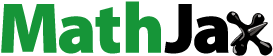
ABSTRACT
The availability of liquid fossil fuels is dwindling. Alternative fuels for CNG and CPO biodiesel that can be used in DF engines are the solution. However, the use of this fuel has reduced engine performance and increased CO and HC emissions. In addition, the use of CPO biodiesel as fuel for ignition in the DF engine is a parameter that has not been developed in previous studies so that it requires adjustment of engine parameter under standard conditions. Pilot injection timing (PIT) is critical in starting combustion in sequential combustion models of DF engine. This study aims to determine the effect of PIT using CPO biodiesel fuel on increasing engine performance and reducing emissions, so that this parameter becomes important. The PIT parameter was varied from 11° to 19° BTDC with 2° intervals at different engine loads on a DF engine. The results showed that CPO biodiesel is an effective pilot fuel in DF engine. PIT has a significant effect on increasing engine performance and reducing emissions. Increased thermal efficiency by 8.87% and HRR by 7.34%, while SFC emissions, CO emissions and HC emissions decreased by 43.85% and 25.33% respectively with a PIT of 15° BTDC at high load.
1. Introduction
The existence of depleting reserves of liquid fossil fuels has become a serious problem today (Agency Citation2020). One solution to overcome this problem is the use of alternative fuels in internal combustion engines. One of the technologies currently developing in the use of alternative fuels is the technology of using dual fuel in diesel engines which is called a dual fuel (DF) engine.
One of the alternative fuels that are widely used in DF engines are compressed natural gas (CNG)/methane and diesel (Königsson Citation2012; Mousavi et al. Citation2016; Mustafi, Raine, and Verhelst Citation2013; Sudarmanta, Bambang, Setiyawan, Atok, P, Ary, Bachtiar, & Yuvenda, Citation2019). Where diesel fuel is used as the main fuel and diesel fuel is used as pilot fuel. Previous studies have reported that DF engines can reduce the consumption of diesel fuel and reduce nitrogen oxide (NOx) emissions and particulate matter (PM) emissions (Mustafi, Raine, and Verhelst Citation2013; Nithyanandan et al. Citation2016; Papagiannakis and Hountalas Citation2004; Yang et al. Citation2017). However, this dual fuel engine technology still faces problems in terms of performance than the standard diesel engine. In addition, its carbon monoxide (CO) and hydrocarbon (HC) emissions are also high (Abdelaal and Hegab Citation2012; Papagiannakis and Hountalas Citation2003, Citation2004).
Several previous studies have analysed engine performance and emissions on dual fuel engines such as; Blasio et al.(Gabriele Di Blasio et al. Citation2015) conducted an experimental evaluation of the effect of compression ratios on engine performance in dual fuel (methane-diesel) light duty engines. The results showed that the compression ratio had an effect on hydrocarbon emissions, especially unburnt methane and combustion efficiency. A reduction in the compression ratio provides the benefit of reducing combustion noise and achieves engine performance at full load. Likewise, carbon dioxide (CO2) emissions can be reduced by substituting natural gas up to 50%. In addition, the study shows that the design of a special combustion system and proper engine calibration can overcome these limitations. Blasio et al.(G Di Blasio, Belgiorno, and Beatrice Citation2017) also conducted further research to determine the effect of variations in the compression ratio on the performance, emissions and particle size distribution of a dual fuel (methane-diesel) light duty engine. The results showed that variations in the compression ratio and injection parameters had an effect on the combustion and emission characteristics. Reducing the compression ratio lowers unburnt methane (UCH4) emissions and reduces combustion noise in a DF engine. A reduction in CO2 emissions by 12% over new European driving cycles (NEDC) with methane (CH4) substitution averaged 50%. The particle concentration in the dual fuel is reduced as is the estimation of the particle number at the NEDC. In addition, a compression ratio of 15.5 provides the best compromise between global efficiency and pollutant emissions on the DF engine. Yuvenda et al. (Yuvenda et al. Citation2020) also analyzes engine performance and emissions on DF engines by optimising injection parameters of CNG fuel under low load. The results showed that by retarding the timing of entering CNG fuel in the air inlet and shortening the injection duration of CNG fuel, it could increase engine power, thermal efficiency, cylinder pressure, heat release rate and reduce fuel consumption and CO and HC emissions.
Then with the development of research on DF engines, several researchers tried to use biodiesel fuel as a substitute for diesel fuel as pilot fuel, as research conducted by previous researchers (Banapurmath et al. Citation2018; Çelebi, Uludamar, and Tosun Citation2017; Hosmath et al. Citation2016; Liu et al. Citation2014; Paul et al. Citation2014, Citation2015; Ryu Citation2013b, Citation2013a; Banapurmath, Basavarajappa, & Tewari, Citation2012).
From the many studies that have been carried out on DF engines, however, the use of crude palm oil (CPO) biodiesel as pilot fuel has not been found in replacing diesel fuel. In fact, the use of CPO biodiesel fuel in standard diesel engines (single fuel) has been done a lot of research as a substitute for diesel fuel as reported in previous studies (Ayetor, Sunnu, and Kesse Citation2019; Bari and Bari Citation2019; Gad et al. Citation2018; Khalid, Tamaldin, Jaat, Ali, & Manshoor, Citation2013; Meisami, Ajam, and Tabasizadeh Citation2017; Wei, Cheung, and Ning Citation2016). This is because the characteristics of CPO biodiesel fuel have the same characteristics of diesel fuel as shown in as follows. In addition to the fuel characteristics that are similar to diesel fuel, the availability of raw materials from CPO biodiesel fuel in Indonesia is very large, this is because Indonesia has extensive oil palm plantations of 14.33 million hectares (Statistics, Citation2019). The production of CPO biodiesel fuel has increased from year to year, where in 2019 it reached an amount of 42.9 million tons (Statistics, S. of E. C Citation2019). Thus, this provides a positive value for the development of renewable energy, especially CPO biodiesel fuel in Indonesia.
Table 1. Fuels characteristics
So far, the use of alternative fuels, namely CNG and biodiesel fuels in DF engines, still causes the same problems as experienced with DF engines using CNG-diesel fuel. This is because the CNG fuel has a higher-octane number and auto-temperature ignition, hence burn with difficulty if ignition of the pilot fuel is not optimal (Abdelaal and Hegab Citation2012; Papagiannakis and Hountalas Citation2004; Sahoo, Sahoo, and Saha Citation2009). Similarly, biodiesel has a high density, viscosity, and shear stress that affects the combustion process (Shahabuddin et al. Citation2013). On the other hand, biodiesel fuel has high cetane and oxygen content, which provide many advantages on emissions (Mofijur et al. Citation2019).
With the use of CPO biodiesel fuel as pilot fuel in this study, the same problems were found with the research previously described on the DF engine which uses other biodiesel as fuel for ignition. Thus it is necessary to improve engine performance and emissions on DF engine through pilot timing injection (PIT) as has been done by; Yang et al. (Yang et al. Citation2016) advancing pilot injection timing increases thermal efficiency, cylinder pressure, HRR, and reduces CO, HC, NOx, and smoke emissions. Wang et al. (Wang et al. Citation2016a) the combustion performance improved while HC emissions decreased with advance pilot injection timing. However, NOx emissions were increased. Yousefi et al. (Yousefi, Guo, and Birouk Citation2019) advancing pilot injection timing increases thermal efficiency, peak cylinder pressure, and engine speeds under all loads. Unburnt methane and carbon dioxide (CO2) emissions significantly reduced under low load and speed. However, there was no significant effect under medium and high load and speed. Since CO and particulate matter (PM) emissions were not analysed, the effect of pilot injection timing was unknown.
This research was conducted using a diesel engine, four steps, one cylinder, which has been modified into a dual fuel engine using CNG fuel and CPO biodiesel. This analysis is more focused on the effect of using CPO biodiesel fuel as pilot fuel on the DF engine which is one of the renewable fuels and then improving engine performance and emissions from the engine through pilot injection timing (PIT) of CPO biodiesel fuel. The analysis is critical due to the importance of the injection timing parameter as combustion initiation on DF engines. The engine performance parameters analysed are power, torque, SFC, and energy per cycle. Combustion process parameters included cylinder pressure, HRR, ignition delay (ID), and combustion duration (CD). The analysed exhaust emissions include CO, HC, and PM. Statistical analysis with regression models was also carried out to determine the effect of injection parameters on engine performance and emissions. The experimental apparatus, the test methodology and the research results are explained in the next session.
2. Experiment apparatus and procedure
2.1 Engine test
The engine used is a direct injection diesel engine, four stroke, one cylinder, naturally aspirated. The engine has a compression ratio of 18: 1. The standard diesel injection timing is 13° BTDC. The details of the engine specifications are described in . The diesel engine is modified to a dual fuel (DF) engine using CNG fuel and CPO biodiesel. CNG fuel acts as the main fuel and CPO biodiesel fuel acts as pilot fuel. The DF engine is also equipped with an electric supercharger in order to forcibly add combustion air to lower the equivalent ratio value that has been studied from previous studies (Trihatmojo, Yuvenda, and Sudarmanta Citation2019). The DF engine is also coupled with an electric generator as a simulation of engine load using lights.
Table 2. Specification of the dual fuel (DF) engines
2.2 Fuel properties and supply system
Dual fuel engine was designed to use dual fuels, the CNG fuel as the main fuel and then the CPO biodiesel used as a lighter (pilot) fuel. The fuel properties can be seen in as follows.
The fuel supply system in a DF engine is divided into two namely; First, the conventional fuel system, used in CPO biodiesel fuel as the pilot fuel. This fuel is injected directly into the combustion chamber without being controlled by an electronic system. The injection pressure of pilot fuel is 200 kg/cm2. Second, the electronic model fuel system is used to regulate the intake of CNG as the main fuel. The CNG fuel is injected through the air inlet with injection pressure 2 bar. The injector for CNG fuel is equipped with an electric magnetic valve that can be controlled by the electronic control unit (ECU) by sending an output signal in the form of an electric voltage. Thus the injection parameters (timing and duration) of CNG fuel can be adjusted according to the crank angle (CA). The value of injection timing (IT) and injection duration (ID) of CNG are 110° ATDC and 70° CA at low load, and 110° ATDC and 110° CA at high load, respectively. The injection parameter value of CNG is based on the optimal value from previous studies (Yuvenda, Sudarmanta, and Wahjudi Citation2019; Yuvenda et al. Citation2020).
2.3 Measuring instruments and data acquisition
shows the schematic experimental setup of this study. The equipments used in this study are as follows; (i) digital manometer and flow metre is used to determine the air flow rate connected to a pitot tube attached to the air inlet using the principles of Bernoulli’s law, (ii) digital gas flow metre is used to determine the CNG flow rate which is installed before the CNG rail injector, (iii) gas analyser and smoke opacity metre connected to the probe attached to the exhaust channel used to determine CO, HC, and PM emissions, (iv) The multimeter is used to determine the voltage and electric current in the electric generator when the engine load is applied using lights, (v) a burette gauge with a volume of 25 ml is used to determine the volume of CPO biodiesel fuel each time by recording the time taken during the volume of the fuel, then used to calculate the mass flow rate of the fuel, (vi) TMR combustion analyser set is then equipped with data acquisition to analyse combustion characteristics. The resolution used in the experiment is 1° CA. Piezoelectric pressure sensors are mounted on the cylinder head to detect pressure, heat release rate, ignition delay, start of combustion, duration of combustion, and end of combustion by providing signals to data acquisition. The encoder device is attached to the crankshaft to detect crank angle (0CA) and engine speed. The specifications of the measuring instrument can be seen in as follows:
Table 3. Measuring instruments specification and measurement uncertainty analysis
2.4 Uncertainty analysis
Every measurement is infested with uncertainty. Several factors influence measurement, including human error in reading measuring instruments, the accuracy of measuring instruments, environmental conditions, and data collection methods (Yuvenda et al. Citation2020). The errors are reduced by calibrating the measuring instrument and warming up the machine to the working temperature. Uncertainty analysis is calculated using equations applied in (Yuvenda et al. Citation2020). The results of the uncertainty analysis are in .
2.5 Test methodology
The engine is tested at different loads. The engine load is changed using the electric generator and a lamp as the engine loads. Halogen lamps have a capacity of 500 watts with a parallel circuit. Each load is varied by the pilot injection timing (PIT). The load percentage is obtained from the following calculations:
Engine loads are then classified into two types. The low load is 22% with the CNG injection duration is 70° CA and the high load is 89% with the CNG injection duration is 110° CA. The CNG injection duration is selected based on previous research. This involves optimising CNG substitution at each engine load by varying the injection duration (Yuvenda et al. Citation2020). The mass of CNG fuel is increased with each increase in engine load, aiming to keep the CNG fuel substitution optimal and to keep the engine power at a constant rotation (2000 rpm). The consequence is that the air mass flow rate at each additional engine load has decreased due to an increase in CNG fuel substitution. Thus the air fuel ratio (AFR) decreases. This is due to the increasing mass of CPO biodiesel and CNG fuel, while the engine speed is kept constant. The equation used to calculate the AFR value is as follows:
The testing procedure is performed and adjusted to the research parameters shown in below.
Table 4. Research parameters
The test used a diesel engine with a conventional fuel system, and the injection timing strategy still uses the manual method. The determination of the pilot injection timing (PIT) variation starts from the standard injection timing of 13° BTDC of pilot. When the PIT is advanced from 13° to 19° BTDC, it reduces the thickness of the shim lying between the injection pump and the engine body. The standard injection timing using an installed the thickness of the shim is 1.2 mm. Each reduction in the thickness by 0.1 mm changes the injection timing angle by 1° CA. The injection timing parameter settings are adjusted in . This method was also conducted by (Sayin and Canakci Citation2009).
3. Results and discussions
This sub-section discusses the effect of load on engine performance, combustion process, and emissions at varied injection timings. Moreover, the section discusses the effect of pilot injection timing (PIT) using CPO biodiesel fuel at certain loads.
3.1 Effects of the pilot injection timing (PIT) on engine performances
Parameters used to measure engine performances include power, brake thermal efficiency (BTE), and specific fuel consumption (SFC). The related definition of these parameters, load is the external force acting on engine components. In this case, the load is given to the electric generator coupled to the engine. SFC is the amount of fuel the engine uses to produce 1 kW of power for an hour. Thermal efficiency is a measure of the heat energy stored in the fuel to be converted into effective power by the internal combustion motor. The relationship between the mass of fuel, SFC, power, and BTE is seen in the following equation (Mahla et al. Citation2018):
Power
Specific fuel consumption
Brake thermal efficiency
shows the comparison of engine performance values between using CPO biodiesel and diesel fuel as pilot fuel under low engine load at standard pilot injection timing (13° BTDC). Engine power and thermal efficiency are lower when CPO biodiesel fuel is used as a pilot fuel at dual fuel engine. This is because CPO biodiesel has a low calorific value, and the energy converted to heat is lower (Raman et al. Citation2019). Also, CPO biodiesel has a high viscosity and density, resulting in large droplets forming during atomisation. This results in an inhomogeneous mixture, as explained by Shrivastava et al. (Shrivastava, Nath, and Pugazhendhi Citation2019). Therefore, the quality of the premix combustion decreases, resulting in low power and thermal efficiency. Tarabet et al. (Tarabet et al. Citation2014) stated that the reduction in BTE was caused by biodiesel fuel’s low calorific value when used as a pilot. According to Channappagoudra et al. (Channappagoudra, Ramesh, and Manavendra Citation2020) the decrease in thermal efficiency using biodiesel fuel as a pilot is due to the low calorific value, high density, and higher viscosity. However, the specific fuel consumption value using CPO biodiesel fuel as a pilot is higher than the use of diesel fuel. This is because CPO biodiesel fuel has low calorific value and high density (Zhang et al. Citation2017).
also shows effect of PIT on power, SFC, and BTE at low engine load. The values of engine power and thermal efficiency increase with PIT advancement, as shown in (Shu et al. Citation2018; Wang et al. Citation2016a; Yang et al. Citation2016; Yousefi and Birouk Citation2017; Yousefi, Birouk, and Guo Citation2017; H. Zhou et al. Citation2019). Advancing the PIT causes the mixing of biodiesel fuel with CNG-air mixture to be more homogeneous before the start of combustion (SOC) because of a longer ignition delay period (Yousefi, Birouk, and Guo Citation2017). Also, there is the formation of an ignition kernel with a greater amount and wider distribution in the combustion chamber. This results in the combustion of more CNG fuels, as evidenced by the HRR, which is increasingly symmetrical by advancing the PIT. Similarly, the decrease before PHRR2 of CNG fuel combustion becomes less visible, as shown in , resulting in high thermal power and efficiency. Zhou et al. (H. Zhou et al. Citation2019) stated that advancing the PIT caused a longer ignition delay, facilitating the fuel-air mixture’s formation before the start of combustion (SOC). This supported the formation of more ignition kernels. The maximum power and thermal efficiency values are 1.048 kW and 17.90%, respectively, at the PIT of 19° BTDC. The percentage increase in power and thermal efficiency values is 0.39% and 1.28% from the standard PIT (13° BTDC). Additionally, SFC values decrease by advancing the PIT under low engine load, as shown in (H. Zhou et al. Citation2019), due to an increase in flame speed (H. Zhou et al. Citation2019). The lowest SFC value was obtained at 0.4393 kg/kW.h, with a reduction percentage of 1.37% from the standard PIT.
shows the comparison of engine performances on a DF engine between the use of diesel and CPO biodiesel fuels under high engine load at standard pilot injection timing (13° BTDC). As with low load, at the standard injection timing (13° BTDC) the rated power and BTE are lower using CPO biodiesel as a pilot on a dual fuel engine. With the same reason, that the calorific value of diesel fuel is higher than CPO biodiesel fuel so that it results in complete combustion. Likewise with a higher SFC value using CPO biodiesel as a pilot. This is due to the increased consumption of the engine to maintain engine performance due to its low calorific value.
When compared with low load, the rated power and BTE are higher under high load. This phenomenon is caused by an increase in pilot fuel to maintain engine performance. As a result, the combustion process increases, which results in high power and BTE. Raman et al. (Raman et al. Citation2019) stated that increasing engine load would increase the power and mass rate of the fuel. Moreover, the addition of engine load increases the combustion temperature in the premix. As a result, the combustion rate of CNG fuel is faster because CNG fuel has a higher flame speed and produces maximum combustion.
also shows the effect of PIT on power, SFC, and BTE at high engine load. The engine power and thermal efficiency values increase by advancing the PIT (Bora and Saha Citation2016; Channappagoudra, Ramesh, and Manavendra Citation2020; Hosmath et al. Citation2016; Sudarmanta, Bambang, Setiyawan, Atok et al., Citation2019). This is due to an increase in the combustion rate from the premix of CPO biodiesel fuel. Although CNG fuel has a low speed and CPO biodiesel has a high viscosity value, advancing the PIT is the right solution. This is because the atomisation and mixing time is longer, making the fuel-air mixture more homogeneous (Hosmath et al. Citation2016). Yousefi et al. (Yousefi, Guo, and Birouk Citation2019) stated that advancing DIT led to an additional ignition delay. Consequently, a more mixture of diesel fuel and gas-air was formed before the TDC’s initial ignition and combustion phase. The high local cylinder gas temperature increased combustion efficiency. The maximum power and thermal efficiency values were 4.242 kW and 39.56% at the PIT of 17 °BTDC. The percentage increase in power value and BTE were 0.33% and 8.87% of the standard PIT (13° BTDC). Also, the SFC value decreased by advancing the PIT under high engine load. This is because the preparation time for combustion is longer before SOC, increasing the ignition delay. Hence, the premix combustion of CPO biodiesel fuel is more maximum, resulting in more effective fuel consumption at the same engine load. The lowest SFC value was obtained at 0.2005 kg/kW.h, with a reduction percentage of 9.43% from the standard PIT.
shows the effect of PIT on energy per cycle at different loads. The equation used to obtain the energy value per cycle is:
Advancing the PIT results in an increase in energy per cycle, though not significant at all loads. The findings prove that PIT advancement results in longer injection duration of CPO biodiesel fuel. This is because it is injected at a lower pressure and temperature during the compression stroke. Therefore, the amount of CPO biodiesel fuel put into the cylinder increases, raising the energy per cycle. shows that increasing the engine load increases the energy value per cycle in a dual-fuel engine. This is due to the addition of CPO biodiesel and CNG fuel to compensate for the additional load to maintain engine performance.
3.2 Effects of the pilot injection timing (PIT) on combustion performance
3.2.1 Pressure and heat release rate
The heat release rate is calculated from the start of combustion, marked with a positive value, to the end with a zero. However, there is a positive value on the heat release rate before combustion. This is because the residual combustion trapped in the cylinder releases heat energy captured by the piezoelectric pressure sensor and given to processing data. Similarly, this phenomenon is seen in (Gharehghani, Hosseini, Mirsalim, & Jazayeri, Citation2015; Yang et al. Citation2014). Theoretically, the calculation of HRR uses the following equation (Heywood Citation1988):
Where, γ is the specific heat ratio, . P is the cylinder pressure and V is the volume of the cylinder.
shows that the cylinder pressure using CPO biodiesel fuel as pilot is slightly higher than use diesel fuel under low load. The findings are in line with (Ryu Citation2013a; Tarabet et al. Citation2014). This is due to the initial combustion of CPO biodiesel fuel. The premix combustion of CPO biodiesel fuel takes place before the end of the compression step due to a higher cetane number (58) and a rich oxygen content (12.7%). Tarabet et al. (Tarabet et al. Citation2014) stated that increasing cylinder pressure using biodiesel as pilot fuel results in rapid premix combustion due to high cetane numbers and oxygen content. However, different conditions are indicated by the heat release rate with decreased biodiesel fuel use as a pilot. This is because CPO biodiesel fuel has a lower calorific value. Therefore, at a lower temperature, less fuel is converted to heat. Moreover, with a higher cetane number than biodiesel fuel, the ignition delay period is shorter than using diesel fuel as a pilot. The time for mixing CPO biodiesel fuel with air during the physical delay process is shorter. Also, the number of ignition points is less and not widely distributed throughout the combustion chamber, hence the premix combustion process is decreased, and less CNG fuel is burnt. Tarabet et al. (Tarabet et al. Citation2014) stated that the heat release rate is reduced when using biodiesel as a pilot because combustion fuel decreases. The contribution of natural gas is insignificant to the heat release rate. The second combustion stage from the end of the premix runs slowly due to the weak contribution of natural gas fuels.
At high load (), the pressure and heat release rate was significantly increased using CPO biodiesel as a pilot compared to diesel fuel. The result was in line with Tarabet et al.(Tarabet et al. Citation2014) and Ghareghani et al. (Gharehghani et al. Citation2015). This is due to an increase in temperature at high load, supported by the high cetane number and oxygen content in the CPO biodiesel molecule. The premix combustion rate increases and more CNG fuel is burnt. The heat released from the two fuels is higher. Tarabet et al. (Tarabet et al. Citation2014) stated that the increase in cylinder pressure and heat release rate when using biodiesel as a pilot is due to the shorter ignition delay. This is caused by the high cetane numbers, rich oxygen content, and lower stoichiometric air requirements. Another reason is the participation of natural gas fuel in the diffusion combustion stage.
Increased cylinder pressure and heat release rate by using CPO biodiesel fuel as a pilot due to the influence of high unsaturated fatty acids. CPO biodiesel fuel has a fairly high percentage of unsaturated fatty acids such oleic (18: 1) of (37%) and linoleic (18: 2) of (9.07%) as shown in . The increase in unsaturated fatty acids causes an increase in the cetane number so that the ignition delay becomes shorter and the start of combustion becomes faster so that the combustion pressure and heat release rate increase as the peak combustion approaches TDC, this phenomenon is also explained by Pinzi et al. (Pinzi et al. Citation2013). In addition, with saturated fatty acids such as palmatic (C16: 0) which is high (47.9%,) it causes a faster start of ignition so that the fuel burns more at the premix stage and results in an increase in cylinder pressure.
also shows the effect of PIT on cylinder pressure and the HRR under low engine load. Cylinder pressure and HRR rate increased significantly by advancing PIT (Shu et al. Citation2018; Wang et al. Citation2016a; Yang et al. Citation2016; Yousefi and Birouk Citation2017; Yousefi, Birouk, and Guo Citation2017). Advancing the PIT results in higher and peak cylinder pressure leading to TDC. This increase is caused by the high fuel energy released at the compression step due to CPO biodiesel fuel injection.
Advancing of pilot injection timing means introducing CPO biodiesel fuel early into the combustion chamber while the compression stroke is still in place. Where this condition, the pressure and temperature when compression is still low which is termed the low temperature combustion (LTC) model. As a result, the ignition delay is longer so that the formation of a more homogeneous mixture between CPO biodiesel fuel and the air-CNG mixture (Wang et al. Citation2016b; Yousefi and Birouk Citation2017; Yousefi, Birouk, and Guo Citation2017). This will result in a more and more even ignition source throughout the combustion chamber so that the premix combustion rate increases and burns more of the CNG-air mixture as the piston moves towards TDC. Yang et al. (Yang et al. Citation2016) explained that the combustion process in a dual fuel diesel engine is triggered by pilot fuel so that the combustion phase moves towards the upper dead centre (TDC) of the cylinder so that the heat released in the compression step increases. Thus there is a significant increase in cylinder pressure and temperature. Zhou et al. (H. Zhou et al. Citation2019) explained that advancing the pilot injection time causes the ignition delay to be longer and the combustion phase to be delayed so that it helps the formation of more fuel and air mixtures which can support the formation of more ignition kernel thereby increasing cylinder pressure which affects the increase in engine power.
Similarly, the HRR increases significantly with advancing the PIT. This increase was caused by the homogeneous mixture of CPO biodiesel fuel, CNG, and air because of a longer time in the physical delay process. As a result, more ignition was produced and distributed in the wider combustion chamber. The premix combustion of CPO biodiesel and CNG fuels significantly increased due to the initial ignition increase. Consequently, the heat release rate was increased. According to Yang et al. (Yang et al. Citation2016), HRR was increased due to an increase in the proportion of premix combustion from diesel and CNG fuels, which is attributed to a more homogeneous mixture with a longer ignition. The cylinder pressure values and the maximum HRR were 52.64 bar and 59.27 kJ/m3.°CA at the PIT of 19° BTDC. The percentage increase in the value of cylinder pressure and HRR was 23.76% and 23.80%, respectively, from the standard PIT (13° BTDC).
shows the effect of PIT on cylinder pressure and HRR under high engine load. Graphs of cylinder pressure and HRR are more symmetrical and form a peak. The graphs show that the premix combustion rate of CPO biodiesel and CNG fuels increases significantly at high loads. This is due to the addition of CPO biodiesel fuel, which produces more ignition kernels widely spread in the combustion chamber. The cylinder pressure and HRR increased significantly with PIT advancement (Guerry et al. Citation2016; Hosmath et al. Citation2016; Papagiannakis et al. Citation2017; Ryu Citation2013b; Sudarmanta et al. Citation2019a). Advancing of the PIT means introducing CPO biodiesel fuel early into the chamber which occurs during the compression step. As explained at low load, this increase is due to the amount of energy converted from CPO biodiesel fuel during the compression step because the fuel is injected earlier, so that the mixing time of CPO biodiesel fuel with the air-gas mixture is longer due to ignition delays. The consequence of this is the formation of a more homogeneous mixture between CPO biodiesel and the air-gas mixture and the mixture is widely distributed and more evenly in the cylinder. Then when the mixture reaches the flammability limit condition it will produce a larger premix combustion and is compressed by the piston which is still moving towards TDC and this will result in a significant increase in cylinder pressure and temperature at high loads. In addition, at high load conditions, CPO biodiesel fuel consumption increases to compensate for engine power so that there are more sources of ignition in the cylinder. Channappagoudra et al. (Channappagoudra, Ramesh, and Manavendra Citation2020) explained that advancing the injection time led to a longer ignition delay, leading to increased atomisation of the pilot’s fuel spray, increased turbulence intensity and increased heat transfer to the unburned air-fuel mixture.
The increase in cylinder pressure and temperature has an impact on the increase in the heat release rate, as seen in that the rate of heat release increases with advancing the PIT. This requires that when the fuel burns in large quantities due to the high combustion rate, the fuel energy that is converted to heat is also greater. However, the maximum cylinder pressure and heat release rate are at different PIT. The maximum cylinder pressure value of 89.78 bar at the PIT is 19° BTDC, while the maximum HRR is 144.51 kJ/m3.CA at the PIT of 15° BTDC. The percentage increase in the value of cylinder pressure and HRR is 21.46% and 7.34% of the standard PIT (13° BTDC). This is because the combustion temperature is too high so that there is more combustion occurring earlier than CNG fuel so that the heat release rate decreases until the cylinder pressure is too high (Guerry et al. Citation2016).
3.2.2 Ignition delay and combustion duration
shows the comparison of ignition delay and combustion duration using CPO biodiesel fuel with diesel as a pilot. The use of CPO biodiesel as a pilot causes a decrease in ignition delay in all engine loads. This is because CPO biodiesel fuel has a high cetane number (58) and a high oxygen content (12.7%). Therefore, the initiation of combustion is faster than diesel fuel. In line with this, Tarabet et al. (Tarabet et al. Citation2014) stated that ignition delay reduction is due to biodiesel’s higher cetane number and oxygen content.
Combustion duration refers to the time from the start to the end of combustion. According Gharehghani et al. (Gharehghani et al. Citation2015), combustion duration is the rotation period of the crank angle between the start of combustion (SOC) and the end of combustion (EOC). Similarly, Reyes et al. (Reyes and Quiros Citation2017) stated that combustion duration is the difference between EOC and SOC, in the degree of crank angle.
shows that combustion duration is longer when using CPO biodiesel fuel as a pilot at a low load. This is due to the decrease in temperature, which leads to a slower fire rate and longer combustion time. However, combustion duration is decreased at medium and high loads due to increased temperature and heat release rate. The higher cetane number in the CPO biodiesel contributes to a shorter ignition delay, while the richer oxygen content oxidises more fuel.
shows the effect of PIT on ignition delay and combustion duration under different engine loads. The ignition delay value increases by advancing the PIT at all engine loads (Papagiannakis et al. Citation2017; Ryu Citation2013b; Shu et al. Citation2018; Wang et al. Citation2016a; Yousefi, Birouk, and Guo Citation2017; L. Zhou et al. Citation2013). This is because pilot fuel injection is decreased at lower pressure and compression temperatures, which increased the ignition time (Papagiannakis et al. Citation2017; Yousefi, Birouk, and Guo Citation2017). The ignition delay value decreases with increasing engine loads (Bora and Saha Citation2016; Ryu Citation2013b; Sudarmanta et al., Citation2019). This is due to the increased charge temperature caused by an increase in cylinder pressure and HRR (Ryu Citation2013b). Ryu, (Ryu Citation2013b) stated that the decrease in ignition delay was due to an increase in cylinder temperature, which was caused by an increase in engine loads.
The combustion duration value decreases by advancing the PIT at all engine loads (García Valladolid et al. Citation2017; Papagiannakis et al. Citation2017; Sudarmanta et al. Citation2019b; Wang et al. Citation2016a; Yang et al. Citation2015; L. Zhou et al. Citation2013). This is because the flame develops faster due to increased premix combustion (García Valladolid et al. Citation2017; Papagiannakis et al. Citation2017). The minimum combustion duration value was obtained at the PIT of 19° BTDC under low and medium loads, while at high load, it was obtained at the PIT of 15° BTDC. Increased premixed combustion influences the increase in temperature. In consequence, the flame speed is increased, which reduces the time needed to complete the combustion. The graph in shows that the highest heat release peak is obtained in the premix combustion phase with advancing PIT. The premix combustion stage has a higher flame speed than the diffusion stage. Therefore, the flame propagation speed increases, which reduces the combustion duration by advancing the PIT.
The combustion duration increases at medium engine load due to the addition of CNG fuel, which leads to an insignificant temperature rise. Most of the heat is used to prepare CNG fuel combustion, causing a slow flame rate. The combustion duration significantly decreases when the engine load is increased. This is due to a significant rise in temperature, caused by increased premix combustion of CPO biodiesel fuel. The increased combustion is caused by pilot fuel’s addition to offset the engine loads, which raises the charge temperature. Additionally, the extension delay time increases by advancing the PIT. This facilitates the formation of a homogeneous mixture of CPO biodiesel fuel, CNG, and air. Moreover, more ignition sources are produced and evenly spread in the combustion chamber, resulting in maximum premix combustion at high engine load.
3.3 Effects of the pilot injection timing (PIT) on emissions
shows that the concentration of CO, HC, and PM is lower using CPO biodiesel fuel as a pilot fuel at standard pilot injection timing (13° BTDC) under low load. The results are in line with (Gharehghani et al. Citation2015; Tarabet et al. Citation2014). This is because CPO biodiesel fuel has a high oxygen content, which improves the combustion process (Tarabet et al. Citation2014). Raman et al. (Raman et al. Citation2019) stated that the rich oxygen in the CPO biodiesel increases the combustion process beyond stoichiometry. Hence, the CO emissions are reduced due to the combustion of lean mixtures. According to Tarabet et al. (Tarabet et al. Citation2014), HC emissions are reduced because biodiesel fuel has an oxygen content of around 11%, leading to a better ignition source. Also, the higher cetane number shortens the ignition delay period, decreasing the fuel-rich zone during combustion. The CPO biodiesel fuel lacks aromatic content and is low in sulphur, reducing the potential for forming particulate emissions.
In addition, the high content of fatty acids such as palmitic (C16:0) and oleic (C18:1) in CPO biodiesel fuel causes a reduction in HC and CO emissions, it is also explained in the previous literature. PM emission decreased significantly with palmitate (C16:0) and oleic (C18:1) content in biodiesel fuel, as explained by Knothe et al. (Knothe, Cermak, and Evangelista Citation2009).
also shows the effect of PIT on CO, HC, and PM emissions at low engine load. The CO emission is influenced by cylinder temperatures, which control the oxidation and decomposition of the fuel. The HC emission is produced from incomplete or slow combustion (Yang et al. Citation2016). More PM emissions are produced from the extended paraffin family. The CO, HC, and PM emissions decreased significantly with advancing the PIT (Sudarmanta et al. Citation2019b; Wang et al. Citation2016a; Yang et al. Citation2016; Yousefi and Birouk Citation2017; Yousefi, Birouk, and Guo Citation2017). This is due to complete combustion and increased temperature from the high HRR. The heat release rate was increased by mixing CPO biodiesel fuel and a more homogeneous CNG-air mixture. This was facilitated by the longer mixing duration in the ignition by advancing the PIT.
According to Yousefi et al. (Yousefi, Birouk, and Guo Citation2017), the HC emissions were reduced due to high combustion rates and the amount of CNG that burned on premix when diesel fuel was injected earlier. The premix ignition from the air-CPO biodiesel fuel mixture generates a wider and more uniform flame source, increasing the combustion rate than the CNG-air fuel mixture. This causes the flame to propagate faster and spread evenly throughout the chamber. Furthermore, the CO emission significantly decreased by advancing the PIT because more CNG fuels mixed early before ignition. The combustion was faster and complex due to increased temperatures, causing the fuel to decompose easily and be oxidised to form CO2. Bora and Saha (Bora and Saha Citation2016) explained that the reduction in CO emissions was due to the increase in the oxidation mechanism of CO which was caused by an increase in the reaction time interval during the expansion step with the temperature still high in the cylinder, and the decrease in HC emissions because the initial combustion occurred so that the combustion chamber temperature was higher because the air-biogas mixture is compressed by the piston towards TDC. The reduction in CO and HC emissions by advancing the pilot injection timing, due to the relatively high engine temperature which makes the oxidation rate easier (Guerry et al. Citation2016; H. Zhou et al. Citation2019). Also, PM concentrations were influenced by HC molecules. A reduction in HC emissions inhibits the process of particle formation. Therefore, advancing the PIT causes a reduction in PM emissions (Ryu Citation2013b; Yang et al. Citation2016). The reduction in PM emission by advancing the pilot injection time is also due to the increase in cylinder charge temperature so that the rate of the oxidation mechanism of the soot is high (Papagiannakis et al. Citation2017).
The minimum CO, HC, and PM emission values were obtained at 0.146%, 286 ppm, and 2.6%, respectively, at the PIT of 19 °BTDC. The percentage reduction in CO, HC, and PM emission concentrations was 26.26%, 19.43%, and 31.57%, respectively, from the standard PIT (13 °BTDC).
Based on fatty acid composition, CPO biodiesel fuel has a relatively high percentage of oleic (37%). Oleic is an unsaturated acid with at least one unsaturated bond between the constituent carbon atoms. The double bonds in unsaturated acids readily react with oxygen (easily oxidised). This means that more C and H molecules react with O, allowing high amounts of biodiesel fuel to burn. This leads to maximum premix combustion. Since CPO biodiesel acts as a lighter, the combustion quality of premix and diffusion fuels is affected by the ignition power and points. This has been proven by reducing HC and CO emissions, as well as particulate matter, using CPO biodiesel as a pilot.
shows that the concentration of CO, HC, and PM is lower using CPO biodiesel fuel as a pilot fuel at standard pilot injection timing (13° BTDC) under high load. High loads have lower concentration values of emission (CO, HC, and PM) when compared to low and medium loads at standard injection conditions, which is in line with previous studies (Banapurmath et al. Citation2018; Sudarmanta et al. Citation2019b; Yuvenda et al. Citation2020). This is due to the increase in the amount of CPO biodiesel fuel. As a result, the ignition points and power were increased, as explained by (Yuvenda et al. Citation2020). Furthermore, CPO biodiesel fuel has a high oxygen content. Therefore, it overcomes the oxygen shortage in diesel DF engine, which until now is a problem with the presence of CNG fuel. The high oxygen content of CPO biodiesel fuel improves the quality of the air fuel ratio (AFR). Consequently, combustion becomes complete, resulting in reduced HC emissions. Moreover, high oxygen from the CPO biodiesel in the cylinder reduces CO emissions. This is because the CO emissions are mainly caused by insufficient oxygen in the air-fuel mixture.
also shows the effect of PIT on CO, HC, and PM emissions at high engine load. The CO, HC, and PM emissions decreased significantly with advancing the PIT (Guerry et al. Citation2016; Papagiannakis et al. Citation2017; Sudarmanta et al. Citation2019b). The oxidation rate of CO, HC, and PM molecules is affected by combustion temperature. According to Guerry et al. (Guerry et al. Citation2016), advancing the PIT positively affects the increase in temperatures. This is because the mixture of CPO biodiesel fuel and air-CNG mixture are more homogeneous due to a longer ignition process. Consequently, a greater ignition source is produced and distributed in the chambers, significantly increasing the air-CNG mixture’s premix combustion rate. The increases in temperature is due to an earlier ignition when the piston moves towards TDC, raising the cylinder pressure and HRR, as shown in . High temperatures produce more OH concentrations (Yousefi and Birouk Citation2017), which readily react with CO molecules to form CO2 + H molecules. Additionally, high temperatures produce faster flame velocity, which develops and reaches the zones far from ignition sources, including cylinder walls and piston crevice, burning more air-CNG mixes.
In addition, by advancing the injection time, there is an increase in the spray atomisation of the pilot fuel, the turbulence intensity and heat transfer of the cylinder to the unburned air-fuel mixture and the homogeneity of the air-fuel mixture, thereby increasing the combustion of the premix and resulting in reduced HC emissions. Likewise, there is a decrease in CO emissions because advancing the pilot injection timing provides a longer mixing time between air and fuel when the oxidation process with high cylinder temperatures results in complete combustion (Channappagoudra, Ramesh, and Manavendra Citation2020). Advancing the pilot injection timing also affects the reduction of PM emissions. This is contributed by an increase in charge temperature due to an increase in the rate of heat dissipation as the pilot injection timing is advanced thereby increasing the oxidation rate of the particulate (Papagiannakis et al. Citation2017).
The minimum CO, HC, and PM emission values were obtained at 0.032%, 56 ppm, and 5.4% at the PIT of 15° BTDC. The percentage reduction in CO, HC, and PM emission concentrations was 43.85%, 25.33%, and 25%, respectively, from the standard PIT (13° BTDC).
3.4 Statistical analysis
3.4.1 Regression analysis
The effect of the effect of pilot injection timing parameter on power, SFC, BTE, CO emissions, HC emissions, and PM emissions were also statistically analysed using linear regression equations, namely:
where, Y is the response variable, a is a constant, b is the regression coefficient and X is the factor variable.
The regression test results of engine performance at low and high loads in knowing the effect of the pilot injection timing parameters on the DF engine as described in below:
Table 5. Regression analysis of engine performance at low load
Table 6. Regression analysis of engine performance at high load
From the results of the regression analysis, it shows that the p-value of power, SFC and BTE is smaller than the significance value of 0.05, so it can be concluded that the pilot injection timing has an effect on power, SFC and BTE at low loads. Likewise, at high loads, it can be seen that the P-value of SFC and BTE is smaller than the significance value of 0.05, so it is concluded that the pilot injection timing has an effect on SFC and BTE at high loads, but on power there is no significant effect.
The regression test results of emissions at low and high loads in knowing the effect of the pilot injection timing parameters on the DF engine as described in below:
Table 7. Regression analysis of emissions at low load
Table 8. Regression analysis of emissions at high load
From the results of the regression analysis, it shows that the p-value of CO, HC and PM emissions is smaller than the significance value of 0.05, so it is concluded that the pilot injection timing has an effect on CO, HC and PM emissions at low loads. However, at high loads, it can be seen that the P-value of CO, HC and PM emissions is greater than the significance value of 0.05, so it is concluded that the pilot injection timing has no effect on CO, HC and PM emissions at high loads. This is also seen in the , where with the variation in injection time there is no significant difference.
3.5 Analyse the cost of fuel consumption
This discussion explains the economic analysis between a standard diesel engine (single fuel) and a dual fuel engine fuelled by CNG and CPO biodiesel.
1. Economic analysis on a single fuel diesel engine at low load.
It is known:
The mass flow rate of diesel fuel = 0.0001122 kg/s = 0.4696 litres/hour
1 litre of diesel fuel = IDR 9,400.00
Diesel fuel consumption = 0.4696 litres/hour
= 0.4696 liters/hour x IDR 9,400.00
= IDR 4,414.94/hour
So the cost needed for fuel consumption from a diesel engine per hour is IDR 2,203.53/hour
2. Economic analysis on a dual fuel engine at low load.
It ss known:
Mass flow rate of CPO biodiesel fuel = 0.000056 kg/s = 0.234419 litres/hour
CNG fuel mass flow rate = 0.000073 kg/s = 0.00205 m3/hour
1 litre of CPO biodiesel = IDR 10,229.00
1 litre of CNG = IDR 4,500.00
a. CPO biodiesel fuel Consumption = 0.234419 litres/hour
= 0.234419 liters/hour x IDR 10,229.00
= IDR 2,356.76/hour
b. CNG fuel consumption = 0.00205 m3/hour
= 0.00205 m3/hour x 1.032 liters
= 0.002115 liter/hour
= 0.002115 litre/hour x IDR 4,500.00/litre
= IDR 9.52/hour
Total fuel consumption costs = CPO biodiesel + CNG consumption costs:
= IDR 2,356.76/hour + IDR 9.52/hour
= IDR 2366.28/hour
Thus the difference in the cost of fuel consumption in hours between a standard diesel engine and a dual fuel engine is as follows:
Difference in the cost of fuel consumption per hour = Standard diesel engine – Dual fuel engine
= IDR 4,414.94/hour- IDR 2366.28/hour
= IDR 2,048.66/hour
Based on the economic analysis described above, this proves that the dual fuel engine fuelled by CPO biodiesel and CNG has an economic value with a cost savings of IDR 2,048.66/hour, when compared to a standard diesel engine.
4. Conclusion
This study examined the effects of pilot injection timing (PIT) using CPO biodiesel fuel on the dual-fuel engine’s combustion and emission characteristic with CNG as the main fuel. The following conclusions were made.
CPO biodiesel fuel is a renewable fuel that can be used in dual fuel (DF) engine as pilot fuel, however, injection parameter settings are required to improve engine performances and emissions.
The use of CPO biodiesel fuel as a pilot accelerates the ignition delay period and the initial combustion. Also, the general combustion is maximum because CPO biodiesel fuel has a higher cetane number of around (58). The high oxygen content (12.7%) in CPO biodiesel improves the air-fuel ratio and contributes to reducing CO and HC emissions. The high content of palmitic of saturated fatty acid (47.9%) in CPO biodiesel fuel accelerate the initial combustion. The high content of oleic of unsaturated fatty acid (37%) in CPO biodiesel fuel accelerates the fuel’s oxidation. The CPO Biodiesel fuel is free from aromatics and low in sulphur content. Therefore, the potential for particulate formation is lower.
Advancing the pilot injection timing of 19° BTDC on the DF engine at low load there was an increase in brake thermal efficiency by 1.28%, an increase in cylinder pressure by 23.76%, and an increase in HRR by 23.80% while the SFC decreased by 1.37%. Likewise, there was a decrease in CO emissions by 26.26%, and a decrease in HC emissions by 19.43%.
Advancing the pilot injection timing of 15° BTDC on the DF engine at high load there was an increase in brake thermal efficiency by 8.87%, an increase in cylinder pressure by 21.46%, and an increase in HRR by 7.34% while the SFC decreased by 9, 43%. Likewise, there was a decrease in CO emissions by 43.85%, and a decrease in HC emissions by 25.33%.
The pilot injection time affects engine performance and emissions on a dual fuel engine which is strengthened by statistical analysis using regression equations, especially at low loads.
The recommendation for further research is that it is necessary to visualise the combustion process in the combustion chamber using simulation software to see the combustion characteristics using CPO biodiesel fuel as a pilot.
Conflicts of interest
No potential conflict of interest was reported by the author(s).
Nomencalture
CNG Compressed natural gas
CH4Methane
BTE Brake thermal efficiency
CPOCrude palm oil
BTDCBefore top dead center
ATDCAfter top dead center
CACrank angle
PITPilot injection timing
SFCSpecific fuel consumption
COCarbon monoxide
CO2Carbon dioxide
NOxNitrogen oxide
HCHydrocarbon
UCH4Unburnt methane
PMParticulate matter
PHRR1The first peak of heat release rate
PHRR2The second peak of heat release rate
NEDCNew European driving cycles
SOCStart of combustion
EOCEnd of combustion
CDCombustion duration
IDIgnition delay
Acknowledgments
This research was sponsored by the Ministry of Education, Culture, Research and Technology Indonesia, who has financially supported this research. A great appreciation for DDF ITS team who had given their greatest effort in the completion of this research.
Additional information
Funding
Notes on contributors
Bambang Sudarmanta
Bambang Sudarmanta is a lecturer at the Department of Mechanical Engineering, Institut Teknologi Sepuluh Nopember, Surabaya, Indonesia. The research fields currently being carried out are diesel dual fuel, fuel and combustion technology, bioenergy, and gasification.
Dori Yuvenda
Dori Yuvenda is a lecturer at the Department of Automotive Engineering, Universitas Negeri Padang, Indonesia. The research fields currently being carried out are diesel dual fuel, energy conversion, internal combustion engine, and biofuel.
Ary Bachtiar K.P.
Ary Bachtiar Krishna Putrais a lecturer at the Department of Mechanical Engineering, Institut Teknologi Sepuluh Nopember, Surabaya, Indonesia. The research fields currently being carried out are heat transfer, thermodynamics, and energy conversion.
Arif Wahjudi
Arif Wahjudi is a lecturer at the Department of Mechanical Engineering, Institut Teknologi Sepuluh Nopember, Surabaya, Indonesia. The research fields currently being carried out are energy conversion, artificial neural network, and genetic algorithm.
Ahmad Arbi Trihatmojo
Ahmad Arb Trihatmojo is a graduate student at the Department of Mechanical Engineering, Institut Teknologi Sepuluh Nopember, Surabaya, Indonesia. The research fields currently being carried out are combustion and energy system.
References
- Abdelaal, M. M., and A. H. Hegab. 2012. “Combustion and Emission Characteristics of a Natural Gas-fueled Diesel Engine with EGR.” Energy Conversion and Management 64: 301–312. doi:https://doi.org/10.1016/j.enconman.2012.05.021.
- Agency, I. E. 2020. World Energy Outlook 2020. Paris, France: International Energy Agency. doi:https://doi.org/10.1787/557a761b-en.
- Ayetor, G. K., A. K. Sunnu, and M. A. Kesse. 2019. “Engine Performance and Emissions of Fuel Produced from Palm Kernel Oil.” Biofuels 1–7. doi:https://doi.org/10.1080/17597269.2019.1672006.
- Banapurmath, N. R., Basavarajappa, Y. H., and P. G. Tewari. 2012. Effect of mixing chamber venturi, injection timing, compression ratio and EGR on the performance of dual-fuel engine operated with HOME and CNG. International Journal of Sustainable Engineering 5(3): 265–279. doi:https://doi.org/10.1080/19397038.2011
- Banapurmath, N. R., P. G. Tewari, Y. H. Basavarajappa, N. R. Banapurmath, P. G. Tewari, and Y. H. Basavarajappa. 2011. “International Journal of Sustainable Engineering Effect of Mixing Chamber Venturi, Injection Timing, Compression Ratio and EGR on the Performance of Dual- Fuel Engine Operated with HOME and CNG.” Cell and Tissue Banking 12 (1): 37–41. April 2015. doihttps://doi.org/10.1080/19397038.2011.603847.
- Banapurmath, N. R., V. S. Yaliwal, R. S. Hosmath, M. R. Indudhar, S. Guluwadi, and S. Bidari. 2018. “Dual Fuel Engines Fueled with Three Gaseous and Biodiesel Fuel Combinations.” Biofuels 9 (1): 75–87. doi:https://doi.org/10.1080/17597269.2016.1257316.
- Bari, S., and S. Bari. 2019. “ScienceDirect ScienceDirect ScienceDirect Performance and Emission Analysis of a Diesel Engine Running on Performance and Emission Analysis of a Diesel Engine Running on Oil Diesel (POD) Palm Oil Diesel (POD) Assessing the Feasibility the Heat Demand-outdoor and Using Temperature Function for a Long-term District Heat Demand Forecast.” Energy Procedia 160 (2018): 92–99. doi:https://doi.org/10.1016/j.egypro.2019.02.123.
- Bora, B. J., and U. K. Saha. 2016. “Optimisation of Injection Timing and Compression Ratio of a Raw Biogas Powered Dual Fuel Diesel Engine.” Applied Thermal Engineering 92: 111–121. doi:https://doi.org/10.1016/j.applthermaleng.2015.08.111.
- Çelebi, K., E. Uludamar, and E. Tosun (2017). “Experimental and Artificial Neural Network Approach of Noise and Vibration Characteristic of an Unmodified Diesel Engine Fuelled with Conventional Diesel, and Biodiesel Blends with Natural Gas Addition.” 197, 159–173. doi: https://doi.org/10.1016/j.fuel.2017.01.113
- Channappagoudra, M., K. Ramesh, and G. Manavendra. 2020. “Effect of Injection Timing on Modi Fi Ed Direct Injection Diesel Engine Performance Operated with Dairy Scum Biodiesel and Bio-CNG.” Renewable Energy 147: 1019–1032. doi:https://doi.org/10.1016/j.renene.2019.09.070.
- Di Blasio, G., C. Beatrice, V. Fraioli, M. Migliaccio, and I. M. Cnr (2015). “Experimental Evaluation of Compression Ratio Influence on the Performance of a Dual-Fuel Methane-Diesel Light-Duty Engine University of Parthenope Giacomo Belgiorno.” doi: https://doi.org/10.4271/2015-24-2460
- Di Blasio, G., G. Belgiorno, and C. Beatrice. 2017. “E Ff Ects on Performances, Emissions and Particle Size Distributions of a Dual Fuel (Methane-diesel) Light-duty Engine Varying the Compression Ratio.” Applied Energy 204 (July): 726–740. doi:https://doi.org/10.1016/j.apenergy.2017.07.103.
- Gad, M. S., R. El-Araby, K. A. Abed, N. N. El-Ibiari, A. K. El Morsi, and G. I. El-Diwani. 2018. “Performance and Emissions Characteristics of C.I. Engine Fueled with Palm Oil/palm Oil Methyl Ester Blended with Diesel Fuel.” Egyptian Journal of Petroleum 27 (2): 215–219. doi:https://doi.org/10.1016/j.ejpe.2017.05.009.
- García Valladolid, P., P. Tunestål, J. Monsalve-Serrano, A. García, and J. Hyvönen. 2017. “Impact of Diesel Pilot Distribution on the Ignition Process of a Dual Fuel Medium Speed Marine Engine.” Energy Conversion and Management 149: 192–205. doi:https://doi.org/10.1016/j.enconman.2017.07.023.
- Gharehghani, A., R. Hosseini, M. Mirsalim, S. A. Jazayeri, and T. Yusaf. 2015. “An Experimental Study on Reactivity Controlled Compression Ignition Engine Fueled with Biodiesel/natural Gas.” Energy 89: 558–567. doi:https://doi.org/10.1016/j.energy.2015.06.014.
- Guerry, E. S., M. S. Raihan, K. K. Srinivasan, S. R. Krishnan, and A. Sohail. 2016. “Injection Timing Effects on Partially Premixed Diesel – Methane Dual Fuel Low Temperature Combustion.” Applied Energy 162 (x): 99–113. doi:https://doi.org/10.1016/j.apenergy.2015.10.085.
- Heywood, J. B. 1988. Internal Combustion Engine Fundementals. McGrawHill Series in Mechanical Engineering. Vol. 21. 10987654. New York: McGraw-Hill, Inc
- Hosmath, R. S., N. R. Banapurmath, S. V. Khandal, V. N. Gaitonde, Y. H. Basavarajappa, and V. S. Yaliwal. 2016. “Effect of Compression Ratio, CNG Flow Rate and Injection Timing on the Performance of Dual Fuel Engine Operated on Honge Oil Methyl Ester (HOME) and Compressed Natural Gas (CNG).” Renewable Energy 93: 579–590. doi:https://doi.org/10.1016/j.renene.2016.03.010.
- Khalid, A., N. Tamaldin, M. Jaat, M. F. M. Ali, B. Manshoor, and I. Zaman. 2013. “Impacts of Biodiesel Storage Duration on Fuel Properties and Emissions.” Procedia Engineering 68: 225–230. doi:https://doi.org/10.1016/j.proeng.2013.12.172.
- Knothe, G., S. C. Cermak, and R. L. Evangelista. 2009. “Cuphea Oil as Source of Biodiesel with Improved Fuel Properties Caused by High Content of Methyl Decanoate †.” Energy and Fuels 23 (3): 1743–1747.
- Königsson, F. 2012. Advancing the Limits of Dual Fuel Combustion. Stockholm city: Royal Institute of Technology.
- Liu, Y., L. Sun, B. Yang, L. Zhou, K. Zeng, and Z. Huang. 2014. “Effect of the Pilot Quantity on the Ultrafine Particle Emissions and the Combustion Characteristics of a Dual-fuel Engine.” Proceedings of the Institution of Mechanical Engineers, Part D: Journal of Automobile Engineering1–10. doi:https://doi.org/10.1177/0954407014552537.
- Mahla, S. K., A. Dhir, K. J. S. Gill, H. Muk, H. Chang, and B. Singh (2018). “In Fl Uence of EGR on the Simultaneous Reduction of NO X -smoke Emissions Trade-off under CNG-biodiesel Dual Fuel Engine.” 152 ( x). doi: https://doi.org/10.1016/j.energy.2018.03.072
- Meisami, F., H. Ajam, and M. Tabasizadeh. 2017. “Thermo-economic Analysis of Diesel Engine Fueled with Blended Levels of Waste Cooking Oil Biodiesel in Diesel Fuel.” Biofuels7269 February. doi: https://doi.org/10.1080/17597269.2017.1284475
- Mofijur, M., M. Rasul, N. M. S. Hassan, and M. Udin. 2019. “Investigation of Exhaust Emissions from a Stationary Diesel Engine Investigation of Exhaust Emissions from a Stationary Diesel Engine Fuelled with Biodiesel Fuelled with Biodiesel A, Feasibility of Using Assessing the The Hassan Temperature Function Dist.” Energy Procedia 160: 791–797. doi:https://doi.org/10.1016/j.egypro.2019.02.159.
- Mousavi, S. M., R. K. Saray, K. Poorghasemi, and A. Maghbouli. 2016. “A Numerical Investigation on Combustion and Emission Characteristics of A Dual Fuel Engine at Part Load Condition.” Fuel 166: 309–319. doi:https://doi.org/10.1016/j.fuel.2015.10.052.
- Mustafi, N. N., R. R. Raine, and S. Verhelst. 2013. “Combustion and Emissions Characteristics of a Dual Fuel Engine Operated on Alternative Gaseous Fuels.” Fuel 109: 669–678. doi:https://doi.org/10.1016/j.fuel.2013.03.007.
- Nithyanandan, K., Y. Lin, R. Donahue, X. Meng, J. Zhang, and C. F. Lee. 2016. “Characterization of Soot from diesel-CNG Dual-fuel Combustion in a CI Engine.” Fuel 184 (x): 145–152. doi:https://doi.org/10.1016/j.fuel.2016.06.028.
- Papagiannakis, R. G., and D. T. Hountalas (2003). “Experimental Investigation Concerning the Effect of Natural Gas Percentage on Performance and Emissions of a DI Dual Fuel Diesel Engine.” 23, 353–365.
- Papagiannakis, R. G., and D. T. Hountalas. 2004. “Combustion and Exhaust Emission Characteristics of a Dual Fuel Compression Ignition Engine Operated with Pilot Diesel Fuel and Natural Gas.” Energy Conversion and Management 45 (18–19): 2971–2987. doi:https://doi.org/10.1016/j.enconman.2004.01.013.
- Papagiannakis, R. G., S. R. Krishnan, D. C. Rakopoulos, K. K. Srinivasan, and C. D. Rakopoulos. 2017. “A Combined Experimental and Theoretical Study of Diesel Fuel Injection Timing and Gaseous Fuel/diesel Mass Ratio Effects on the Performance and Emissions of Natural Gas-diesel HDDI Engine Operating at Various Loads.” Fuel 202: 675–687. doi:https://doi.org/10.1016/j.fuel.2017.05.012.
- Paul, A., R. Sekhar, D. Debroy, and P. Kumar. 2014. “Effect of Compressed Natural Gas Dual Fuel Operation with Diesel and Pongamia Pinnata Methyl Ester (PPME) as Pilot Fuels on Performance and Emission Characteristics of a CI (Compression Ignition) Engine.” Energy 68: 495–509. doi:https://doi.org/10.1016/j.energy.2014.03.026.
- Paul, A., R. Sekhar, D. Debroy, and P. Kumar. 2015. “An Experimental Study of the Performance, Combustion and Emission Characteristics of a CI Engine under Dual Fuel Mode Using CNG and Oxygenated Pilot Fuel Blends.” Energy 86: 560–573. doi:https://doi.org/10.1016/j.energy.2015.04.050.
- Pinzi, S., P. Rounce, J. M. Herreros, A. Tsolakis, and M. P. Dorado (2013). “The Effect of Biodiesel Fatty Acid Composition on Combustion and Diesel Engine Exhaust Emissions.” 104, 170–182. doi: https://doi.org/10.1016/j.fuel.2012.08.056
- Raman, L. A., B. Deepanraj, S. Rajakumar, and V. Sivasubramanian. 2019. “Experimental Investigation on Performance, Combustion and Emission Analysis of a Direct Injection Diesel Engine Fuelled with Rapeseed Oil Biodiesel.” Fuel246 January: 69–74. doi: https://doi.org/10.1016/j.fuel.2019.02.106
- Reyes, J. G. T., and E. N. Quiros (2017). “Determination of the Start and End of Combustion in a Direct.” 1–6.
- Ryu, K. 2013a. “Effects of Pilot Injection Pressure on the Combustion and Emissions Characteristics in a Diesel Engine Using Biodiesel – CNG Dual Fuel.” Energy Conversion and Management 76: 506–516. doi:https://doi.org/10.1016/j.enconman.2013.07.085.
- Ryu, K. 2013b. “Effects of Pilot Injection Timing on the Combustion and Emissions Characteristics in a Diesel Engine Using Biodiesel – CNG Dual Fuel.” Applied Energy111: 721–730. doi:https://doi.org/10.1016/j.apenergy.2013.05.046.
- Sahoo, B. B., N. Sahoo, and U. K. Saha. 2009. “Effect of Engine Parameters and Type of Gaseous Fuel on the Performance of Dual-fuel Gas Diesel Engines — A Critical Review.” Renewable and Sustainable Energy Reviews13 (x): 1151–1184. doi: https://doi.org/10.1016/j.rser.2008.08.003.
- Sayin, C., and M. Canakci. 2009. “Effects of Injection Timing on the Engine Performance and Exhaust Emissions of a Dual-fuel Diesel Engine.” Energy Conversion and Management 50 (1): 203–213. doi:https://doi.org/10.1016/j.enconman.2008.06.007.
- Shahabuddin, M., A. M. Liaquat, H. H. Masjuki, M. A. Kalam, and M. Mofijur. 2013. “Ignition Delay, Combustion and Emission Characteristics of Diesel Engine Fueled with Biodiesel.” Renewable and Sustainable Energy Reviews 21: 623–632. doi:https://doi.org/10.1016/j.rser.2013.01.019.
- Shrivastava, P., T. Nath, and A. Pugazhendhi. 2019. “An Experimental Evaluation of Engine Performance and Emisssion Characteristics of CI Engine Operated with Roselle and Karanja Biodiesel.” Fuel 254 (July): 115652. doi:https://doi.org/10.1016/j.fuel.2019.115652.
- Shu, J., J. Fu, J. Liu, L. Zhang, and Z. Zhao. 2018. “Experimental and Computational Study on the Effects of Injection Timing on Thermodynamics, Combustion and Emission Characteristics of a Natural Gas (Ng)-diesel Dual Fuel Engine at Low Speed and Low Load.” Energy Conversion and Management 160 (January): 426–438. doi:https://doi.org/10.1016/j.enconman.2018.01.047.
- Statistics, S. of E. C. 2019. Indonesian Oil Palm Statistic9. Vol. 2019. Jakarta: Directorate General of Estate Crops.
- Sudarmanta, B., A. Setiyawan, A. B. K. Putra, D. Yuvenda, and J. Silva. 2019a. “Optimization of Pilot Diesel Injection Timing on Load Variation Dual Fuel Diesel-CNG Engine on Combustions and Emissions Characteristics.” International Review of Mechanical Engineering 13(1): 58–69. 13 January.
- Sudarmanta, B., A. Setiyawan, P. Ary, K. Bachtiar, and D. Yuvenda. 2019b. “Optimization of Pilot Diesel Injection Timing on Load Variation Dual Fuel Diesel-CNG Engine on Combustions and Emissions Characteristics.” Intenational Review of Mechanical Engineering 13 (1): 1–12.
- Tarabet, L., K. Loubar, M. S. Lounici, K. Khiari, T. Belmrabet, and M. Tazerout. 2014. “Experimental Investigation of DI Diesel Engine Operating with Eucalyptus Biodiesel/natural Gas under Dual Fuel Mode.” FUEL 133: 129–138. doi:https://doi.org/10.1016/j.fuel.2014.05.008.
- Trihatmojo, A. A., D. Yuvenda, and B. Sudarmanta (2019). “The Effects of Pilot Injection Timing on the Combustion Process and Exhaust Emissions in Dual-fuel Diesel Engine Using Biodiesel- CNG at High Load the Effects of Pilot Injection Timing on the Combustion Process and Exhaust Emissions in Dual-Fuel Diesel Engine Using Biodiesel-CNG at High Load.” 020031(December).
- Wang, Z., W. Chen, D. Wang, M. Tan, Z. Liu, and H. Dou. 2016a. “A Novel Combustion Evaluation Method Based on In-cylinder Pressure Traces for Diesel/natural Gas Dual Fuel Engines.” Energy 115: 1130–1137. doi:https://doi.org/10.1016/j.energy.2016.09.030.
- Wang, Z., Z. Zhao, D. Wang, M. Tan, Y. Han, Z. Liu, and H. Dou. 2016b. “Impact of Pilot Diesel Ignition Mode on Combustion and Emissions Characteristics of a Diesel/natural Gas Dual Fuel Heavy-duty Engine.” Fuel 167: 248–256. doi:https://doi.org/10.1016/j.fuel.2015.11.077.
- Wei, L., C. S. Cheung, and Z. Ning. 2016. “Influence of Waste Cooking Oil Biodiesel on the Nanostructure and Volatility of Particles Emitted by a Direct-injection Diesel Engine.” Aerosol Science and Technology 50 (9): 893–905. doi:https://doi.org/10.1080/02786826.2016.1203390.
- Yang, B., C. Xi, X. Wei, K. Zeng, and M. C. Lai. 2015. “Parametric Investigation of Natural Gas Port Injection and Diesel Pilot Injection on the Combustion and Emissions of a Turbocharged Common Rail Dual-fuel Engine at Low Load.” Applied Energy 143: 130–137. doi:https://doi.org/10.1016/j.apenergy.2015.01.037.
- Yang, B., L. Ning, W. H. Chen, B. Wang, K. Zeng, and W. Dong. 2017. “Parametric Investigation the Particle Number and Mass Distributions Characteristics in a Diesel/natural Gas Dual-fuel Engine.” Applied Thermal Engineering 127: 402–408. doi:https://doi.org/10.1016/j.applthermaleng.2017.07.153.
- Yang, B., L. Wang, L. Ning, and K. Zeng. 2016. “Effects of Pilot Injection Timing on the Combustion Noise and Particle Emissions of a Diesel/natural Gas Dual-fuel Engine at Low Load.” Applied Thermal Engineering 102 (x): 822–828. doi:https://doi.org/10.1016/j.applthermaleng.2016.03.126.
- Yang, B., X. Wei, C. Xi, Y. Liu, K. Zeng, and M. C. Lai. 2014. “Experimental Study of the Effects of Natural Gas Injection Timing on the Combustion Performance and Emissions of a Turbocharged Common Rail Dual-fuel Engine.” Energy Conversion and Management 87: 297–304. doi:https://doi.org/10.1016/j.enconman.2014.07.030.
- Yousefi, A., H. Guo, and M. Birouk. 2019. “Effect of Diesel Injection Timing on the Combustion of Natural Gas/diesel Dual-fuel Engine at Low-high Load and Low-high Speed Conditions.” Fuel235: 838–846. August 2018.
- Yousefi, A., and M. Birouk. 2017. “Investigation of Natural Gas Energy Fraction and Injection Timing on the Performance and Emissions of a Dual-fuel Engine with Pre-combustion Chamber under Low Engine Load.” Applied Energy 189 (x): 492–505. doi:https://doi.org/10.1016/j.apenergy.2016.12.046.
- Yousefi, A., M. Birouk, and H. Guo. 2017. “An Experimental and Numerical Study of the Effect of Diesel Injection Timing on Natural Gas/diesel Dual-fuel Combustion at Low Load.” Fuel 203 (x): 642–657. doi:https://doi.org/10.1016/j.fuel.2017.05.009.
- Yuvenda, D., B. Sudarmanta, and A. Wahjudi. 2019. “Optimization of CNG Injection Duration on Combustions and Emissions Characteristics on CNG-CPO Biodiesel Dual Fuel Engine with Load Variations Optimization of CNG Injection Duration on Combustions and Emissions Characteristics on CNG-CPO Biodiesel Dual Fuel Engine with Load Variations.” AIP Conference Proceedings 020026-1 -020026-8.
- Yuvenda, D., B. Sudarmanta, A. Wahjudi, and O. Muraza. 2020. “Improved Combustion Performances and Lowered Emissions of CNG-diesel Dual Fuel Engine under Low Load by Optimizing CNG Injection Parameters.” Fuel 269: 117202. November. 2019. doi:https://doi.org/10.1016/j.fuel.2020.117202.
- Zhang, C., A. Zhou, Y. Shen, Y. Li, and Q. Shi. 2017. “Effects of Combustion Duration Characteristic on the Brake Thermal Efficiency and NOx Emission of a Turbocharged Diesel Engine Fueled with diesel-LNG Dual-fuel.” Applied Thermal Engineering 127: 312–318. doi:https://doi.org/10.1016/j.applthermaleng.2017.08.034.
- Zhou, H., H. Zhao, Y. Huang, J. Wei, and Y. Peng. 2019. x: Effects of Injection Timing on Combustion and Emission Performance of Dual-Fuel Diesel Engine under Low to Medium Load Conditions. Energies 12 (12). doi:https://doi.org/10.3390/en12122349
- Zhou, L., Y. F. Liu, C. B. Wu, L. Sun, L. Wang, K. Zeng, and Z. H. Huang. 2013. “Effect of the Diesel Injection Timing and the Pilot Quantity on the Combustion Characteristics and the Fine-particle Emissions in a Micro-diesel Pilot-ignited Natural-gas Engine.” Proceedings of the Institution of Mechanical Engineers, Part D: Journal of Automobile Engineering 227 (8): 1142–1152. doi:https://doi.org/10.1177/0954407013480452.