ABSTRACT
This study provides an overview of the current energy deficit in Nigeria due to the massive challenges linked to the energy value-chain particularly the generation, distribution and decentralisation in Nigeria. The review discusses in detail, the possibility of using alternative resources to increase the usage of clean energy. The resources are represented by a huge biomass potential and solar energy availability in the country, which can be harnessed to solve the energy challenges. Therefore, solar-biomass pyrolysis technology was suggested as an appropriate technology to harness the combined energy potential presented by solar energy and biomass. This review helps to make the best design, choice, and adoption of suitable technology approaches for Nigeria. Moreover, the existing research on solar-biomass pyrolysis design architecture, configurations, were also reviewed. The choice of incorporating a solar-pyrolysis system for energy supplies and environmental resilience will be a novel attempt in the energy model using the Nigeria case.
1. Introduction
The global energy demand will continue to increase as the world population is projected to increase to about 9.3 billion people in 2050 (Crossette Citation2011). The primary concern has been the global decrease of the fossil-fuel reserves with its consequences on the environment. However, exploring new renewable energy resources and distributed generation model will bridge the global energy gap (Energy Commission of Nigeria (ECN) Citation2013). Nigerian refineries are located just in three states out of the 36 states of the federation while imported fuel mostly come through the lagos and portharcourt ports. This has created distribution challenge in terms of transportation and logistics. Location of biofuel plants clusters close to customers or in area that will make for easy supply linkage will help to brigde the energy gap. Development of an energy co-conversion generation technology for renewable energy sub-systems integration in a smart grid model will provide opportunities for energy clusters in Nigeria for power generation too (Ohunakin et al. Citation2014). These can serve as emergency power in terms of power generation. However, for a country like Nigeria, the solution to the energy need must also involve transportable fuel for both companies and households far from the electricity grid due to low electricity penetration density. From all perspectives, renewable energy (RE) resources such as solar and biomass and its co-conversion to biofuel are promising. These two energy resources have been considered sustainable and can play a key role in solving the Nigerian energy deficit (Salman, Nomanbhay, and Foo Citation2018). Looking at the solar energy potential of Nigeria, it is readily available and easily adaptable to support human activities. However, the major challenge is its intermittent nature and the availability of technology to capture the radiations, store and transport it in its present form. The prospects of solar energy application directly in complete vehicular, sea or air transportation and electric power generation require a massive local investment in solar photovoltaic cells, modules, and arrays. Such a transport system requires a well-configured infrastructural architecture to operate which, Nigeria does not have. Therefore, sub-system integration of solar and biomass to produce biofuel has been exploited.
In Nigeria, the focused technologies for biofuel production are extraction, fermentation, and anaerobic digestion to produce biogas and bioethanol (Ben-Iwo, Manovic, and Longhurst Citation2016). Biodiesel production is at the pilot scale and cost-effectiveness of the processes still ongoing (Ohimain Citation2010). However, few studies abound on thermal decomposition of biomass via pyrolysis or gasification (Salman, Nomanbhay, and Salema Citation2019). These are the only technologies that can have the capacity to process all the biomolecules (Barber and Warnken Citation2008). The process provides a bio-yield of very high energy density, which can be stored and transported (Lehmann, and Joseph Citation2009). It produces biodiesel, biogas, bio-oil, and biochar (Deal et al. Citation2011; Vamvuka Citation2011).
The benefit of biofuel in Nigeria’s energy mix includes lessening carbon emission by its incorporation into the petrol product to replace the octane enhancers (Ben-Iwo, Manovic, and Longhurst Citation2016). Biodiesel can drive the transport and industrial sectors. At the same time, biogas can replace the cooking gases and fuel to electric thermal plants (Ben-Iwo, Manovic, and Longhurst Citation2016). Additionally, biochar can be used as fertiliser. This will create employment and generate revenue that will impact positively on the economy. Several researches have been conducted regarding RE potential in Nigeria (Ibitoye and Adenikinju Citation2007). Onyeka, Taylan, and Baker (Citation2016) highlighted the potentials of utilising solar energy for electricity generation in Onitsha, Kano, and Lagos. Olatomiwa et al. (Citation2016) investigated on various configurations of the hybrid RE systems for electric power generation. A comprehensive review of solar and biomass potential for clean energy generation and the opportunity it presents for the country to achieve sustainable energy generation in Nigeria has been presented by Giwaa et al. (Citation2017). In line with the sustainable energy goal, Dioha and Kumar (Citation2020) reviewed a system model for future sustainable energy pathways for the Nigerian household sector. Chanchangi et al. (Citation2020) presented the obstacle posed by poor PV management in achieving Nigeria’s renewable energy potential. A detailed review of the past, current, and future status of solar integration in Nigeria, was reported by Ozoegwu, Mgbemene, and Ozor (Citation2017), while Ben-Iwo, Manovic, and Longhurst (Citation2016) reviewed biomass potentials in Nigeria. Most recently, Ogbonnaya et al. (Citation2020) looked at upcoming technologies for power generation in Nigeria. The authors presented an up to date summary of past review work on different framework focusing on the renewable potential in Nigeria from 2001 to 2018.
Overall, there are significant amounts of scientific literature regarding RE sources in Nigeria as shown in . However, none of these reviews emphatically addressed the energy generation system for biofuel with a clear energy co-conversion technology model for distributed renewable energy resources in Nigeria with available designs to explore to create energy pathways for energy sustainability in the country. According to Ohunakin et al. (Citation2014), renewable generation can be managed properly, controlled and transportable, or more grid-friendly, by adopting novel system design and sustainable operational technology at the generation level. For Nigeria, different operational technology and grid systems are still reviewed, discussed and suggestions made. The approach for most review is to highlight the challenges, update the information on the renewable energy potential of the country, present available conversion technology as previously discussed and finally dwelling on a new technology approach. Most recently, Ogbonnaya et al. (Citation2020) has proposed distributed hybrid/integrated power systems and introduction of hydrogen-based energy systems conversion model. However, the choice of solar-biomass pyrolysis system advocacy in this review for energy supplies and environmental resilience is a novel attempt in defining a new energy generation model for Nigeria in the face of hostile interest on fosile-based energy globally. This represents a relevant and significant contribution to knowledge. Most of the existing studies are geared mainly to achieve stable electricity, nevertheless fail to identify the best combination of bioenergy resource and approaches needed to ensure sustainable and eco-friendly high-density energy yield that can serve all sectors of the Nigeria economy including electricity, transport, and household. This knowledge gap is a setback for the advancement of clean and RE in Nigeria. Additionally, the techno-economic and environmental feasibility of adopting a new RE technological approach and model for any country is tied to the country’s RE policy, drivers, and barriers, available RE resources, technological choice and readiness, implementation strategy, economics, environmental, health, and safety considerations. Therefore, this review does not only bring holistically all the energy decision components together but also aims to advocate the synergising of the abundant solar energy and biomass resources in the country to produce environmentally clean and high energy density fuel, which can be utilised in all sectors of the Nigerian economy.
Table 1. Summary of previous literature review on renewable resources issues in nigeria and the review gap to be filled
2. Overview of Nigeria energy deficit
Nigeria has a landmass of 923,768 km2 (World Bank. Fiscal statistics Citation2017) and a population density of 212.04/km2. Although crude petroleum contributes over 70% of its revenue, the country imports over 70% of its domestic fuel needs (Nigerian Bureau of Statistics (NBS) Citation2018). This raises the countries import bill with the government subsidising the oil imports (Nigerian Bureau of Statistics (NBS) Citation2018). Regardless of this situation, Nigeria is suffering from an energy deficit, as shown in . This deficit affects the economy of the country and the daily household consumption.
shows that Nigeria produced 70,140 bb/day of refined petroleum product in 2014 but consumed 316,000 in 2015 (2018). Between 2003 and 2009, Nigeria energy consumption per capita decreased from 174.6 kWh/capita to 135.2 kWh/capita, while African and global average consumption is about 563–571 kWh/capita and 2596–2782 kWh/capita, respectively (Bala and Pam Citation2012). At this same period, the energy intensity decreased from 0.244 to 0.075 kg toe/US$ (Bala and Pam Citation2012). The energy deficit shown in is linked to the household and was estimated by the National Energy Consumption (NEC) model and reported by a National Energy Study for the industrialisation of Nigeria (Ladan Citation2009). This report took into consideration the line tendency of the government in terms of the whole economy and the entire energy sector. It shows the over-reliance of the households on wood as the primary source of energy for cooking and heating, which is the major cause of deforestation. From , the International Energy Agency report revealed that the residential home is the major consumer of the primary energy in Nigeria (International Energy Agency (IEA) Citation2018). Most of this energy comes from firewood and other off-grid energy.
Figure 2. Nigerian energy consumption by the economic sector (Ktoe) in 2013. Adapted from (International Energy Agency (IEA) Citation2018)
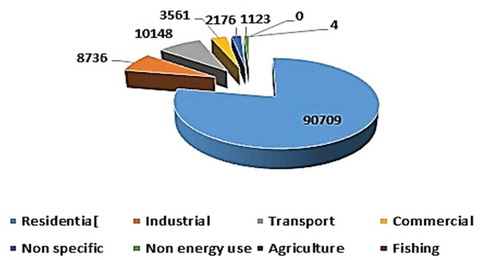
Table 2. Nigeria energy profile 2013–2018 (Nigeria Energy Future Citation2015)
Table 3. NEC calculations for the Household (HH) sector in 2006 (Ladan Citation2009)
In another report reported by Sambo (Citation2009) the rural areas have low electricity penetration and petroleum products. Therefore, these regions depend entirely on wood fuel. Nigeria has failed to move with the current global energy trend into the tapping of its abundant RE potential. Most of Nigeria’s thermal plants are natural gas-fired. These plants frequently suffer system failures partly due to gas supply cut by restive militants in the Niger Delta region (CitationEnergypeadia). Regardless of this situation, Nigeria continued to give licence to independent power plants (IPP), which depend heavily on conventional fuel as shown in (Ladan Citation2009)
Table 4. Some of the licenced and operational IPP’s in Nigeria and their capacities (Ladan Citation2009)
2.1. Overview of biofuel production capacity
This section discusses the availability of biofuel production in utilisation in Nigeria. Currently, the market of biofuel in Nigeria is foreign dependent on about 1.3 billion litres of ethanol fuel demand in 2007 and a planned capacity increase to 2 billion litres by 2020 (Ben-Iwo, Manovic, and Longhurst Citation2016; Galadima et al. Citation2011). Within the period under review, the biodiesel demand was 480 million litres, with an anticipated demand of 900 million litres by the year 2020 (Azil Citation2007). These quantities reflect the huge market, which can be considered as a good market for foreign investors because only 133 million litres of ethanol was locally produced by 2010 (Elijah Citation2010). The government has made efforts to establish the Renewable Energy Division as a subsidiary of Nigeria National Petroleum Company (NNPC) in August 2005 with the responsibility to expand resources and train staff in RE integration. However, nothing concrete has been achieved, in terms of local production. They entered into some partnerships with private ventures to produce automotive biofuel or bioethanol, which is still at the planning or the conception stage, as shown in . Also, the mantle for biofuel research has been taken by the universities with the establishment of the Centre for Energy Research and Development, Obafemi Awolowo University, Ile-Ife, Centre for Energy Research and Training, Ahmadu Bello University, Zaria, National Centre for Energy Research and Development, University of Nigeria, Nsukka, and Sokoto Energy Research Centre, Usman Dan-Fodio University, Sokoto. These centres were saddled with the responsibility of working in different RE resources (Ladan Citation2009). Currently, there are no bio-fuel fired thermal plants in the country. Therefore, numerous perspectives exist from the generation of biomass resources into electricity generation and other industrial effluents such as ethanol production, biogas generation and bio-fertiliser production.
Table 5. Biofuel projects in Nigeria (Ohimain Citation2010)
3. A review of previous studies on Nigeria biomass potential
Sharma, Vishnu, and Dongke, (Citation2015) defined biomass as a mixture of a hydrocarbon comprising of carbon, hydrogen, oxygen and other trace elements such as sulphur and nitrogen. Commonly, biomass consists of polymeric materials, extractives, and minerals (Yaman Citation2004). The content of organic and inorganic constituents can vary from 1 to 50% depending on the type (Fitz et al. Citation1996; Fapetu Citation2000). Biomass can be generated from agricultural wastes, energy crops, industrial processes, household activities, woodchips, and refuse dumping. Biomass can further be classified into woody, non-woody, or processed waste. Several authors have conducted a systematic literature review of biomass potential in Nigeria. However, these authors identified several biomass and reaching several disaggregated conclusion. This section is intended to collate them and aggregate them for proper insight. Abila (Citation2010) conducted a review to identify the biomass potential available in Nigeria in 2010. He identified wood chips, energy crops, crop residues, shrub residues, animal and aquatic wastes, and municipal wastes as the major-identified biomass potential. The authors concluded by separating the biomass available into regions and concluded that the tropical rainforest located in the south produces most woody biomass, while in the north; the savannah produces more crop residues. This crop residues have been found to be generated from sugar cane, cassava, sorghum, soybean, maize, oil palm, millet, cocoa bean, etc. and can produce 110.2 to 15500 million litres of biodiesel per year (Ogwueleka Citation2009). Ngumah et al. (Citation2013) reviewed municipal west availability in Nigeria and the potential to generate biofuel. They reported that Nigeria produces about 542.5 million tons of biological waste, with the capacity to produce 25.53 billion m3 of biogas and 88.19 million tons of bio-fertiliser, at a projected revenue generation of about 29.29 USD billion. Another review estimated that 206.1 million tons of dry biomass can be obtained from residues from forage, shrub, crops, and municipal wastes in Nigeria (Ikuponisi Citation2004). Jekayinfa and Scholz, (Citation2007) estimated the total animal residues available in the country to be above 5.36 × 109 m3. In another review report, Nigeria can produce an estimated 0.23 million tons of animal waste daily, which has the potential of yielding around 6.8 million m3 of biogas daily (Akinbami Citation2001). Bala (Citation2012) reviewed the biomass availability in Nigeria and reported that as at 2012 animal wastes dominated the bio-waste generation in Nigeria with 245 million tonnes generated per year as shown in . Waste from the wood industry was identified as another huge source of biomass in Nigeria by Ogunwusi and Joalaoso (Citation2012) and Ohunakin (Citation2010) in their own review. As of 1993, Nigeria generated about 3.87 million m3 of wood waste out of which 20–30 % is saw dust and these values increased by 1.8 million tonnes per year of sawdust in 2009. Simonyan and Fasina (Citation2013) Provided insight into huge biomass resources and the need to focus on the conversion technology. They identified rice straw, maize husk, stalk and cob, cassava peelings, groundnut shell and pods, soya bean pods and straws, cotton stalk, sugarcane leaves, millet and sorghum straw, cowpea shell and palm fruit residues as abundant biomass in Nigeria that can be utilised for energy conversion. Jekayinfa et al. (2020) in their review identified several wood and woody biomass, Herbaceous Biomass, Agricultural Residues from coconut, fruits and vegetables ground nut, maize, etc., available in Nigeria. Giwaa et al. (Citation2017) identified Jatropha with high oil contents, sweet sorghum and molasses; food crops such as cassava, rice, coconut, cashew, millet, rice, oil palm, maize, and yam; agricultural residues and wastes from food crops such as cassava peels, cassava liquid sludge, mango peel, mango seed kernel, rice husks (RH), corn residues, oil palm derivatives, sugar cane straw, and bagasse; forest resources; municipal solid wastes; and animal wastes as available biomass potentials in Nigeria. This concluded that the resources can be exploited to support different kinds of biofuel production. They concluded that despite all these biomas potential, implementation technology for conversion to more useful energy is still lacking. Ndukwu, Akani, and Simonyan (Citation2015) identified that In the last 10 years, Nigeria produced about 3.8 million tonnes of milled rice per year with husk heaped all over the rice-producing states. Using biomass to generate electricity or biofuel will present an independent opportunity for agricultural sectors especially the big farms to be auto sufficient in terms of electricity consumption. The Nigerian government has projected about 5–100 MW biomass electricity generations, while the government will invest in 500–8000 bio-digesters between 2012 and 2030 (Bala and Pam Citation2012)
Figure 3. Available bio-waste resources (million tons/year) and the projected amounts in Nigeria. Adapted from (Bala Citation2012)
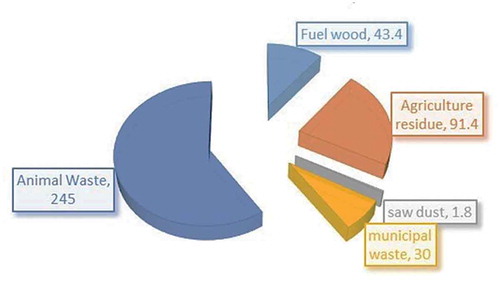
4. A review of previous studies on the potential of Nigeria in solar energy
Solar radiation is electromagnetic radiation of variable wavelengths existing from μ-rays of 10−6μm to radio waves of 108 μm wavelength (Chukwujindu Citation2017). This electromagnetic radiation is a function of not only meteorological parameters but also geographical, astronomical, and physical parameters such as temperature, sunshine hours, humidity, precipitation, geographical elevation, sun azimuth angle, the distance of the earth from the sun, amount of dust particles in the air, and composition of gases in the air (Chukwujindu Citation2017). Solar radiation plays a role in plant food synthesis, heating up of the atmosphere, creates the differential pressure for driving the land and sea breeze, drives the earth water cycles, dry agricultural products and plays a role in human health therapy (Chukwujindu Citation2017). , showed that parts of Nigeria lie on a high sunshine belt with a mean annual incident solar radiation of 1,831.06 kWh and average daily value of 5.25 kWh/m2 around the coastal areas and 7.0 kWh/m2 around the Sahel area (Abam. et al. Citation2014). The mean daily sunshine duration is 3.5 h for the coastal regions and 9.0 h towards the savannah north (Ohunakin Citation2010).
Figure4. Global horizontal irradiation for Nigeria. Source: (Solargis, Citation2018)
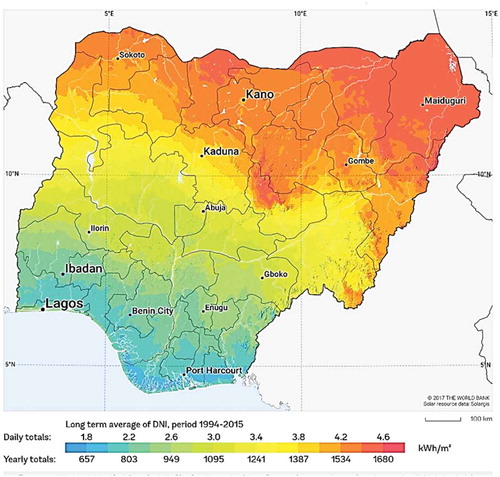
It is reported that solar modules covering 1% of Nigeria’s landmass can generate up to 627,260 MW of electricity yearly at 5% efficiency (Uzoma et al. Citation2011) compared to the current power generation of 6803 MW. This capacity is enough to power the country’s energy needs up to 2050. Nigeria’s energy mix is dominated by gas and hydroelectricity in that order. Still, there is an ambitious plan to introduce 40,000 MW of solar electricity by 2030 (Sambo Citation2009). The projected values have not been met, although some solar electricity projects are going on in Nigeria (Nwofe Citation2014). Solar energy utilisation in Nigeria has two main applications: either solar thermal systems or photovoltaic (PV) systems (Riti and Shu Citation2016). However, the technology for the former is easily adaptable for drying purposes (Ndukwu and Bennamoun Citation2017; Ndukwu et al. Citation2017) in particular agricultural products, wood, and its derivative biomass. The pilot schemes of the various arms of the country’s energy commission have executed some PV – installations around the country between 1999 to date, with an installed capacity of 6 MW (Riti and Shu Citation2016). These installations are mainly for streetlights and water pumping. Other pilot projects have been carried out in the area of solar drying, cooking, water boiling, incubators, etc., by Sokoto Energy Research Centre (SERC), National Centre for Energy Research and Development (NCERD), and independent researchers (Ndukwu, Bennamoun, and Abam Citation2018). In the 2012–2030 RE action plan, Nigeria targeted to deploy between 150 and 6000 solar dryers (Bala Citation2013). None of these steps has yielded any results in solving the energy deficit in Nigeria. The high cost of PV modules and inverters are also a problem for small-scale energy generators to engage solar application systems.
5. Review of the technology pathways availability for biomass conversion to biofuel in Nigeria
shows the pathways for bio-waste conversion available for Nigeria that includes pyrolysis, gasification, extraction, and fermentation based on available biomass feedstock. The chosen technology for a conversion process depends on the type of biomass, quantity existing, available primary energy for the conversion process, desired output, type of reactor available, particle size, feedstock distribution, cost, and environmental factors (Uzoma et al. Citation2011). Among the existing biomass conversion processes, only pyrolysis and gasification can process all the biomolecules, as shown in . However, direct combustion accounts for most of the global bioenergy generation especially in thermal plants for electricity generation. Nevertheless, the major limitation is that it cannot be used in biofuel production, which can be used in other sectors (Demirba, Citation2008; Elbehri, Segerstedt, and Liu Citation2013). Pyrolysis process decomposes biomass at a temperature of 300–600°C in the absence of oxygen in an inert atmosphere (Ringer, Putsche and Scahill, Citation2006). The process produces a liquid of about 75 wt %, which can be useful as high-density energy carriers with broad industrial applications (Morales et al. Citation2014; Papari and Hawboldt Citation2015). Gasification process partially oxidises biomass at a temperature above 800°C to produce syngas that can be used in gas engines and turbines (Simonyan and Fasina Citation2013).
Table 6. Primary conversion technologies for biological materials (Simonyan and Fasina Citation2013)
Presently, the technology for anaerobic digestion, extraction, and fermentation on a pilot scale has been explored in the country with the capacity for constructing floating dome and fixed dome bio-digesters using various biomass sources (Sambo Citation2009). Some of these pilot plants are human faeces and cow dung biogas plants located in Zaria and 18 m3 capacity pig waste biogas plant located in Lagos State (Sambo Citation2009). Technologies for biofuel production via the thermal reduction of biomass have not fully been exploited in the country. The major reason might be the use of conventional fuel for heating. One of the proposed approaches to reduce the application of conventional fuel in the process is the use of solar energy, which is available in abundance in the country. This approach could make the process completely eco-friendly. Thermal degradation involves the decomposition of biopolymers available in the biomass in an inert atmosphere with the application of heat (Heidari et al. Citation2014). This process was initially used in the decomposition of coal (Elijah Citation2010; Tyler Citation1979; Brownsort Citation2009; Antal and Grønli Citation2003) and seeing the benefits, the process was extended to biomass decomposition. During pyrolysis, the yield of liquid biofuels is majorly due to hemicellulose and cellulose decomposition while Lignin degenerates to liquid, gas, and solid biochar (Meier and Faix Citation1999; Wiktorsson and Wanzl, Citation2000). Minerals are retained as ash in the biochar, while the extractives contribute to gas and liquid by-products by decomposition or volatilisation (Bala, Citation2011). This process takes place in a reactor, which can be fixed or fluidised beds, ablative systems, kilns and drums, plasma type, auger type, microwave or free-fall type of different configurations and operating modes (Bridgwater, Citation2011; Basu Citation2010). However, the fluidised beds and the auger reactors are the most common type of reactors because of the rapid temperature rise of the feedstock due to high heat and mass transfer between the material and its environment (Casa, Canga, Díez and Alvarez, Citation2005).
5.1. Integration of solar and biomass renewable energy sub-system for biofuel generation: a co- conversion approach for biofuel production
Thermal decomposition requires an external source of energy to reach the needed high temperatures (Nzihou, Flamant, and Stanmore Citation2012). This external source is always derived from conventional fuel. However, research has shown that this external energy can be replaced with eco-friendly solar heat (Hopkins, Dejenga, and Antal Citation1985). This can be performed by concentrating the solar radiation into a reactor using optical devices, which will help to reach the desired temperature inside the reactor (Joardder et al. Citation2014). This path can relieve Nigeria with the problem of pollution already exacerbated by gas flaring in the Niger Delta region. Some researchers have reviewed integration of solar and biomass renewable energy sub system for biofuel generation as a sustainable method for biofuel production. In the methods described by Nzihou, Flamant, and Stanmore (Citation2012), solar-biomass gasification could be achieved by direct irradiation, through the wall of a reactor or the intermediate fluid carrying heat. In gasification, oxygen or water is needed to supply heat to the system while in pyrolysis the feedstock is processed in the absence or minimal presence of oxygen. The focus of gasification is on the production of syngas while pyrolysis is the production of liquid fuel and char. Therefore, the operating parameters and conditions such as heating rate, temperature, configuration, and design of the reactors are the main challenges to perform an efficient thermal process (Nzihou, Flamant, and Stanmore Citation2012). Apart from being environmentally friendly and sustainable, biomass-solar thermal conversion takes advantage of the mutual qualities of both biomass and solar energy (Chueh et al. Citation2010). Combining the two sources by supplying the high temperature needed for biomass thermal decomposition through the concentrated solar radiation produces high-energy solar fuel (Chueh et al. Citation2010). The process stores the concentrated solar energy as chemical energy in the form of biochar and bio-oils, thereby upgrading the biomass to bioenergy (Zeng et al. Citation2015). This modus is very appealing because the energy yields are of very high-energy density that is easily moveable (Zeng et al. Citation2015). The yields are of lower CO2 emission levels compared to the other conventional thermal processes. Biomass solar concentrated decomposition has the advantage of high temperature and fast heating rate resulting in fast/flash pyrolysis (Zeng et al. Citation2015) in a direct solar reactor. The rise of heating rates leads to a weak decrease in carbon content and increase in the hydrogen and oxygen amount in the biochar (Laurendeau Citation2009) that boosts reactivity due to the availability of more functional sites (Kurosaki et al. Citation2003). Zeng et al. (Citation2015) stated that this could be explained from the angle of increased pore volume and surface area. Similarly, increased heating rate reduces the tar vapours residence time in pores and shortens the condensation reaction resulting in the char reactivity increase (Zeng, Gauthier, and Flamant Citation2014; Phuphuakrat, Namioka, and Yoshikawa Citation2010).
The major thermal limitation of solar-biomass conversion is the high initial moisture content of some biomass feedstock (Antal and Grønli Citation2003). Additionally, solar insolation is intermittent, and its applications are characterised by sunshine and off-sunshine periods. It requires a rapid flow of the heat to maintain the desired temperature; however, the transient response of the system could be altered by the rapid fluctuation in solar irradiance, which characterises all solar heating processes. This can be remedied by charging the reactor with thermal storage materials like molten salt (Adinberg, Epstein, and Karni Citation2004).
5.2. Existing and emerging solar and biomass energy co-conversion technology to biofuel available for Nigeria
According to Joardder et al. (Citation2014), two heating approach can be adopted in solar-biomass conversion to fuel. These involve heating of the reactors with solar and other heat sources such as microwave or pump laser (Ugolin Citation2010; Limon-Vazquez and Chavez-Lara Citation2012) simultaneously or intermittently and heating or irradiating continuous with solar energy only. In other methods, reactor heating can be done continuously or intermittently by solar energy. The latter is currently much sort after although most research is still in the early stage and the quantity of biomass handled is still very small. Likewise, solar-assisted reactors methods can also be viewed based on the method of irradiations of the biomass inserted into the reactor (Kodama et al. Citation2010). Here, there are two methods: direct irradiation and indirect irradiation. In direct irradiation, the concentrated solar irradiance is focused on the biomass directly through glass or transparent glazing. The challenge of keeping the windows free from dust and other contaminants is the major issue in this design (Kodama et al. Citation2010). Additionally, in the indirect solar reactor, two stages are required before the solar energy is transferred to the biomass. A black body, which, at the same time, serves as the wall of the reactor, initially absorbs the solar irradiance then the energy is transferred to the biomass through conduction (Piatkowski, Wieckert, and Steinfeld Citation2009). This has a similar operation principle with indirect solar dryers. It has a temperature range limitation imposed by the thermo-kinetic properties of the black body and the reactants (Piatkowski, Wieckert, and Steinfeld Citation2009). This can be solved using intermediate integration of thermal storage materials with high specific latent heat properties such as phase change materials (Chueh et al. Citation2010)., liquid metals, and gases between the wall and the reactor. Some design architectures have been presented in the literature for solar-biomass co-conversion. Zeng, Gauthier, and Flamant (Citation2014) presented research in solar pyrolysis of beech wood with the study of the effects of parameters on the product distribution and gas product composition. The experimental loop is presented in . The reactor was set-up at the focal point of the vertical solar furnace. The integrated sensor tracks the sun location and the heliostats are automatically tweaked to focus on the sun such that its reflected beam is vertical to illuminate a down-facing parabolic mirror. A shutter controlled the reflected solar beam and the incident solar radiations with a moving blade. The maximum flux density and power are approximately 15000 kW/m2 and 1.5 kW, respectively. The reactor is made of a transparent pyrex balloon with a needle valve controlling the pressure. In evaluating the process parameters, temperature, pressure, heating rate, and argon flow rate on product yields were studied. The authors concluded that pyrolysis temperature and heating rates increased the total gas lower heating value while the influence of argon flow rate and pressure is minimal.
Figure 7. Schematics of solar pyrolysis experimental set up with a vertically placed furnace. Adapted from (Zeng, Gauthier, and Flamant Citation2014)
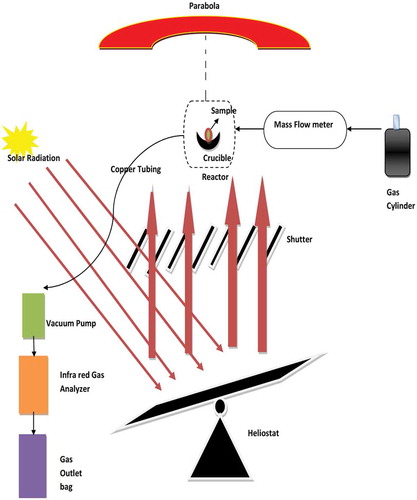
Zeng, Gauthier, and Flamant (Citation2014) and Zeng et al. (Citation2017)) investigated the influence of operating parameters on biochar properties and product distribution of beech wood using the same experimental setup. They found out that bio-char properties are more ordered with the increase of the temperature and the decrease of the heating rate. Zeng, Gauthier, and Flamant (Citation2014) provided a CFD simulation to determine the distribution of the temperature zones in the reactor at different flow rates of the sweep gas. Grassmann et al. (Citation2015) presented a solar experimental set with linear mirror II for pyrolysis of wheat straw. The linear mirror II was made up of an array of 4 × 2 mirrors consisting of four segments with an inclination of about 2° and follows the Zenith and the Azimuth positions of the Sun by the virtue of its rotating motors. The reactor was a horizontal rotary stainless cylindrical retort kiln powered by a motor revolving at 2 rpm. A deflector placed at the focal plane of the linear mirror deflects the concentrated irradiance towards the reactor; thus, the light entered the reactor from below and heats the biomass, while the gaseous yields are swept from the other sides. Morales et al. (Citation2014) presented a solar-biomass pyrolysis experimental setup for orange peel, shown in . The reactor was made of a borosilicate glass tube with a transmittance rate of 0.92. The irradiance was directly concentrated and focused on the orange peel, then the borosilicate glass, allows most of the irradiation to pass through its walls. The concentrator is a 1.3 m wide parabolic trough covered with a silver mirror coating. The concentrator and the reactor were mounted on a metallic hub with a single axis rotation to re-focus the system. The operational loop involved concentrating the solar irradiance over a small area of the reactor. The energy was then absorbed by the biomass. The nitrogen flow generated the inert atmosphere, which increased the temperature to the desired level. The gaseous yield was swept to a condensation train immersed in liquid nitrogen at −200°C.
Figure 8. Schematic experimental set for the solar-biomass pyrolysis system with a horizontally placed furnace. Adapted from.Morales et al. (Citation2014)
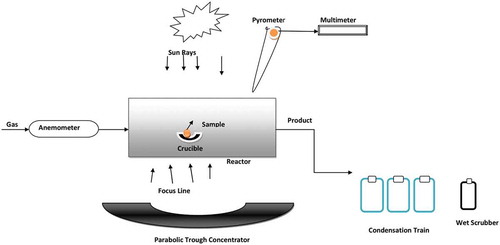
Li et al. (Citation2016) presented an experimental setup for solar-biomass pyrolysis of agricultural waste and forestry biomass residues. The setup was the same vertical furnace, such as the one presented by Zeng et al. (Citation2015). However, the only difference was the indication of the fluorine window, which was not incorporated in the earlier setup.
Beattie, Berjoan, and Coutures (Citation1983) presented a solar thermal reactor for the pyrolysis of biomass shown in . The components of the system include a stainless-steel dish, heliostat, 0.85 m focal length parabolic trough mirror measuring 2 m in diameter. The dish was covered by a 399 ml quartz dome. The mirror was located at the overhang section of a building while the heliostat was located on the south side of the building. The entire apparatus assembly was supported with a hub below the mirror, which tracked the focal line. The incident concentrated solar irradiation was directly absorbed by the biomass samples with a variation of its position below or above the plane of the furnace. The flux level ranged between 100 and 200 W/cm2.
Figure 9. Schematics of the experimental set by Adapted from .Beattie, Berjoan, and Coutures (Citation1983)
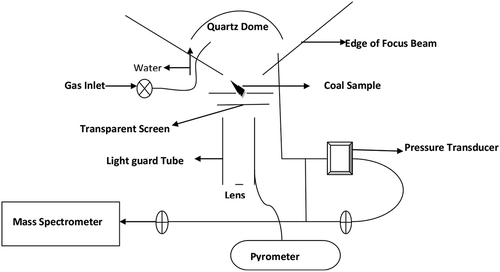
Adinberg, Epstein, and Karni (Citation2004) reported a parametric study of a large-scale solar-biomass pyrolysis/gasification reactor integrated with molten salt storage for the production of syngas. The system consisted of a spherical or a cylindrically shaped tank surrounded by vertical tubes filled with molten salts of lower thermal conductivity than the tubes, which served as the thermal storage material. The tubes absorbed the solar irradiation. The entire setup was placed in an insulated dome with openings to let penetration of the concentrated solar irradiation. Surrounding heliostats concentrated the solar irradiation through a parabolic concentrator and heated the molten salt. The heated molten salt was used to drive the biomass pyrolysis process.
Joardder et al. (Citation2014) presented a novel hybrid solar-assisted biomass pyrolysis reactor used to pyrolyse date seeds. The components of the pyrolysis system are stainless steel fixed bed reactor, external biomass heater with rice husk, a double 1.2 m diameter parabolic trough solar concentrator, liquid collector, and condenser. Ugolin (Citation2010) stated that the system could produce hot exhaust gas at a temperature up to 162°C. They concluded that by assisting the biomass pyrolysis with a solar heater, 32.4% of CO2 emission could be abetted from entering the atmosphere compared to the normal process.
5.3. Importance of the solar optical concentrators in solar and biomass co-conversion to biofuel
About 60% of the solar irradiance emitted by the sun reaches the earth directly or indirectly (World Energy Council, Citation2013). However, the annual daily flux density at a mean earth-sun distance and normal to the solar beam is 1.366 kW/m2 which, is further reduced by a factor of 4 due to losses in passing through the atmosphere (World Energy Council, Citation2013). These leave the earth with an average horizontal surface irradiance of about 170–300 W/m2. This value can produce a temperature of about 100°C or less indirect irradiation during the day using a flat-plate collector (World Energy Council, Citation2013). This range of temperature can be useful in many applications such as water heating or solar drying although it cannot drive the biomass thermal reduction process, which requires a temperature above 300°C (Das Citation2001; Kurosaki et al. Citation2003). Therefore, the concentration of the irradiation is necessary to reach the required temperature. The concentrating optics focuses on the irradiation into a small area of the reactor, reducing the area of the reactor by a factor of 500–1000 in some cases (World Energy Council, Citation2013). Different concentrators and their temperature ranges have been listed by Morales et al. (Citation2014). They are ranged from the low-temperature concentrator, such as a compound parabolic mirror to the central dish that attains a temperature above 1000°C. These high-powered concentrators are also deployed in industrial heat production. High-powered linear mirrors equipped with or without heat exchangers have been deployed with the thermal power of up to 9 kW (Grassmann et al. Citation2015). These concentrators can be beamed down or mounted on a building or a tower and line with the reactor (Kuravi et al. Citation2013). The installation schematics of some of the concentrators are shown in . According to the U.S Department of Energy, solar concentrators cost 150 USD − 250 $/m2 which is about half of the total-installed total cost of concentrating solar power (CSP) plant (Sun shot Citation2012). This price can be reduced if the entire set up is properly optimised. In Nigeria, labour is cheap; therefore, the cost of installation coupled with available and free biomass that will serve as a raw material will reduce the cost of the project in solar-biomass bio-fuel production.
Figure 10. Schematic diagrams of solar concentrator. Adapted From (Morales et al. Citation2014; Kuravi et al. Citation2013)
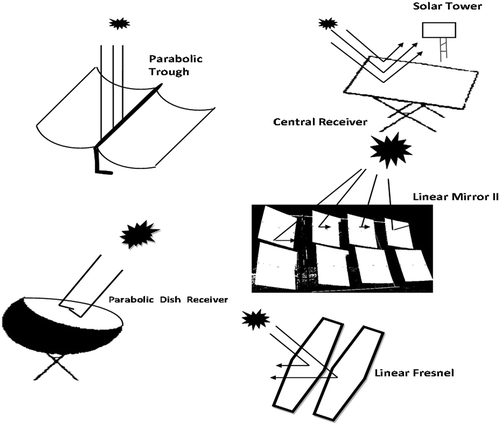
6. Nigeria renewable energy policies and barriers
Many countries of the world that integrated RE into the energy mix did so with a bold action plan, strategic policies and implementation of robust mechanisms (Ölz Citation2011). Governments have been a major driver of energy projects all over the world. From 2001 to 2015, the Nigerian government formulated various policies and programs towards achieving energy sufficiency in various sectors of the economy (Emodi and Ebele Citation2016). These policies are mainly hinged on power sector reforms. Most of these strategic policies remain at the draft stage with the continuation of a gap between these frameworks and implementation. These policies were drafted with the help of the National Planning Commission, the Federal Ministry of Power, the Nigerian Electricity Regulatory Commission, NEC, Federal Ministry of Water Resources (FMWR), NNPC and donor agencies such as the World Bank, EU, African Development Bank (AFDB), US Agency for International Aids, the Japan International Cooperation Agency (JICA). The policies include the National electric power policy (NEPP) 2001, National Power Sector Reform Act (NPSRA) 2005, National Energy Policy (NEP) 2003, 2006 and 2013 (Emodi and Ebele Citation2016).
The main objective of NEPP and NPSRA was to increase competition in the power sector, unbundling and privatisation of the government power company. However, NEP was concerned with ensuring energy security through diversification of energy carriers and suppliers in the country (Emodi and Ebele Citation2016; Energy Commission of Nigeria (ECN) Citation2013). It was NEP that mainly recognised the importance of RE in achieving sustainable energy supply and development throughout Nigeria. However, other policies were also developed for achieving RE integration into the country’s energy mix. These policies were driven by the need to increase the use of the electricity generation in rural areas, reduce dependence on imported fuel, reduce environmental pollution, mitigate climate change and create jobs by establishing viable companies that will stimulate growth in the economy (Emodi and Ebele Citation2016). The RE policies included the Renewable Energy Master Plan (REMP) 2006 and 2012, Renewable Electricity Action Program 2006, Renewable Electricity Policy Guidelines 2006, Nigerian Biofuel Policy and Incentives (NBPI) 2007, National Renewable Energy and Energy Efficiency Policy (NREEEP) 2015, Rural Electrification Strategy and Implementation Plan 2014 and the Integrated Infrastructure Master plan (IIMP) (Emodi and Ebele Citation2016; Nigeria Energy Future Citation2015). Also, JICA joint with FMWR created Hydropower Master Plans 1993, 1995, 2013 and, the Solar Energy Master Plan 2007.
The present strategic document is the Intended Nationally Determined Contributions termed Nigeria’s low carbon development strategy (Nigeria Energy Future Citation2015). These policies present government action plan to expand energy generation through RE integration, attract major players in the industry in partnership with the government, provide strategies for monitoring, evaluation and risk assessment and also provide incentives to interested partners (Energy Commission of Nigeria (ECN) Citation2005; CitationFederal Ministry of Power and Steel(FMPS); Nigerian National Petroleum Corporation (NNPC) Citation2007; Energy Commission of Nigeria (ECN) Citation2003; American Public Power Association Public Power Utilities (APPAPU): Net Metering Programs Citation2014). The target of REMP is 10% overall RE contribution to Nigerian energy mix by 2025 through incremental renewable electricity, added to the national grid, starting from 16,000 MW in 2015 to 192000 MW by 2030. The Multi-Year Tariff Order 1 and 2, 2008 and 2012 drawn by NEC which is made to be implemented in phases, giving incentives to power producers. The first phase, which ran from 2008 to 2012, was targeting consumers, while the final phase was focusing on empowering power producers to run the power infrastructures. Also, it mentioned how energy tariffs can be adjusted based on inflation, power generation capacity, dollar to the naira exchange rate, etc. NREEEP is a power roadmap designed to increase RE target to 20%, whereas IIMP targets were 30% by 2023 (Nigeria Energy Future Citation2015). NREEEP committed the government to establish Renewable Portfolio Standards (RPS), give Power Production Tax Credit (PTC), grants and loans, licencing incentives and feed-in tariff (Nigeria Energy Future Citation2015). INDC suggested that PV cells alone will contribute 13,000 MW to the power grid by 2030. NBPI proposed an increase in the domestic fuel supply by utilising biomass and other agricultural products. Despite all these policies, progress in RE implementation in Nigeria is very slow. Emodi and Ebele (Citation2016) have identified the major barrier to RE development in Nigeria to include, none identification of appropriate technology for production of particular energy carrier, low capital investment, inconsistent policies and strategies, inadequate fiscal incentives to investors, politics, lack of environmental support plan, acceptability and lack of continuity when the government is changed.
Very few African countries have successfully integrated RE into their energy mix, though some forms of policies and strategies have been developed by others. Congo DRC, Ghana, South Africa, and Rwanda are investing in cleaner electricity through solar power projects that will provide heat and light to many homes (Adam Citation2018). Lessons can be learned from Morocco which with the help of the AFDB, German investment bank (KfW), the European Investment Bank (EIB) and the World Bank is exploiting it over 3000 h of sunshine per year to build a thermo-solar farm in the city of Ouarzazate near the Sahara Desert (Adam Citation2018). The first phase which was completed in 2016 was able to power over 650,000 homes when switched on in 2016 with a targeted 580 MW in 2020 that will account for about 42% of its energy need (Adam Citation2018). Other lessons can be learned from European countries that have successfully integrated RE into their energy mix. For instance, since 1992, energy consumers in Denmark and Sweden have been charged CO2 tax, which is invested back into RE electricity (Leflaive Citation2012; KPMG Citation2013). This strategy increased the cost of energy from fossil fuel, while the energy from RE deceased. Countries like Germany, Greece, Spain, and Ireland stimulated their RE generation by giving one form of tax incentives or the other for both RE consumers and generators (United Nation Development Programme (UNDP) Citation2010; Ruijs and Vollebergh Citation2013). Quick depreciation on investment was given by the Netherlander RE investors (United Nation Development Programme (UNDP) Citation2010; Ragwitz et al. Citation2012). Legislations such as green certificates, feed-in-tariff, tendering provision, and net-metering programs have been employed in countries like Romania, Spain, Denmark, Belgium, Poland and Germany (United Nation Development Programme (UNDP) Citation2010; Ragwitz et al. Citation2012). Countries like Germany and Australia have a competitive grant scheme on research and developments in RE technologies (Hogg and O’Regan Citation2010; Choung Citation2010; Sharma, Vishnu, and Dongke, 2015).
These examples can serve as a basis for Nigeria to show commitment towards achieving RE sustainability. The developed RE policies must provide a cost recovery framework to investors through loans, grants and subsidies. Presently, Nigeria lacks an institutional framework to monitor and implement RE policies and strategies. The myriads of the institution and several bodies set up by successive governments resulted in an overlapping of the mandate that created complexity in the regulatory process (Ben-Iwo, Manovic, and Longhurst Citation2016). Technocrats and legislators need to be involved to harmonise and draft out appropriate legislation that will favour RE application. Policies developed, should work interactively to complement each other as the current policies have conflicting objectives. The recent policies did not address environmental and economic sustainability along with the value chain. Some of the primary feedstocks are in competition with land for food production and food need for the population. This is important because energy has to be supplied in a way that makes it available at a realistic cost without triggering environmental and economic crises. Investment in research and development coupled with financial incentives to investors and end-users are key components in realising a sustainable RE system. However, the first step is to identify RE potential in Nigeria and the appropriate technology required in converting them to useful energy carriers.
7. Challenges facing the implementation co-conversion technology in Nigeria
Presently, biofuel production in Africa has not started at a commercial scale because the very few bio-ethanol plants are using crude imported ethanol (Amigun, Musango, and Stafford Citation2011). Therefore, the conceptualisation of any biofuel plant will start from scratch. The needed products, the method of decomposition and feedstock available should be identified. This will give a clear picture of the choice of reactor type and, the liquid yield as it has been mentioned in the previous section. For example, in fast pyrolysis, fixed bed and the associated systems are unlikely to produce high liquid yield but more of phase-separated products (Bridgwater, Citation2011). While gasification has existed for many years, however, it has been very few successfully operating units available, while combustion is a well-known technology, which its development has been focused on resolving environmental issues (Koppejan and Van Loo Citation2009). Therefore, the moderate temperature needed and a wide range of product derived, pyrolysis presents the best route for solar-biomass decomposition. It can be operational for the production of a liquid fuel that can be directly applied as a transitional pre-treatment phase to transform solid biomass into a higher energy density transportable liquid for future processing for different kinds of energy and chemicals. This process is very versatile with improved efficiency and can be operational in a short term (Bridgwater, 2012).
A commercial pyrolysis system requires three design stages to take delivery of the desired product. The first stage is a system to receive the feedstock, store, handles and carry out some pre-treatments before further processing (Maggi and Elliott Citation1997). The second stage is the system to convert the pre-treated feedstock into crude bio-oil and the last structure is to clean and refine the bio-oil into a useful product. Due to incompatibility with conventional fuel quality upgrading facilities can be included, which the method can be chemical, physical, or catalytic (Maggi and Elliott Citation1997). The three stages require reactor, transmission equipment, catalytic chamber, a cooling tube, heavy tank, oil-water separator, condenser, light oil tank, safety system, vacuum system, dedusting system, draft fan, and chimney (Bridgewater 2012). Among these components, the reactor and the cooling systems form the heart of the pyrolysis plant. Therefore, an appropriate solar system reactor(s) will be required for an efficient application. This technology is not available locally in Nigeria and can be sourced elsewhere for now while local content capacity is built.
Because of the higher solar intensity prevalent in the northern region of Nigeria and the large agricultural waste generated in that area, it presents a good site for solar-biomass pyrolysis. This area is disadvantaged in Nigeria in terms of fuel and electricity supply because it is far from where oil is produced, refined and electricity thermal plants are located. Even the imported petroleum products land at the southern ports far from this area and require long haulage to get in. Nigerian government pays parts of this cost to level the petrol and electricity price across the country. Hence, solar-biomass pyrolysis will be sustainable in this largely agrarian area. The biomass storage and pre-treatment plants can be established close to the major feedstock production area for onward transportation to the solar – biomass oil mill and bio-refining facilities in the north. Small to medium scale biofuel production could be established to produce biodiesel for automobiles, household cooking and to power electricity thermal plants. This will also create jobs for people across the country and reduce poverty.
Prior to the establishment of any solar-biomass biofuel production system, industries and research institutions that have developed capacity in any form of pyrolysis should be consulted. They should identify the appropriate management methods for the heating process in terms of total or partial solar-assisted heating vis-a-vis the environmental factors. Some of these companies include but not limited to BTG Bio-liquid – Technology Group Netherland, Agritherm Canada, RTI, Canada, Biomass Engineering Ltd, UK, Polyax – Ukraine LLC, Ukraine, Metso/UPM, Finland, BioEcon, Netherlands, etc.
8. Recommendations
It is a unanimous belief that the sustainable production of distributable and transportation fuels from biomass feedstock resources in Nigeria requires different; policy, feedstock and new technological approach (Jaroenkhasemmeesuk and Tippayawong Citation2015). The changing of Nigeria to a biofuel-based economy, where biomass replaces crude oil, will materialise if all sectors of the economy identify the technological gaps and policy formulators that puts sustainability issues at the forefront of energy generation and utilisation. Identifying the pressing energy carrier mostly needed to power the economy will determine the biomass conversion technology mostly required by Nigeria. The fact that solar-pyrolysis technology can process all the biomolecules and produce almost all energy carriers in the supply chain offers a good route for consideration in Nigeria. The management option can be full solar heating or partial solar heating of the reactor. Additionally, defining the biomass decomposition routes as solar pyrolysis will coalesce technology innovation and capacity built in that direction. Policy and management approach should put environmental issues, energy decentralisation and the availability of resources is paramount. The quantity and type of feedstock, solar intensity, the location of available refining facility which can serve as the co-processing facility as opposed to standing alone bio-refinery, technological transfer and any available local capacity should be a factor for choosing a location to invest this technology. For bio-fuel acceptability and increased sales, Nigeria as a matter of policy should enforce the global 10% blending of biofuel into transportation fuel with increased licencing of IPP’s with bio-based thermal plants and given them tax breaks until they breakeven.
9. Conclusion
This study gave an overview of the energy deficit in Nigeria and the challenges facing energy production and distribution in Nigeria until date. The study identified the need for alternative energy and suggested a technological route to sustainable biofuel production. The article also highlighted different biomass and solar potential available in the country. Nigerian energy infrastructure is tilting towards the southern region due to available crude oil in that region, thereby leaving the largely agrarian north with potential energy gap and the huge cost is incurred in energy transportation and distribution towards this region. Therefore, there is a need for energy decentralisation between the north and the south, and since the north does not have the crude carbon, RE offers the best possible alternative. To achieve these. Nigeria should show a serious commitment to achieving RE sustainability. The developed Government policies should be re-tuned to provide a cost recovery framework to investors through loans, grants, and subsidies. Technocrats and legislators should be involved to draft out appropriate legislation that will favour RE generation and utilisation. Policies should be developed to work interactively to complement each other as the current policies have conflicting objectives. Abundance solar energy and humongous agricultural residues available in various regions should be exploited. Considering these two resources together (i.e. solar energy and biomass), solar-biomass pyrolysis technology offers a good choice for the thermal conversion of the biomass. Moreover, it is presented as an environmentally and eco-friendly process. The review of design architecture, configurations, and research results showed considerable growth and increase of research activities done in the last few years with more advances in the types of reactor configurations and solar concentrators studied by researchers in different academic institutions.
Nigeria could exploit the newly conceived rail lines from the north to the south to move biomass for processing. The initial technological need could be sourced anywhere in the world and local capacity built over time. The blending of biofuel into the country’s energy mix should be gradual and sustained.
Acknowledgments
The authors appreciate the various agencies whose data sources are used in the preparation of this research work, especially, the Energy Commission of Nigeria (ECN), Nigerian Bureau of Statistics (NBS), World Bank Group, International Energy Agency (IEA), World Energy Council, African Energy Commission (AFREC), Thisdaylive and all the authors whose work is cited in this research work.
Disclosure statement
This research work did not receive funding from any source and there is no conflict of interest among the authors.
Additional information
Notes on contributors
M. C. Ndukwu
Dr M.C Ndukwu is an associate professor and researcher whose interest lies at the intersection of technologies and process parameters for developing efficient crop processing equipment and Agro-biomass valorization. Currently he conducts research on energy and exergy analysis , solar energy application in crop processing and valorization of Agro-Waste for energy generation.
D. I. Onwude
Dr Onwude is a lecturer and researcher with interest in model developments and renewable energy.
L. Bennamoun
Dr lyes Bennamoun is a researcher in the department of mechanical engineering with special interest in renewable energy.
F. I. Abam
Dr Abam is an associate professor of mechanical engineering with special interest in exergy, energy and environment.
M. Simo-Tagne
Dr Simo-Tagne is a researcher with LERMaB France with special interest in modeling of renewable energy process.
I. T. Horsfall
Mr HorSfall is a researcher with emphasis on pyrolysis.
T.A. Briggs
Dr Briggs is a Senior lecturer in mechanical engineering also with interest in energy conversion.
References
- Abam., F., B. I. Nwankwojike, O. S. .Ohunakin, and S. A. Ojomu. 2014. “Energy Resource Structure and On-going Sustainable Development Policy in Nigeria: A Review.” International Journal of Energy and Environmental Engineering 2014 5 (102): 1–16.
- Abila, N. 2010. Biofuels Adoption in Nigeria: A Preliminary Review of Feedstock and Fuel Production Potential, 1–11. Vaasa, Finland: Dept. of Industial Management, University of Vaasa.
- Adam, J. “Morocco Is Building a Giant Thermo-solar Farm in the Sahara Desert”. World economic forum. Accessed 5th December 2018. www.weforum.org/agenda/2018/05/morocco-is-building-a-solar-farm-as-big-as-paris-in-the-sahara-desert/.
- Adinberg, R., M. Epstein, and J. Karni. 2004. “Solar Gasification of Biomass: A Molten Salt Pyrolysis Study.” Journal of Solar Energy Engineering 126 (3): 850–857. doi:10.1115/1.1753577.
- Akinbami, J. F. K. 2001. “Renewable Energy Resources and Technologies in Nigeria: Present Situation, Future Prospects and Policy Framework.” Mitigation and Adaptation Strategies for Global Change 6: 155–181. Kluwer Academic Publishers, Netherlands. doi:10.1023/A:1011387516838.
- American Public Power Association Public Power Utilities (APPAPU): Net Metering Programs. 2014. Accessed November 30, 2018. www.energy.gov.ng.
- Amigun, B. J., K. Musango, and W. Stafford. 2011. “Biofuels and Sustainability in Africa.” Renewable and Sustainable Energy Reviews 15 (2): 1360–1372. doi:10.1016/j.rser.2010.10.015.
- Antal, M. J., and M. Grønli. 2003. “The Art, Science, and Technology of Charcoal Production.” Industrial & Engineering Chemistry Research 42 (8): 1619–1640. doi:10.1021/ie0207919.
- Azil, I. 2007. “Biofuels Demand: Opportunities for Rural Development in Africa (Nigerian Casestudy).” Being a Paper Presented at the 2nd European Forum Ion Sustainable Development, June 18 – 21, 2007, Berlin Germany.
- Bala, E. J. 2012. “Renewable Energy Policy and Masterplan for Nigeria.” Presentation Made at a One Day Sensitization Workshop on National Energy Masterplan, Rockview (Classic) Hotel, Wuse II, Abuja, 5thDecember 2012, Abuja.
- Bala, E. J. 2013. “Achieving Renewable Energy Potential in Africa.” Presentation Made at Joint WEC, AUC and APUA Workshop “Solutions for Sustainable Energy in Africa: Energy Efficiency, Renewable Energy and Interconnections, 17-18thJune, 2013, Addis Ababa, Ethiopia.
- Bala, E. J., and G. Y. Pam. 2012. “Energy Sources and Sustainable Development of the North.” A Paper Presented at Conference on “The North and Strategies for Sustainable Development,” Organized by Arewa House, Kaduna, 5th-6thDecember 2012.
- Bala E. J. 2011. “Technology frontier in renewable energy and energy efficiency„ .Presentation made at the Workshop on Strengthening the Institutional Capacity of Research Centers in Niger Delta and Sahel Regions to cope with Climate Change using Renewable Energy and Efficiency, Benin City, Nigeria, 17 –18th October, 2011
- Barber, M., and M. Warnken. 2008. Biomass Technology Review: Processing for Energy and Materials. Victoria: Crucible carbon for sustainability. www.sustainability.vic.gov.au/resources/documents/biomass_Technology_Review.
- Basu, P. 2010. Biomass Gasification and Pyrolysis Practical Design. 1st ed. Kidlington, Oxford: Academicpress.
- Beattie, W. H., R. Berjoan, and J.-P. Coutures. 1983. “High-Temperature Solar Pyrolysis of Coal.” Solar Energy 31 (2): 137–E43. doi:10.1016/0038-092X(83)90074-9.
- Ben-Iwo, J., V. Manovic, and P. Longhurst. 2016. “Biomass Resources and Biofuels Potential for the Production of Transportation Fuels in Nigeria.” Renewable and Sustainable Energy Reviews 63: 172–192. doi:10.1016/j.rser.2016.05.050.
- Bridgwater A.V. 2011. “Review of fast pyrolysis of biomass and product upgrading„. Biomass Bioenergy 38: 68–94. http://dx.doi.org/10.1016/j.biombioe.2011.01.048.
- Brownsort, P. A. 2009. “Biomass Pyrolysis Processes: Review Of Scope, Control And Variability.” UKBRC Working Paper 5: 1–39.
- Casa M.D., C.S. Canga, M.A. Díez, R. Alvarez and C. Barriocanal. 2005. “Low-temperature pyrolysis of coals with different coking pressure characteristics.” J. Anal. Appl. Pyrolysis 74: 96–103.
- Chanchangi, Y. N., A. Ghosh, S. Sundaram, and T. K. Mallick. 2020. “Dust and PV Performance in Nigeria: A Review.” Renewable and Sustainable Energy Reviews 121: 109704. doi:10.1016/j.rser.2020.109704.
- Choung, Y. 2010. “Quick Look: Renewable Energy Development in South Korea.” Renewable Energy World. Accessed November 30, 2018. www.renewableenergyworld.com
- Chueh, W. C., C. Falter, M. Abbott, D. Scipio, P. Furler, S. M. Haile, and A. Steinfeld. 2010. “Solar-Driven Thermochemical Dissociation Of CO2 And H2O Using Nonstoichiometric Ceria.” Science 330: 1797–1801. doi:10.1126/science.1197834.
- Chukwujindu, N. S. 2017. “A Comprehensive Review of Empirical Models for Estimating Global Solar Radiation in Africa.” Renewable and Sustainable Energy Reviews 78: 955–995. doi:10.1016/j.rser.2017.04.101.
- Crossette, B. 2011. Estado De La Población Mundial (2011). 1st ed. New York: Fondo De Poblaciónde Las Naciones Unidas.
- Das, T. K. 2001. “Evolution Characteristics Of Gases During Pyrolysis Of Maceral Concentrates Of Russian Coking Coals.” Fuel 80 (4): 489–500. doi:10.1016/S0016-2361(00)00126-5.
- Deal, C., C. E. Brewer, R. C. Brown, M. A. E. Okure, and A. Amoding. 2011. “Comparison of Kiln-Derived and Gasifier-Derived Biochars as Soil Amendments in the Humid Tropics.” Biomass & Bioenergy 37: 161–168.
- Demirba S A. 2008. “Biofuels sources, biofuel policy, biofuel economy, and global biofuel projections.” Energy Convers Manag 49:2106–16. http://dx.doi.org/10.1016/j.enconman.2008.02.020
- Dioha, M. O., and A. Kumar. 2020. “Exploring Sustainable Energy Transitions in sub-Saharan Africa Residential Sector: The Case of Nigeria.” Renewable and Sustainable Energy Reviews 117: 109510. doi:10.1016/j.rser.2019.109510.
- Elbehri, A., A. Segerstedt, and P. Liu. 2013. “Biofuels and the Sustainability Challenge: A Global Assessment of Sustainability Issues, Trends, and Policies for Biofuels and Related Feedstocks.” Rome: Food and Agriculture Organization of the United Nations, (FAO).
- Elijah, I. O. 2010. “Emerging Bio-ethanol Projects in Nigeria: Their Opportunities and Challenges.” Energy Policy Reviews 38 (11): 7161–7168. doi:10.1116/j.enpol.2010.07.038.
- Emodi, N. V., and E. Ebele. 2016. “Policies Enhancing Renewable Energy Development and Implications for Nigeria.” Renewable and Sustainable Energy 4: 7–16. doi:10.12691/rse-4-1-2.
- Energy Commission of Nigeria (ECN). 2003. National Energy Policy. Abuja: Federal Republic of Nigeria.
- Energy Commission of Nigeria (ECN). 2005. “And United Nations Development Programme (UNDP) Renewable Energy Master Plan (REMP) (2005).” Accessed November 30, 2018. www.spidersolutionsnigeria.com.
- Energy Commission of Nigeria (ECN). 2013. National Energy Policy, Draft Revised ed. (NEP)The Federal Republic of Nigeria. Accessed November 30, 2018 www.energy.gov.ng
- Energy Commission of Nigeria (ECN) and the United Nations Development Programme (UNDP). 2012. “Renewable Energy Master Plan (REMP).” Accessed November 30, 2018. www.energy.gov.ng.
- Energy Commission of Nigeria(ECN). 1998. World Solar Programme, 1996 – 2005. Projects of the Government of Nigeria: Project Documents, ECN Abuja.
- Energypeadia. “Nigeria Energy Situation.” Accessed 13th March 2018. https://energypedia.info/wiki/Nigeria_Energy_Situation.
- Fapetu, O. P. 2000. “Management of Energy from Biomass.” Nigerian Journal of Engineering Management 1: 14–19.
- Federal Ministry of Power and Steel(FMPS). “Renewable Electricity Action Program (REAP).” Federal Republic of Nigeria. Accessed November 30, 2018. www.iceednigeria.org/
- Fitz, H. C., E. B. De Bellevue, R. Costanza, R. Boumans, T. W. Maxwell, L. Wainger, and F. H. Sklar. 1996. “Development Of A General Ecosystem Model For Arrange Of Scales And Ecosystems.” Ecological Modelling 88 (1–3): 263–295. doi:10.1016/0304-3800(95)00112-3.
- Galadima, A., Z. N. Garba, B. M. Ibrahim, M. N. Almustapha, L. Leke, and I. K. Adam. 2011. “Biofuels Production in Nigeria: The Policy and Public Opinions.” Journal of Sustainable Development 4: 22–31. doi:10.5539/jsd.v4n4p22.
- Giwaa, A., A. Alabia, A. Yusufa, and T. Olukan. 2017. “A Comprehensive Review on Biomass and Solar Energy for Sustainable Energy Generation in Nigeria.” Renewable and Sustainable Energy Reviews 69: 620–641. doi:10.1016/j.rser.2016.11.160.
- Grassmann, H., M. Boaro, M. Citossi, M. Cobal, E. Ersettis, E. Kapllaj, and A. Pizzariello. 2015. “Solar Biomass Pyrolysis with the Linear Mirror I.” I. Smart Grid and Renewable Energy 6: 179–186. doi:10.4236/sgre.2015.67016.
- Heidari, A., I. R. Stah, H. Younesi, Rashidia, N. Troeger, and A. A. Ghoreyshi. 2014. “Effect of Process Conditions on Product Yield and Composition of Fast Pyrolysis of Eucalyptus Grandis in Fluidized Bed Reactor.” Journal of Industrial and Engineering Chemistry 20 (4): 2594–2602. doi:10.1016/j.jiec.2013.10.046.
- Hogg, K., and R. O’Regan. 2010. Renewable Energy Support Mechanisms: An Overview. London, United Kingdom: Pricewaterhouse Coopers LLP, Globe Law, and Business.
- Hopkins, M. W., C. Dejenga, and M. J. Antal. 1985. “The Flash Pyrolysis Of Cellulosic Materials Using Concentrated Visible Light.” Solar Energy 32: 547–551. doi:10.1016/0038-092X(84)90269-X.
- Ibitoye, F. I., and A. Adenikinju. 2007. “Future Demand for Electricity in Nigeria”.” Applied Energy 84 (5): 492–504. doi:10.1016/j.apenergy.2006.09.011.
- Ikuponisi, F. S. 2004. Status of Renewable Energy in Nigeria. One Sky Energetic Solutions Conference, November 21-27, 1-48. Nigeria: Background Paper.
- In:, B. R., J. Lehmann, and S. Joseph. 2009. Biochar Production Technology. London: Biochar for Environmental Management Earthscan.
- International Energy Agency (IEA). 2018. “Sustainable Energy for All, 2013, Global Tracking Framework.” Accessed 6 June 2018. http://www.iea.org/statistics/topics/Electricity/
- Jakayinfa, S .O, J. I. Orisaleye, and R. Pecenka. 2020. “An Assessment of Potential Resources for Biomass Energy in Nigeria.” Resources 9 (92): 1–41.
- Jakayinfa S.O, and V. Scholz. 2007. “Assessment of Availability and Cost of Energetically Usable Crop Residues in Nigeria”. In Conference on International Agricultural Research for Development, University of Kassel-Witzenhausen and University of Gottingen.
- Jaroenkhasemmeesuk, C., and N. Tippayawong. 2015. “Technical and Economic Analysis of A Biomass Pyrolysis Plant.” Energy Procedia 79: 950–955. doi:10.1016/j.egypro.2015.11.592.
- Joardder, U. H., P. K. Halder, A. Rahim, and N. Paul. 2014. “Solar Assisted Fast Pyrolysis: A Novel Approach of Renewable Energy Production.” Journal of Engineering Article ID 252848. doi:10.1155/2014/252848.
- Kodama, T., N. Gokon, S. Enomoto, S. Itoh, and T. Hatamachi. 2010. “Coal Coke Gasification in a Windowed Solar Chemical Reactor for Beam-Down Optics.” Journal of Solar Energy Engineering 132 (4): 041004. doi:10.1115/1.4002081.
- Koppejan, J., and S. Van Loo. 2009. “Biomass Combustion: An Overview.” In Thermal Biomass Conversion, edited by A. V. Bridgwater, H. Hofbauer, and S. van Loo. Liberty HouseGreenham Business ParkThatcham, Berks: CPL Press.
- KPMG. 2013. Taxes and Incentives for Renewable Energy. Accessed November 30, 2018. www.kpmg.com/Global
- Kuravi, S., J. Trahan, D. Y. Goswami, M. Rahman, and E. Stefanakos. 2013. “Thermal Energy Storage Technologies and Systems for Concentrating Solar Power Plants.” Progress in Energy and Combustion Science 39 (4): 285–319. doi:10.1016/j.pecs.2013.02.001.
- Kurosaki, F., K. Ishimaru, T. Hata, P. Bronsveld, E. Kobayashi, and Y. Imamura. 2003. “Microstructure of Wood Charcoal Prepared by Flash Heating.” Carbon 41 (15): 3057–3062. doi:10.1016/S0008-6223(03)00434-2.
- Ladan, M. T. 2009. “Policy, Legislative and Regulatory Challenges in Promoting Efficient and Renewable Energy for Sustainable Development and Climate Change Mitigation in Nigeria.” A Paper Presented: The 3rd Symposium and 2nd Scientific Conference of Assellau. Nairobi, Kenya: University of Nairobi, faculty of law/caselap, 23th – 25th march, 2009.
- Laurendeau, N. M. 2009. “Heterogeneous Kinetics of Coal Char Gasification and Combustion.” Progress of Energy Combustion Science 35: 121–140.
- Leflaive, X. 2012. “Water Outlook to 2050: The OECD Calls for Early and Strategic Action.” In Discussion Paper 1219. Canberra Australia: Global Water Forum.
- Li, R., K. Zeng, J. _. E. Soria, G. _. A. Mazza, D. Gauthier, R. Rodriguez, and G. Flamant. 2016. “Product Distribution from Solar Pyrolysis of Agricultural and Forestry Biomass Residues.” Renewable Energy 89: 27e- E35. doi:10.1016/j.renene.2015.11.071.
- Limon-Vazquez, N., and F. Chavez-Lara. 2012. “Collector And Systems For Generating Steam From The Use Of Solar Energy”, Mexican Patent MX 2010012078.
- Maggi, R., and D. Elliott. 1997. “Upgrading Overview.” In Developments in Thermochemical Biomass Conversion, edited by A. V. Bridgwater and D. G. G. Boocock, 575e88, Blackie Academic and Professional.
- Meier, D., and O. Faix. 1999. “State of the Art of Applied Fast Pyrolysis of Lignocellulosic materials — A Review.” Bioresource Technology 68 (1): 71–77. doi:10.1016/S0960-8524(98)00086-8.
- Morales, S., R. Miranda, D. Bustos, T. Cazares, and H. Tran. 2014. “Solar Biomass Pyrolysis for the Production of Bio-Fuels and Chemical Commodities.” Journal of Analytical and Applied Pyrolysis 109: 65e–78. doi:10.1016/j.jaap.2014.07.012.
- Müller, S., B. Adam and S. Ölz. 2011. Renewable Energy Policy Considerations for Deploying Renewable. International energy agency. http://www.iea.org/w/bookshop/add.aspx?id=414
- Ndukwu, M. C., A. A. Akani, and K. J. Simonyan. 2015. “Nigeria’s Grain Resource Structure and Government Sustainable Policy: A Review.” Agricultural Engineering International: CIGR Journal 17: 441–457.
- Ndukwu, M. C., and L. Bennamoun. 2017. “Potential of Integrating Na2SO4 · 10H2O Pellets in Solar Drying System.” Dry Technology. doi:10.1080/07373937.2017.1366506.
- Ndukwu, M. C., L. Bennamoun, and F. I. Abam. 2018. “Experience of Solar Drying in Africa: Presentation of Designs, Operations, and Models.” Food Engineering Reviews 10 (4): 211–244. doi:10.1007/s12393-018-9181-2.
- Ndukwu, M. C. L., Bennamoun, F. I. Abam, A. B. Eke, D. Ukoha, and L. Bennamoun. 2017. “Energy and Exergy Analysis of a Solar Dryer Integrated with Sodium Sulfate Decahydrate and Sodium Chloride as Thermal Storage Medium.” Renewable Energy 113: 1182–1192. doi:10.1016/j.renene.2017.06.097.
- Ngumah, C. C., J. N. Ogbulie, J. C. Orji, and E. S. Amadi. 2013. “Biogas Potential of Organic Waste in Nigeria.” Journal of Urban and Environmental Engineering 7 (1): 110. doi:10.4090/juee.2013.v7n1.110116.
- Nigeria Energy Future. 2015. “Nigeria’s Renewable Energy Policy (NREEEP). (Nesgroup.org).” [retrieved 30th November 2018]. Nigeria Energy Profile 2018). Accessed 6 June 2018. www.indexmundi.com/nigeria/
- Nigerian Bureau of Statistics (NBS). 2018. “Nigerian Gross Domestic Product Report (Q3 2017).” Accessed 6 June 2018. www.nigerianstat.gov.ng
- Nigerian National Petroleum Corporation (NNPC). 2007. Draft Nigerian Bio-Fuel Policy and Incentives. Abuja: Nigerian National Petroleum Corporation.
- Njokuji, O. J. 2019. “Renewable Energy as an Alternative to Fossil Fuel Use: A Legal Framework for Advancing Low Carbon Energy Transition in Nigeria.” Electronic Thesis and Dissertation Repository, 6651. https://ir.lib.uwo.ca/etd/6651
- Nwofe, P. A. 2014. “Utilization of Solar and Biomass Energy- a Panacea to Energy Sustainability in a Developing Economy.” International Journal of Energy and Environmental Research 2: 10–19.
- Nzihou, A., G. Flamant, and B. Stanmore. 2012. “Synthetic Fuels from Biomass Using Concentrated Solar Energy - A Review.” Energy 42: 121–131. doi:10.1016/j.energy.2012.03.077.
- Ogbonnaya, C., C. Abeykoonb, U. M. Damoa, and A. Turan. 2020. “The Current and Emerging Renewable Energy Technologies for Power Generation in Nigeria: A Review.” Thermal Science and Engineering Progress 13 (2019): 100390. doi:10.1016/j.tsep.2019.100390.
- Ogunwusi, A. A., and M. A. Joalaoso. 2012. “Forest Products Industry in Nigeria.” Africa Review 6 (4): 199–205.
- Ogwueleka, T. C. 2009. “Municipal Solid Waste Characteristics and Management in Nigeria.” Iran Journal of Environ Mental Health Science Engineering 6: 173–180. doi:10.1016/j.wasman.2007.09.039.
- Ohimain, E. I. 2010. “Emerging Bio-ethanol Projects in Nigeria: Their Opportunities and Challenges.” Energy Policy 38 (11): 7161–7168. doi:10.1016/j.enpol.2010.07.038.
- Ohunakin, O. S. 2010. “Energy Utilization and Renewable Energy Sources in Nigeria.” Journal of Engineering and Applied Sciences 5 (2): 171–177. doi:10.3923/jeasci.2010.171.177.
- Ohunakin, O. S., M. S. Adaramola, O. M. Oyewola, and R. O. Fagbenle. 2014. “Solar Energy Applications and Development in Nigeria: Drivers and Barriers.” Renewable and Sustainable Energy Reviews 32: 294–301. doi:10.1016/j.rser.2014.01.014.
- Olatomiwa, L., S. Mekhilef, M. S. Ismail, and M. Moghavvemi. 2016. “Energy Management Strategies in Hybrid Renewable Energy Systems: A Review.” Renewable and Sustainable Energy Reviews 62: 821–835. doi:10.1016/j.rser.2016.05.040.
- Onyeka, C., O. Taylan, and D. K. Baker. 2016. “Solar Energy Potentials in Strategically Located Cities in Nigeria- Review, Resource Assessment and PV System Design.” Renewable and Sustainable Energy Reviews 55: 550–556. doi:10.1016/j.rser.2015.10.154.
- Ozoegwu, C. G., C. A. Mgbemene, and P. A. Ozor. 2017. “The Status of Solar Energy Integration and Policy in Nigeria.” Renewable and Sustainable Energy Reviews 70: 457–471. doi:10.1016/j.rser.2016.11.224.
- Papari, S., and K. Hawboldt. 2015. “A Review on the Pyrolysis of Woody Biomass to Bio-oil: Focus on Kinetic Models.” Renewable and Sustainable Energy Reviews 52: 1580–1595. doi:10.1016/j.rser.2015.07.191.
- Phuphuakrat, T., T. Namioka, and K. Yoshikawa. 2010. “Tar Removal from Biomass Pyrolysis Gas in Two-Step Function of Decomposition and Adsorption.” Applied Energy 87 (7): 2203–E11. doi:10.1016/j.apenergy.2009.12.002.
- Piatkowski, N., C. Wieckert, and A. Steinfeld. 2009. “Experimental Investigation of A Packed-Bed Solar Reactor for the Steam-Gasification of Carbonaceous Feedstocks.” Fuel Processing Technology 90 (3): 360–366. doi:10.1016/j.fuproc.2008.10.007.
- Ragwitz, M., J. Winkler, C. Klessmann, M. Gephart, and G. Resch. 2012. “Recent Developments of Feed-in Systems in the EU-A Research Paper for the International Feed-In Cooperation.” Nature Conservation and Nuclear Safety (BMU), 1–16. Bonn: Ministry for the Environment.
- Ringer M., V. Putsche, and J. Scahill. 2006. “Large-Scale Pyrolysis Oil Production: A Tech-Nology Assessment and Economic Analysis.” NREL, Colorado.
- Riti, J. S., and Y. Shu. 2016. “Renewable Energy, Energy Efficiency, and Eco-friendly Environment (R-E5) in Nigeria. Energy.” Sustainability and Society 2016: 6. doi:10.1186/s13705-016-0072-1.
- Ruijs, A., and H. R. J. Vollebergh. 2013. “Lessons from 15 Years of Experience with the Dutch Tax Allowance for Energy Investments for Firms.” SSRN Electronic Journal. doi:10.2139/ssrn.2282436.
- Salman, B., S. Nomanbhay, and A. A. Salema. 2019. “Microwave-synthesised Hydrothermal Co-pyrolysis of Oil Palm Empty Fruit Bunch with Plastic Wastes from Nigeria.” Biofuels 1–17. doi:10.1080/17597269.2019.1626000.
- Salman, B., S. Nomanbhay, and D. C. Y. Foo. 2018. “Carbon Emissions Pinch Analysis (CEPA) for Energy Sector Planning in Nigeria.” Clean Technologies and Environmental Policy. https://doi.org/10.1007/s10098-018-1620-5.
- Sambo, A. S. 2009. “Strategic Developments in Renewable Energy in Nigeria.” International Association of Energy Economics 4: 15–19.
- Sharma, A., V. Pareek, and D. Zhang. 2015. “Biomass Pyrolysis—A Review Of Modelling, Process Parameters And Catalytic Studies.” Renewable and Sustainable Energy Reviews 50: 1081–1096. doi:10.1016/j.rser.2015.04.193.
- Sharma. A, P. Vishnu, and Z. Dongke . 2015. “Biomass Pyrolysis—A Review Of Modelling, Process Parameters And Catalytic Studies”. Renewable and Sustainable Energy Reviews 50: 1081–1096.
- Simonyan, K., and O. Fasina. 2013. “Biomass Resources and Bioenergy Potentials in Nigeria.” African Journal of Agriculture, no. 8: 4975–4989. doi:10.5897/AJAR2013.6726.
- Solargis. 2018. “Solar resource maps of Nigeria.” Global Solar Atlas 2.0. The World Bank. available at https://solargis.com/maps-and-gis-data/download/nigeria
- Sun shot 2012. “Low-Cost, Lightweight Solar Concentrator.” Concentrating Solar Power. www.solar.energy.gov/sunshot/csp.html
- Tyler, R. J. 1979. “Flash Pyrolysis of Coals. Devolatilization of A Victorian Brown Coal in A Small Fluidized-Bed Reactor.” Fuel 58 (9): 680–686. doi:10.1016/0016-2361(79)90223-0.
- Ugolin, N. 2010. “Method Using Solar Energy, Microwaves And Plasmas To Produce A Liquid Fuel And Hydrogen From Biomass Or Fossil Coal”, US Patent. US 8388706.
- United Nation Development Programme (UNDP). 2010. “International Financing Mechanisms for Renewable Energy in the Pacific Island Countries”. Accessed November 30, 2018. www.climateparl.net
- Uzoma, C. C., C. E. Nnaji, C. N. Ibeto, C. G. Okpara, O. O. Nwoke, I. O. Obi, and O. U. Oparaku. 2011. “Renewable Energy Penetration in Nigeria: A Study of the South-East Zone.” Continental Journal of Environmental Science 5 (1): 1–5.
- Vamvuka, D. 2011. “Bio-oil, Solid and Gaseous Biofuels from Biomass Pyrolysis processes-An Overview.” International Journal of Energy Research 35 (10): 835–862. doi:10.1002/er.1804.
- Wiktorsson L.P, and W. Wanzl. 2000.“ Kinetic Parameters For Coal Pyrolysis At Low And High Heating Rates—A Comparison Of Data From Different Laboratory Equipment.” Fuel 79: 701–716.
- World Bank. Fiscal statistics Jan - July 2017. “Contribution to GDP; World Bank National Accounts Data, and OECD National Accounts Data Files.” [ Accessed 6 June 2018. https://data.worldbank.org/indicator/NY.GDP.MKTP.CD
- World energy council. 2013. “World Energy Resources 2013 Survey.” Available at www.worldenergy.org
- Yaman, S. 2004. “Pyrolysis of Biomass to Produce Fuels and Chemical Feedstocks.” Energy Conversion and Management 45 (5): 651–671. doi:10.1016/S0196-8904(03)00177-8.
- Zeng, K., D. Gauthier, D. P. Minh, E. Weiss-Hortala, A. Nzihou, and G. Flamant. 2017. “Characterization Of Solar Fuels Obtained From Beech Wood Solar Pyrolysis.” Fuel 188: 285–293. doi:10.1016/j.fuel.2016.10.036.
- Zeng, K., D. Gauthier, and G. Flamant. 2014. “High Temperature Flash Pyrolysis of Wood in A Lab-Scale Solar Reactor.” In Proceedings of ASME 2014 8th International Conference on Energy Sustainability. Boston, USA.
- Zeng, K., D. Gauthier, R. Li, and G. Flamant. 2015. “Solar Pyrolysis of Beech Wood: Effects of Pyrolysis Parameters on the Product Distribution and Gas Product Composition.” Energy 93: 1648e–1657. doi:10.1016/j.energy.2015.10.008.