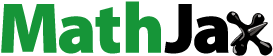
ABSTRACT
The existing drainage systems consider storm water as a waste product and its main focus is on collecting the runoff from urban catchments as quickly as possible and discharging it into the nearby outlets. These traditional drainage systems cannot consider important utilisation of storm water. Therefore, a decentralised storm water management option, known as, Water Sensitive Urban Design (WSUD) technologies, can be implemented in the urban catchments to minimise the negative hydrological impacts due to urbanisation. Hydraulic designs of three different types of infiltration-based WSUD technologies (leaky wells, soakaways and infiltration trenches) were designed for South Australian contexts. Modelling equations to estimate optimum numbering of infiltration systems were developed using SPSS and six different independent variables, such as soil hydraulic conductivity, size of the device, average recurrence interval of rainfall events, critical storm duration, rainfall intensity and roof size, were considered. The developed modelling equations were statistically significant and were applied in the real-case scenarios of South Australian catchments.
1. Introduction
Rapidly growing form of land-use change, in other words, urbanisation, has caused a reduction of catchment retention and infiltration of storm water runoffs in the urban landscape (de Haan, Rogers, and Brown Citation2015). Consequently, urbanisation greatly affects the flow regime in direct or indirect ways including: (i) increasing the magnitude and frequency of floods (Larenson et al. Citation2013; Winchester Citation2010); (ii) increasing the duration of rainfall runoff flows (Palla and Gnecco Citation2015); and (iii) altering regime flood and associated base flow statistics (Islam et al. Citation2011). In extend, urbanisation also diminishes the quality of stream runoff and water catchment (Rodriguez et al. Citation2011; Zhang et al. Citation2018). There is increasing evidence that the existing drainage system considers storm water as a waste product and is responsible for altering ecological cycle in the catchment (Argue Citation2004/2013).
Fundamentally, the flow regime takes account of the stream geomorphology and the aqua-ecosystem’s health and function. In order to alleviate the water quantity and quality issues of conventional urban drainage systems, recent research has suggested a conceptual idea of sustainability, which is focusing on source control, i.e. hold the rain where it falls (Argue Citation2004/2013; Mitchell Citation2006). The best practices are to retain storm water at upstream and keep evapotranspiration process undisturbed; thus, help to restore components of the hydrologic cycle (Liu, Cao, and Li Citation2017). The configuration of natural flow regime in today’s urban water system would be a difficult task. Subhashini, Hewa, and Pezzaniti (Citation2013) recommended mimicking critical components of the natural flow regime and implementation of those compositions would be a practical approach.
Fletcher et al. (Citation2014) reported that source-control specific storm water management techniques have different terms in different countries, i.e. Water Sensitive Urban Design (WSUD) in Australia, Low Impact Development (LID) in the United States, Sustainable Drainage System (SuDS) in the United Kingdom and Low Impact Urban Design and Development (LIUDD) in New Zealand. Some WSUD devices are designed primarily to manage storm water through evapotranspiration processes (Broadbent et al. Citation2017), i.e. green roofs, rain gardens and bio-swales. With more conservative WSUD devices, in other words, ‘Infiltration-based WSUD systems’, i.e. bio-retention systems, leaky wells, infiltration systems, permeable pavements and wetlands are designed to control storm water runoff through infiltration, storage and evapotranspiration (Larenson et al. Citation2013). The complete controls over storm water quantity and quality have been identified as an effective means of reducing channelisation into urban waterways (Bali et al. Citation2017; Bali and Tlili Citation2018) . Storm water management guidelines have been widely developed at the national and local governments’ level; however, there is still no affirmative document that supports WSUD system nationally as a set of objectives for storm water management in Australia (Saenz and Rodriguez Citation2016).
Three different types of infiltration systems are commonly used in Australia including leaky wells, sokaways and infiltration trenches (Ahammed Citation2017; Argue Citation2004/2013). Leaky wells are usually suitable for small-scale residential allotments; infiltration of storm water usually occurs using large diameter (around 2 m) perforated pipes or wells (Ahammed, Hewa, and Argue Citation2013). Soakaways are also underground infiltration structures filled with materials such as gravel or highly porous plastic media; they provide temporary storage for storm water runoff from roofs and allow infiltration of storm water into the surrounding soils (Roldin et al. Citation2012). According to Ahammed (Citation2017), excavations of infiltration trenches consist of 0.5 to 1 m deep; these are filled with modular plastic cells or sometimes with gravels and modular plastic cells are usually wrapped with geo-textile fabrics.
The objectives of storm water management may vary from low-flow conservation to flood protection with a binding purpose on water quality improvements (Shi et al. Citation2017). The implementation of WSUD systems in some local councils of Greater Adelaide regions, City of Onkaparinga and Salisbury in South Australia has achieved water quality objectives (Kazemi, Beecham, and Myers Citation2013; Radcliffe et al. Citation2017). Barton and Argue (Citation2009) reported 13 cases of residential projects those adopted WSUD technologies at the aim of achieving storm water quality and quantity in several major capital cities of Australia including Adelaide, Brisbane, Melbourne and Sydney. Current scientific literature continuously supports uses of WSUD simulation approach, which help in designing storm water source control from the determination of full range of rainfall variability. According to Tu and Traver (Citation2019), infiltration systems are suitable in the ultra-urban areas for flood management. Bai et al. (Citation2017) and Small et al. (Citation2019) noted that infiltration systems may have important functions beyond the flood management in the urban catchments, such as, removal of phosphorus and nitrogen, increasing aesthetics and quality of wildlife habitat.
This article aims to develop equations for optimum numbering and sizing of infiltration-based WSUD technologies for residential areas of South Australia. Hydraulic designs of WSUD were performed and modelling equations were developed using statistical techniques. Several real cases were also considered.
2. Methodology
2.1. Hydraulic designs of infiltration-based WSUD technologies
Three basic types of infiltration devices – leaky wells, soakaways and infiltration trenches () – are used in Australia (Dechesne, Barraud, and Bardin Citation2005; Argue Citation2004/2013). EquationEquations (1(1)
(1) )–(Equation3
(3)
(3) ) (Argue Citation2004/2013) are the basic formulae used for designing leaky wells, soakaways and infiltration trenches, respectively.
Where, D = diameter of leaky well,
A = area of soakaway,
e = void space ratio
L = length of infiltration trench,
b = width of infiltration trench,
H = height of leaky well/soakaway/infiltration trench,
V = critical storm water runoff volume,
Kh = soil hydraulic conductivity,
τ = critical storm duration (tc) + site time of concentration (ts),
U = moderation factor; 0.5, 1.0 and 2.0 for sandy, sandy clay and clay soil, respectively (Argue Citation2004/2013).
Soil hydraulic conductivity values are usually obtained from site tests on small test pits and boreholes (Hou et al. Citation2018). When these test results are applied to design infiltration-based WSUD devices, it is found that the systems are too big, where site soil is clay and too small, when site soil is sandy. This observation has led to the introduction of a correction factors termed as moderation factor (U).
It is important to ensure that the storage volume of the system is empty before the arrival of a succeeding significant storm (Meng et al. Citation2017; Tennakoon and Argue Citation2011). Emptying times of leaky wells, soakaways and infiltration trenches can be estimated using EquationEquations (4(4)
(4) )–(Equation6
(6)
(6) ) (Argue Citation2004/2013). The recommended emptying time is around 1 day for New Zealand (Auckland City Council Citation2003) and 3 days for the United States (Browne et al. Citation2008). In Australia, this value varies from 12 hours to 3.5 days depending on the flood frequencies of average recurrence interval (Argue Citation2004/2013).
2.2. Factors affecting optimum numbering and sizing of WSUD technologies
The optimum numbering of infiltration-based WSUD technologies for storm water management in South Australia depends on the following factors:
storm water management strategy,
dimensions of WSUD technologies,
rainfall intensity,
critical storm duration,
soil hydraulic conductivity,
average recurrence interval of rainfall events and
roof size.
Brief descriptions of above factors are shown in .
Table 1. Brief description of factors/criteria affecting optimum numbering of WSUD technologies
2.3. Development of modelling equations
The relationship of optimum numbering of infiltration-based WSUD technologies and related factors is shown in EquationEquation (7)(7)
(7) .
Where, N = number of WSUD units required,
S = storm water management strategy,
D = dimensions of WSUD technologies (L = length of infiltration trench, A = area of soakaway and D = diameter of leakywell)
I = rainfall intensity,
T = critical storm duration,
Kh = soil hydraulic conductivity
Y = average recurrence interval (or AEP annual exceedance probability of rainfall event),
RS = roof size.
The modelling equation for the optimum number of infiltration-based WSUD units required for storm water quantity control in South Australia was established as EquationEquation (8)(8)
(8) . Two scenarios of modelling equations for rainwater tank were considered to perform the modelling equation: i) without any rainwater tank and ii) with 1 kL rainwater tank.
Where, a, b, c, d, e, f = co-efficient,
g = constant.
2.4. Application of multiple regression analysis
Statistical Package for Social Science (SPSS – version 25.0) by IBM is a useful model for analytical study; two or more sets of data can be compared using SPSS to determine the statistical relationship or the strength of association between the data (Pascale and Pascale Citation1980; Massey Citation2010). Multiple regression using SPSS was performed to obtain modelling equations of infiltration-based WSUD technologies. Multiple regression process using SPSS is shown in . Land-use patterns of residential allotments of South Australia vary in terms soil type, roof size, slope of surface, percentage of impervious area and rainfall properties. Data for all possible scenarios of residential allotments mentioned in were entered into SPSS to perform multiple regression analysis.
3. Results and discussions
The outcomes of regression analysis using SPSS for infiltration trenches are shown in . The level of significance values for independent variables are less than 5% indicating that multiple regression equation for infiltration trench is statistically significant. As the p values of independent variable in are 0.000 for all cases and these are less than the threshold value (0.05), the developed modelling equations of infiltration-based WSUD technologies for residential areas of South Australia shown in EquationEquations (9)(9)
(9) –(11), are statistically significant.
Table 2. SPSS output of multiple regression analysis for infiltration trench
A portion of residential properties in South Australia consist of 1 kL rainwater tank. It has been a common practice to include at least 1 kL rainwater tank for future residential properties in South Australia. Many old residential properties also have the desire to include 1 kL rainwater tanks. The modelling equations of infiltration systems were further performed to include 1 kL rainwater tanks; the outcomes are shown in EquationEquations (12)(12)
(12) –(14). According to Razzaghmanesh et al. (Citation2018), inclusion of rainwater tank between roof and infiltration system may increase the life span of WSUD systems beyond 20 years.
Real case scenario of Mawson Lakes, South Australia, was considered to understand the optimum numbering and sizing of infiltration trench. The roof size for the plot 33 () was calculated using the google map as 333.27 m2. The soil type is sandy clay and the soil hydraulic conductivity (Kh) is 3 × 10−5 m/s. The considered length of trench is 8 m. Design criteria are based on 20 years ARI for storm duration of 20 min. The rainfall intensity by using the IFD relationships is 60.5 mm/h. The estimated number of infiltration trench required for the plot 33 using EquationEquation (9)(9)
(9) is 1.
Ten properties of Lockleys, South Australia, () were randomly selected to estimate optimum numbering and sizing of soakaways. The adopted length, width and height of soakaway were 4, 4 and 0.5 m, respectively. Soil type in Lockleys suburb is consider as sandy clay as Kh is 3 × 10−5 m/s. Design rainfall intensity was estimated from IFD relationship, using design storm and design critical storm duration. The results of optimum numbering of soakaways for Lockley suburb, South Australia is shown in . It is recommended that 5 kL rainwater tank can be estimated in addition of sokaways in Lockleys of South Australia.
Table 3. Optimum numbering of soakaways in Lockleys, South Australia
A real case to manage roof accepted runoff using leaky wells were considered for Morialta Conservation Park, South Australia (). The case study was conducted considering the minor and major events as 10 years ARI and 100 years ARI, respectively. The critical storm events considered are ranged from 5-min intervals started at 5 to 30 min. The leaky wells dimensions of 2, 2.2 and 2.5 m diameter have been taken into consideration in determining the number of leaky wells needed in different case scenarios. shows the required numbers of leaky wells with the provision of no rainwater tanks, while shows the required number of leaky wells to manage roof accepted runoff with the consideration of 1 kL rainwater tanks.
Table 4. Required number of leaky well to manage roof accepted runoff (without consideration of rainwater tank) in the case study area
Table 5. Required number of leaky well to manage roof accepted runoff (with consideration of rainwater tank) in the case study area
After the analysis, it was revealed that optimum sizes (length, height and width) of infiltration trench were 8, 1 and 1 m, respectively. Equal dimension of length and width for soakaway was adopted and the optimum dimension for residential areas of South Australia was observed as 4 m. The height and free board of soakaway were adopted as 0.5 and 0.3 m, respectively. The optimum diameter of leaky well varied from 2 to 2.5 m with 0.3 m free board.
4. Conclusions
Modelling equations for optimum numbering and sizing of three different types of infiltration systems including leaky wells, soakaways and infiltration trench were developed for South Australian context. SPSS was used to perform the regression equations and outputs confirmed that the modelling equations were statistically significant. The considered independent variables were soil hydraulic conductivity, size of the device, average recurrence interval of design storm, critical storm duration, rainfall intensity and roof size. The developed equations were also applied in the real-life case scenarios; provisions of with and without of rainwater tanks were also considered.
By implementing the WSUD technologies and preaching the principles not only support proper management and improvement of storm water quality and maintaining the quantity of the availability of water resources, but it can also reverse the negative impacts caused by urbanisation on the surrounding environment. There is significant shift evidently observed through literature in which it shows that application of WSUD guidelines and strategies during the initial stage of urban planning and design may reduce the stress and dependency on the water resources and degradation of the environment. The public attitudes and perceptions towards the storm water runoff should change and have a positive outlook by considering these resources as a useful amenity and not ‘nuisance’.
The WSUD principles, strategies and procedures applied for this study may prove to be an effective approach for sustainably managing storm water runoff in residential areas located in South Australia. The same design methodological framework applied for infiltration systems in this article can be implemented in other residential areas and cities.
Disclosure statement
No potential conflict of interest was reported by the authors.
Additional information
Notes on contributors
Faisal Ahammed
Dr Faisal Ahammed is a Senior Lecturer and Program Director of Civil Engineering Postgraduate Program at the University of South Australia (UniSA). He completed BSc Civil Engineering and Master of Advanced Engineering Management degree from Bangladesh University of Engineering and Technology in 2002 and 2009 respectively. He completed his PhD degree on Water Engineering from UniSA in 2014. He obtained several fellowships in his career including Early Career Development Fellowship and Endeavour Fellowship.
George Rohita Sara
George Rohita Sara completed her Master of Engineering (Civil and Infrastructure) degree from the University of South Australia in December 2018.
Hii Paul Kai
Hii Paul Kai completed his Master of Engineering (Civil and Infrastructure) degree from the University of South Australia in December 2018.
Li Yan
Li Yan completed her Master of Engineering (Civil and Infrastructure) degree from the University of South Australia in December 2018.
References
- Ahammed, F. 2017. “A Review of Water-sensitive Urban Design Technologies and Practices for Sustainable Stormwater Management.” Sustainable Water Resources Management 3: 269–282. doi:10.1007/s40899-017-0093-8.
- Ahammed, F., G. A. Hewa, and J. R. Argue. 2013. “Introducing Laky Well Concept for Stormwater Quantity Control for Dhaka, Bangladesh.” Applied Water Science 3 (1): 115–123. doi:10.1007/s13201-012-0065-y.
- Argue, J. R. 2004/2013. Water Sensitive Urban Design: Basic Procedure for Source Control of Stormwater: A Handbook for Australian Practice. Adelaide, Australia: Urban Water Resource Centre, University of South Australia.
- Auckland City Council. 2003. Soakage Design Manual. New Zealand: Auckland City Council.
- Bai, M., D. Xu, Y. Li, S. Zhang, and S. Liu. 2017. “Coupled Impact of Spatial Variability of Infiltration and Microtopography on Basin Irrigation Performances.” Irrigation Science 35 (5): 437–449. doi:10.1007/s00271-017-0550-z.
- Bali, M., H. Jridi, S. Farhat, B. Louhichi, and R. Boukchina. 2017. “Treatment of Secondary Effluents by Infiltration-percolation Process Using Sand Fortified with Activated Charcoal.” International Journal of Environmental Science and Technology 14 (6): 1209–1216. doi:10.1007/s13762-016-1222-x.
- Bali, M., and H. Tlili. 2018. “Removal of Heavy Metals from Wastewater Using Infiltration-percolation Process and Adsorption on Activated Carbon.” International Journal of Environmental Science and Technology 16 (1): 249–258. doi:10.1007/s13762-018-1663-5.
- Barton, A. B., and J. R. Argue. 2009. “Integrated Urban Water Management for Residential Areas: A Reuse Model.” Water Science and Technology 60 (3): 813–823. doi:10.2166/wst.2009.401.
- Broadbent, A. M., A. M. Coutts, N. J. Tapper, M. Demuzere, and J. Beringer. 2017. “The Microscale Cooling Effects of Water Sensitive Urban Design and Irrigation in a Suburban Environment.” Theoretical and Applied Climatology 134 (1–2): 1–23. doi:10.1007/s00704-017-2241-3.
- Browne, D., A. Deletic, G. M. Mudd, and T. D. Fletcher. 2008. “A New Saturated/unsaturated Model for Stormwater Infiltration Systems.” Hydrological Processes 22 (25): 4838–4849. doi:10.1002/hyp.v22:25.
- de Haan, F. J., B. C. Rogers, N. Frantzeskaki, and R. R. Brown. 2015. “Transitions through a Lens of Urban Water.” Environmental Innovation and Societal Transitions 15: 1–10. doi:10.1016/j.eist.2014.11.005.
- Dechesne, M., S. Barraud, and J. P. Bardin. 2005. “Experimental Assessment of Stormwater Infiltration Basin Evaluation.” Journal of Environmental Engineering 131 (7): 1090–1098. doi:10.1061/(ASCE)0733-9372(2005)131:7(1090).
- Fletcher, T. D., W. Shuster, W. F. Hunt, R. Ashley, D. Butler, and S. Arthur. 2014. “SUDS, LID, BMPs, WSUD and More – The Evolution and Application of Terminology Surrounding Urban Drainage.” Urban Water Journal 1 (1): 1–18.
- Hou, L., B. X. Hu, M. He, X. Xu, and W. Zhang. 2018. “Effect of Intermittent Operation Model on the Function of Soil Infiltration System.” Environmental Science and Pollution Research 25 (10): 9615–9625. doi:10.1007/s11356-018-1238-5.
- Islam, M M.., F. N.-F.. Chou, and M. R. Kabir. 2011. “Feasibility and Acceptability Study Of Rainwater Use to The Acute Water Shortage Areas in Dhaka City, Bangladesh.” Natural Hazards 56 (1): 93-111. doi:10.1007/s11069-010-9551-4.
- Kazemi, F., S. Beecham, and B. Myers. 2013. “Water Quality Effects of a Water Sensitive Urban Design Retrofit in an Urban Streetscape in Adelaide, Australia.” Acta Horticulture 999: 321–327. doi:10.17660/ActaHortic.2013.999.46.
- Larenson, G., S. Laurenson, N. Bolan, S. Beecham, and I. Clark. 2013. “The Role of Bioretention Systems in the Treatment of Stormwater.” Advances in Agronomy 120: 223–274.
- Liu, B., W. Cao, and Y. Li. 2017. “Evaluation of Turfgrid Porous Pavements for Urban Stormwater Management in Xiamen Island, Southeast China.” Sustainable Water Resources Management 4 (3): 617–626. doi:10.1007/s40899-017-0147-y.
- Massey, D. S. 2010. “Special Issue of 21st Century Society: Journal of the Academy of Social Sciences: Climate Change in the Social Sciences.” Twenty-First Century Society 5 (2): 194.
- Meng, C., J. Zhou, M. Dai, S. Zhu, X. Xue, and L. Ye. 2017. “Variable Infiltration Capacity Model with BGSA-based Wavelet Neural Network.” Stochastic Environmental Research and Risk Assessment 31 (7): 1871–1885. doi:10.1007/s00477-017-1413-0.
- Mitchell, V. 2006. “Applying Integrated Urban Water Management Concepts: A Review of Australian Experience.” Environmental Management 37 (5): 589–605. doi:10.1007/s00267-004-0252-1.
- Palla, A., and I. Gnecco. 2015. “Hydrologic Modeling of Low Impact Development Systems at the Urban Catchment Scale.” Journal of Hydrology 528 (1): 361–368. doi:10.1016/j.jhydrol.2015.06.050.
- Pascale, P. J., and J. S. Pascale. 1980. “Item Analysis Using the Statistical Package for the Social Sciences (SPSS).” Educational and Psychological Measurement 40 (1): 163–164. doi:10.1177/001316448004000126.
- Radcliffe, J. C., D. Page, B. Naumann, and P. Dillon. 2017. “Fifty Years of Water Sensitive Urban Design, Salisbury, South Australia.” Frontiers of Environmental Science & Engineering 11 (4): 7. doi:10.1007/s11783-017-0937-3.
- Razzaghmanesh, M., and S. Beecham. 2018. “A Review of Permeable Pavement Clogging Investigations and Recommended Maintenance Regimes.” Water 10 (3): 337. doi:10.3390/w10030337.
- Rodriguez, D. B., E. J. Carter, M. Bryant, S. Diemont, and R. Smardon. 2011. Sustainability Assessment of Green Infrastructure Practices for Stormwater Management: A Comparative Emergy Analysis. New York: UMI Dissertation Publishing.
- Roldin, M., O. Mark, G. Kuczera, P. S. Mikkelsen, and P. J. Binning. 2012. “Representing Soakaways in a Physically Distributed Urban Drainage Model –upscaling Individual Allotments to an Aggregated Scale.” Journal of Hydrology 414–415: 530–538. doi:10.1016/j.jhydrol.2011.11.030.
- Saenz, Q. A., and G. A. Rodríguez. 2016. “Guidelines for Inclusion: Ensuring Indigenous Peoples’ Involvement in Water Planning Processes across South Eastern Australia.” Journal of Hydrology 542: 828–835. doi:10.1016/j.jhydrol.2016.09.050.
- Shi, X., S. Quilty, T. Long, A. Jayakaran, L. Fay, and G. Xu. 2017. “Managing Airport Stormwater Containing Deicers: Challenges and Opportunities.” Frontiers of Structural and Civil Engineering 11 (1): 35–46. doi:10.1007/s11709-016-0366-6.
- Small, G. E., E. Q. Niederluecke, P. Shrestha, B. D. Janke, and J. C. Finlay. 2019. “The Effects of Infiltration-based Stormwater Best Management Practices on the Hydrology and Phosphorus Budget of a Eutrophic Urban Lake.” Land and Reservoir Management 35 (1): 38–50. doi:10.1080/10402381.2018.1514549.
- Subhashini, W. H. C., G. A. Hewa, and D. Pezzaniti. 2013. Assessing the Ability of Infiltration-based WSUD Systems to Manage Channel-forming Flow Regimes in Greenfield Catchment Developments: A Catchment Scale Investigation. Australia: Modelling and Simulation Society of Australia and New Zealand Inc.
- Tennakoon, A., and J. R. Argue. 2011. “Managing Urban Regrowth with an at Capacity Stormwater Infrastructure”. Proceedings of the 34th IAHR World Congress and 33rd Hydrology and Water Resources Symposium, Brisbane, Australia, 2720–2727.
- Tu, M. C., and R. G. Traver. 2019. “Optimal Configuration of an Underdrain Delivery System for a Stormwater Infiltration Trench.” Journal of Irrigation and Drainage Engineering 145 (8): 05019007. doi:10.1061/(ASCE)IR.1943-4774.0001408.
- Winchester, T. 2010. A Water Quality Comparison of Stormwater Runoff from Surface and Subsurface Drainage Systems. Canada: Patrimoine de l’édition Heritage Branch.
- Zhang, X., Y. Lei, Y. Yuan, J. Gao, Y. Jiang, Z. Xu, and S. Zhao. 2018. “Enhanced Removal Performance of Cr(VI) by the Core-shell Zeolites/layered Double Hydroxides (Ldhs) Synthesized from Different Metal Compounds in Constructed Rapid Infiltration Systems.” Environmental Science and Pollution Research 25 (10): 9759–9770. doi:10.1007/s11356-018-1303-0.