 Research approach
The procedure used to carry out the case study is illustrated in . This study begins with the research and literature survey on sustainable product development. A random sawmill organisation was selected where construction timber was chosen as a product. Then, an industrial visit was carried out to grasp the necessary information needed for the investigation. CAD modelling of the timber was made, and sustainability analysis of the product was conducted to identify the current environmental impact. The important areas of interest were identified to navigate the remodelling of the manufacturing processes using DFM/S principles. After the proposed solution was finalised, a cost analysis was also conducted.
3. Case study
This section presents the details about the product, industry, production processes or current facility layout, and the sustainability analysis for the current facility layout for the considered product.
3.1 About the product
The 6 x 2 inch pine timber is considered as a product for the present investigation. The CAD model and its specifications are shown in . The product is used around the central and western part of Viti-Levu, Fiji Island, which indicates that the consumer of the product from the company is in the aforementioned region only. It was identified from the field survey that the product has a maximum lifespan of 50 years but limits the usable duration to 45 years. It was further revealed that about 25% of the timber is reused when maintenance work is carried out in houses and buildings. The 65% is incinerated, and the remaining 10% is disposed of in landfills.
3.2 About the industry
The industry is situated in the central part of Viti-Levu and it is producing construction timber, weatherboards, quarter rods, and pallets. The company has been producing these products since the 1980s from not only pine and mahogany trees but also from native trees such as, ‘Dakua’, ‘Sadau’, ‘Damanu’ and so forth (these are native names used to describe the timber). The facility is isolated; hence, it generates its electricity through a diesel generator.
3.3 Current facility set up for timber production
To understand the environmental impact of the product, the entire processes were considered in the present investigation. These processes can be divided into three different categories that include the extraction of the raw material in the forest, the processing of the raw material at the facility, and the chemical treatment in the facility. illustrates all the three categorised stations in sequence. In the early stages of production (, forest subsystem), the native trees are sawn down where bulldozers are required to pull these trees along the forest floor through a winch. The chainsaw operators trim off the barks to allow the logs to be easily manoeuvred onto the trucks with a loader. The logs then make their way to the facility along the fifty-kilometre journey. The trucks take a treacherous route through thirty-four kilometres of the muddy road with a naked river in between, followed by the rest of the sixteen-kilometres of loose gravel to reach the sawmill. Once, the trucks reach the facility (, sawmill subsystem), the logs are unloaded with the use of loaders. The same loaders are used to carry the logs to the starting point of the mill. From here, conveyers transport the logs to connective processors where the operations take place.
The product undergoes six processes at the sawmill subsystem including the chemical processor; the facility layout is drawn using Flex-Sim software and is shown in . At Processor 1 (P1), the log is rotated with the use of pneumatic jaws, which help to manoeuvre the log through a band saw. This process is carried out several times until the log comes to a regular shape, say a rectangular cross-section of 12 × 8 inches. In addition, this cross-section is further reaped into two halves to carry out further steps of operations in processor 2 (P2). Now, the remains from P1 is sent to Processor 3 (P3) for further extraction of material.
In the second stage, the slabs enter Queue 3 where they are sent through P2 that consist of a smaller bandsaw (relative to P1). The end-product from P2 is sent to Processor 4 (P4) in a conveyor, whereas the remaining timber from the slab returns back to Queue 3 (at the starting point of the band saw) through a conveyor creating a loop. This looping continues until as much as a product (say in this case 6 x 2 inch) can be extracted at P2. After the slab is sawn, the remaining material sent to P3 for further material extraction. In addition, P4 is a drop-saw, which is used to crosscut the timber from P2 to the desired length. On the following day, P3 is used to convert the waste material from P1 and P2 into usable timber. This timber is manufactured into pallets and is mainly consumed for the companies purpose itself. However, if there are left-over material which cannot be further processed, they are sold as firewood.
The slab comes out of P4 is then transferred to Processor 5 (which is a multi-line planar), is used to improve the surface finish of the timber along the four sides. After surface finish, the timber is sent to Processor 6 (chemical treatment facility) where it is treated in a closed chamber. This process takes 2 hours, after that the timber is kept in the timber yard for at least 2 weeks to complete the curing process before it can be delivered to the clients. In addition, the facility is equipped with the underground sawdust management system, which uses chain conveyers to exit waste material to the far end of the facility, where a loader is used to dispose of it. In , the ‘source 1’ represents the logs, which arrive from the forest in the trucks. ‘Rack 1’ represents the storage of the finished product and ‘sink’ represents the secondary product (timber for pallets) storage.
3.4 Sustainability analysis for current facility setup
The considered product is modelled using ACAD, which is then interfaced with SOLIDWORKS to carry out the sustainability analysis using SustainabilityXpress applications. The software needs various inputs in order to generate the environmental impact of the product, which are collected from the current facility, and it is portrayed in .
Table 1. Data input for sustainability analysis as per the current facility setup.
For the case study, the material has been specified as Pine. The volume, surface area, and weight are computed. The manufacturing process has been specified as custom where the production energy consumption and the material wastage from the raw material have been specified. In addition, the total travel distance, region of use and end of life has also been stated in . While specifying the input data, the region selected was Australia. This is mainly because the software only offers certain regions around the globe. Since Australia is geographically similar to Fiji, and most standards followed in Fiji are Australian based, this particular region opted.
It is noted that fuels meet the energy demand of the plant facility, predominantly by diesel, the total breakdown of energy usage and the summary of energy consumption is given in . The total BTU is 2315083.41 per timber, which is equivalent to 146 BTU/gm is used as manufacturing energy consumption as shown in for sustainability analysis software. The sustainability analysis report for the product with respect to current facility setup generated by the software is shown in . The environmental impact is assessed in terms of carbon footprint (CFP), energy consumption, air acidification and water eutrophication for the parameters such as materials, manufacturing, use, end of life and transportation. The careful observation of this table reveals that manufacturing causes the most impact on the environmental, in particular on the carbon footprint of 6% and energy consumption of 93.8%; while the remaining parameters’ contribution on the EI indexes is relatively negligible.
Table 2. Fuel energy breakdown for manufacturing the timber.
Table 3. Environmental Impact based on current facility setup.
4. Process analysis and remodelling using DFM/S principle
In this section, problem identification and alternative solutions for the identified problems are discussed in the context of manufacturability and sustainability. Then, the remodelled facility setup and corresponding sustainability analysis are also discussed.
4.1 Problem identification
It was understood from the sustainability report () that the manufacturing creates maximum environmental impact; in particular, the energy consumption is the highest followed by carbon footprint. As explained in Section 3 that the manufacturing of timber is accounted from the forest to sawmill facility; out of which the chances of reducing the energy consumption was found to be prominent and feasible in sawmill subsystem rather than in the forest subsystem. Since bringing logs from the forest to the facility is almost rigid; alternatively, the careful observation of the processors 1 through 6 exhibited the fact that certain processors are found to be consuming the power much more than it is needed. More specifically, there were two problems identified, which are: First problem is the motor size for processor 1, 2 and 3 that were the same (75KW). Although for processor 1 the motor size is found to be valid, for processor 2 and 3 it is meaningless as compared to the job it performs. Secondly, at P2, the slabs were required to pass through the processor several times where the majority of the energy is needed to transfer the slab back and forth through the conveyers. This contributed to a significant amount of energy consumption in the production line. Hence, the reduction in energy consumption in the facility is expected to reduce the fuel consumption eventually that reduces the environmental impact.
While developing the solution for the identified problem, the DFM/S philosophies are considered. The DFM/S refers to the design for manufacturability and/or sustainability; this means any solution should be manufacturable, environmentally sustainable and cost-wise affordable. The components of DFM concentrates predominantly on part design simplification and standardisation, at the same time it is also not failed to emphasise on a review of manufacturing processes and its environmental impact (Gebisa and Lemu Citation2017). Meanwhile, the philosophy of design for sustainability (D4S) stresses on two important facts, one is ‘doing the right things’ and the other is ‘doing things right’ (‘Econation for people and planet’ Citation2018). The ‘former’ compels that any activities are done should not harm human and/or the environment, if so it is the wrong thing and the ‘later’ compel on the activities that should consume less energy and possess high durable and recyclable. Therefore, in our case study, the product is simple and straightforward, which is 6 x 2 inch pine timber and it is kept unchangeable. However, the manufacturing processes can be reviewed and can be made sure, that its environmental impact shall be reduced as compared to the existing facility by means of reducing the energy consumption at the same time improving the productivity, which becomes the direct object of the present investigation.
4.2 Phase 1: multi-blade processor at P2
At Processor 2, the timber slab is further sawn to produce almost to the required dimension especially the width and thickness, in our case; it is 6 × 2 inches. This product is now sent to processor 4 to cut the edges, whereas the leftover timber slab after processor 2 is transferred back to a buffer (queue 3) along with a conveyor (). The timber is again sent through the Processor 2 and the process is repeated until all the maximum 6 × 2 inches timber is extracted. The leftover material is transferred to Processor 3 to make pallets. It was identified that a seventy-five kilowatt (75KW) electric motor is used to run a single blade band saw, where pneumatic cylinders are used to align the timber slabs to the processor.
Therefore, a multi-blade processor (MBP) was identified as an appropriate solution to address the issue. Even though the processing dimensions of 2 inches thickness and 6 inches width were desired for this case study, the industry produces ranges of products. The chosen MBP can process as much as up to 15 inches thickness and the width up to 24 inches thickness (‘Multi-blade processor’ Citation2018) thereby meets the company’s requirement. This is a multi-band saw ripping machine, specifications illustrated in , where the whole slab can be processed through the processor once without the need of transferring the slab back and forth while using less energy. Most importantly, the machine’s power consumption is 76 KW (11 KW/blade and 10 KW for conveyer) to produce six pieces. Since only two blades are needed for the given case study (the pine slab dimension can maximum yield only up to two 6 × 2 inches timbers at P2), the net power consumption is expected to be 32KW. It is noted that the machine has the capacity of mounting and running required numbers of blades at a given time, which will cater to the company’s product variety need as well.
Table 4. Technical specifications comparison for current and proposed setup.
In addition, the power currently required to transfer the unprocessed slab through the conveyor, from Processor 2’s exit point to Processor 2’s inlet buffer (Queue 3) was 10 KW and the power required to move the slab along the buffer was 15 KW, so a total of 100KW is required. With the proposed processor, the conveyor and the buffer (Queue 3) can be eliminated as shown in . It is carefully noted that as per the existing setup, there is one staff dedicated to aligning the timbers on both sides of the Queue 3. However with the proposed solution that eliminates the Queue 3, which not only ensures the reduction in inventory by 50% due to single flow of the material, but also the employee workload reduces significantly (roughly by 50%) that accounts for the improvement of the social aspect of sustainability.
To summarise, with the installation of the proposed processor, the power consumption is expected to be reduced from 100 KW to 21 KW to process one timber at Processor 2 (). Thus, the reduction in power consumption to produce one timber can be achieved by 79% without compromising the product quality, while increasing the production rate, as the machine capable to produce two finished slabs in a single pass in place of one-slab produces with the current facility. Moreover, since workers at the facility are used to work with the band saw machine, the facility is already equipped with the necessary tools/machines needed to carry out maintenance on the proposed processor. illustrates the comparison of existing and the proposed setup power consumption to process one timber and also with respect to other essential features for both P2 and P3.
4.3 Phase 2: single blade table saw at P3
At Processor 3, the uneven timber from Processor 1 and left-over timber from Processor 2 are sawn to make timber planks sized suitably for pallet making (i.e. 3 × 1 inch and 2 × 1 inch dimension). It is noted that the electric motor used in Processor 3 also possess 75KW power. However, the timber that reaches P3 has a peak thickness of 3 inches, hence the existing setup is overdesigned than it is essential for the process. Thus, an alternate solution proposed in view of both manufacturability and sustainability is an electric motor capable of 9KW power that performs required varieties of tasks with high quality (‘Single-blade table saw’ Citation2018). It is a single blade table saw, has a sawing thickness of 85mm (3.35 inch) and high cutting speed for good surface finish, apart from saving power up to 88%. The comparison of the proposed machine specification to that of the existing machine is given in . The proposed machine also has additional features such as infrared alignment that reduces the human effort of placing the planks in order.
4.4 Sustainability analysis for the proposed facility setup
The proposed solutions for the two different phases are combined to create a proposed facility layout, which is shown in . In the first phase, the loop and its corresponding queue 3 can be eliminated at P2, apart from the proposed machine of 11KW capacity for each blade to produce one product at a time. Similarly, at P3 the motor alone can be replaced without compromising the purpose, however, the layout remains as it is. Therefore, the net power at P2 reduces from 100 KW to 21 KW, similarly at P3 reduces from 75 KW to 9 KW, hence the total electrical load requirement reduced by 145KW to produce one product. The proposed combined solutions in the processes lead to reduce manufacturing natural gas input as 108Btu/gm from 146 BTU/gm. This data is fed for the sustainability analysis and the corresponding environmental impact is shown in . It can be noted that the proposed solutions shall bring down the total energy consumption by 26%, which lead to reducing the environmental impact in terms of total carbon footprint by 6.77%, total energy consumption and total air acidification by 11% each and finally total eutrophication by 2.77%.
Table 5. Environmental Impact for the revised facility setup.
5. Cost analysis
The determined solutions although proven as sustainable and manufacturable, the feasibility of implementations is still found to be unclear. As the discussion held with the chief technician revealed the general perception is that such changes lead to heavy cost investment, although it’s a ‘word of mouth’ still it is powerful for not bringing any changes in the industry. Thus, cost analysis has been carried out to give completeness on this investigation. depicts all other necessary details that are required to calculate break-even point (BEP), such as variable costs, fixed costs, selling price and average production per day. The EquationEq. 1(1)
(1) , is used for BEP calculation (Stevenson Citation2018). It is noted that as per , the ‘construction timber’ details are used to calculate BEP for phase 1; whilst, the ‘pallets’ details are used for Phase 2, BEP calculations. The break-even point predicted to be achieved for phase 1 and phase 2 are in 44 days and 183 days respectively.
Table 6. Break-even point.
6. Results discussion
In the present investigation, the sustainability analysis of a sawmill industry is not only studied in an overall context but also, each and every activity have been viewed as it is environmentally conscious or the activities are just played to produce goods for the required quality to turn it as a profitable business. To produce a pine product of 6 × 2 inches in a sawmill industry, all the operational activities are initially observed and how much energy is being utilised for each activity are also found (). This data has been given as input () to the SOLIDWORKS software for initial sustainability analysis, which revealed that manufacturing is the major contributor () in carbon footprint, energy consumption, air acidification, and water eutrophication as 47.2, 77.9, 79, and 19 percentages respectively. Whereas, the rest of the attributes such as material, usage, end of life and transportation causes relatively negligible amount. The close observation of further reveals that of all the ‘environmental impact indexes’, the ‘energy consumption’ is a maximum contributing factor. Thus, the careful observation of all the activities () in the context of DFM/S principle was made. It was realised that in the sawmill subsystem, especially in the manufacturing processes; the P2 and P3 possess high power rated machines to perform an operation (). Consequently, there was an unnecessary buffer placed near the processor 2 (), which also consumes nearly 25 KW power. Thus, alternative machines for the respective processors were suggested (), which not only ensures to process for the chosen product in the investigation, but also for other product varieties that are taken in the sawmill industry. Accordingly, the new layout is proposed (), for which the energy requirement has been obtained and fed as data input to get the sustainability analysis for it (). The environmental impact comparison for the existing and the proposed setup is obtained from the software, which is shown in . This reveals that EC in manufacturing can be reduced by 18.8% and in CFP reduced by 13.3% and in AA reduced by 14.5% and in WE reduced by 15.8%.
While ensuring the solutions made to the manufacturing processes are manufacturable and sustainable, it is imperative to study whether the solutions are economically affordable. Thus, a cost analysis was carried out, in which all the necessary cost details such as fixed cost, variable costs, the selling price of the products and the total quantity produced from the existing facility were obtained (). These data are used to determine the break-even point, which revealed an important understanding is that the no loss no profit margin shall be attained in the shortest period of time and then it just yields to profit only. Another important observation is that these solutions are also lead to a higher production rate than the existing setup. For instance, the proposed motor at P2 has the flexibility to mount a maximum of 6 blades at a time that enables to cut timber of required dimension in one pass, subsequently, it helps to attain BEP earlier than the calculated figure.
7. Conclusion
The important facts that are understood out of the case study in a sawmill industry follow:
Sustainability analysis for the existing production process revealed that out of material, manufacturing, product usage, end of life and transportation, the manufacturing causes a maximum environmental impact in terms of carbon footprint, energy consumption, air acidification, and water eutrophication. More importantly, the impact of manufacturing in the energy consumption is much higher.
After surveyed the entire processes, it was determined that the processor 2 and processor 3 consume more energy than it is required. Consequently, the alternative solutions were identified and were ensured that it is manufacturable (for all the product varieties), sustainable (less energy consumes) and economical (able to reach the BEP in short period).
The sustainable analysis for the proposed production processes revealed that manufacturing in CFP, in energy consumption, in air acidification, and in water eutrophication respectively reduced about 13.3%, 18.75%, 14.5% and 15.78%.
7.1 Limitations and future work
The present investigation of sustainability analysis, although considers the entire production process, it is limited to particular product size, 6 × 2 inches. Importantly, this size is highly produced in the facility, so it has been chosen for the analysis. However, there are several other sizes are also being manufactured. Similarly, several other timber materials are also processed, each timber material initial log sizes are largely differ, for instance, mahogany is seven times bigger than the pine. Therefore, it is important to notify the practical limitation is that in this project only one particular timber material, in which one particular size has been considered to determine the alternative solutions. In addition, the energy or the power input for this analysis was assumed to be for full load capacity especially for sawmill subsystem; whereas in reality it may be expected to be less.
As mentioned before, in this investigation only pine and the size, 6 × 2 inches is considered for the sustainable analysis, which can further be extended to the waste management side as well. Apart from that, the forest subsystem shall also be considered to draft a plan to reduce the possible ways of fuel consumption. Moreover, the entire process shall be looked into the aspect of the lean system, by which the chances of improving the production cycle efficiency, reducing the wastes, reducing the energy shall be achieved. Finally, the investigation shall also be extended to perform sustainable analysis for other timber materials and sizes.
Acknowledgments
The authors would like to thank the management and staffs of the sawmill for accepting the proposal of carrying out the sustainability analysis on their product, as well as for rendering full cooperation in obtaining the required data throughout the phase of the project work.
Disclosure statement
No potential conflict of interest was reported by the authors.
Additional information
Notes on contributors
Jaishneel Krishant Sharma
Jaishneel Krishant Sharma is an MSc engineering student in the mechanical engineering division of The University of the South Pacific. His research interest include sustainable development in the manufacturing industries, operations management, lean system and process improvement.
Ananthanarayanan Rajeshkannan
Ananthanarayanan Rajeshkannan is an associate professor in mechanical engineering, FSTE at The University of the South Pacific. His research interest includes metal forming, operations management and tool design. He earned PhD, M.Tech and BE in Mechanical Engineering and put up vast research and academic experience in the tertiary institution.
Arnit Avinesh Sharma
Arnit Avinesh Sharma has completed his Professional B.E in Mechanical Engineering from The University of the South Pacific. His interest lies with operations management, particularly in the field of Environment Conscious product design, process design, MCDM.
Devi Rengamani Seenivasagam
Devi Rengamani Seenivasagam, earned her M.Com, M.Phil and PhD in General Management. She had put the vast experience in academic as well as in industry. Her research interest is in Marketing Management, Retail Management, Lean Management, Total Quality Management, MCDM, Supply Chain Management.
References
- Alemam, A., and S. Li. 2016. “Matrix Based Quality Tools for Concept Generation in Eco-Design.” Concurrent Engineering 24 (2): 113–128. doi:10.1177/1063293X15625097.
- Dyllick, T., and Z. Rost. 2017. “Towards True Product Sustainability.” Journal of Cleaner Production 162: 346–360. doi:10.1016/j.jclepro.2017.05.189.
- Econation for People and Planet. 2018. “Design for Sustainability.” Accessed August 10 2018. https://econation.co.nz/design-for-sustainability/
- Gebisa, A. W., and H. G. Lemu. 2017. “Design for Manufacturing to Design for Additive Manufacturing: Analysis of Implications for Design Optimality and Product Sustainability.” Procedia Manufacturing 13: 724–731. doi:10.1016/j.promfg.2017.09.120.
- Henao, R., W. Sarache, and I. Gomez. 2019. “Lean Manufacturing and Sustainable Performance: Trends and Future Challenges.” Journal of Cleaner Production 208: 99–116. doi:10.1016/j.jclepro.2018.10.116.
- Multi-blade Processor. 2018. “Multi Head Horizontal Style Resaw Band Saw for Wood Cutting.” Accessed May 5 2018. https://www.alibaba.com/product-detail/Multi-Head-Horizontal-Style-Resaw-Band_60711827917.html?spm=a2700.7724838.2017115.1.24d9168ch24O2K
- Reyes, J. A. G., J. T. Romero, K. Govindan, A. Cherraffi, and U. Ramanathan. 2018. “A PDCA-Based Approach to Environmental Value Stream Mapping (E-VSM).” Journal of Cleaner Production 180: 335–348. doi:10.1016/j.jclepro.2018.01.121.
- Romli, A., R. Setchi, P. Prickett, and M. P. de la Pisa. 2018. “Eco-Design Case-Based Reasoning Tool: The Integration of Ecological Quality Function Deployment and Case-Based Reasoning Methods for Supporting Sustainable Product Design.” Proceedings of the Institution of Mechanical Engineers, Part B: Journal of Engineering Manufacture 232 (10): 1778–1797. doi:10.1177/0954405416668928.
- Sakao, T. 2009. “Quality Engineering for Early Stage of Environmentally Conscious Design.” The TQM Journal 21 (2): 182–193. doi:10.1108/17542730910938164.
- Salari, M., and N. Bhuiyan. 2016. “A Proposed Approach to Improve Current Sustainable Product Development.” Journal of Industrial and Production Engineering 33 (5): 297–307. doi:10.1080/21681015.2016.1172122.
- Salonitis, K. 2015. “Energy Efficiency Assessment of Grinding Strategy.” International Journal of Energy Sector Management 9 (1): 20–37. doi:10.1108/IJESM-04-2013-0009.
- Schoormans, J. 2018. “Routledge Handbook of Sustainable Product Design.” The Design Journal 21: 553–557. doi:10.1080/14606925.2018.1467661.
- Seow, Y., N. Goffin, S. Rahimifard, and E. Woolley. 2016. “A ‘design for Energy Minimization’ Approach to Reduce Energy Consumption during the Manufacturing Phase.” Energy 109: 894–905. doi:10.1016/j.energy.2016.05.099.
- Single-blade Table Saw. 2018. “Wood Cutting Machine Rip Saw MJ153.” Accessed May 8 2018. https://www.alibaba.com/product-detail/wood-cutting-machines-rip-saw-MJ153_60620941471.html?spm=a2700.7724838.2017115.1.74ca4b64d6V2tV
- Stevenson, W. J. 2018. Operations Management. 13th ed. New York: McGraw Hill.
- Suresh, P., S. Ramabalan, and U. Natarajan. 2016. “Integration of DFE and DFMA for the Sustainable Development of an Automotive Component.” International Journal of Sustainable Engineering 9 (2): 107–118. doi:10.1080/19397038.2015.1096313.
- Sutherland, J. W., D. P. Adler, K. R. Haapala, and V. Kumar. 2008. “A Comparison of Manufacturing and Remanufacturing Energy Intensities with Application to Diesel Engine Production.” CIRP Annals 57 (1): 5–8. doi:10.1016/j.cirp.2008.03.004.
- UzZaman, A. 2015. “A Comprehensive Review of the Development of Zero Waste Management: Lessons Learned and Guidelines.” Journal of Cleaner Production 91: 12–25. doi:10.1016/j.jclepro.2014.12.013.
- Vinodh, S. 2010. “Sustainable Product Design Using CAD: A Case Study in an Indian Rotary Switches Manufacturing Organization.” International Journal of Sustainable Engineering 3 (3): 181–188. doi:10.1080/19397031003606494.
- Vinodh, S. 2011. “Environmental Conscious Product Design Using CAD and CAE.” Clean Technologies and Environmental Policy 13: 359–367. doi:10.1007/s10098-010-0310-8.
- Vinodh, S., and K. Jayakrishna. 2014. “Development of Integrated ECQFD, LCA and Sustainable Analysis Model, a Case Study in an Automotive Component Manufacturing Organization.” Journal of Engineering, Design and Technology 12 (1): 102–127. doi:10.1108/JEDT-07-2011-0052.
- Vinodh, S., and D. Rajanayagam. 2010. “CAD and DFM: Enablers of Sustainable Product Design.” International Journal of Sustainable Engineering 3 (4): 292–298. doi:10.1080/19397038.2010.501870.