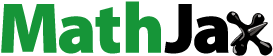
ABSTRACT
Environmental awareness of the effects of single-use plastic packaging and the shortage of non-renewable resources have encouraged the search for alternative materials. Most of single-use plastic packaging is in the food sector, including bags, straws, bottle lids, coffee stirrers, soda and water bottles. Biopolymers and biocomposites have a high potential to be eco-friendly, economic and technically competitive with fossil-based plastics and conventional composites. This paper presents a case study of life-cycle analysis supporting the design and development of a bottle lid as food packaging by applying a life-cycle engineering methodology to select a biocomposite material. Two current materials with high density polyethylene (HDPE) and polylactic acid (PLA) and 10 alternative biocomposite materials of hybrid matrix (mixtures of HDPE and PLA) and contents of banana fibre (BF) were evaluated. The analysis is performed by integrating of life cycle assessment, life cycle cost and technical analysis. The results show that the material with higher BF content is the best alternative both technically and environmentally, while the material with lower BF content is the best alternative economically. Therefore, the importance attributed to each dimension of analysis is pondered and traded-off to allow an aggregated analysis of the alternatives and a well-informed decision-making.
1. Introduction
In 2018, 380 million tons of plastic was discarded, and packaging sector contributed approximately 36% (Westminster Citation2018), mostly single-use packaging. Around 79% of plastic waste is improperly discarded, and it accumulates in sanitary landfills and oceans, exceeding its capacity due to its non-biodegradability. Among the most contaminating single-use packaging are bottles and lids (Ocean-Conservancy Citation2017). In Colombia, only between 10% and 15% is recycled (Pastori Citation2019). Alternatively, single-use packs of environmentally friendly materials could be one of the options to counteract the effects of plastics.
From the point of view of environmental protection, biocomposites are considered a viable and sustainable option since they can replace fossil-based polymers in different applications (Correa et al., 2019; Papong et al., 2014). There has been an increase of 11% in demand of biocomposites between 2014 and 2019 with uses in automotive industry, construction sector and short-term and long-term applications (e.g. packaging and furniture, respectively) (Gurunathan, Mohanty, and Nayak Citation2015; Mohammed et al. Citation2015; Sun Citation2017). The biocomposite materials containing bio-based resources, like natural fibres and/or biopolymers, increase their degradation rate by composting process and decreasing the emission of toxic components (Gunti, Ratna Prasad, and Gupta Citation2018; Loureiro and Esteves Citation2019). Since agriculture in developing countries supports the livelihoods of millions of small farmers and low-wage workers, the production of materials with agricultural resources is a great opportunity for their social and economic progress. (Kim and Kim Citation2013; Luz, Caldeira-Pires, and Ferrão Citation2010). However, broad sustainability analysis is essential for any investment in biocomposite industries to alleviate environmental, economic, and safety risks regarding its commercial applications.
The selection of a sustainable product must include the economic and environmental assessments during its life cycle (Zuoren et al. Citation2016). This is a multi-objective problem, being the optimal selection of the best match found between the available material profiles for the functional performance required for the application. Therefore, simultaneous evaluation of life-cycle assessment (LCA), life cycle cost (LCC) and technical assessment provides useful information to improve and optimise the decision process in the selection of biocomposites (Ahmed and Tsavdaridis Citation2018; Alves et al. Citation2011; Duigou and Baley, Citation2014). The most applied method that includes these three dimensions is life-cycle engineering (LCE), which has been used for the selection of materials in banana and flax fibre biocomposites (Ribeiro et al. Citation2019; Rodríguez et al. Citation2018). In the LCE method, the importance attributed to each dimension is weighted and traded-off to permit an aggregated analysis of the options. LCE is related to sustainability as the optimal development of products, whilst minimising the economic and environmental impacts over the entire life cycle of the product (Luttikhuis, Toxopeus, and Lutters Citation2015).
This study investigates the evaluation of using a new material with different biocomposite blends through the application of the LCE approach developed by Ribeiro et al., (Citation2008) and following the LCA steps. A previous study was conducted to analyse the LCA of bottle lids made from biocomposites (L. Joana Rodríguez et al. Citation2020). As a step forwards in the quantification of the sustainability of materials, in this work, economic and technical dimensions were integrated from a life cycle perspective. In addition, the decision-maker’s strategy was taken into account when assigning relevance/importance to each of the dimensions in the final selection. In the particular case of bottle lids for food packaging, different BF, biopolymer and polymer blends in the product will be compared regarding its sustainability performance from a life cycle perspective.
2. LCE model
This section describes the application of the LCE methodology to evaluate disposable bottle lids made of various mixtures of materials.
2.1 LCE methodology and tools
shows the general methodology of LCE. First are defined the goal and scope, and the common boundaries for all the scenarios for the integration of the dimensions. The environmental and economic dimensions were performed by the LCA and LCC methods. The technical dimension was evaluated by the performance of the materials based on their properties. Specific inventory data is collated for the product and analysed using decision matrices according to Ribeiro et al., Citation2008.
The performance for each material and dimension was obtained by weighing the evaluation in a single indicator. Global evaluation becomes a multi-criteria decision problem which is represented in a ternary diagram; each axis of the triangle represents one dimension (). A point within the triangle represents a weighting combination, and the mixing triangle always adds up to 100% (Hofstetter et al. Citation1999). The relative weight can be changed according to strategic decisions of stakeholders or designers for sensitivity analysis. The alternatives are represented as areas within the triangle, and for each weighting set, the triangle shows graphically which alternatives score best.
2.2 Environmental assessment LCA
The LCA method was based on the ISO standard (ISO 14,040, Citation2006) and on the International References Life Cycle Data System Hand Book (ILCD, Citation2010). The life cycle impact assessment was performed using the software SimaPro, version 8.2.3 (PReConsultants, the Netherlands). The ReCiPe EndPoint 2016 method with a hierarchical perspective (World ReCiPe H/H) including Human Health, Ecosystems and Resources were selected.
2.3 Economic evaluation LCC
According to AS/NZS 4536:1999 standard, LCC is defined as a process to determine total expenses related with a product (Standard ANZ Citation1999). The LCC model () allows the assessment of the product’s life cycle cost by mass streams, facilities, labour, utilities and transport.
The simulator SuperPro Designer® (SPD) version 10 (Intelligent, Inc. US) was used to support the modelling of lid processing. The input information () includes capital costs, operating costs, raw materials, logistic costs (transport and storage) and processing characteristics (labour, utility requirements, facility parameters) and other auxiliary costs to produce 550.000 lids per month. The size of the equipment can be specified initially, or the simulator can determine the necessary size, both cases are verified based on the material balance (Intelligen Citation2019). The simulator also calculates the duration of operations based on processing time and loading speed and the demand for resources (e.g., demand for labour, raw materials, power) as a function of time. The report of results includes information on capital investment, annual production, unit production cost, revenues, profit, gross margin, return on investment and payback time. The costs are listed as $/kg, $/Batch, and $/Year (Intelligen Citation2019).
A simulation of the current material and process was done to validate the real industrial cost of the lid. The dimensionless and weighing of the costs must be done according to the results in a product with lower LCC, having this material a 100% score.
2.4 Technical evaluation
The technical evaluation refers to material properties and processing characteristics of the prototype and their contribution to the technical requirements of the product, in this case, the lids. outlines the steps of this evaluation in two matrices: product requirements and material technical evaluation. The result is a score for each material under evaluation, which is a relative quantification of the appropriateness of each material for the technical performance of the product. The first matrix identifies the product requirements Reqj, and their relative importance Rj. Then, the relevant material properties related with the requirements are identified Pi and their relative importance Wij. The sum of the indexes Wij in each line must be equal to Rj i.e., . Therefore, the value of Rj must be preset and distributed through one or several properties, the Wij can also be zero, meaning that there is no correlation. The absolute importance is the sum of the correlation of Ri and Wij i.e.,
. Finally, the relative importance wPi for the product performance (requirement weights) will be calculated:
The product requirements are related to the material technical evaluation matrix through indices wPi, that reflect the contribution of the property Pj to accomplish the requirement. The second matrix is the construction of a comparison table of the materials with the weighting index of their quantified properties. The values of the properties Value1k are calculated using different characterisation methods. Then, the properties must be dimensionless AV1k and weighted according to the material property weight, . Finally, the addition of all the weighted material indexes MIk is the sum of
. The potentially best technical option material will be the one with the highest MIk. Therefore, the capacity of a specific material to fulfill the requirements depends on its properties.
3. Results and discussion
This section presents the environmental, economic and technical assessment results and the global evaluation by the application of the LCE model for material selection in food packaging. No finite-element analysis was done to identify the number of layers and fibre percentage required to achieve the same mechanical behaviour and use phase is not translated in neither cost nor environmental damage (since relevant effects do not exist). Therefore, the alternative technical performance comparison was based on the measured properties of prototypes fabricated from biocomposites materials.
3.1 Disposable lids for food packaging
With the aim of evaluating the replacement of current petrochemical base polymer by bio-based materials, we developed a case study with a Colombian company of plastic packaging that produces disposable lids. One of the options is PLA; however, the high cost of this material reduces its competitiveness compared with synthetic polymers and presents low thermal and water resistance and some fragility (Gupta Citation2014; Jantanasakulwong et al. Citation2016). One option studied to reduce costs while maintaining mechanical properties is natural fibres as fillers in biocomposites (Gallego et al. Citation2014; Lu et al. Citation2016). BF can be extracted from pseudostems as agricultural residues of the plantain postharvest banana crops. Therefore, biocomposites would reduce the use of non-renewable and non-biodegradable resources and increase biobased sources such as BF and biopolymers such as PLA.
In this study, we evaluated biocomposites from hybrid matrix HDPE-synthetic/PLA–biopolymer and the use of BF as filler as a way to get a balance between environmental and economic parameters while increasing or maintaining the technical performance. Therefore, this study analyses the use of different blends of materials for bottle lids. Different mixture compositions were selected using experimental design on blends with BF (10–40%), PLA (30%–60%) and HDPE (30%-60%). shows the different material alternatives. Bottle lids from 100%-HDPE and 100%-PLA as current materials are also included for the study.
Table 1. Biocomposite blend alternatives for bottle lids
Table 2. LCA inventory data sources of the disposable lid life cycle
Table 3. LCC inventory data sources of the disposable lid life cycle
3.2 Lids life cycle
shows some stages of the process that begin after the harvest with the collection of the pseudostem residues (raw material for fibre extraction) (). The pseudostem corresponds to the 15.2% of the total weight of the plant, about 25.0% corresponds to the fruit and the remaining 59.8% is agricultural residues (Mazzeo Meneses et al. Citation2012). The pseudostems from three farms were cut, and their external layer was removed (). The leaves, internal stems and bulbs were left in the soil as organic matter.
The BF was extracted from a decorticator, washed and dried outdoors (). The transport was from the farms to the collection centres and the manufacturing plant.
At the manufacturing plant, the fibres were milling and drying. Then, fibres, HDPE (Esenttia), PLA (NatureWorks) from corn starch, and anhydride-modified polyethylene (Dupont) were mixed in a torque rheometer according to the different blend formulations () and injected (). During the use phase, the material/energy required for the sealing process was not considered because it does not differ from one alternative to another. Service life is around 1 year, and then the waste is collected by the local garbage service for the landfill.
3.3 Goal and scope
The aim of this study is to select a biocomposite material containing different blends of BF, HDPE and PLA for a bottle lid by applying the LCE approach that includes environment, economy and technology assessment. The functional unit corresponds to a lid of 53 mm diameter and 13.44 g to cover a bottle.
shows the system boundaries within the stages for the fabrication of disposable lids in environmental, economic, and technical dimensions. For analysis the geographical scope of this study is Colombia. The system contains the postharvest collection of raw material banana-pseudostems, transportation, BF production, BF preparation, pellet production (BF, PLA, HDPE, and compatibilizer), lid/prototype fabrication and final disposition. A comprehensive analysis is assured from a life cycle perspective, so all the life cycle phases relevant for the case study are assessed, using life cycle-based methodologies to measure the environmental performance (LCA) and the economic performance (LCC). The LCA and LCC include the cradle-to-grave system (orange and green shading in ). Technical evaluation is performed with the characterisation of the required properties of the materials for the injection of the lids. Therefore, the boundaries of technical dimensions correspond to the characterisation of injected prototypes (grey shading).
3.4 Life Cycle Inventory
displays the input-output data by stage and unit process for LCA. The inventory data were based on primary information from local farms, agricultural associations, businesses, laboratory analysis, pilot processes, and secondary information from bibliographic sources. Relevant data were the composition of the pseudostem, transport distances, energy consumption, utilities, and the waste generated within the limits of the system. The data for the background system, like machinery, electricity and waste emissions were taken from the Ecoinvent version 3. The complete inventory data of LCA for bottle jars can be consulted in L Joana Rodríguez et al., (Citation2020).
The input-output data (material balance and energy demand) for LCC were the same as those used for LCA. shows the costs taken from fibre production at a pilot scale and from processing fibre milling until biocomposites injection at the laboratory scale, supplemented with manufacturing and web data. Administrative costs were not taken into account.
The technical evaluation has considered the most important requirements by the disposable lid material: light, stiff, allow forming processes, easy to handle and resistant. The properties of interest were stabilisation torque (N.m.) and stabilisation time (min) in the mixer and density (g/cm3), tensile strength (MPa) and ductility (%). Data were measured from the torque curve during the fabrication of the composites by melt blending of HDPE/PLA/BF in a Haake rotor mixer (Model RHEODRIVE 7 HAAKE POLYLAB OS, Germany) and manufactured by prototypes in an injection moulding (LIEN YU Machinery Co. Ltd, Taiwan). The densities were calculated by dividing the weight by the volume for the composite sample. The tensile test was conducted according to ASTM D 638-14 using Universal Testing Machine (Instron 10 KN) (ASTM_D638-14 Citation2014).
3.5. LCE assessment
3.3.1. Environmental evaluation
shows the life-cycle impact assessment (LCIA) results for different alternatives. The 100%-HDPE lids have the worst behaviour followed by the 100%-PLA and then the studied alternative blends of biocomposite lids. The inclusion of BF in the lids positively influenced the environmental impact since the higher the fibre content, the lower the impact, being the 40% fibre lid with the lowest impact. In general, for all lids, the greatest impact occurs on resources, followed by the impact on human health and the least impact on ecosystems. Table A2 shows the impact values of the different alternatives.
Figure 6. ReCiPe ENDPOINT impact categories for the impacts human health, ecosystems and resource of two base lids 100%-HDPE and 100%-PLA and ten biocomposite lid alternative
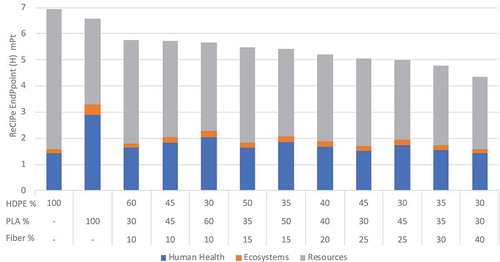
shows the contributors to environmental impacts. Among the different lids, the largest contributors were the production of HDPE and PLA, providing with 70% to 80% of the total impact. The operations related to fibre such as collection, extraction and treatment contributed less than 10%.
3.3.2 Economic evaluation
The flowchart design was based on the inventory data and the process engineering expertise of the authors. displays the flowsheet of the overall process of lids with 40%-BF, 30%-PLA and 30%-HDPE (Table A3 shows the total results of this model). The procedures were modelled with batch process simulators. The process flow shows an initial raw material demand of 1500 kg of wet pseudostem total that is transported to the defibration zone. Depending on the difference between the inflow and the amount that is defibrated, a storage stage is required. At the end of the production line, an amount of 27 kg/batch of dry fibre is obtained and stored. Then it goes on to the fibre preparation and extrusion process with PLA, HDPE and compatibilizer. Finally, the lids of biocomposite materials are injected with a production rate of 67 kg/batch or 5007 lids/batch. The and Table A4 show the costs per lid of the real process (0.051 USD simulated vs. 0.050 USD real value) confirming the accuracy of the simulation.
Figure 8. Flowsheet of the overall process of lids with 40% of banana fibre, 30% of PLA and 30% of HDPE
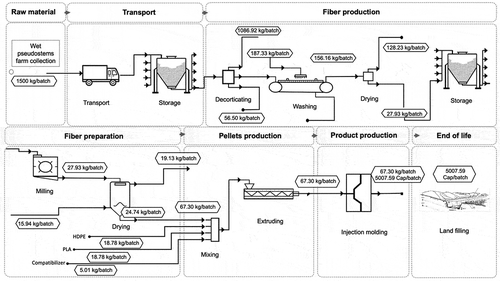
shows the LCC for different alternatives which were manufactured according to the process flowsheet of . The costs for all alternatives are in Table A5. In all the cases, the lids with biocomposite materials showed a higher LCC than the lids made with HDPE. The final costs of the biocomposite increased between 50% and 112% respect to HDPE. However, with respect to lids made from 100%-PLA the relative costs of biocomposite lids fluctuated between 14% lower and 22% higher, depending on their composition.
Analysing the , the lids manufactured with biocomposite materials, pellet production was the largest cost component of their total production costs (from 35% to 57% for 40% BF lids and 10% BF lids, respectively) followed by lid production cost (from 17% to 24% for 40% BF lids and 10% BF lids, respectively), and BF production cost (from 10% to 48% for 10% BF lids and 40% BF lids, respectively). Since fibre production demands a lot of unskilled labour, in contrast to highly automated industrial production of PLA and HDPE, there is room for cost reduction, should the demand for fibre allow the establishment of a profitable operation.
3.3.3 Technical evaluation of alternatives
The requirements that must meet the lid material () were weighted according to the different levels of importance for the performance of the lid. The technical evaluation was then correlated to material properties although the weight of some of them could be zero. shows the different weight of the requirements to fulfill the lid material overall quality. The analysis points out that the ductility and the processing characteristics of stabilisation torque and time are the most important properties for the selection of a material.
Table 4. Product requirements evaluation and weights applied for the material properties
The material properties were measured on the fabricated prototype, dimensionless and compared with the objective for each material property () and multiplied by the weight importance of each property. The total score of each material represents its technical performance evaluation, being 100% the highest possible score. The material with the highest score in performance evaluation was the lids with 40% of BF, 30% of PLA and 30% of HDPE. On the contrary, the worst score was attributed to the lids with 10% of BF, 40% of PLA and 40% of HDPE.
Table 5. Technical performance evaluation
Dim: dimensionless
3.4 Global evaluation
The results obtained from the environmental-LCA, economic-LCC and technical dimension were integrated in for performing the global evaluation. As the dimensions have different units, the values are dimensionless to allow the attribution of importance weights (dimension weights) in the ternary diagram. The evaluator can perform different combinations; thus, the selection of a material is directly affected by the different combinations of weights. diagram illustrates the material selected for a particular set of important weights and the domain of weights for this selection. Two situations were evaluated: first, the compositional alternatives include lids of pure HDPE and PLA (Approach 1 in ); second, only lids made of mixtures of BF, HDPE and PLA were considered (Approach 2 in ). The best options for each of the dimensions are underlined in grey, so the lids with the greatest amount of BF are the best environmental alternative in both approaches.
Table 6. Dimensionless (Dim) data from the environmental, economic and technical performance
Figure 11. Global evaluation of materials for lids based on environmental, economic and technical performance dimensions. Weight criteria: environmental 50% economic 30% and technical 20%. a. Approach 1 b. Approach 2 (Table 6)
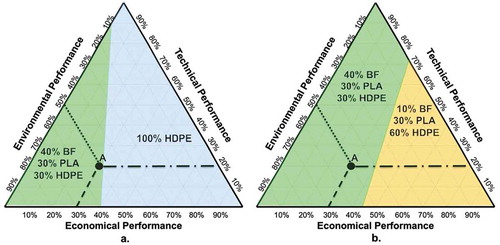
For example, for a set of weights of importance of 30% for to the economic dimension, 50% for the environmental dimension and 20% for the technical performance; then the scenario can be characterised by the point A (. and b.) approach 1 (40%BF/30%PLA/30%HDPE and 100%HDPE) and approach 2 (40%BF/30%PLA/30%HDPE and 10%BF/30%PLA/60%HDPE). In both cases, the best choice is the material with higher content in BF. Instead, if the goal is to give more importance to economic dimension (>50%), then the best material options would be pure HDPE (approach 1) or 10%BF/30%PLA/60%HDPE (approach 2). The above shows that among the three components, the greatest influence on the combined performance in three dimensions is BF. If the percentage of BF does not vary, the greater amount of HDPE influences a lower cost of the lids and a higher technical performance and with a greater amount of PLA the environmental impact decreases. In approach 2, the result shows that only the extreme blends (lower and higher BF content) may appear selected as the best option– depending on the different weighting criteria, while those of intermediate fibre content do not obtain the best scores, so they are not an overall acceptable alternativeusing the ternary diagram.
4. Conclusions
In this paper, the evaluation and the selection of different biocomposite blends as alternative materials for current plastic lids were evaluated using theLCE approach. The evaluation integrated environmental, economic and technical dimensions using LCA, LCC and technical assessment, respectively. The materials were evaluated by comparison of two current materials HDPE and PLA and 10 alternative biocomposite materials (mixtures of BF 10%–40% and HDPE and PLA 30%–60%. A complete inventory of data was collected for each material. Results of impact assessment were obtained for every dimension. In the environmental dimension, the lowest impact was attained by the lids with the highest BF content at the recipe end point (H) method. In contrast, the life cycle costs increase with the highest percentage of fibre with respect to the current materials. With regard to the technical performance, the behaviour was not correlated with the percentage of fibre, but there were found other factors such as the amount of PLA and HDPE influenced the results. The lids with the best technical composition were the ones with the highest fibre content. With the LCE and the use of diagrams, it was possible to visualise and vary the weighting of each dimension, and thus select the best option, according to a specific goal. The extreme blends (lower and higher BF content) appeared as best alternatives by the ternary diagram method, while the middle BF blends never gave the highest score option for any set of weights. Through multi-dimensional evaluation, it is possible to provide easy, quick and meaningful evaluation criteria that are very useful for decision makers.
Supplemental Material
Download Zip (32.2 KB)Supplemental Material
Download MS Word (163.5 KB)Acknowledgments
The authors acknowledge the financial support of Departamento Administrativo de Ciencia, Tecnología e Innovación, Doctorados Nacionales [727, 2015], the Fondo Regional de Tecnología Agropecuaria, FONTAGRO [ATN/RF-16111 RG, 2016], and by FCT, through IDMEC, under LAETA, project UIDB/50022/2020. Also, this article is the outcome of the work developed through the “Programa de investigación reconstrucción del tejido social en zonas de pos-conflicto en Colombia”[SIGP 57579] with the research project “Competencias empresariales y de innovación para el desarrollo económico y la inclusión productiva de las regiones afectadas por el conflicto colombiano” [SIGP 58907], financed in the framework of the Colombia Científica Call [FP44842-213-2018].
Disclosure statement
No potential conflict of interest was reported by the author(s).
Supplementary material
Supplemental data for this article can be accessed here.
Additional information
Funding
Notes on contributors
L. Joana Rodríguez
L. Joana Rodríguez is Industrial Engineering, M.Eng, and PhD student of the Universidad Nacional de Colombia. She has worked at the Instituto de Biotecnología y Agroindustria at the Universidad Nacional de Colombia, where she has focused his work on various areas such as the physical-chemical characterization of biocomposite materials with natural fibers such as the pseudostem of banana, evaluation of sustainability through analysis of the cycle life, life cycle cost and social life cycle analysis.
Sebastian Ospina
Sebastian Ospina is a PhD student from the Universidad de Zaragoza, España, and Chemical Engineer M.Eng. from the Universidad Nacional de Colombia. He has been a member of the Alimentos Frutales research group for more than four years, where his work has focused on the design and process engineering of food related production systems such as the obtention of prebiotic fiber, design of minimally processed food products, accelerated shelf-life analysis, among others, while keeping in focus a sustainable perspective and processes adapted to the reality of Colombian production systems.
Inês Ribeiro
Inês Ribeiro is assistant professor of the Manufacturing Technology and Industrial Management Scientific Area of the Mechanical Department of Instituto Superior Técnico at Universidade de Lisboa and researcher of IDMEC, the mechanical engineering institute of LAETA - Associated Laboratory for Energy, Transports and Aeronautics. Her research is focused on the fields of process-based models, sustainability, technology evaluation related with business models and Life Cycle Design.
Paulo Peças
Prof. Paulo Peças is associated professor of the Manufacturing Technology and Industrial Management Scientific Area of the Mechanical Department of Instituto Superior Técnico at Universidade de Lisboa. He is also senior researcher of IDMEC, the mechanical engineering institute of LAETA - Associated Laboratory for Energy, Transports and Aeronautics. He as published dozens of publications in scientific journals, books and conference proceeding, as well as coordinate and participate various research projects in the field of life cycle engineering, sustainable production, technology and materials selection models, and Lean manufacturing.
Carlos E. Orrego
Carlos E. Orrego is a Chemical Engineer, Specialist in Science - Physics, Specialist in Food Science and Technology and PhD in Science - Chemistry from The Universidad Nacional de Colombia. He is currently full professor and the head of the Instituto de Biotecnología y Agroindustria at the Universidad Nacional de Colombia- Manizales. . His research interests include drying and freeze-drying of foods, agri-food processing, food design, processing for adding value to agricultural and agro-industrial waste and enzyme catalysis.
References
- Ahmed, I. M., and K. D. Tsavdaridis. 2018. “Life Cycle Assessment (LCA) and Cost (LCC) Studies of Lightweight Composite Flooring Systems.” Journal of Building Engineering 20: 624–633. doi:https://doi.org/10.1016/j.jobe.2018.09.013.
- Alves, C., A. P. S. Dias, A. C. Diogo, P. M. C. Ferrão, S. M. Luz, A. J. Silva, L. Reis, and M. Freitas. 2011. “Eco-composite: The Effects of the Jute Fiber Treatments on the Mechanical and Environmental Performance of the Composite Materials.” Journal of Composite Materials 45: 573–589. doi:https://doi.org/10.1177/0021998310376111.
- ASTM_D638-14, 2014. Standard Test Method for Tensile Properties of Plastics.
- Duigou, A.Le, and C. Baley. 2014. “Coupled Micromechanical Analysis and Life Cycle Assessment as an Integrated Tool for Natural Fibre Composites Development.” Journal of Cleaner Production 83: 61–69. doi:https://doi.org/10.1016/j.jclepro.2014.07.027
- EMAS, 2019. Servicio público de Aseo 2019. Accessed 8.22.19. https://www.emas.com.co/
- Gallego, R., S. López-Quintana, F. Basurto, K. Núñez, N. Villarreal, and J. C. Merino. 2014. “Synthesis of New Compatibilizers to Poly(lactic Acid) Blends.” Polymer Engineering & Science 54: 522–530. doi:https://doi.org/10.1002/pen.23589.
- Gunti, R., A. V. Ratna Prasad, and A. V. S. S. K. S. Gupta. 2018. “Mechanical and Degradation Properties of Natural Fiber-reinforced PLA Composites: Jute, Sisal, and Elephant Grass.” Polymer Composites 39: 1125–1136. doi:https://doi.org/10.1002/pc.24041.
- Gupta, K. M. 2014. “Engineering Materials: Research.” Applications and Advances 28 (4): 18–33.
- Gurunathan, T., S. Mohanty, and S. K. Nayak. 2015. “A Review of the Recent Developments in Biocomposites Based on Natural Fibres and Their Application Perspectives.” Composites Part A: Applied Science and Manufacturing 77: 1–25. doi:https://doi.org/10.1016/j.compositesa.2015.06.007.
- Hofstetter, P., A. Braunschweig, T. Mettier, R. Müller-Wenk, and O. Tietje. 1999. “The Mixing Triangle: Correlation and Graphical Decision Support for LCA-based Comparisons.” Journal of Industrial Ecology 3: 97–115. doi:https://doi.org/10.1162/108819899569584.
- ILCD, 2010. European Commission - Joint Research Centre - Institute for Environment and Sustainability: International Reference Life Cycle Data System (ILCD) Handbook - General guide for Life Cycle Assessment - Detailed guidance. First edition March 2010. EUR 24708 EN. Luxembourg. Publications Office of the European Union; 2010.https://doi.org/10.2788/94987
- Intelligen, I., 2019. SuperPro Designer Product Features. URL https://www.intelligen.com/index.html ( accessed 4.27.20).
- ISO 14040, 2006. ISO 14040:2006 Environmental Management — Life Cycle Assessment — Principles and Framework.
- Jantanasakulwong, K., N. Leksawasdi, P. Seesuriyachan, S. Wongsuriyasak, C. Techapun, and T. Ougizawa. 2016. “Reactive Blending of Thermoplastic Starch and Polyethylene-graft-maleic Anhydride with Chitosan as Compatibilizer.” Carbohydrate Polymers 153: 89–95. doi:https://doi.org/10.1016/j.carbpol.2016.07.091.
- Kim, H.-S., and H.-J. Kim. 2013. “Miscibility and Performance Evaluation of Natural-flour-filled PP/PBS and PP/PLA Bio-composites.” Fibers Polymer 14: 793–803. doi:https://doi.org/10.1007/s12221-013-0793-0.
- Loureiro, N. C., and J. L. Esteves. 2019. “Green Composites in Automotive Interior Parts: A Solution Using Cellulosic Fibers.” In Green Composites for Automotive Applications, edited by G. Koronis and A. Silva, 81–97, Woodhead Publishing-Elsevier. doi:https://doi.org/10.1016/B978-0-08-102177-4.00004-5
- Lu, X., L. Tang, L. Wang, J. Zhao, D. Li, Z. Wu, and P. Xiao. 2016. “Morphology and Properties of Bio-based Poly (Lactic Acid)/high-density Polyethylene Blends and Their Glass Fiber Reinforced Composites.” Polymer Test 54: 90–97. doi:https://doi.org/10.1016/j.polymertesting.2016.06.025.
- Luttikhuis, E. J. O., M. E. Toxopeus, and E. Lutters. 2015. “Effective Integration of Life Cycle Engineering in Education.” Procedia CIRP 29: 550–555. doi:https://doi.org/10.1016/j.procir.2015.02.040.
- Luz, S. M., A. Caldeira-Pires, and P. M. C. Ferrão. 2010. “Environmental Benefits of Substituting Talc by Sugarcane Bagasse Fibers as Reinforcement in Polypropylene Composites: Ecodesign and LCA as Strategy for Automotive Components.” Resources, Conservation & Recycling 54: 1135–1144. doi:https://doi.org/10.1016/j.resconrec.2010.03.009.
- Mazzeo Meneses, M., L. León Agatón, L. F. Mejía Gutiérrez, L. E. Guerrero Mendieta, and J. D. Botero. 2012. “Aprovechamiento industrial de residuos de cosecha y poscosecha del plátano en el Departamento de Caldas.” Review of Educational Research 5: 128–139.
- Mohammed, L., M. N. M. Ansari, G. Pua, M. Jawaid, and M. S. Islam. 2015. “A Review on Natural Fiber Reinforced Polymer Composite and Its Applications.” International Journal of Polymer Science 15. doi:https://doi.org/10.1155/2015/243947.
- Ocean-Conservancy, 2017. International Coastal Cleanup 2017 Report. URL https://oceanconservancy.org
- Pastori, D., 2019. “Colombia pierde $2 billones anuales por no reciclar desechos plásticos.” El Her.
- Ribeiro, I., J. Kaufmann, U. Götze, P. Peças, and E. Henriques. 2019. “Fibre Reinforced Polymers in the Sports Industry– Life Cycle Engineering Methodology Applied to a Snowboard Using Anisotropic Layer Design.” International Journal of Sustainable Engineering 12: 201–211. doi:https://doi.org/10.1080/19397038.2018.1508318.
- Rodríguez, L. J, Fabbri, S., Orrego, C. E., and Owsianiak, M., 2020. “Comparative Life Cycle Assessment of Coffee Jar Lids Made from Biocomposites Containing Poly(lactic Acid) and Banana Fiber„. Journal of Environmental Management 266. doi:https://doi.org/10.1016/j.jenvman.2020.110493
- Ribeiro, I., P. Peças, A. Silva, and E. Henriques. 2008. “Life Cycle Engineering Methodology Applied to Material Selection, a Fender Case Study.” Journal of Cleaner Production 16: 1887–1899. doi:https://doi.org/10.1016/j.jclepro.2008.01.002.
- Rodríguez, L.J., Orrego, C.E., Ribeiro, I., Peças, P., 2018. “Life-Cycle Assessment and Life-Cycle Cost study of Banana (Musa sapientum) fiber Biocomposite materials”. Procedia CIRP. 69: 585-590. doi:10.1016/j.procir.2017.11.145
- Rodríguez, L. J., S. Fabbri, C. E. Orrego, and M. Owsianiak. 2020. “Life Cycle Inventory Data for Banana-fiber-based Biocomposite Lids.” Data in Brief 30: 105605. doi:https://doi.org/10.1016/j.dib.2020.105605.
- Standard ANZ, 1999. Life Cycle Costing: An Application Guide.
- Sun, Z. 2017. “Progress in the Research and Applications of Natural Fiber-reinforced Polymer Matrix Composites.” Science and Engineering of Composite Materials 25: 835–846. doi:https://doi.org/10.1515/secm-2016-0072.
- Torrico Silva, I. E., 2016. Natural Gas Vs. Gasoline: the user decides. URL https://www.elmundo.com/portal/noticias/economia/gas_versus_gasolina_decide_el_usuario.php#.XjycQhNKgWo ( accessed 8.20.18).
- UPME, 2019. Measure Mining Energt Planning Unit, Colombia’s.
- Westminster, 2018. Plastic Waste – Everything You Need to Know. Commer. Waste. URL https://cleanstreets.westminster.gov.uk/plastic-waste-complete-guide/( accessed 3.24.20).
- Zuoren, N., L. Yu, B. Sun, W. Zhihong, and Z. Tieyong. 2016. “Research Progress of Life Cycle Engineering and Eco-Design in Materials Industry.”Materials Chinadoi:https://doi.org/10.7502/j.1674-3962.2016.03.01.