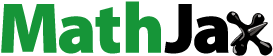
ABSTRACT
Global warming and population growth have been contributing factors to the decline of freshwater resources around the globe. More than 45% of the desalination plants are in the Middle East. Due to the severe scarcity of freshwater resources, Iran also needs to construct several desalination plants, since by 2021 the ratio of freshwater per capita in the country to the world average per capita will be 0.8. The uses of solar, wind, geothermal, and wave are practical in desalination systems. The average solar radiation in Iran is 15.3 kWh/m2/day, which includes more than 2800 hours of radiation per year in central regions. Also wind, geothermal, and wave energy potentials are equalled to 100106 MW, 200 MW, and 20 kW/m, respectively. The purpose of this paper is to evaluate the feasibility of using renewable energy for desalination in arid and semi-arid regions and Iran has been considered as a case study.
1. Introduction
Owing to the increase of the population in metropolises, several problems arise; increasing municipal solid wastes generation, growing energy consumption, and reducing freshwater resources are the main complications of urbanisation (Ahmadi et al. Citation2019; Esmaeilion, Ahmadi, and Dashti Citation2021; Makkeh et al. Citation2020). In the meantime, lack of freshwater resources has a direct impact on human quality of life, such that water scarcity would affect societies which in the next decades expected to become the most valuable thing in arid and semi-arid regions (Gohari et al. Citation2013). Dam construction, desalination process, water transmission, cloud seeding, wastewater treatment, and improvement of the functioning of the groundwater reserves are all paving the way for this crisis (Ahmadi et al. Citation2021; Al-Jasser Citation2007; Jung et al. Citation2015; Kucera Citation2019; Sanza, Bonnélyea, and Cremerb Citation2007; Sauvet-Goichon Citation2007). Among these, the highest yield with low volatility belongs to desalination. The desalination methods have been employed for a long time in arid lands like the Middle-East where contributed the most at 65%. That’s because of water scarcity and the high rate of fossil-fuel availability in these realms. KSA (Kingdom of Saudi Arabia) and UAE (United Arab Emirates), have the highest desalination capacities since 2002 in this region (Proskynitopoulou and Katsoyiannis Citation2018). In 2015, approximately 10% of the world’s population consumed desalinated water and predicted that until 2025, around 4% of urban inhabitants will join this group (Chandrashekara and Yadav Citation2017).
Water stresses in industrialised and developing nations have different rates; they can vary based on the economy, culture, social conditions, and natural resources. In the United States, for example, water consumption is 300 gallons per household each day, but in developing countries, it is limited to 150 litres per day, and in Africa, this equates to 20 litres per day (Esmaeilion Citation2020). Population growth and global warming have resulted in a growth in freshwater consumption, which through the World Water programme (WWP) estimates, by 2030 freshwater resources would reach levels that meet the needs account for only 60% of consumption, and by 2050 and 2010 it will reach 55% and 40%, each, causing a severe water crisis worldwide (Caldera, Bogdanov, and Breyer Citation2016; Saboori, Sapri, and Bin Baba Citation2014). This will increase consumption at a rate that doubles every 20 years, with only 10% of water consumption worldwide accounting for domestic consumption, while the rest being the shares of agriculture and industry sectors (70% and 20%, respectively) (Eltawil, Zhengming, and Yuan Citation2009). Emerging additional resources for freshwater alongside former standard water bodies like lakes, rivers, and wells (shallow) are an extra technique to address the rising demands of freshwater. Available supplementary sources contain sea-water, groundwater, deep-wells, and salty aquifers (Be et al. Citation2014). Using desalination methods for freshwater production has two-fold; increasing freshwater production regardless of environmental conditions and inefficiency in cost and energy. Therefore, the relationship between water desalination with production cost, energy consumption, and peripheral conditions are inevitable (Gorjian, Hashjin, and Ghobadian Citation2011; Grubert, Stillwell, and Webber Citation2014).
According to available data, in recent years the capacity of desalination plants has increased by 57% worldwide, which in turn indicates that in coastal areas the use of salty water as desalination plant feedstock and extracting freshwater for drinking uses is increasing dramatically (Jones et al. Citation2019; Zirakrad, Hashemian, and Ghaneian Citation2013). By 2019, overall desalination capacity across the world was around which reported that were around 16,000 operational desalination facilities around the globe (Esmaeilion Citation2020). Currently, most of them use thermal methods where sited in the regions with water insufficiency and abundance of oil resources. Specifically, more than 45% of global working desalination capacity is in the southwest of Asia, this specifies that 4826 sites in this area have capacity near
(Jones et al. Citation2019). On the other hand, hyper-saline concentrate (or brine), which is the output of the desalination plant, has detrimental effects on economic and environmental aspects. Obtained results by Jones et al. (Citation2019) represented that this value could be achieved 142 million m3/day that 55% of that belongs to Middle East countries. As mentioned before, Iran is surrounded by three main water body that in the Oman Sea coastal line construction of a desalination plant is a vital need, since this region is an arid area with a high-temperature average and it’s expected to produce 3.5 bcm/day freshwater by desalination systems until next decade (Gorjian and Ghobadian Citation2015). Based Caldera, Bogdanov, and Breyer (Citation2016) the demands for desalinated water and associated electrical power for Iran would be 89419
106 m3 and 708.7 TWhel, respectively (in 2030).
It’s pervasive that Iran isn’t amongst the countries by high desalination capacity and because of that requires rapid progress in this field to reach the Middle-East average. The earliest desalination unit in Iran was built in 1960 on Khark-Island, based on multi-stage, while the capacity equalled to m3 per day in small-scale systems. Subsequent further development resulted in a capacity of
m3 per day (Emami, Ghajar, and Kaynia Citation1977).
In Iran, the coastal areas of the Persian Gulf and Oman Sea are suffering from severe water crises due to the high mean temperatures during the year and the lack of groundwater resources. At the same time, these areas have the potential to benefit from seawater as a system feedstock and fossil fuels and solar energy as fuel consumption for desalination systems. Significant water shortages in these areas have led to the depletion of most of Iran’s desalination plants. One of the first desalination plants in Iran is located in Chabahar which reached 15,000 m3/day using MSF systems and in the second phase reached 35,000 m3/day (by RO technology). The government’s development plan is to solve the water crisis in these areas by 2021 with the help of desalination systems.
2. Iran’s geographical and topological status
Iran is an arid country placed in the dry belt that more than 70% of its areas are arid or semi-arid. Nowadays, drought and shortage of drinkable water are some of the main problems in Iran, however, it’s planned to reach renewable water at 800 m3 per capita until 2021 yet it would be less than global quantities (about 200 m3). It’s important to mention that country is bounded by three main water bodies, named: the Caspian Sea, Sea of Oman, and the Persian Gulf which is located at the northern and southern borders. Fossil fuel resources provide favourable conditions in southern regions for producing freshwater from seawater; nonetheless, these types of energies are confined by the economic, environmental, and geographical conditions (Gorjian and Ghobadian Citation2015). The latitude and longitude coordinates of Iran are in the range of 44 to 64 eastern and 25 to 40 northern. Iran is ranked 17th and 18th in terms of population and area, respectively. In recent decades, with the rise of urbanisation and the advancement of technology following industrialisation, the amount of energy consumed has increased, making it the 20th most consumer country in the world (Iran Foreign Policy & Government Guide, Vol. Citation1348. USA: International Business Publications; 2006 n.d). It is one of the largest realms with a high range of energy reserves as it ranks fourth and second in the world in terms of oil and natural gas resources, respectively. This has made fossil fuels the largest share of electricity generation in Iran, but the biggest drawback is the large volume of pollutants emitted
Iran has a high diversity in different climates so that a wide range of temperature variations can be observed in different months of the year (−20 to +50°C). These climate changes have caused different weather conditions across the country (Madani Citation2014; Soltani, Saboohi, and Yaghmaei Citation2012). The average precipitation in Iran is less than one-third of the worldwide average. Furthermore, in this country, rainfall has irregular patterns that affect the industrial, agricultural, and urban sectors. Likewise, the prolonged drought in some areas has caused problems for the residents of these areas (Faramarzi et al. Citation2009; Tir, Momeni, and Boboevich Citation2014). Precipitation has a key role in providing water sources. The average value for annual precipitation in the country is about 400 billion cubic metres (BCM); however, it fluctuates throughout the country. illustrates the distribution of precipitation in Iran. Most of the areas enjoy a smaller amount of rainfall annually (around 100 mm).
Figure 1. Distribution of precipitation in Iran (Khalili and Rahimi Citation2014)
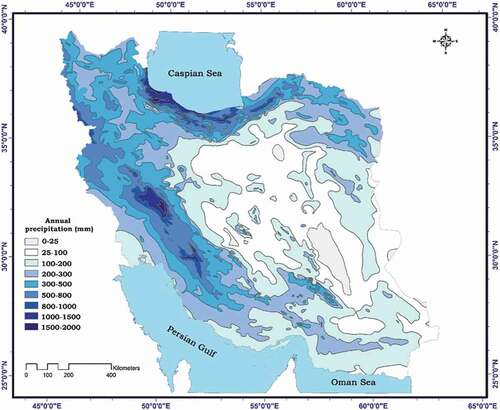
The coastal areas in Iran enjoy more rainfall. On the other hand, altitude is a factor that changes the amount of precipitation and its type, which makes it slightly less than one-third of the total precipitation in Iran. Annual data confirm that the precipitation in Iran is almost third of the worldwide mean. In the last decade, with global warming and climate change, precipitation patterns in the country have changed, causing seasonal floods, prolonged droughts, and damage to agriculture and industries (Negewo Citation2012).
Over the last 60 years, per capita, renewable water has declined substantially (from 7000 cubic metres to 1700 cubic metres) (Motevallian and Tabesh Citation2011). However, forecasts indicated that, until 2021, this amount will reach 800 cubic metres per year, which is 200 cubic metres below the threshold of the water crisis (Roostaei Citation2004). Studies show that in the year 2021, Iran needs to produce water by 103 annually, of which about 7% of it will be used in domestic and industrial sectors and the remaining amount would be dedicated to the agricultural sector. In the light of the situation ahead, dams are constructed over the rivers of the country which 316 dams have been built so far, 132 dams are under construction and 340 dams will be built soon (Heydari, Othman, and Noori Citation2013; Madani Citation2014). Reducing rainfall, increasing population, and increasing demands for water in industry and agriculture have led Iran to turn to groundwater that has provided certain environmental conditions for drying groundwater. It’s interesting to mention that the agricultural industry in Iran has the largest share of water consumption, while 90% of the country’s water resources are allocated to this sector, while water use efficiency is about 15% to 36% and imposes a large number of losses on the water network (Faramarzi Citation2010). Estimations of aquifer renewal showed that the eastern part of the country would witness reductions of up to 50–100% in groundwater recovery in areas that are already threatened by lack of water resources. The northwestern region of the country would enjoy a growth in groundwater recharge owing to an increase in rainfalls. All developments in the future specify an upsurge in the aquifer refresh in this area. The future models show an increase in water reservoirs in the western part of the realm through the central and eastern zones will suffer from a shortage in the limited water resources. By now, a vast part of the country suffers from water scarcity. Furthermore, hydrological variations in the country are not restricted to surface waters, but also cover the groundwater resources, with considerable reductions in groundwater level for each area of the country ().
Figure 2. The locations of dams, reservoirs, water transfers, and groundwater harvest in the country
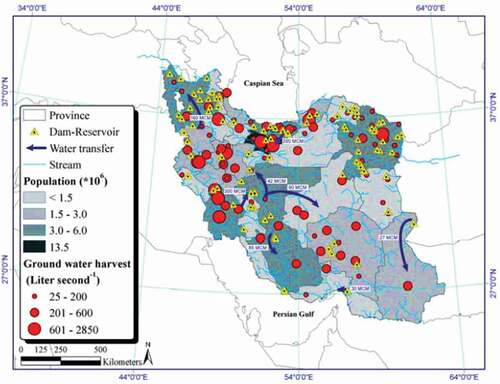
Current data confirm that, if the present development strategies continue, the capability of the installed renewable energy systems will achieve to 2.8 GW unit 2030. This trend entails over 2800 million US$ investment in 20 years, specifically, from 2010 to 2030. Regardless of the benefits of employing solar energy, like decreasing GHGs, it is vital to mention that solar power is 2.5 to 5 times as costly as electrical power from current conventional power resources. With the intention of inviting people to employ solar power, there is a necessity to modify the laws and introduce a combined energy regulation, including tax policy mechanisms to assist the distribution of solar applications in Iran. Since Iran is reliant on fossil fuels, the move from fossil fuels to renewable needs the acceptance of an inclusive policy and integrated regulation nationwide, taking a multi-disciplinary methodology. This accomplishment needs four objectives for sustainable progress in the regulation. Providing these four aims is essential to attain an applied sustainable development at the nationwide level: (1) continuous employ of natural resources, (2) conservation of stable levels of economic development and employment, (3) social evolvement, and (4) operative protection of the environment. Correspondingly, Progress of energy markets, augmented transactions, manufacture of private sector hydroelectric and renewable energy power plants, development of power plants, growth in the effectiveness of power plants, and maintenance of turbines after operating for 100 GW, are selected strategies used by the Ministry of Energy in Iran. In the view that geothermal plants are sited in countryside regions, which normally have high joblessness rates, the geothermal developments can make various occupation openings in these regions. Also, numerous specific challenges cause complications about geothermal progress in Iran like deficiency of national or local legislation, poor management of human resources, and incapability of technology transmission. To spur geothermal knowledge and overcome these challenges, guidelines at the national or local level can provide a variety of motivations for the manufacturing, installation, and utilisation of renewable energies (Noorollahi et al. Citation2019).
3. Desalination industry in Iran
3.1. A mini-review of conventional desalination methods
In general, the desalination technology of seawater has two-fold: Membrane (MD) and Thermal. The MD usually takes benefits from mechanised tension, electric power, or concentration differences in place of the motivating tool for penetrating semi-permeable membranes, causing material separation. Instead of the thermal process, thermal energy is used to vaporise water from saltwater. There is more experience in thermal desalination technology because for a while it was the only available technique for the desalination process (Wetterau Citation2011). Estimated costs for freshwater production by Electrodialysis- multi-stage flash (EDMSF), Membrane desalination (MD), Reverse Osmosis (RO), Multi-stage flash (MSF), Vapour compression (VC) and Refrigeration freezing (RF) are 0.44, 0.53, 0.52, 0.45, 0.39 and 0.35 $/m3, respectively (Borsani and Rebagliati Citation2005; Tofigh and Najafpour Citation2012; Zhou and Tol Citation2005).
3.1.1. Membrane desalination
The membrane desalination process is established by the abilities of the semi-permeable membrane to allow specified ions to get it through. In this technology propulsion forces for ions are mechanical pressure differences, electrical potential differences, and concentration differences. The membrane desalination method has a variety of subcategories that RO, ED, EDR, FO, and nanofiltration (NF) are from this category which their propellant forces are mechanical pressure differences, electrical potential differences (ED and EDR), concentration differences, and hydraulic pressure respectively. Among the methods mentioned, RO is the most commonly driven technique in membrane desalination which due to the low cost compared to other methods became very popular in different regions across the globe, particularly for Middle East (Amy et al. Citation2017; Yuan et al. Citation2017).
3.1.2. Thermal desalination
All the processes used in the thermal method are based on changing the water phase and applying energy to the solution while the steam arrives at the unit and converts to less saline water and is a convenient approach for solutions with high salinity. For the reason that the Persian Gulf has one of the saltiest waters on earth, this method is very popular in the Middle East, for example, KSA, UAE, Oman, Qatar, and Kuwait were extremely employing regarded process which around 75% of total systems of this type are sat up in Persian Gulf states. There exists a great potential for this type in Persian Gulf shores where the cost of energy is next to nothing and for this reason, KSA owes 34.8% of the overall total (around ) and UAE desalinates about
. Thermal approaches are MSF, MED, and VC while for the first two cases the direct type of heat exchanger was utilised for supplying the required heat for the dehydration process, however, for VC, leaving thermal energy plays this role. Among them, the hoariest method is MSF that entails an excessive quantity of vapour at elevated temperatures. MED and VC remain the more innovative systems and reducing the boiling temperature have enhanced the associated productivities (Al-Othman et al. Citation2018; Kim et al. Citation2016; Ortega-Delgado, Garcia-Rodriguez, and Alarcón-Padilla Citation2017). Due to the great energy demand for these methods, thermal processes are extremely reliant on limited settings concerning capital and current charges. It’s beneficial to integrate these sorts of systems for generating power and freshwater, concurrently (Bennett Citation2015). This category of mending is commonly applied in the Middle East, where there aren’t any strict environmental restraints while the thermal desalination systems have appropriate performances for operating for about 10 to 15 years (Chandrashekara and Yadav Citation2017). Thermal methods have the potential to provide freshwater with a minimal amount of salt (less than 0.01 mg/m3) by saline water with a large amount of TDS in the range of 60–70 mg/m3 (Cotruvo et al. Citation2010).
The fraction of produced water to the required steam is the GOR (Gained Output Ratio) which based on the equipment, ambient conditions, and feedwater quality, this value fluctuates between 4% and 40%. The upper value of GOR denotes advanced productivity (Voutchkov Citation2012). The involvement shares of named methods are 69, 18, 7, 3, 2, and 1% for RO, MSF, MED, NF, ED, and others (technologies like Electrodeionization, Freezing, and Nuclear methods).
3.2. Desalination developments in Iran
provides information on Iran’s largest desalination plants, along with their capacity, location, and technology (Fried and Serio Citation2012; Gorjian and Ghobadian Citation2015; Intelligence et al. Citation2011; Renewable Energy and Energy Efficiency Organization Citation2020). As indicated in the table, Iran employed all three technologies. Meanwhile, the only hybrid gas-desalination plant on the island of Qeshm has the technology to utilise the heat dissipated exhaust gas turbine. The system, which has a capacity of producing 18,000 cubic metres of freshwater per day, uses a temperature of 200°C and a pressure of 12 bar exhaust as a heat source, while the turbine has a capacity of 25 MW (CDM–ExecutiveBoard Citation2014). Shirazi, Kargari, and Shirazi (Citation2012) assessed an optimum operation of membrane desalination of Persian Gulf water for achieving an ideal purity. The use of desalination plants in the agricultural industry in the south of the country (Bushehr) in 2015 showed that desalinated water production was 5000 m3 per hectare per season of crop production (Karami, Karami, and Zand-Parsa Citation2017).
Table 1. Specifications of biggest desalination plants in Iran (Fried and Serio Citation2012; Gorjian and Ghobadian Citation2015; Intelligence et al. Citation2011; Renewable Energy and Energy Efficiency Organization Citation2020)
There are three MED-TVC desalination plants with capacity for producing freshwater at the utility site, in Assaluyeh–Iran () (Kouhikamali and Sharifi Citation2012). In the considered system, some of the operational parameters are: Boiler temperature, Steam flow rate, Seawater temperature, Seawater flow rate, and GOR equalled to 170°C, 8 ton/h, 33°C, 420 ton/h, and 8, respectively (Kamali and Mohebinia Citation2008).
Figure 3. Illustration of three MED-TVC integrated systems installed in Assaluyeh-Iran (Kouhikamali and Sharifi Citation2012)
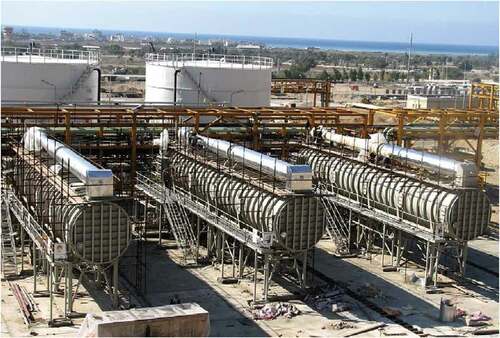
Experimental studies of a double-stage unit (solar humidification – dehumidification) located in Qom-Iran, represented that the efficiency can be increased by 20% in comparison with a single-stage unit (Zamen et al. Citation2014). Techno-economic analysis of the combined cycle plant in Asalouyeh for the most optimum technique for desalination presented that by adding a definite number of the tube to deaerator, a cost-effective option for freshwater production with the capacity of 5200 m3 per day is achievable (Gomar, Heidary, and Davoudi Citation2011). Additionally, the analysis of the physical and chemical features of the RO desalination system in Birjand for parameters such as pH, Cr, Mn, and salinity at the inlet was as high as 7.7, 0.078 (m/l), 0.1 (mg/l), and 0.8 that reached to 7.5 0.034, 0 and 0, at the output, respectively (Khodadadi et al. Citation2016). In another study, for Bandar Lengeh Port, RO plants reduced the chemical compounds in seawater in a way that TDS, Sodium, Chloride, and Sulphate decreased from 37,749, 9715, 22,020, and 3067 mg/l to 1233, 436, 710, and 58 mg/l, each (Zirakrad, Hashemian, and Ghaneian Citation2013). Also, Shafaghat et al. designed a 42 MW combined cycle power plant to supply an MSF desalination system in the Persian Gulf seashores (Shafaghat et al. Citation2012). Mazini et al. established a mathematical dynamic exemplar for the MED-VC plant in Kish Island that presented an ideal design of the system (Mazini, Yazdizadeh, and Ramezani Citation2014). The efficiency of the RO process in the southern part of Iran (Jask city) was 94.16% that this strategy improved the quality of the potable water considerations (Yousefi, Motallebi, and Takdastan Citation2014).
The water issues in the country are substantial to move from all hesitations about the circumstance that Iran is suffering from a severe water crisis. Presently, a great share of the Iran water source is from seawater desalination. Regrettably, fossil fuels are the only energy resource applied to power all the treatment plants located in the southern coastal areas. Producing freshwater by using fossil fuel-assisted units is not a sustainable and ecological method to defeat the water shortage in Iran because of depletion threats of accessible energy sources and the growth of GHGs. Consequently, emerging processes that are renewable for producing freshwater in Iran are important. Despite the overwhelming statement that Iran has no high-load operating plant with a major capacity of renewable energy desalination for producing freshwater, analyses of the present renewable energy production and the applicable potential expose abundant opportunities for Iran. As can be observed, Iran has the greatest prospects in solar and wind-powered energy generation and by integrating all the measurements, the country can be entirely pleased with its water stresses which this value can reach 28 billion cubic metres of drinkable water. Also, nuclear treatment is recommended as a utility for facing water scarcity problems. The total productivity of this type of desalination system can significantly be improved by means of combination with thermal desalination plants. The assessment of the capability is yet optimistic as there are numerous obstacles. There exist countless undiscovered sections like transportation infrastructures and pipelines. There exist several threats to be measured relational to each area of the country. The challenges for absorbing suitable investment in renewable water treatment can be an additional serious problem for Iran as the outlays of these developments are considerably dissimilar from other conventional water desalination plants. Though renewable energy has potential for development in Iran’s rural areas because of environmental, social, and economic benefits, it could face some infrastructural, managerial, socio-cultural, and economic challenges (Norouzi, Yeganeh, and Yusaf Citation2021).
3.3. Water resources
The desalination method can process a wide range of water resources, that is, seawater, brackish water, saline groundwater, and wastewater. It’s reasonable to note that seawater is the most used and important source among others whereas from the last two decades the contributions of other types have been raised (Abrams Citation2018). The world health organisation (WHO) stated that the permitted limitation for salt is from 500 ppm to 1000 ppm for various circumstances. While most sources have salts of more than 10,000 ppm (Eltawil, Zhengming, and Yuan Citation2009). The term ‘freshwater’ is assigned to water by 100–500 ppm salinity. Approximately from 3 to 5% of the globe’s waterfalls place in this category (Reif and Alhalabi Citation2015). demonstrates water-insoluble solid particles (g/ m3) based on the TDS (total dissolved solids) that characterise the quantity of NaCl (sodium chloride) (DeZuane Citation1997). The TDS of groundwater sources has an extensive range based on surface soil and drainage conditions. Also, the brackish water salinity is in the range of freshwater and seawater (approximately 5000–35,000 ppm) which makes up 23% of the Earth’s water. The seawater’s salinity varies from 35,000 to 45,000 ppm that contributes to 58% of the globe’s water. The remaining water sources belong to rivers (roughly 7%), waste-waters and sewages (around 5%), and others (El-Ghonemy Citation2012).
Table 2. The categorisations of water by TDS (DeZuane Citation1997)
Seawater has been used as a feedwater resource in numerous desalination plants throughout the world (about 58.85%) (Kucera Citation2019). International Desalination Association (IDA) reported that the applied capacity raised around 75 million m3 per day (from 5 to 80 m3 per day) over 33 years (Caldera, Bogdanov, and Breyer Citation2016). points out that the seawater in the Middle East contributed the most as feedwater in desalination plants (Latteman Citation2010).
Table 3. Water resources in desalination plants and corresponding contributions at different resources (Latteman Citation2010)
4. The energy in Iran: present conditions and prospected capacities
In the last decade, with the increase in urbanisation and the progress in technology, industrialisation has increased, with energy consumption rising to the top 20 countries in the world (Ghorashi and Rahimi Citation2011a). Iran is one of the biggest energy resources, ranked fourth and second in terms of oil and nature gas resources, respectively, which these resources are 9% and 15.8% of global sources and led to fossil fuels to be the principal source of energy for producing electricity, nevertheless, the main drawback is the greenhouse gas emissions. Based on a long-term plan, by 2030, 29% of electricity would be generated by renewables (International Energy Agency Citation2020). Even though in recent years, efforts in this area have led to a reduction of electricity generation by fossil fuels and raising the contributions of renewables. This broad platform is engrossed in the production of oil derivatives and lessening fossil fuel consumption. A glance at provided reveals some substantial evidences of produced renewable electricity over the course of 45 years. It’s pervasive that the main renewable energy resource for electricity generation in Iran is hydropower.
Figure 4. Electricity produced and projected by plant type in Iran (International Energy Agency Citation2020)
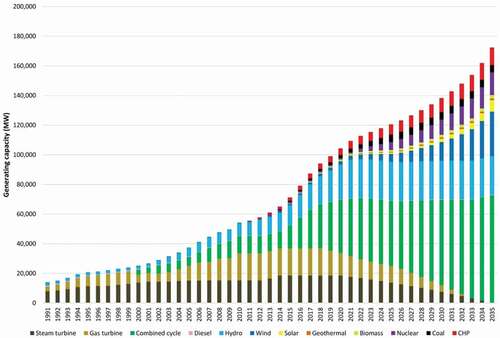
Iran is capable of decreasing the consumption of fossil fuels by focusing on renewable energy sources (RES) and consequently reducing corresponding emissions. Increasing energy consumption in urban areas is more than in rural areas, due to the more population in cities and more use of various appliances for reaching more comfort (Bakhoda et al. Citation2012). Principally, energy consumption in the world is based on the electricity quantity and while fossil fuels contributing the most in this field (approximately 66%) (Heshmatzadeh Citation2000). According to the investigated results from the International Energy Agency (IEA), oil and natural gas have limited resources, in 41.8 and 60.3 years these resources will be exhausted. These statics are estimated and have been approved by the Ministry of Energy for Iran. For that reason, growing focuses on renewable energy sources and considering the environmental aspects are crucial. The energy mix used in Iran has presented in .
Figure 5. Energy Mix in Iran (Ahmadi et al. Citation2019)
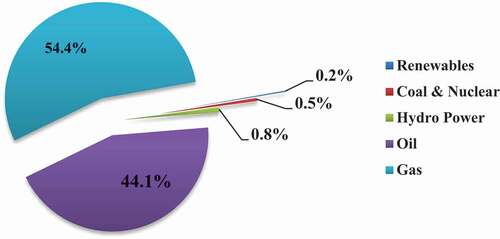
The fluctuations in the growth of energy consumption, expenditures, and obstacles to finding alternative energy resources would be caused by a crisis in the energy sector (Awan and Khan Citation2014; Pietrosemoli and Rodríguez-Monroy Citation2019).
5. Feasibility assessments of renewable energy sources for desalination
Using renewable energies to produce potable water is a viable and affordable option that can sustain system performance by fixing disadvantages in fossil fuel desalination systems. Fossil fuel resources are costly and air-contaminating and would have wide effects on the atmosphere and environment. Moreover, consuming them isn’t cost-effective for isolated zones (even for seaside regions). In numerous places, the lack of access to fossil fuels and potable water sources has made it a viable option to utilise desalination systems using renewable energy sources. Furthermore, efforts for reducing GHG emissions will lead to better investment in renewable sources (Gilau and Small Citation2008). Renewable energies are widely used in various processes, in which wind, solar, geothermal, and wave energies are extensively used in desalination processes (Foster, Ghassemi, and Cota Citation2009; Tabak Citation2009). The influence of renewables on energy supply will be raised from 14% to 63% (for 2015 and 2050, each), in which solar and wind energy will be around 70% of renewable energy sources that year. (Gielen et al. Citation2019; Ummel and Wheeler Citation2008). To achieve sustainability in freshwater production, assessing energy resources is a primary option, in this case for instance to overcome the difficulties and charges associated with transferring power to distant zones, using hybrid systems comprised of solar and wind energy becomes sensible (based on their local potentials) (Ashok Citation2007; Esmaeilion Citation2020; Liu and Wang Citation2009).
The solar and wind energy resources have contributed the most between each type of renewable energy desalination; still, other techniques like biomass, hydroelectric power, and ocean energy are conducted to produce electricity for small-scaled plants. The most drawbacks of wind and solar are impulsiveness and dependency on meteorological conditions which ordinarily batteries were employed. However low-storage lifecycle, high-maintenance expenses, and ecological impacts are their main drawbacks (Energy Citation2012; Nagaraj et al. Citation2016; Spyrou and Anagnostopoulos Citation2010). In recent times, scientists have integrated fuel cells with electrolysers that made them consistent and eco-friendly (Askarzadeh Citation2013; Maleki and Askarzadeh Citation2014; Shatat and Riffat Citation2012). represents the associated chart of renewable energy desalination processes (Brauns Citation2010; He, Wang, and Shaheed Citation2015; Tufa et al. Citation2015, Citation2019). determines the influence of renewable energy resources on desalination.
So far, about 20,000 desalination stations are under operation in Iran by around capacity and there is a long-term plan to achieve greater quantities of freshwater production capacity of 700,000 cubic metres per day, while fossil fuels remained to be a key source of energy for these systems. For example, the energy required to produce
of freshwater from desalination units requires about 10 million tons of oil annually, indicating excessive energy consumption in these systems (Gude and Nirmalakhandan Citation2010). However, the abundance of fossil fuel sources and their relatively low price does not make it viable to capitalise on the renewables sector (Ghorashi and Rahimi Citation2011a; Keivani Hafshejani et al. Citation2012).
The desalination industry using fossil fuels has a lot of environmental detriments, and its continued use increases emanations of GHGs, particularly CO2, which is a foremost participant in global warming (Lotfalipour, Falahi, and Bastam Citation2013). As represented in , the rate of CO2 emissions increased during the period from 1960 to 2016. Predictions indicate that the CO2 emission would be reached at 925.68 million tones by 2020 and that shows a 50% growth in this amount over five years (The World Bank Citation2020). In this case, presents the environmental characteristics of the desalination technologies matrix of major desalination processes (based on the Ecopoints 97 and EI 99 evaluation methods). It’s pervasive that, RO produced lower emission in comparison with other mentioned technologies.
Table 4. Environmental and technical comparative characteristics of MSF, RO, and MED plants (Borsani and Rebagliati Citation2005; Caldera, Bogdanov, and Breyer Citation2016; El-Ghonemy Citation2012; Mezher et al. Citation2011; Raluy, Serra, and Uche Citation2006; Sommariva, Hogg, and Callister Citation2004)
Figure 6. Iran carbon (CO2) emissions 1960–2016, (a) kilotons per year and (b) metric tons per capita per year (The World Bank Citation2020)
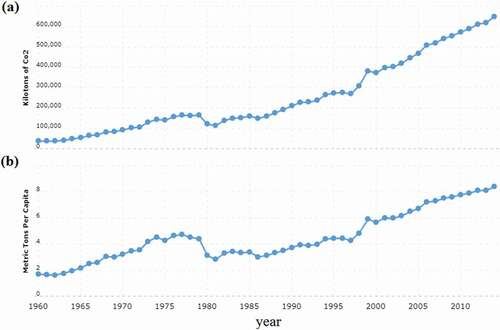
Figure 7. Diagram of combining various desalination systems by renewable energy resources (Brauns Citation2010; He, Wang, and Shaheed Citation2015; Tufa et al. Citation2015, Citation2019)
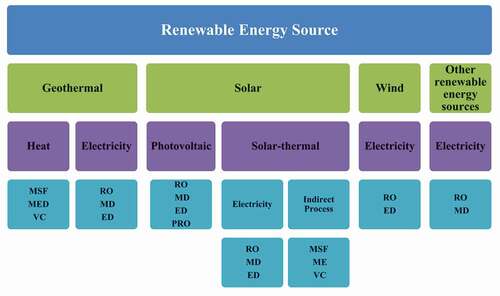
5.1. Solar water desalination
Semi-arid areas are typically sited in the Middle East and northern parts of Africa, where transmitting drinkable water from other zones is an expensive approach. At present, the benefits of solar energy have the most contribution among entire sorts of renewables. The potential for solar power in arid and semiarid areas of the Middle-East and Northern parts of Africa is to the extent that by covering 1% of these areas with solar collectors, it is possible to generate electricity on demand while these regions receive from 1700 to 2200 kWh per m2 of solar energy annually (Hosseini, Soltani, and Valizadeh Citation2005; Sukhatme and Nayak Citation2017). Though, some issues are containing the effectiveness of the system, the unstable degree of energy expected from the sun, and infrastructural shortage as restraints in large-scale desalination capacities (Shatat, Worall, and Riffat Citation2013b). Predominantly, two categories of solar-based energy systems have been introduced:
Photovoltaic (PV) plants collect the radiations and transform them into electrical power for use in RO and ED systems.
Solar-thermal plants by converting the energy of radiations to thermal energy, while being in direct and indirect modes. Solar distillers, solar collectors, and solar ponds are from this category.
shows the various approaches to the desalination process based on solar energy. represents the contributions of different desalination systems integrated with solar energy systems has been demonstrated.
Figure 9. Various approaches for solar desalination (Caldera, Bogdanov, and Breyer Citation2016)
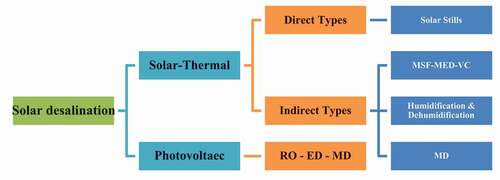
5.1.1 Iran’s solar energy potential
Due to the geographical and geological status in Iran, most of the locations have the potential to benefit from the sustainable use of solar energy, making this option a different case to other renewable energies. The average solar radiation equalled 15.3 kWh/m2/days, while includes over 2800 hours of radiation per year in central regions. As shows, the amount of radiation in the central and southern parts is more than in the northern areas of the country, while these regions are suffering from a severe water crisis and this is an opportunity to use solar energy as propulsion of desalination systems (Esmaeilion et al. Citation2020). In recent years, exceptional consideration has been allocated to increasing investment and improvement of solar energy use for seawater desalination (Alamdari, Nematollahi, and Alemrajabi Citation2013; Bahrami and Abbaszadeh Citation2013; Gorjian et al. Citation2013; Vafaeipour et al. Citation2014). High-potentials of solar energy in Iran are revealed to be at its uppermost level for Bushehr-port (in the southwest coastline) with a 26.1% irradiation conversion rate, and Anzali-port (central-north coastline) with a minimum level of 16.1% conversion rate (Mollahosseini et al. Citation2017). Regarding the existing installed solar power plants, present energy generation by PV plants is expected to be about 650 MW, whereas Iran has projected to achieve further than 139,000 MW by 2030. It is noteworthy that the country maintains the potential to the extent of 40,000 GW power generation.
Figure 11. Global horizontal irradiation for Iran with higher potential in central and southern regions (Esmaeilion et al. Citation2020)
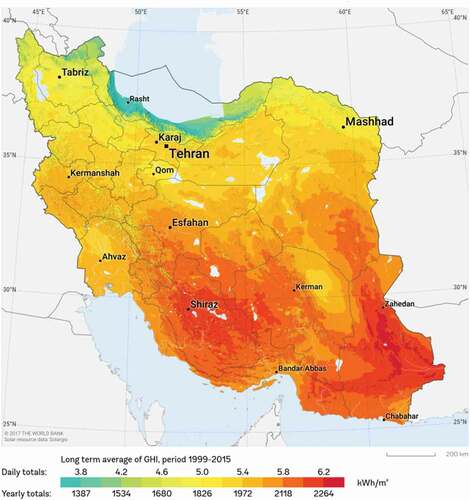
Solar energy can provide expectations for the production of thermal energy. The exploit of solar energy as a propellant provides the capacity to intensify the sustainability, efficiency, and environmental advantages relatively (Colangelo et al. Citation2016; Ganesh Citation2015; Khan and Arsalan Citation2016; Lewis Citation2015; Li et al. Citation2017; Oruc et al. Citation2016; Pichel, Vivar, and Fuentes Citation2016; Purohit and Purohit Citation2018; Qiblawey and Banat Citation2008; Rajaei, Atabi, and Ehyaei Citation2017). Solar energy extraction has several ways, including direct conversion by PV arrays, indirectly by parabolic trough collector (PTC), concentrated solar power (CSP), solar ponds, and linear Fresnel collector (LFC) (Esmaeilion, Ahmadi, and Ehyaei Citation2021; Singh Citation2013). Various factors drive the need for energy towards renewable energies, the most prominent of which are environmental factors, the limited availability of fossil energy sources, and the increasing need for energy consumption that draws more attention to renewable energies. Iran’s solar energy potential is such that if it covers 1% of Iran with solar collectors, the amount of electricity produced will be five times greater than the net amount of current electricity which Iran has an area of 16 km2 with 300 sunny days per year (Besarati et al. Citation2013; Gholamhasan Najafi et al. Citation2015).
Degradation of PV plants because of dust deposition is one of the chief concerns of PV specialists, particularly the PV modules which are installed in the hot and dry regions of the country. The deposition of dust on the glasses reduces solar energy production during the year. The research outcomes displayed that the average dust deposition is about 4.6 × 10−3 g/m2 per day throughout the year. In the case of no-precipitation and no-cleaning, this quantity will touch 2.74 × 10−2 g/m2 per day. The proportion of solar energy lessening because of dust deposition is varying from 2 to 16% throughout the year. The average reduction in solar power is 7% during a year, and this value can be maximised at 44% in the case of non-precipitation and non-cleaning (Abdolzadeh and Nikkhah Citation2019; Bakhshi-Jafarabadi, Sadeh, and Dehghan Citation2020).
5.2. Wind-powered desalination
The atmospheric pressure difference created by solar energy is the main driver of wind generation, with 0.6% of the solar energy reaching the Earth’s atmosphere (173,000 terawatts). Wind energy could be obtained by means of wind turbines, as the current of air blows through the turbine’s aerodynamic blades, the generator rotates and the motive energy of the wind is converted into electricity. How the turbine blades are positioned divides it into horizontal or vertical types (Yılmaz and Söylemez Citation2012). Since wind turbines are capable of operating in remote areas with appropriate wind potential, the connection of small desalination plants to wind turbines is a viable solution for the production of freshwater by saline and seawater (Kalogirou Citation2005). Presenting strategies contained the integrated system consisted of wind turbines and RO or MVC desalination methods is a common approach for freshwater production in small-scale systems. Gökçek and Gökçek (Citation2016) studied the techno-economical approaches of small-scale wind turbine integrated with an RO system. The results represented that the freshwater cost in a separated unit differs from 2,962 to 6,457 US$ per cubic metre related to the turbine power (from 6 kW to 30 kW). Also, it’s noteworthy to mention that by implementing a 30 kW wind turbine, the carbon dioxide rate would be reached at 80 tons per year. Further studies have also addressed the use of wind energy in RO systems in economic, technical, and environmental aspects (Peñate et al. Citation2011; Segurado et al. Citation2015). As confirms, the use of wind turbines is a practical way of developing renewable energy-based desalination plants. In this regard, wind power provided more than 56% freshwater compared to using a power grid separately. In this cycle, the investment expenditures regarding the prices of the wind turbines are 2,000 €/kW, fossil fuel-based units are 1,200 €/kW, and the desalination units are 1,000 €/m3 per day.
Figure 12. Integrating wind turbines, electrical network and desalination system aimed at freshwater production (Segurado et al. Citation2015)
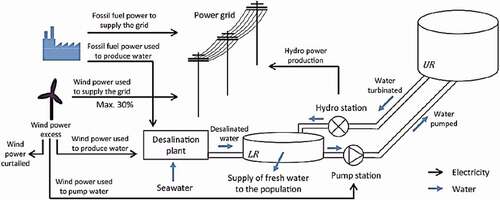
Solar radiation is only available during the day, while wind profiles at night are faster, so these two sources of energy can complement each other. Obtained results from an integrated system consisted of MED and MVC systems, solar energy, and wind turbine represented that the plant’s site, the amount of radiation, speediness of wind, environment conditions, and salinity of intaken water are key parameters on operating the system (Ismail et al. Citation2016). (Nagaraj et al. Citation2016) investigated the system performance of brackish water desalination by wind turbine and PV unit. In this type of system, the power supply would be guaranteed over the year ().
Figure 13. Producing fresh water by combining wind, PV, and RO systems (Nagaraj et al. Citation2016)
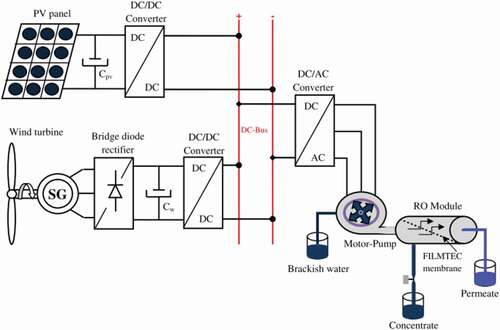
5.2.1. Iran’s wind energy prospective
Wind energy has the most economic conditions for generating electricity, and researches show that it would grow its contributions in near future (Smith et al. Citation2009). Geographical maps of Iran show that due to the low air pressure in the central and southern parts, in comparison with high air pressure in the northern and northwest parts, it has the potential for winds in summer and winter (Najafi and Ghobadian Citation2011b). Estimations show that Iran’s wind potential is 100,000 MW. One of the important factors in creating seasonal winds in Iran is the difference of temperature and pressure of air currents entering the region; One is from the west and northwest by the Mediterranean Sea and the Atlantic Ocean starting point and the other from the east by the Indian Ocean source, which generates 120 days of gales in the warm season in the southern parts and Sistan and Baluchistan regions. However, due to the appropriate wind capacity in the north and south, the wind power industry has had little development in Iran (Mousavi, Ghanbarabadi, and Moghadam Citation2012). Estimates indicate that Iran’s primary capacity is 6500 MW and can be upgraded to 15 GW with further researches and developments (Fadai Citation2007; GWEC, Citation2017). Still, it’s the only country in Middle-East to benefit from large-scale wind energy and has a production capacity of about 0.12 million barrels of oil, which saw a 27% growth over the 10 years from 1998 to 2008 (Hosseini et al. Citation2013b). Most of Iran’s wind power plants are located in Guilan (north of the country) and Khuzestan (southwest of the country) and due to their proximity to the seas, they can be used in desalination plants (the former is located on the Caspian-coasts and the latter on the Persian Gulf coasts) (P Alamdari, Nematollahi, and Mirhosseini Citation2012), Which Iran’s largest wind power plant is located in Manjil with a capacity of 61180 kW in Guilan (Mohammadnejad et al. Citation2011). Obtained results from (Mostafaeipour Citation2010) showed that Iran’s offshores have a desirable potential, located adjacent to the occupied islands and seaside cities besides the seaboards of the Persian Gulf, the Caspian Sea, and the Gulf of Oman. illustrates the average value of wind speed (m/s) for different regions of Iran (Global Wind Atlas Citation2020). The areas near the eastern border of the country have high wind speeds with low inhabitants, which is why building a large-scale wind power desalination plant is not economical for this region.
Figure 14. Mean wind speed map of Iran (Global Wind Atlas Citation2020)
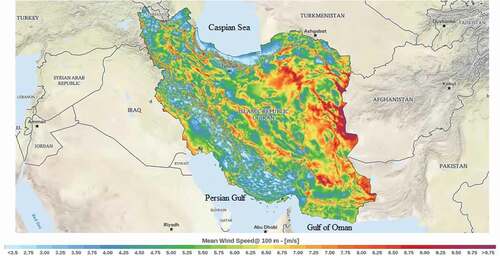
Rahmani et al. (Citation2014) and Razavieh et al. (Citation2017) studied the potential of wind power and wind turbines performance in the southeast of Iran, which showed that in warm months of the year, wind turbine output power and wind speed are higher at different altitudes. However, in the northern regions, such as Tehran, in spring (March and April) the highest output power is achieved (Keyhani et al. Citation2010). In this field, Aghahosseini et al. (Citation2018) presented a scheme to attain a 100% contribution of renewables production in the energy sector of the country, with the help of wind and solar combination and utilisation of power storage systems. With the aid of integrated scenarios, the total renewable energy installed capacities could be improved affectedly from 172.4 to 1089.4 GW to produce 2269.9 TWh (+601%) for seawater RO desalination. Mostafaeipour et al. (Citation2017) observed the stability and possibility of wind-powered seawater desalination plants for 10 ports and 5 islands in the southern parts of Iran.
5.3. Geothermal and wave-powered desalination methods
Geothermal energy is widely dispersed throughout the globe and has the potential to be used in membrane and thermal desalination plants. Among renewable energy sources, geothermal comes in third, behind wind and solar. The benefits of this energy source include the stability of performance on different days of the year, as the depth of temperature of the Earth has constant stability and this characteristic makes these systems distinct. Another advantage of this energy is the need for no energy storage systems (Kalogirou Citation2005). In these types of systems, drilling wells of 100 metres or more can achieve optimum temperature and stability for use in desalination systems. There are two common strategies for using these types of energy sources: 1. Thermal extraction between 70 and 90°C and the use of water obtained in MED systems or 2. Electricity generation by steam turbines and using electricity generated in the RO systems; this method is highly applicable for desalination of highly saline waters such as the Persian Gulf. illustrates the desalination methods by named approaches. While in the proposed cycle the cost of freshwater is around 2.2 USD/m3.
Figure 15. The integrated plant comprised of RO, MED, and geothermal well-producing freshwater (Ghaffour et al. Citation2014)
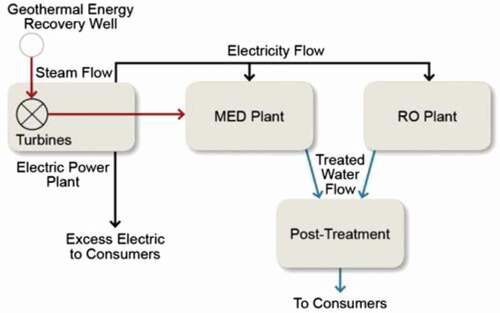
Theoretical and experimental feasibility of integrating geothermal energy plants with various desalination processes have been investigated by different studies (Goosen, Mahmoudi, and Ghaffour Citation2010; Sarbatly and Chiam Citation2013; Tomaszewska and Bodzek Citation2013; Turchi et al. Citation2017). In addition to desalination, it is capable of generating heat, electricity, and cooling by simultaneously connecting CSP, PV, MED, geothermal energy, and lithium bromide chiller. (Mohamed and El-Minshawy Citation2009) compared the cost of desalination for desalination plant driven by various options. In this case, the price was 1.15 US$/m3 when powered by geothermal energy resources. Though this value for powered desalination plant by fossil fuels equalled to 4 US$/m3. Likewise, this cost for MSF-back pressure steam turbine, MSF-gas turbine and waste heat boiler, MSF/TVC with gas turbine and waste heat boiler, and RO-single stage with energy recovery reached 1.57, 1.44, 1.31, and 1.39 US$/m3.
Another available alternative to desalination by renewable energy is wave energy. These systems have a high investment cost and severe fluctuations in power output, which has led to focused attention on modifying these defects (Falcão Citation2014; Foteinis and Tsoutsos Citation2017; Salter et al. Citation2010). One of the studies in this context refers to the use of wave energy by Davis in 2005 with one-metre waves (or more) to produce power for the production of freshwater (Davies Citation2005). The first middle scaled wave energy-power grid system has been mounted in Australia that produced demanded power for freshwater production (Alkaisi, Mossad, and Sharifian-Barforoush Citation2017). The prime example of these systems is located in Garden Island-Australia (Viola et al. Citation2016). The system, which can desalinate seawater (150 m3/day) by RO method, utilises multiple buoys incorporated in CETO equipment and connected to the desalination pilot plant (DPP). The representation of the desired technology is exposed in (Viola et al. Citation2016).
Figure 16. The schematic of an integrated plant including CETO technology and DPP (Viola et al. Citation2016)
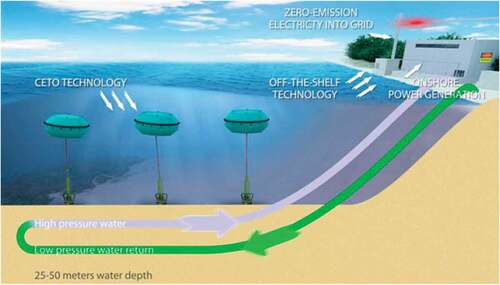
5.3.1. Geothermal and wave potentials in Iran
By locating on the global geothermal belt, Iran is a country with huge potential in this type of energy; while the distribution of numerous warm water streams and volcanoes all over the country are proofs for this point (Ghorashi and Rahimi Citation2011a). The first assessment for geothermal plant construction in Iran started in 1995, where the Sabalan region was selected to be analysed in terms of feasibility and productivity. By drilling three deep wells at a depth of 3197 metres, a maximum temperature of 240°C has been achieved (Bromley, Khosrawi, and Talebi Citation2000). The results of (Noorollahi et al. Citation2009) specified that 8.8% of Iran’s lands as prospected geothermal zones are located in 18 fields. Long-term strategies in this sector caused to the installation of a 50 MW geothermal plant in northern provinces and expecting to reach 200 MW in a near future. In northern parts of Iran several potentially geothermal regions have been explored; namely: Meshkinshahr, Sabalan, Khoy–Maku, and Sarine which their estimated thermal energy is 14.84, 16.48, 30.4, and 16.65 (108 J), each (Ghobadian et al. Citation2009). Yousefi, Ehara, and Noorollahi (Citation2007) examined the regional-scale geothermal resources for future use in Iran by Geographic Information System (GIS) to declare regions with high potential in this regard. Obtained results demonstrated the geothermal prospects in the northern parts of the country. Providing a map of the extent of high geothermal energy density areas can be rewarding in constructing and developing power generation, heating, and desalination systems in the future. In , the regions with geothermal capacity are ranked in descending order. Obviously from the figure, some of the high potential areas are situated in shorelines and have access to seawater for desalination processes. It’s noteworthy to mention that, geothermal energy has a low contribution in energy sectors around the world; though the accessibility, environmentally friendly, and economical specifications (0.04–0.7 $/kW), this type of energy has convinced administrations to focus on that (Jacobson and Delucchi Citation2011; Noorollahi et al. Citation2009). Meanwhile, the charge of geothermal resources will not vary, so the price of electrical power from innovative geothermal power plants fluctuates between 4 and 10 US cents per kWh. Based on , around 9% of Iran is in high-potential geothermal areas. According to , some specific sites, for example, Damavand, Sabalan, Maku-Khoy, Sahand, and Taftan are the locations that have high-potential for generating electrical power. There exist around 82 MWt installed capacity of geothermal direct utilisation in Iran (Lund and Toth Citation2020). Currently, the geothermal hot water wells are used for swimming pools, space-heating, greenhouse-heating, pound-heating, and tourism site utilisations. A great deal of the direct operations are swimming and spas which are reported in 189 sites all over the country for the annual energy use of 1102 TJ/yr with a capacity factor equalled to 0.41.
Figure 17. Regions with high potential in geothermal energy (Yousefi et al. Citation2010)
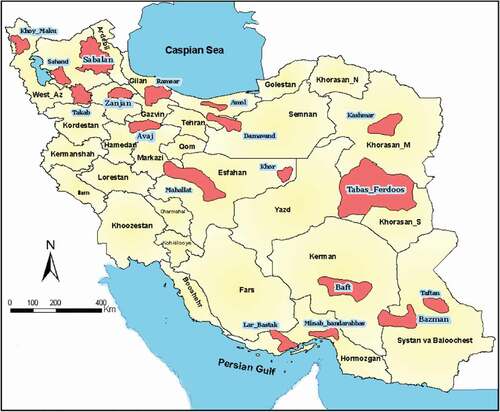
Table 5. High-potentially geothermal regions in Iran (Bahrami and Abbaszadeh Citation2013; Gholamhassan Najafi and Ghobadian Citation2011a)
Figure 18. Wave heights for the southern part of the Caspian Sea (a), Persian Gulf (b), and Gulf of Oman (c) throughout 100-year (Golshani et al. Citation2005; Khojasteh and Kamali Citation2016)
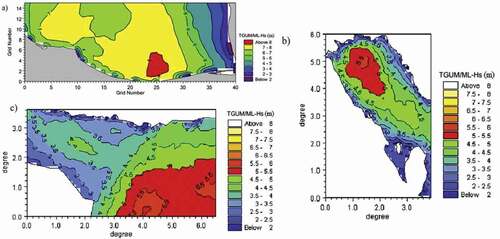
Recently, the Renewable Energy Organization of Iran has proposed a series of country-wide potential examinations to achieve the most appropriate regions for upcoming investment predominantly targeting direct heat uses in the isolated areas. The nationwide geothermal potential investigation correspondingly recommended 10 zones in different regions. They are expected to enjoy sensible potential and are suggested for direct applications (Tofigh and Abedian Citation2016). (Noorollahi, Taghipoor, and Sajadi Citation2017) observed the geothermal energy resources in Iran and provided a short-term explanation of geothermal approaches in this country. They determined that direct exploitation of thermal water is more cost-effective than generating electrical power. Moreover, they surveyed employing abandoned oil wells in the geothermal seawater desalination process (by simulating a geothermal MSF). Outcomes of this research revealed that this advanced method can produce 565 m3/day of freshwater. Based on the conducted researches and energy resources in Iran, geothermal energy doesn’t have a significant influence on Iran’s prospect energy market (Mollahosseini et al. Citation2017; Mostafaeipour and Mostafaeipour Citation2009).
As stated earlier, Iran has access to three large bodies of water that make it possible to use the energy of the waves and tidal; however, this potential has not been used satisfactorily. Wave energy covers the uppermost energy density value between clean energy sources which has the unique specifications that make it a promising option in the renewable energy sector; like stability and environmentally friendly behaviours (S Astariz and Iglesias Citation2016, Citation2015; Iglesias et al. Citation2009). In the north, the Caspian Sea has great amounts of wave power that average and maximum values are 14 kW/m and 30 kW/m, each (Khojasteh et al. Citation2018). Rusu and Onea (Citation2013) investigated the wave energy content in the Caspian Sea and reported that based on the sensorized data, central parts have a great deal of this energy type. Findings indicated that these parts, in average annual conditions, have wave height and average power equal to 3 m and 20 kW/m, while in winter the maximum wave height and power would be reached at 6 m and 100 kW/m. Additionally, Golshani, Taebi, and Chegini (Citation2007) stated that coastal regions in Iran have a high amount of wave energy in the Caspian Sea shoreline. In this field, several studies have been performed for the Caspian Sea coastal line. For Tonekabon (Tavana Citation2009), Mazandaran province (Nejad, Shariati, and Zin Citation2013), Anzali port (Hadadpour et al. Citation2014), and Babolsar (Khojasteh, Khojasteh, and Kamali Citation2016) obtained results represented that the mean wave power would be varied from 9 kW/m to 18 kW/m in different regions. In the south, there is a proper potential of wave energy in the Persian Gulf and the Gulf of Oman, whereas the maximum and average powers in the Persian Gulf islands are 16.6 kW/m and 19 kW/m, respectively. Besides the average value for wave power in Gulf of Oman is at approximately 12.6 (Zabihian and Fung Citation2011). It is evident that the highest wave energy can be attained by higher wave while at peak stages reach to 1.5–6 m for Boushehr, and Assalouyeh in costal line of Persian Gulf (Kamranzad et al. Citation2015). The maximum wave energy flux at the final a selection of locations (ports and islands with higher population) has been reported by Zanous et al. (Citation2019), which in Khark, Kangan, Kish, Lenge, Gheshm and Chabahar regarded value is 28.8, 64.2, 45.2, 45.9, 46 and 42.7 kW/m, respectively. Saket et al. stated that the most monthly average wave energy in southern coastal regions is available in July and August (Saket and Etemad-Shahidi Citation2012). This coincides with the increase in temperature and water consumption, which makes it suitable for use in desalination plants. demonstrated the wave heights for three main bodies of water in Iran over a coarse of 100-year (Golshani et al. Citation2005; Khojasteh and Kamali Citation2016).
5.4. Other types of renewables for desalination
It is valuable to note that other types of renewable energy have the potential to be used in desalination processes, but some of them are limited to laboratory models and are not presented on large commercial scales or are of little use in Iran. For the reason that access to seawater as the main matter and proximity to the sea are essential parameters that must be evaluated in Iran, on the other hand, the evaluation of these types of energy needs further studies. Therefore, this section covers the desalination models utilising other types of renewable energy.
Fuel cells established a groundbreaking process for freshwater production from saline or seawater through no energy consumption and environmental impacts. In this field, microbial desalination cells (MDC) are introduced by the integration of microbial fuel cells (MFC) and electrodialysis (Borjas, Esteve-Núñez, and Ortiz Citation2017). illustrated a simple schematic of a microbial desalination cell for potable water production and electrical power generation in a single cogeneration system. Using MFC or MDC as a bio-electrochemical treatment system for the desalination of synthetic wastewater in addition to power generation has been investigated (Cao et al. Citation2009; Mohanakrishna, Mohan, and Sarma Citation2010; Sevda et al. Citation2015). In this process with the aid of anode and cathode, while the electrical current was generated by existing bacteria on the anode, in the central space between anode and cathode, ionic matters were relocated towards the electrode chambers. At this time, contaminated water would be desalinated in the mid chamber; which has 100% efficiency in removing particles like NaCl. Luo et al. (Citation2012) developed an MDC for municipal wastewater desalination, producing energy, and improving water characterization. Outcomes represented that the MDC has removed 66% of contained salts in the feedwater. The main constrain in desalination efficiency for this method is the voltage produced employing the available bacteria (Mehanna et al. Citation2010).
Figure 19. Schematic of microbial desalination cells (MDC) for desalination purpose (modified from (Borjas, Esteve-Núñez, and Ortiz Citation2017))
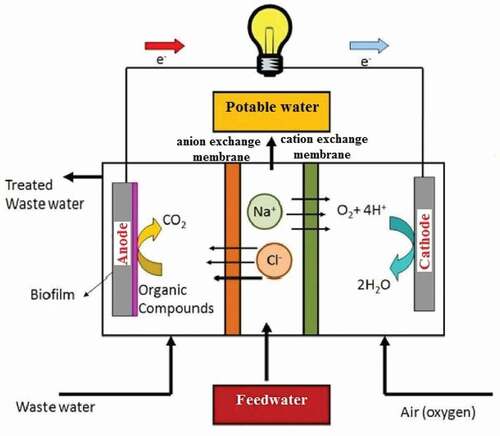
Kokabian and Gude (Citation2013) presented an MDC model based on the passive algae bio-cathodes and the same level of desalination productivity in comparison with common MDC models. By replacing forward osmotic (FO) membrane instead of the anodic membrane, microbial osmotic desalination cell (MODC) would be produced that water flux would be increased while the saltwater turned to diluted state; in addition to increasing the productivity of freshwater production, it will be able to increase the removal of organic matters (Sophia et al. Citation2016; Zhang, Brastad, and He Citation2011). Zhang and He (Citation2013) improved the MODC performance and salt reduction efficiency in the regarded system. Desalination efficiency and organic removal of synthetic seawater by a microbial osmotic fuel cell (MOFC) have reported being 35% and 90%, respectively (Werner et al. Citation2013). (Touati et al. Citation2012) designed a hybrid PV/Fuel Cell for providing demanded power in a desalination plant. The system mechanism is in such an approach that at the solar load peak, the PV is responsible for supplying the electrical power, and at the other times, the fuel cell provides the required power; while 95% of demanded hydrogen is provided by the system itself.
Hosseini et al., (Citation2013b) used solid oxide fuel cell (SOFC) and micro-gas-turbine for freshwater production; while obtained results have shown that the pressure of fuel cell stack has a great influence on the unit capacity. Analysing a coupling of the gas turbines, SOFC, and RO for water purification indicated that the maximum energy destruction belonged to SOFC accounting for 32.9%, while the system water production was 494 m3/h. In another study, a molten carbonate fuel cell integrated with a MED produced 118.944 m3/h freshwaters (Ghorbani, Mehrpooya, and Mousavi Citation2019).
Another energy resource for desalination processes is waste heat. Due to the inefficiencies and heat transfer in thermal components, this type of energy is accessible and free for various applications. Using discharged heat from a diesel engine has been evaluated by Schwantes et al. (Citation2013), that the MD method had 80% efficiency by utilising 1176 kWh from solar energy and waste heat. Ishaq, Dincer, and Naterer (Citation2018) used wasted thermal energy from a glass melting kiln for hydrogen and freshwater production at the rate of 12 g/s and 17.4 kg/s, each. During the purification process, seawater salinity was reduced from 35000 to 450 ppm.
In the energy sector, biofuel and biomass have continuously been a sustainable selection in the renewable energy market. Biomass production is contingent on several elements, like the magnitude and superiority of solid wastes, climates, and environmental conditions (Ahmadi et al. Citation2019). Rajan, Raja, and Marimuthu (Citation2014) proposed a desalination system powered by a biomass heat source. In another investigation, the solar-biomass system achieved to produce 0.02 m3/h freshwater in a novel multi-generation system (Ghasemi, Heidarnejad, and Noorpoor Citation2018). Utilising biomass energy in the HDH desalination system led to freshwater production in the range of 3.5–6.1 kg/h based on the not preheating and preheating supplied air (Rajaseenivasan and Srithar Citation2017). RO desalination method powered by a solar-based integrated system, provided 14.6 and 1500 kg/hr hydrogen and water, each, simultaneously (Siddiqui and Dincer Citation2018). Luo, Jenkins, and Ren (Citation2011) modelled a system for freshwater production and hydrogen generation, concurrently. Considered comprised of microbial electrolysis and desalination cells, with hydrogen production equalled to 1.6 mL/h and desalination efficiency reached up to 95%. Also, Kim et al. used a novel approach by photo-electrochemical desalination device for freshwater and H2 production (Kim et al. Citation2018). The developed model of the trigeneration solar-biomass system for producing freshwater by MEHD system can produce about 2 kg/s freshwater, studied by Sahoo et al. (Citation2017). Biogas fuelled the tri-generation system concept for cooling, power generation, and desalination (HDH) have been established by Jabari et al. (Citation2019). In a similar study, a combined solar-driven gas turbine and ORC have been studied by Hogerwaard, Dincer, and Naterer (Citation2017). In this system, power generation, cooling load, and desalination capacity were 220 kW, 8.5 kW, and 1.5 m3/day, each. shows the associated cycle of biogas-trigeneration for water desalination.
Figure 20. The biogas-trigeneration cycle for freshwater production by HDH (Jabari et al. Citation2019)
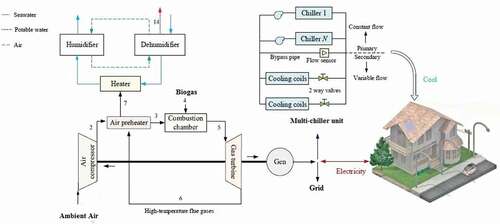
Da Silva et al. carried out cost analyses associated with the implementation of the RO method by different energy sources (Da Silva et al. Citation2018). Based on the outcomes, biogas provides more capacity than solar as energy resources (Abanades et al. Citation2021). However, natural gas is projected to be the most feasible energy source.
6. Economic study
Economic considerations have substantial roles in each system, and desalination plants should not be excluded from this subject. Several elements can have influential behaviours on the cost and desalination technique selection. Numerous researches displayed that investment, operation, and maintenance charges are reliant on the site settings (Balfaqih et al. Citation2017; Darwish et al. Citation2016; Pinto and Marques Citation2017; Shatat, Worall, and Riffat Citation2013a; Younos Citation2005). Researches have revealed that the price of freshwater produced by fossil fuels is lower than renewable energy resources. In general, because of the progress in technology, the price of freshwater production has been dropped, nonetheless, it should be admitted that applying renewable energy increases the charges (Shatat, Worall, and Riffat Citation2013b). provides a group of unit water costs of several desalination processes for comparison. As it’s obvious, the table presents a summary of some cost evaluations of desalination technologies. Obtained results declared the techno-economic of these systems based on their capacity (m3/day) and it’s conspicuous that output rates of plants plays a crucial role. Based on the local geological settings, the most favourable-renewable energy-powered freshwater supply. In this case, demonstrates the operating cost comparison across major desalination technologies for common capacities. It’s apparent from the information provided that MSF has the most capital cost among common desalination technologies for common capacities while RO takes the lead in electrical consumption.
Figure 21. Operating cost comparison across major desalination technologies for common capacities (electricity cost assumed to be 0.05 US$/kWh and an oil price of 60 US$/bbl) (Bernat et al. Citation2010; Ullah and Rasul Citation2019)
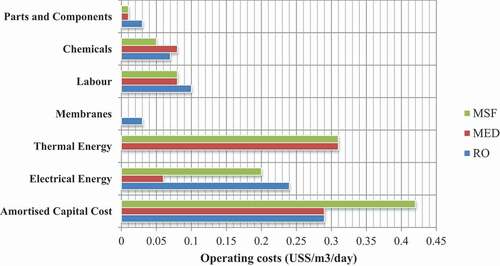
Table 6. Unit costs of freshwater of applied desalination technologies
categorises various settings and consequently exposes the potable water production capacity in Iran. Over 80% of the existing desalination capacity is based on RO.
Table 7. Estimated values of Iran’s renewable energy desalination water production (Mollahosseini et al. Citation2019)
7. Conclusion and prospects
It’s reasonable to surmise that, demands for freshwater in arid and semi-arid areas are growing significantly as available resources are reducing. Seawater desalination is a promising solution for overcoming this crisis in areas with access to the seas or oceans (like Middle East countries). Desalination of seawater is a technique in which a high level of energy is consumed and fossil fuels are used in these areas. However, despite the problems and shortcomings in these energy sources, the shift to the use of renewable energy increases the sustainability of desalination plants. Therefore, the feasibility of using renewable energies based on the potentials of each region can increase the efficiency and sustainability of desalination plants and minimise production costs. The objective of this paper was to study and estimate the conditions of renewable energy use in Iran as a case study. This research investigated Iran’s water backgrounds and renewable energy potentials, moreover to the prospective of RE-powered desalination. The study described proposes that Iran’s potential in renewable energy desalination is further than 28 billion m3 only by solar and wind capabilities. Increased governmental supports, easy access to seawater in the north and south regions, and a high level of renewable energy potential have increased attention in the country to this opportunity. This study investigates solar, wind, geothermal, and wave energies in Iran. Results represented that, the average solar radiation in Iran is about 15.3 kWh/m2/days, which includes more than 2800 hours of radiation per year in central regions. Also, Wind, geothermal and wave energy potentials are equalled to 100 × 106 MW, 200 MW, and 20 kW/m, respectively. Though there is a suitable potential in the Caspian Sea, the Persian Gulf, and Oman Sea coasts to benefit from renewable energy desalination, while these regions suffering from a severe water crisis and can benefit from renewable energies to produce freshwater. Studies on desalination systems indicated that these systems have taken an approach to decrease the involvements of fossil fuel and to grow the efficiency and use of renewable energy in these methods. The followings are overviews of the promising approaches for seawater desalination with renewable energies:
This study evaluated Iran’s potentials for renewable energy desalination. Lots of elements were reviewed in this study ,that is, various methods capacity and charges, current freshwater production capacity and related potential, applicable technologies, etc. Whereas the country enjoys significant potential, which can start Iran’s way out from water scarcity.
In Iran, there can be a production capacity of potable water of more than 28 billion m3. The approximation is still hopeful by way of the presence of many obstacles to overcome. There are countless unrevealed regions like transportation organisations and pipelines. There exist various risk factors to be studied relative to each area of the country. The challenges for captivating suitable investment in renewable energy desalination might be an additional serious concern for Iran as the outlays of considered projects are considerably dissimilar from other conventional water treatment plants.
Applying solar energy to assist desalination plants is turning a dependable and techno-economically developed substitute to the common units and can be an encouraging supportable way out which might operate both to responding water inadequacy issues and reducing carbon productions, particularly in areas with great potentials of solar energy.
One of the new and advanced methods of seawater desalination is ocean thermocline systems that produce desalinated water using the temperature difference between surface water (high temperature) and deep part of the sea (low temperature) (Ng and Shahzad Citation2018). The development of renewable energy-based cogeneration systems provides the potential to improve freshwater extraction from freeze desalination (FD) systems (Kalista et al. Citation2018).
Achieving sustainability in the issues involved in the desalination process will allow for further development in the use of renewable energy. Sustainability of energy, economic and environmental sectors, including freshwater production at the lowest cost, environmental impacts, and energy consumption, while the integration of desalination plants with renewable energy systems, provides these objectives. Using bio-organic molecules in the materials of membranes is a novel solution in the field (Ridgway, Orbell, and Gray Citation2017).
Sea pretreatments by bio-filters for improving water production and organic matter reduction in desalination plants have been considered (Naidu et al. Citation2013). Introducing new and optimal methods in the pretreatment section can intensify the effectiveness of the desalination systems.
The use of hybrid energy systems in desalination plants increases the efficiency and production of freshwater, by achieving sustainability and reducing production costs. The solar-powered FO and evaporation are a process based on using saturated steam as a carrier gas for vaporising seawater and producing freshwater by solar energy (Ahmed, Hashaikeh, and Hilal Citation2019).
The introduction of energy storage systems to gain assistance from the absence of renewable energy improves system performance and reduces volatilities in operating life. Reference (Gude Citation2015) reviewed various renewable energy resources in the desalination process and their association with energy storage systems, which examined solar ponds, phase change materials (PCM), thermal energy storage (TES), solar stills, and waste heats. With the help of experimental tests, the solar-waste heat system could produce 0.05 m3/d freshwater by 130–180 MJ/day thermal energy from waste heat and 15–29 MJ/m2/day by solar radiations (Elminshawy, Siddiqui, and Sultan Citation2015).
More researches and monitoring of methodological characteristics of desalination plant uses are desirable. This might increase the efficiency of these technologies. Desalination plants are moderately costly technology and cannot be suitable for all areas. Economic investigations of desalination plant applications are required for sustainable development. Improvements ineffective disposal methods of brine are desired. Continuous environmental impact evaluations of desalination units are essential to avoid upcoming problems. Other water resources, for example, waste-water reuse should be compared to desalination plants in Iran.
This study could contain an all-inclusive project design process of renewable energy desalination plants with optimum methods optimisation in terms of both technical and economical (energy and cost). Present research can stimulate the involvement of government, policy-makers, and investors in renewable energy desalination plants.
Nomenclature
bbl | = | barrel of oil |
CA | = | Cellulose Acetate |
CSP | = | Concentrated solar power |
DCC | = | Direct capital cost |
DEIM | = | Department of Energy, Information Engineering and Mathematical Models |
DPP | = | Desalination Pilot Plant |
DPR | = | Direct potable reuse |
DWEER | = | Dual Work Exchanger Energy |
ED | = | Electrodialysis |
EDI | = | Electrodeionization |
EDR | = | Electrodialysis reversal |
EIA | = | Environmental Impact Assessment |
EL | = | Electrolysis |
FC | = | Fuel cells |
FO | = | Forward osmosis |
GHG | = | Greenhouse Gas |
HYB | = | Hybrid |
IAEA | = | International Atomic Energy Agency |
IDA | = | International Desalination Association |
IDA | = | International desalination association |
IPR | = | Indirect Potable Reuse |
MFC | = | Microbial fuel cell |
MDC | = | Microbial desalination cell |
MD | = | Membrane distillation |
MED | = | Multi-Effect Distillation |
MSF | = | Multi-Stage Flash |
MVC | = | Mechanical Vapor Compression |
NF | = | Nanofiltration |
OECD | = | Organization for Economic Co-operation and Development |
OER | = | Osmotic energy recovery |
PV | = | Photovoltaic |
RE | = | Renewable Energy |
RO | = | Reverse osmosis |
SHDD | = | Solar humidification and dehumidification desalination |
SWRO | = | Seawater Reverse osmosis |
TCC | = | Total capital cost |
TDS | = | Total dissolved solids |
TFC | = | Thin film composite |
TVC | = | Thermal Vapor Compression |
VP | = | Vapor compression |
WHO | = | World Health Organization |
WSI | = | Water stress index |
WWP | = | World Water programme |
WWTP | = | Wastewater Treatment Plant |
Disclosure statement
The authors declare that they have no known competing financial interests or personal relationships that could have appeared to influence the work reported in this paper.
Additional information
Notes on contributors
Farbod Esmaeilion
Farbod Esmaeilion got his Bachelor's and Master's from the ArakUT and IUST. He has a good background in Mechanical Engineering and Energy area with a passion and expertise in Thermodynamics, Renewable Energies, HVAC, CCHP, and Desalination.
Abolfazl Ahmadi
Abolfazl Ahmadi is an academic staff in the Department of Energy systems engineering (School of Advanced Technologies (New Technologies), Iran University of Science and Technology) since 1992. He also obtained his Ph.D. in Mechanical Engineering from Sheffield University in the UK. His research interests are in energy systems optimization.
Siamak Hoseinzadeh
Siamak Hoseinzadeh Experienced Teacher with a demonstrated history of working in the nanotechnology industry. Skilled in Science Education, Nanomaterials, Coaching, Microsoft Word, and Scanning Electron Microscopy. Strong education professional with a Doctor of Philosophy (Ph.D.) focused in Mechanical Engineering from Islamic Azad University.
Mehdi Aliehyaei
Mehdi Aliehyaei is an associate professor of Pardis Branch, Islamic Azad University. He received his Ph.D. in mechanical engineering from the Sharif University of Technology in 2007 (First rank: GPA 19.13/20) and his postdoc from Ontario university in 2010. FOR 92% OF HIS PUBLICATIONS HE DID A MATHEMATICAL MODELING AND COMPUTER SIMULATION. Research interest: Renewable energy Exergy analysis; Dispersed power generation Advanced and extended exergy analyses; Fuel cell; Thermal cycle.
Seyed Ali Makkeh
Seyed Ali Makkeh Research interest: Renewable energy Exergy analysis; Dispersed power generation Advanced and extended exergy analyses; Fuel cell; Thermal cycle.
Davide Astiaso Garcia
Davide Astiaso Garcia PhD in Thermal Science, Energy Technology and Building Physics in 2011, is Assistant Professor of Thermal Sciences, Energy Technology and Building Physics at the Department of Planning, Design, and Technology of Architecture (DPDTA) of the Sapienza University of Rome.
References
- Abanades, S., H. Abbaspour, A. Ahmadi, B. Das, M. A. Ehyaei, F. Esmaeilion, M. El Haj Assad, et al. 2021. “A Critical Review of Biogas Production and Usage with Legislations Framework across the Globe.” International Journal of Environmental Science and Technology. doi:https://doi.org/10.1007/s13762-021-03301-6.
- Abdolzadeh, M., and R. Nikkhah. 2019. “Experimental Study of Dust Deposition Settled over Tilted PV Modules Fixed in Different Directions in the Southeast of Iran.” Environmental Science and Pollution Research 26 (30): 31478–31490. doi:https://doi.org/10.1007/s11356-019-06246-z.
- Abrams, R. H. 2018. Legal Control of Water Resources Cases and Materials. Faculty Books and Book Contributions 33. West Academic. https://commons.law.famu.edu/faculty-books/33
- Aghahosseini, A., D. Bogdanov, N. Ghorbani, and C. Breyer. 2018. “Analysis of 100% Renewable Energy for Iran in 2030: Integrating Solar PV, Wind Energy and Storage.” International Journal of Environmental Science and Technology 15 (1): 17–36.
- Ahmadi, A., F. Esmaeilion, A. Esmaeilion, M. A. Ehyaei, and J. L. Silveira. 2019. “Benefits and Limitations of Waste-to-energy Conversion in Iran.” Renewable Energy Research and Application. doi:https://doi.org/10.22044/rera.2019.8666.1007.
- Ahmadi, A., M. A. Ehyaei, D. H. Jamali, M. Despotovic, F. Esmaeilion, A. Abdalisousan, and E. B. Hani. 2021. “Energy, Exergy, and Economic Analyses of Integration of Heliostat Solar Receiver to Gas and Air Bottom Cycles.” Journal of Cleaner Production 280: 124322. doi:https://doi.org/10.1016/j.jclepro.2020.124322.
- Ahmed, F. E., R. Hashaikeh, and N. Hilal. 2019. “Solar Powered desalination–Technology, Energy and Future Outlook.” Desalination 453: 54–76.
- Alamdari, P., O. Nematollahi, and A. A. Alemrajabi. 2013. “Solar Energy Potentials in Iran: A Review.” Renewable and Sustainable Energy Reviews 21: 778–788.
- Alamdari, P., O. Nematollahi, and M. Mirhosseini. 2012. “Assessment of Wind Energy in Iran: A Review.” Renewable and Sustainable Energy Reviews 16 (1): 836–860.
- Al-Jasser, A. O. 2007. “Chlorine Decay in Drinking-water Transmission and Distribution Systems: Pipe Service Age Effect.” Water Research 41 (2): 387–396.
- Alkaisi, A., R. Mossad, and A. Sharifian-Barforoush. 2017. “A Review of the Water Desalination Systems Integrated with Renewable Energy.” Energy Procedia 110: 268–274.
- Al-Othman, A., M. Tawalbeh, M. E. H. Assad, T. Alkayyali, and A. Eisa. 2018. “Novel Multi-stage Flash (MSF) Desalination Plant Driven by Parabolic Trough Collectors and A Solar Pond: A Simulation Study in UAE.” Desalination 443: 237–244.
- Amy, G., N. Ghaffour, Z. Li, L. Francis, R. V. Linares, T. Missimer, and S. Lattemann. 2017. “Membrane-based Seawater Desalination: Present and Future Prospects.” Desalination 401: 16–21.
- Ashok, S. 2007. “Optimised Model for Community-based Hybrid Energy System.” Renewable Energy 32 (7): 1155–1164.
- Askari, I. B., M. Ameri, and F. Calise. 2018. “Energy, Exergy and Exergo-economic Analysis of Different Water Desalination Technologies Powered by Linear Fresnel Solar Field.” Desalination 425: 37–67.
- Askarzadeh, A. 2013. “A Discrete Chaotic Harmony Search-based Simulated Annealing Algorithm for Optimum Design of PV/wind Hybrid System.” Solar Energy 97: 93–101.
- Astariz, S., and G. Iglesias. 2015. “The Economics of Wave Energy: A Review.” Renewable and Sustainable Energy Reviews 45: 397–408.
- Astariz, S., and G. Iglesias. 2016. “Selecting Optimum Locations for Co-located Wave and Wind Energy Farms. Part I: The Co-Location Feasibility Index.” Energy Conversion and Management 122: 589–598.
- Awan, A. B., and Z. A. Khan. 2014. “Recent Progress in Renewable energy–Remedy of Energy Crisis in Pakistan.” Renewable and Sustainable Energy Reviews 33: 236–253.
- Bahrami, M., and P. Abbaszadeh. 2013. “An Overview of Renewable Energies in Iran.” Renewable and Sustainable Energy Reviews 24: 198–208.
- Bakhoda, H., M. Almassi, N. Moharamnejad, R. Moghaddasi, and M. Azkia. 2012. “Energy Production Trend in Iran and Its Effect on Sustainable Development.” Renewable and Sustainable Energy Reviews 16 (2): 1335–1339.
- Bakhshi-Jafarabadi, R., J. Sadeh, and M. Dehghan. 2020. “Economic Evaluation of Commercial Grid-connected Photovoltaic Systems in the Middle East Based on Experimental Data: A Case Study in Iran.” Sustainable Energy Technologies and Assessments 37: 100581.
- Balfaqih, H., M. T. Al-Nory, Z. M. Nopiah, and N. Saibani. 2017. “Environmental and Economic Performance Assessment of Desalination Supply Chain.” Desalination 406: 2–9.
- Barron, O. 2006. Desalination Options and Their Possible Implementation in Western Australia: Potential Role for CSIRO Land and Water. Canberra: CSIRO: Water for a Healthy Country National Research Flagship; 2006. http://hdl.handle.net/102.100.100/176100?index=1
- Bennett, A. 2015. “Developments in Desalination and Water Reuse.” Filtration+ Separation 52 (4): 28–33.
- Bernat, X., O. Gibert, R. Guiu, J. Tobella, and C. Campos. 2010. “The Economics of Desalination for Various Uses.” Re-Thinking Water and Food Security vol 1: 349–372. doi:https://doi.org/10.1201/b10541-27.
- Besarati, S. M., R. V. Padilla, D. Y. Goswami, and E. Stefanakos. 2013. “The Potential of Harnessing Solar Radiation in Iran: Generating Solar Maps and Viability Study of PV Power Plants.” Renewable Energy 53: 193–199.
- Borjas, Z., A. Esteve-Núñez, and J. M. Ortiz. 2017. “Strategies for Merging Microbial Fuel Cell Technologies in Water Desalination Processes: Start-up Protocol and Desalination Efficiency Assessment.” Journal of Power Sources 356: 519–528.
- Borsani, R., and S. Rebagliati. 2005. “Fundamentals and Costing of MSF Desalination Plants and Comparison with Other Technologies.” Desalination 182 (1–3): 29–37.
- Brauns, E. 2010. “An Alternative Hybrid Concept Combining Seawater Desalination, Solar Energy and Reverse Electrodialysis for a Sustainable Production of Sweet Water and Electrical Energy.” Desalination and Water Treatment 13 (1–3): 53–62.
- Bromley, C., K. Khosrawi, and B. Talebi. 2000. “Geophysical Exploration of Sabalan Geothermal Prospects in Iran.” In Proceedings of the World Geothermal Congress. Japan.
- Caldera, U., D. Bogdanov, and C. Breyer. 2016. “Local Cost of Seawater RO Desalination Based on Solar PV and Wind Energy: A Global Estimate.” Desalination 385: 207–216.
- Cao, X., X. Huang, P. Liang, K. Xiao, Y. Zhou, X. Zhang, and B. E. Logan. 2009. “A New Method for Water Desalination Using Microbial Desalination Cells.” Environmental Science & Technology 43 (18): 7148–7152.
- CDM–ExecutiveBoard. 2014. Implementation of Co-generation Plant for Production of Potable Water in Qeshm Island. Germany/Clean Development Mechanism (CDM).
- Chandrashekara, M., and A. Yadav. 2017. “Water Desalination System Using Solar Heat: A Review.” Renewable and Sustainable Energy Reviews 67: 1308–1330.
- Christ, A., B. Rahimi, K. Regenauer-Lieb, and H. T. Chua. 2017. “Techno-economic Analysis of Geothermal Desalination Using Hot Sedimentary Aquifers: A Pre-feasibility Study for Western Australia.” Desalination 404: 167–181.
- Colangelo, G., E. Favale, P. Miglietta, and A. de Risi. 2016. “Innovation in Flat Solar Thermal Collectors: A Review of the Last Ten Years Experimental Results.” Renewable and Sustainable Energy Reviews 57: 1141–1159.
- Cotruvo, J., N. Voutchkov, J. Fawell, P. Payment, D. Cunliffe, and S. Lattemann. 2010. Desalination Technology: Health and Environmental Impacts. United States: CRC Press.
- Da Silva, W. F., I. F. S. dos Santos, M. C. C. de Oliveira Botan, A. P. M. Silva, and R. M. Barros. 2018. “Reverse Osmosis Desalination Plants in Brazil: A Cost Analysis Using Three Different Energy Sources.” Sustainable Cities and Society 43: 134–143.
- Darwish, M. A., H. K. Abdulrahim, A. S. Hassan, and A. A. Mabrouk. 2016. “PV and CSP Solar Technologies & Desalination: Economic Analysis.” Desalination and Water Treatment 57 (36): 16679–16702.
- Davies, P. A. 2005. “Wave-powered Desalination: Resource Assessment and Review of Technology.” Desalination 186 (1–3): 97–109.
- DeZuane, J. 1997. Handbook of Drinking Water Quality. Canada: John Wiley & Sons.
- El Saliby, I., Y. Okour, H. K. Shon, J. Kandasamy, and I. S. Kim. 2009. “Desalination Plants in Australia, Review and Facts.” Desalination 247 (1–3): 1–14.
- El-Ghonemy, A. M. K. 2012. “Future Sustainable Water Desalination Technologies for the Saudi Arabia: A Review.” Renewable and Sustainable Energy Reviews 16 (9): 6566.
- Elminshawy, N. A. S., F. R. Siddiqui, and G. I. Sultan. 2015. “Development of a Desalination System Driven by Solar Energy and Low Grade Waste Heat.” Energy Conversion and Management 103: 28–35.
- Eltawil, M. A., Z. Zhengming, and L. Yuan. 2009. “A Review of Renewable Energy Technologies Integrated with Desalination Systems.” Renewable and Sustainable Energy Reviews 13 (9): 2245–2262.
- Emami, M., G. H. J. Ghajar, and N. Kaynia. 1977. “State of Desalination Projects in Iran.” Desalination 23 (1–3): 465–470.
- Energy, U. R. 2012. Water Desalination Using Renewable Energy. Iran: IRENA.
- Esmaeilion, F. 2020. “Hybrid Renewable Energy Systems for Desalination.” Applied Water Science 10 (3): 84. doi:https://doi.org/10.1007/s13201-020-1168-5.
- Esmaeilion, F., A. Ahmadi, A. Esmaeilion, and M. A. Ehyaei. 2020. “The Performance Analysis and Monitoring of Grid-connected Photovoltaic Power Plant.” Current Chinese Computer Science 1: 1. doi:https://doi.org/10.2174/2665997201999200511083228.
- Esmaeilion, F., A. Ahmadi, and M. A. Ehyaei. 2021. “Low-grade Heat from Solar Ponds: Trends, Perspectives, and Prospects.” International Journal of Ambient Energy. doi:https://doi.org/10.1080/01430750.2021.1938672.
- Esmaeilion, F., A. Ahmadi, and R. Dashti. 2021. “Exergy-Economic-Environment Optimization of the Waste-to-Energy Power Plant Using Multi-Objective Particle-Swarm Optimization (MOPSO).” Scientia Iranica. doi:https://doi.org/10.24200/SCI.2021.55633.4323.
- Fadai, D. 2007. “The Feasibility of Manufacturing Wind Turbines in Iran.” Renewable and Sustainable Energy Reviews 11 (3): 536–542.
- Falcão, A. F. O. 2014. Modelling of Wave Energy Conversion. Lisbon, Portugal: Instituto Superior Técnico, Universidade Técnica de Lisboa.
- Faramarzi, M. 2010. Assessment of Regional Water Endowments, Crop Water Productivity, and Implications for Intra-country Virtual Water Trade in Iran. Zürich- Switzerland: ETH Zurich.
- Faramarzi, M., K. C. Abbaspour, R. Schulin, and H. Yang. 2009. “Modelling Blue and Green Water Resources Availability in Iran.” Hydrological Processes: An International Journal 23 (3): 486–501.
- Foster, R., M. Ghassemi, and A. Cota. 2009. Solar Energy: Renewable Energy and the Environment. United States: CRC Press.
- Foteinis, S., and T. Tsoutsos. 2017. “Strategies to Improve Sustainability and Offset the Initial High Capital Expenditure of Wave Energy Converters (Wecs).” Renewable and Sustainable Energy Reviews 70: 775–785.
- Fried, A., and B. Serio. 2012. Water Industry Segment Report Desalination. San Diego: World Trade Center San Diego (WTCSD).
- Ganesh, I. 2015. “Solar Fuels Vis-a-vis Electricity Generation from Sunlight: The Current State-of-the-art (A Review).” Renewable and Sustainable Energy Reviews 44: 904–932.
- Ghaffour, N., S. Lattemann, T. Missimer, K. C. Ng, S. Sinha, and G. Amy. 2014. “Renewable Energy-driven Innovative Energy-efficient Desalination Technologies.” Applied Energy 136: 1155–1165.
- Ghasemi, A., P. Heidarnejad, and A. Noorpoor. 2018. “A Novel Solar-biomass Based Multi-generation Energy System Including Water Desalination and Liquefaction of Natural Gas System: Thermodynamic and Thermoeconomic Optimization.” Journal of Cleaner Production 196: 424–437.
- Ghobadian, B., G. Najafi, H. Rahimi, and T. F. Yusaf. 2009. “Future of Renewable Energies in Iran.” Renewable and Sustainable Energy Reviews 13 (3): 689–695.
- Ghorashi, A. H., and A. Rahimi. 2011a. “Renewable and Non-renewable Energy Status in Iran: Art of Know-how and Technology-gaps.” Renewable and Sustainable Energy Reviews 15 (1): 729–736. doi:https://doi.org/10.1016/j.rser.2010.09.037.
- Ghorbani, B., M. Mehrpooya, and S. A. Mousavi. 2019. “Hybrid Molten Carbonate Fuel Cell Power Plant and Multiple-effect Desalination System.” Journal of Cleaner Production 220: 1039–1051.
- Gielen, D., F. Boshell, D. Saygin, M. D. Bazilian, N. Wagner, and R. Gorini. 2019. “The Role of Renewable Energy in the Global Energy Transformation.” Energy Strategy Reviews 24: 38–50.
- Gilau, A. M., and M. J. Small. 2008. “Designing Cost-effective Seawater Reverse Osmosis System under Optimal Energy Options.” Renewable Energy 33 (4): 617–630.
- Global Wind Atlas. 2020. Mean Wind Speed Map, Islamic Republic of Iran. https://globalwindatlas.info/
- Gohari, A., S. Eslamian, A. Mirchi, J. Abedi-Koupaei, A. M. Bavani, and K. Madani. 2013. “Water Transfer as a Solution to Water Shortage: A Fix that Can Backfire.” Journal of Hydrology 491: 23–39.
- Gökçek, M., and Ö. B. Gökçek. 2016. “Technical and Economic Evaluation of Freshwater Production from a Wind-powered Small-scale Seawater Reverse Osmosis System (WP-SWRO).” Desalination 381: 47–57.
- Golshani, A., S. Taebi, and V. Chegini. 2007. “Wave Hindcast and Extreme Value Analysis for the Southern Part of the Caspian Sea.” Coastal Engineering Journal 49 (4): 443–459.
- Golshani, A. A., A. Nakhaei, S. Taebi, V. Chegini, and A. V. Jandaghi. 2005. Wave Hindcast Study of the Caspian Sea. Iran/JOURNAL OF MARINE ENGINEERING.
- Gomar, Z., H. Heidary, and M. Davoudi. 2011. “Techno-economics Study to Select Optimum Desalination Plant for Asalouyeh Combined Cycle Power Plant in Iran.” World Academy of Science, Engineering and Technology 1: 51.
- Goosen, M., H. Mahmoudi, and N. Ghaffour. 2010. “Water Desalination Using Geothermal Energy.” Energies 3 (8): 1423–1442.
- Gorjian, S., and B. Ghobadian. 2015. “Solar Desalination: A Sustainable Solution to Water Crisis in Iran.” Renewable and Sustainable Energy Reviews 48: 571–584.
- Gorjian, S., T. Tavakkoli Hashjin, B. Ghobadian, and A. Banakar. 2013. “Modeling Global Solar Radiation over Iran Based on Meteorological Data Using ANN Technique.” In 11th Iranian Conference on Intelligent Systems (ICIS2013), Iran, 1–8.
- Gorjian, S., T. T. Hashjin, and B. Ghobadian. 2011. “Seawater Desalination Using Solar Thermal Technologies: State of the Art.” In Proceedings of the 10th International Conference on Sustainable Energy Technologies, Istanbul-Turkey, 4–7.
- Grubert, E. A., A. S. Stillwell, and M. E. Webber. 2014. “Where Does Solar-aided Seawater Desalination Make Sense? A Method for Identifying Sustainable Sites.” Desalination 339: 10–17.
- Gude, V. G. 2015. “Energy Storage for Desalination Processes Powered by Renewable Energy and Waste Heat Sources.” Applied Energy 137: 877–898.
- Gude, V. G. 2016. “Geothermal Source Potential for Water desalination–Current Status and Future Perspective.” Renewable and Sustainable Energy Reviews 57: 1038–1065.
- Gude, V. G., and N. Nirmalakhandan. 2010. “Sustainable Desalination Using Solar Energy.” Energy Conversion and Management 51 (11): 2245–2251.
- GWEC, G. W. E. C. 2017. Global Wind Report. 2015. Brussels: GWEC.
- Hadadpour, S., A. Etemad-Shahidi, E. Jabbari, and B. Kamranzad. 2014. “Wave Energy and Hot Spots in Anzali Port.” Energy 74: 529–536.
- He, W., Y. Wang, and M. H. Shaheed. 2015. “Stand-alone Seawater RO (Reverse Osmosis) Desalination Powered by PV (Photovoltaic) and PRO (Pressure Retarded Osmosis).” Energy 86: 423–435.
- Heshmatzadeh, M. 2000. Iran and Oil Sociology of Political Sociology of Oil in Iran. Tehran: Recognition Center of Islam and Iran’s.
- Heydari, M., F. Othman, and M. Noori. 2013. “A Review of the Environmental Impact of Large Dams in Iran.” International Journal of Advancements Civil Structural and Environmental Engineering, IJACSE 1 (1): 4.
- Hoang, M., B. Bolto, C. Haskard, O. Barron, S. Gray, and G. Leslie. 2009. Desalination in Australia. Australia: Commonwealth Scientific and Industrial Research Organisation (CSIRO).
- Hogerwaard, J., I. Dincer, and G. F. Naterer. 2017. “Solar Energy Based Integrated System for Power Generation, Refrigeration and Desalination.” Applied Thermal Engineering 121: 1059–1069.
- Hosseini, M., I. Dincer, P. Ahmadi, H. B. Avval, and M. Ziaasharhagh. 2013a. “Thermodynamic Modelling of an Integrated Solid Oxide Fuel Cell and Micro Gas Turbine System for Desalination Purposes.” International Journal of Energy Research 37 (5): 426–434.
- Hosseini, R., M. Soltani, and G. Valizadeh. 2005. “Technical and Economic Assessment of the Integrated Solar Combined Cycle Power Plants in Iran.” Renewable Energy 30 (10): 1541–1555.
- Hosseini, S. E., A. M. Andwari, M. A. Wahid, and G. Bagheri. 2013b. “A Review on Green Energy Potentials in Iran.” Renewable and Sustainable Energy Reviews 27: 533–545.
- Iglesias, G., M. López, R. Carballo, A. Castro, J. A. Fraguela, and P. Frigaard. 2009. “Wave Energy Potential in Galicia (NW Spain).” Renewable Energy 34 (11): 2323–2333.
- Intelligence, G. W., I. D. A. D. Yearbook, G. W. Summit, and R. Card. 2011. “Global Water Intelligence.” Global Water Intelligence 12 (10): 1–72.
- International Energy Agency. 2020. Produces Electricity by Renewable Resources . www.iea.org
- Iran Foreign Policy & Government Guide, Vol. 1348. USA: International Business Publications; 2006. n.d.
- Ishaq, H., I. Dincer, and G. F. Naterer. 2018. “New Trigeneration System Integrated with Desalination and Industrial Waste Heat Recovery for Hydrogen Production.” Applied Thermal Engineering 142: 767–778.
- Ismail, T. M., A. K. Azab, M. A. Elkady, and M. M. A. Elnasr. 2016. “Theoretical Investigation of the Performance of Integrated Seawater Desalination Plant Utilizing Renewable Energy.” Energy Conversion and Management 126: 811–825.
- Jabari, F., B. Mohammadi-ivatloo, H. Ghaebi, and M.-B. Bannae-sharifian. 2019. “Biogas Fueled Combined Cooling, Desalinated Water and Power Generation Systems.” Journal of Cleaner Production 219: 906–924.
- Jacobson, M. Z., and M. A. Delucchi. 2011. “Providing All Global Energy with Wind, Water, and Solar Power, Part I: Technologies, Energy Resources, Quantities and Areas of Infrastructure, and Materials.” Energy Policy 39 (3): 1154–1169.
- Jiménez Cisneros, B.E., T. Oki, N.W. Arnell, G. Benito, J.G. Cogley, P. Döll, T. Jiang, S.S. Mwakalila, Thomas Fischer, Dieter Gerten, Regine Hock, Shinjiro Kanae, Xixi Lu, Luis José Mata, Claudia Pahl-Wostl, Kenneth M. Strzepek, Buda Su, B. van den Hurk. 2014. Freshwater resources. Climate Change 2014: Impacts, Adaptation, and Vulnerability. Part A: Global and Sectoral Aspects. Contribution of Working Group II to the Fifth Assessment Report of the Intergovernmental Panel on Climate Change : 229–269. ScholarBank@NUS Repository
- Jones, E., M. Qadir, M. T. H. van Vliet, V. Smakhtin, and S. Kang. 2019. “The State of Desalination and Brine Production: A Global Outlook.” Science of the Total Environment 657: 1343–1356.
- Jung, D., D. Kang, M. Kang, and B. Kim. 2015. “Real-time Pump Scheduling for Water Transmission Systems: Case Study.” KSCE Journal of Civil Engineering 19 (7): 1987–1993.
- Kalista, B., H. Shin, J. Cho, and A. Jang. 2018. “Current Development and Future Prospect Review of Freeze Desalination.” Desalination 447: 167–181.
- Kalogirou, S. A. 2005. “Seawater Desalination Using Renewable Energy Sources.” Progress in Energy and Combustion Science 31 (3): 242–281.
- Kamali, R. K., and S. Mohebinia. 2008. “Experience of Design and Optimization of Multi-effects Desalination Systems in Iran.” Desalination 222 (1–3): 639–645.
- Kamranzad, B., A. Etemad-Shahidi, V. Chegini, and A. Yeganeh-Bakhtiary. 2015. “Climate Change Impact on Wave Energy in the Persian Gulf.” Ocean Dynamics 65 (6): 777–794.
- Karagiannis, I. C., and P. G. Soldatos. 2008. “Water Desalination Cost Literature: Review and Assessment.” Desalination 223 (1–3): 448–456.
- Karami, S., E. Karami, and S. Zand-Parsa. 2017. “Environmental and Economic Appraisal of Agricultural Water Desalination Use in South Iran: A Comparative Study of Tomato Production.” Journal of Applied Water Engineering and Research 5 (2): 91–102.
- Keivani Hafshejani, M., A. Baheri, M. Ojakeh, A. Sedighpour, A. Arad, and S. Choopani. 2012. “Impact of Solar Energy Application on Warming, Health Caring and Pollution Prevention in Iran.” Life Science Journal-Acta Zhengzhou University Overseas Edition 9 (4): 1849–1853.
- Keyhani, A., M. Ghasemi-Varnamkhasti, M. Khanali, and R. Abbaszadeh. 2010. “An Assessment of Wind Energy Potential as a Power Generation Source in the Capital of Iran, Tehran.” Energy 35 (1): 188–201.
- Khalili, A., and J. Rahimi. 2014. “High-resolution Spatiotemporal Distribution of Precipitation in Iran: A Comparative Study with Three Global-precipitation Datasets.” Theoretical and Applied Climatology 118 (1–2): 211–221.
- Khan, J., and M. H. Arsalan. 2016. “Solar Power Technologies for Sustainable Electricity generation–A Review.” Renewable and Sustainable Energy Reviews 55: 414–425.
- Khodadadi, M., A. H. Mahvi, M. T. Ghaneian, M. H. Ehrampoush, H. Dorri, and L. Rafati. 2016. “The Role of Desalination in Removal of the Chemical, Physical and Biological Parameters of Drinking Water (A Case Study of Birjand City, Iran).” Desalination and Water Treatment 57 (53): 25331–25336.
- Khojasteh, D., D. Khojasteh, and R. Kamali. 2016. “Wave Energy Absorption by Heaving Point Absorbers at Caspian Sea.” In 24th Annual International Conference on Mechanical Engineering-ISME. Yazd, Iran.
- Khojasteh, D., D. Khojasteh, R. Kamali, A. Beyene, and G. Iglesias. 2018. “Assessment of Renewable Energy Resources in Iran; with a Focus on Wave and Tidal Energy.” Renewable and Sustainable Energy Reviews 81: 2992–3005.
- Khojasteh, D., and R. Kamali. 2016. “Evaluation of Wave Energy Absorption by Heaving Point Absorbers at Various Hot Spots in Iran Seas.” Energy 109: 629–640.
- Kim, B., R. Kwak, H. J. Kwon, V. S. Pham, M. Kim, B. Al-Anzi, G. Lim, and J. Han. 2016. “Purification of High Salinity Brine by Multi-stage Ion Concentration Polarization Desalination.” Scientific Reports 6: 31850.
- Kim, S., G. Piao, D. S. Han, H. K. Shon, and H. Park. 2018. “Solar Desalination Coupled with Water Remediation and Molecular Hydrogen Production: A Novel Solar Water-energy Nexus.” Energy & Environmental Science 11 (2): 344–353.
- Kokabian, B., and V. G. Gude. 2013. “Photosynthetic Microbial Desalination Cells (Pmdcs) for Clean Energy, Water and Biomass Production.” Environmental Science. Processes & Impacts 15 (12): 2178–2185.
- Kouhikamali, R., and N. Sharifi. 2012. “Experience of Modification of Thermo-compressors in Multiple Effects Desalination Plants in Assaluyeh in IRAN.” Applied Thermal Engineering 40: 174–180.
- Kucera, J. 2019. Desalination: Water from Water. Massachusetts-United States: John Wiley & Sons.
- Latteman, S. 2010. Development of an Environmental Impact Assessment and Decision Support System for Seawater Desalination Plants. United States: CRC press.
- Lewis, N. S. 2015. Introduction: Solar Energy Conversion. United States: ACS Publications.
- Li, C., Y. Goswami, and E. Stefanakos. 2013. “Solar Assisted Sea Water Desalination: A Review.” Renewable and Sustainable Energy Reviews 19: 136–163.
- Li, W., Y. Ling, X. Liu, and Y. Hao. 2017. “Performance Analysis of a Photovoltaic-thermochemical Hybrid System Prototype.” Applied Energy 204: 939–947.
- Liu, L., and Z. Wang. 2009. “The Development and Application Practice of Wind–solar Energy Hybrid Generation Systems in China.” Renewable and Sustainable Energy Reviews 13 (6–7): 1504–1512.
- Lotfalipour, M. R., M. A. Falahi, and M. Bastam. 2013. “Prediction of CO2 Emissions in Iran Using Grey and ARIMA Models.” International Journal of Energy Economics and Policy 3 (3): 229–237.
- Loutatidou, S., and H. A. Arafat. 2015. “Techno-economic Analysis of MED and RO Desalination Powered by Low-enthalpy Geothermal Energy.” Desalination 365: 277–292.
- Lund, J. W., and A. N. Toth. 2020. “Direct Utilization of Geothermal Energy 2020 Worldwide Review.” Geothermics 1: 101915.
- Luo, H., P. Xu, T. M. Roane, P. E. Jenkins, and Z. Ren. 2012. “Microbial Desalination Cells for Improved Performance in Wastewater Treatment, Electricity Production, and Desalination.” Bioresource Technology 105: 60–66.
- Luo, H., P. E. Jenkins, and Z. Ren. 2011. “Concurrent Desalination and Hydrogen Generation Using Microbial Electrolysis and Desalination Cells.” Environmental Science & Technology 45 (1): 340–344.
- Madani, K. 2014. “Water Management in Iran: What Is Causing the Looming Crisis?” Journal of Environmental Studies and Sciences 4 (4): 315–328.
- Makkeh, S. A., A. Ahmadi, F. Esmaeilion, and M. A. Ehyaei. 2020. “Energy, Exergy and Exergoeconomic Optimization of a Cogeneration System Integrated with Parabolic Trough Collector-wind Turbine with Desalination.” Journal of Cleaner Production, JCLP273: 123122.
- Maleki, A., and A. Askarzadeh. 2014. “Comparative Study of Artificial Intelligence Techniques for Sizing of a Hydrogen-based Stand-alone Photovoltaic/wind Hybrid System.” International Journal of Hydrogen Energy 39 (19): 9973–9984.
- Mazini, M. T., A. Yazdizadeh, and M. H. Ramezani. 2014. “Dynamic Modeling of Multi-effect Desalination with Thermal Vapor Compressor Plant.” Desalination 353: 98–108.
- Mehanna, M., P. D. Kiely, D. F. Call, and B. E. Logan. 2010. “Microbial Electrodialysis Cell for Simultaneous Water Desalination and Hydrogen Gas Production.” Environmental Science & Technology 44 (24): 9578–9583.
- Mezher, T., H. Fath, Z. Abbas, and A. Khaled. 2011. “Techno-economic Assessment and Environmental Impacts of Desalination Technologies.” Desalination 266 (1–3): 263–273.
- Mohamed, A. M. I., and N. A. S. El-Minshawy. 2009. “Humidification–dehumidification Desalination System Driven by Geothermal Energy.” Desalination 249 (2): 602–608.
- Mohammadnejad, M., M. Ghazvini, T. M. I. Mahlia, and A. Andriyana. 2011. “A Review on Energy Scenario and Sustainable Energy in Iran.” Renewable and Sustainable Energy Reviews 15 (9): 4652–4658.
- Mohanakrishna, G., S. V. Mohan, and P. N. Sarma. 2010. “Bio-electrochemical Treatment of Distillery Wastewater in Microbial Fuel Cell Facilitating Decolorization and Desalination along with Power Generation.” Journal of Hazardous Materials 177 (1–3): 487–494.
- Mollahosseini, A., A. Abdelrasoul, S. Sheibany, M. Amini, and S. K. Salestan. 2019. “Renewable Energy-driven Desalination opportunities–A Case Study.” Journal of Environmental Management 239: 187–197.
- Mollahosseini, A., S. A. Hosseini, M. Jabbari, A. Figoli, and A. Rahimpour. 2017. “Renewable Energy Management and Market in Iran: A Holistic Review on Current State and Future Demands.” Renewable and Sustainable Energy Reviews 80: 774–788. doi:https://doi.org/10.1016/j.rser.2017.05.236.
- Mostafaeipour, A. 2010. “Feasibility Study of Offshore Wind Turbine Installation in Iran Compared with the World.” Renewable and Sustainable Energy Reviews 14 (7): 1722–1743.
- Mostafaeipour, A., M. Saidi-Mehrabad, M. Rezaei, and M. Qolipour. 2017. “The Ranking of Southern Ports and Islands of Iran for Seawater Desalination Plants Using ELECTRE III Method.” Journal of Renewable Energy and Environment 4 (2&3): 10–22.
- Mostafaeipour, A., and N. Mostafaeipour. 2009. “Renewable Energy Issues and Electricity Production in Middle East Compared with Iran.” Renewable and Sustainable Energy Reviews 13 (6–7): 1641–1645.
- Motevallian, S., and M. Tabesh. 2011. “Sustainable Development of Urban Water Supply and Distribution Systems in Iran: Challenges and Opportunities.” In Proceedings of the International Conference on Water & Wastewater, United States, 1–8.
- Mousavi, S. M., M. B. Ghanbarabadi, and N. B. Moghadam. 2012. “The Competitiveness of Wind Power Compared to Existing Methods of Electricity Generation in Iran.” Energy Policy 42: 651–656.
- Nagaraj, R., D. Thirugnanamurthy, M. M. Rajput, and B. K. Panigrahi. 2016. “Techno-economic Analysis of Hybrid Power System Sizing Applied to Small Desalination Plants for Sustainable Operation.” International Journal of Sustainable Built Environment 5 (2): 269–276.
- Naidu, G., S. Jeong, S. Vigneswaran, and S. A. Rice. 2013. “Microbial Activity in Biofilter Used as a Pretreatment for Seawater Desalination.” Desalination 309: 254–260.
- Najafi, G., and B. Ghobadian. 2011a. “Geothermal Resources in Iran: The Sustainable Future.” Renewable and Sustainable Energy Reviews 15 (8): 3946–3951.
- Najafi, G., and B. Ghobadian. 2011b. “LLK1694-wind Energy Resources and Development in Iran.” Renewable and Sustainable Energy Reviews 15 (6): 2719–2728.
- Najafi, G., B. Ghobadian, R. Mamat, T. Yusaf, and W. H. Azmi. 2015. “Solar Energy in Iran: Current State and Outlook.” Renewable and Sustainable Energy Reviews 49: 931–942.
- Negewo, B. D. 2012. Renewable Energy Desalination: An Emerging Solution to Close the Water Gap in the Middle East and North Africa. United States: World Bank Publications.
- Nejad, M. F., O. Shariati, and A. A. B. M. Zin. 2013. “Feasibility Study of Wave Energy Potential in Southern Coasts of Caspian Sea in Iran.” In 2013 IEEE 7th International Power Engineering and Optimization Conference (PEOCO), Langkawi, Malaysia, 57–60.
- Ng, K. C., and M. W. Shahzad. 2018. “Sustainable Desalination Using Ocean Thermocline Energy.” Renewable and Sustainable Energy Reviews 82: 240–246.
- Noorollahi, Y., H. Yousefi, R. Itoi, and S. Ehara. 2009. “Geothermal Energy Resources and Development in Iran.” Renewable and Sustainable Energy Reviews 13 (5): 1127–1132.
- Noorollahi, Y., M. S. Shabbir, A. F. Siddiqi, L. K. Ilyashenko, and E. Ahmadi. 2019. “Review of Two Decade Geothermal Energy Development in Iran, Benefits, Challenges, and Future Policy.” Geothermics 77: 257–266.
- Noorollahi, Y., S. Taghipoor, and B. Sajadi. 2017. “Geothermal Sea Water Desalination System (GSWDS) Using Abandoned Oil/gas Wells.” Geothermics 67: 66–75.
- Norouzi, M., M. Yeganeh, and T. Yusaf. 2021. “Landscape Framework for the Exploitation of Renewable Energy Resources and Potentials in Urban Scale (Case Study: Iran).” Renewable Energy 163: 300–319.
- Ortega-Delgado, B., L. Garcia-Rodriguez, and D.-C. Alarcón-Padilla. 2017. “Opportunities of Improvement of the MED Seawater Desalination Process by Pretreatments Allowing High-temperature Operation.” Desalination Water Treatment 12: 97.
- Oruc, M. E., A. V. Desai, P. J. A. Kenis, and R. G. Nuzzo. 2016. “Comprehensive Energy Analysis of a Photovoltaic Thermal Water Electrolyzer.” Applied Energy 164: 294–302.
- Peñate, B., F. Castellano, A. Bello, and L. García-Rodríguez. 2011. “Assessment of A Stand-alone Gradual Capacity Reverse Osmosis Desalination Plant to Adapt to Wind Power Availability: A Case Study.” Energy 36 (7): 4372–4384.
- Pichel, N., M. Vivar, and M. Fuentes. 2016. “Performance Analysis of a Solar Photovoltaic Hybrid System for Electricity Generation and Simultaneous Water Disinfection of Wild Bacteria Strains.” Applied Energy 171: 103–112.
- Pietrosemoli, L., and C. Rodríguez-Monroy. 2019. “The Venezuelan Energy Crisis: Renewable Energies in the Transition Towards Sustainability.” Renewable and Sustainable Energy Reviews 105: 415–426.
- Pinto, F. S., and R. C. Marques. 2017. “Desalination Projects Economic Feasibility: A Standardization of Cost Determinants.” Renewable and Sustainable Energy Reviews 78: 904–915.
- Proskynitopoulou, V., and I. A. Katsoyiannis. 2018. “Review of Recent Desalination Developments for More Efficient Drinking Water Production across the World.” New Mater Compound Applications 2 (3): 179–195.
- Purohit, I., and P. Purohit. 2018. “Performance Assessment of Grid-interactive Solar Photovoltaic Projects under India’s National Solar Mission.” Applied Energy 222: 25–41.
- Qiblawey, H. M., and F. Banat. 2008. “Solar Thermal Desalination Technologies.” Desalination 220 (1–3): 633–644.
- Rahmani, K., A. Kasaeian, M. Fakoor, A. Kosari, and S. Alavi. 2014. “Wind Power Assessment and Site Matching of Wind Turbines in Lootak of Zabol.” International Journal of Renewable Energy Research (IJRER) 4 (4): 965–976.
- Rajaei, G., F. Atabi, and M. A. Ehyaei. 2017. “Feasibility of Using Biogas in a Micro Turbine for Supplying Heating, Cooling and Electricity for a Small Rural Building.” Advances in Energy Research 5 (2): 129.
- Rajan, A. S., K. Raja, and P. Marimuthu. 2014. “Multi Basin Desalination Using Biomass Heat Source and Analytical Validation Using RSM.” Energy Conversion and Management 87: 359–366.
- Rajaseenivasan, T., and K. Srithar. 2017. “An Investigation into a Laboratory Scale Bubble Column Humidification Dehumidification Desalination System Powered by Biomass Energy.” Energy Conversion and Management 139: 232–244.
- Raluy, G., L. Serra, and J. Uche. 2006. “Life Cycle Assessment of MSF, MED and RO Desalination Technologies.” Energy 31 (13): 2361–2372.
- Razavieh, A., A. Sedaghat, R. Ayodele, and A. Mostafaeipour. 2017. “Worldwide Wind Energy Status and the Characteristics of Wind Energy in Iran, Case Study: The Province of Sistan and Baluchestan.” International Journal of Sustainable Energy 36 (2): 103–123.
- Reif, J. H., and W. Alhalabi. 2015. “Solar-thermal Powered Desalination: Its Significant Challenges and Potential.” Renewable and Sustainable Energy Reviews 48: 152–165.
- Renewable Energy and Energy Efficiency Organization. 2020. http://www.satba.gov.ir/
- Ridgway, H. F., J. Orbell, and S. Gray. 2017. “Molecular Simulations of Polyamide Membrane Materials Used in Desalination and Water Reuse Applications: Recent Developments and Future Prospects.” Journal of Membrane Science 524: 436–448.
- Roostaei, N. 2004. River Basin Challenges and Management in Iran. The Ministry of Environment, Department Human Environ Teh 2: 1-12.
- Rusu, E., and F. Onea. 2013. “Evaluation of the Wind and Wave Energy along the Caspian Sea.” Energy 50: 1–14.
- Saboori, B., M. Sapri, and M. Bin Baba. 2014. “Economic Growth, Energy Consumption and CO2 Emissions in OECD (Organization for Economic Co-operation and Development)’s Transport Sector: A Fully Modified Bi-directional Relationship Approach.” Energy 66: 150–161.
- Sahoo, U., R. Kumar, P. C. Pant, and R. Chaudhary. 2017. “Development of an Innovative Polygeneration Process in Hybrid Solar-biomass System for Combined Power, Cooling and Desalination.” Applied Thermal Engineering 120: 560–567.
- Saket, A., and A. Etemad-Shahidi. 2012. “Wave Energy Potential along the Northern Coasts of the Gulf of Oman, Iran.” Renewable Energy 40 (1): 90–97.
- Salter, S. H., J. M. B. P. Cruz, J. A. A. Lucas, and R. C. R. Pascal. 2010. “Wave Powered Desalination.” In Macro-engineering Seawater in Unique Environments, 657–674. Berlin / Heidelberg: Springer.
- Sanza, M. A., V. Bonnélyea, and G. Cremerb. 2007. “Fujairah Reverse Osmosis Plant: 2 Years of Operation.” Desalination 203 (1–3): 91–99.
- Sarbatly, R., and C.-K. Chiam. 2013. “Evaluation of Geothermal Energy in Desalination by Vacuum Membrane Distillation.” Applied Energy 112: 737–746.
- Sauvet-Goichon, B. 2007. “Ashkelon Desalination Plant—a Successful Challenge.” Desalination 203 (1–3): 75–81.
- Schwantes, R., A. Cipollina, F. Gross, J. Koschikowski, D. Pfeifle, M. Rolletschek, and V. Subiela. 2013. “Membrane Distillation: Solar and Waste Heat Driven Demonstration Plants for Desalination.” Desalination 323: 93–106.
- Segurado, R., M. Costa, N. Duić, and M. G. Carvalho. 2015. “Integrated Analysis of Energy and Water Supply in IslandS. Case Study of S. Vicente, Cape Verde.” Energy 92: 639–648.
- Sevda, S., H. Yuan, Z. He, and I. M. Abu-Reesh. 2015. “Microbial Desalination Cells as a Versatile Technology: Functions, Optimization and Prospective.” Desalination 371: 9–17.
- Shafaghat, R., H. Shafaghat, F. Ghanbari, P. S. Rezaei, and R. Espanani. 2012. “Design of a MSF Desalination Plant to Be Supplied by a New Specific 42 MW Power Plant Located in Iran.” World Academy of Science, Engineering and Technology 6: 463–469.
- Shatat, M., M. Worall, and S. Riffat. 2013a. “Economic Study for an Affordable Small Scale Solar Water Desalination System in Remote and Semi-arid Region.” Renewable and Sustainable Energy Reviews 25: 543–551.
- Shatat, M., M. Worall, and S. Riffat. 2013b. “Opportunities for Solar Water Desalination Worldwide.” Sustainable Cities and Society 9: 67–80.
- Shatat, M., and S. B. Riffat. 2012. “Water Desalination Technologies Utilizing Conventional and Renewable Energy Sources.” International Journal of Low-Carbon Technologies 9 (1): 1–19.
- Shirazi, M. M. A., A. Kargari, and M. J. A. Shirazi. 2012. “Direct Contact Membrane Distillation for Seawater Desalination.” Desalination and Water Treatment 49 (1–3): 368–375.
- Siddiqui, O., and I. Dincer. 2018. “Examination of a New Solar-based Integrated System for Desalination, Electricity Generation and Hydrogen Production.” Solar Energy 163: 224–234.
- Singh, G. K. 2013. “Solar Power Generation by PV (Photovoltaic) Technology : A Review.” Energy 53: 1–13. doi:https://doi.org/10.1016/j.energy.2013.02.057.
- Smith, J. C., R. Thresher, R. Zavadil, E. DeMeo, R. Piwko, B. Ernst, and T. Ackermann. 2009. “A Mighty Wind.” IEEE Power and Energy Magazine 7 (2): 41–51.
- Soltani, S., R. Saboohi, and L. Yaghmaei. 2012. “Rainfall and Rainy Days Trend in Iran.” Climatic Change 110 (1–2): 187–213.
- Sommariva, C., H. Hogg, and K. Callister. 2004. “Environmental Impact of Seawater Desalination: Relations between Improvement in Efficiency and Environmental Impact.” Desalination 167: 439–444.
- Song, J., T. Li, L. Wright-Contreras, and A. W.-K. Law. 2017. “A Review of the Current Status of Small-scale Seawater Reverse Osmosis Desalination.” Water International 42 (5): 618–631.
- Sophia, A. C., V. M. Bhalambaal, E. C. Lima, and M. Thirunavoukkarasu. 2016. “Microbial Desalination Cell Technology: Contribution to Sustainable Waste Water Treatment Process, Current Status and Future Applications.” Journal of Environmental Chemical Engineering 4 (3): 3468–3478.
- Spyrou, I. D., and J. S. Anagnostopoulos. 2010. “Design Study of a Stand-alone Desalination System Powered by Renewable Energy Sources and a Pumped Storage Unit.” Desalination 257 (1–3): 137–149.
- Sukhatme, S. P., and J. K. Nayak. 2017. Solar Energy. United States: McGraw-Hill Education.
- Tabak, J. 2009. Solar and Geothermal Energy. United States: Infobase Publishing.
- Tavana, H. M. 2009. “Study of Wave Energy Potential in Tonekabon (N Iran).” In 2009 1st International Conference on the Developements in Renewable Energy Technology (ICDRET), Dhaka-Bangladesh, 1–3.
- The World Bank. 2020. Iran Carbon (CO2) Emissions 1960-2020. United States.
- Tir, N. A., F. Momeni, and G. T. Boboevich. 2014. “Exploring the Effects of Water Sector Investment in Economic Development in Iran.” Procedia-Social and Behavioral Sciences 131 (5): 396–405.
- Tofigh, A. A., and G. D. Najafpour. 2012. “Technical and Economical Evaluation of Desalination Processes for Potable Water from Seawater.” Middle-East Journal of Scientific Research 12 (1): 42–45.
- Tofigh, A. A., and M. Abedian. 2016. “Analysis of Energy Status in Iran for Designing Sustainable Energy Roadmap.” Renewable and Sustainable Energy Reviews 57: 1296–1306.
- Tomaszewska, B., and M. Bodzek. 2013. “Desalination of Geothermal Waters Using a Hybrid UF-RO Process. Part I: Boron Removal in Pilot-scale Tests.” Desalination 319: 99–106.
- Touati, S., A. Belkaid, R. Benabid, K. Halbaoui, and M. Chelali. 2012. “Pre-feasibility Design and Simulation of Hybrid PV/fuel Cell Energy System for Application to Desalination Plants Loads.” Procedia Engineering 33: 366–376.
- Tufa, R. A., E. Curcio, E. Brauns, W. van Baak, E. Fontananova, and G. Di Profio. 2015. “Membrane Distillation and Reverse Electrodialysis for Near-zero Liquid Discharge and Low Energy Seawater Desalination.” Journal of Membrane Science 496: 325–333.
- Tufa, R. A., Y. Noviello, G. Di Profio, F. Macedonio, A. Ali, E. Drioli, E. Fontananova, K. Bouzek, and E. Curcio. 2019. “Integrated Membrane Distillation-reverse Electrodialysis System for Energy-efficient Seawater Desalination.” Applied Energy 253: 113551.
- Turchi, C., S. Akar, T. Cath, J. Vanneste, E. Gustafson, and J. Akerley. 2017. Desalination of Impaired Water Using Geothermal Energy. Golden, CO (United States): National Renewable Energy Lab. (NREL).
- Tzen, E. 2006. “Overview of the Desalination Technologies Powered by Renewable Energies (RE).” In Proceedings of International Seminar Desalination Systems Powered by Renewable Energy. Amman, Jordan.
- Ullah, I., and M. G. Rasul. 2019. “Recent Developments in Solar Thermal Desalination Technologies: A Review.” Energies 12 (1): 119.
- Ummel, K., and D. Wheeler. 2008. “Desert Power: The Economics of Solar Thermal Electricity for Europe, North Africa, and the Middle East.” Center for Global Development Working Paper, 156.
- Vafaeipour, M., S. H. Zolfani, M. H. M. Varzandeh, A. Derakhti, and M. K. Eshkalag. 2014. “Assessment of Regions Priority for Implementation of Solar Projects in Iran: New Application of a Hybrid Multi-criteria Decision Making Approach.” Energy Conversion and Management 86: 653–663.
- Viola, A., V. Franzitta, M. Trapanese, D. Curto, and D. Viola. 2016. “Nexus Water & Energy: A Case Study of Wave Energy Converters (Wecs) to Desalination Applications in Sicily.” International Journal of Heat and Technology 34 (2): S379–S386.
- Voutchkov, N. 2012. Desalination Engineering: Planning and Design. Australia: McGraw Hill Professional.
- Werner, C. M., B. E. Logan, P. E. Saikaly, and G. L. Amy. 2013. “Wastewater Treatment, Energy Recovery and Desalination Using a Forward Osmosis Membrane in an Air-cathode Microbial Osmotic Fuel Cell.” Journal of Membrane Science 428: 116–122.
- Wetterau, G. 2011. Desalination of Seawater: M61. Vol. 61. United States: American Water Works Association.
- Yılmaz, İ. H., and M. S. Söylemez. 2012. “Design and Computer Simulation on Multi-effect Evaporation Seawater Desalination System Using Hybrid Renewable Energy Sources in Turkey.” Desalination 291: 23–40.
- Younos, T. 2005. “The Economics of Desalination.” Journal of Contemporary Water Research & Education 132 (1): 39–45.
- Yousefi, H., S. Ehara, and Y. Noorollahi. 2007. “Geothermal Potential Site Selection Using GIS in Iran.” In Proceedings of the 32nd Workshop on Geothermal Reservoir Engineering, 174–182. Stanford, California: Stanford University.
- Yousefi, H., Y. Noorollahi, S. Ehara, R. Itoi, A. Yousefi, Y. Fujimitsu, J. Nishijima, and K. Sasaki. 2010. “Developing the Geothermal Resources Map of Iran.” Geothermics 39 (2): 140–151.
- Yousefi, Z., R. Motallebi, and A. Takdastan. 2014. “Water Desalination by Membrane Technology (RO) in Southern Iran (Jask City).” Environmental Health Engineering and Management Journal 1 (1): 13–18.
- Yuan, Y., X. Gao, Y. Wei, X. Wang, J. Wang, Y. Zhang, and C. Gao. 2017. “Enhanced Desalination Performance of Carboxyl Functionalized Graphene Oxide Nanofiltration Membranes.” Desalination 405: 29–39.
- Zabihian, F., and A. S. Fung. 2011. “Review of Marine Renewable Energies: Case Study of Iran.” Renewable and Sustainable Energy Reviews 15 (5): 2461–2474.
- Zamen, M., S. M. Soufari, S. A. Vahdat, M. Amidpour, M. A. Zeinali, H. Izanloo, and H. Aghababaie. 2014. “Experimental Investigation of a Two-stage Solar Humidification–dehumidification Desalination Process.” Desalination 332 (1): 1–6.
- Zanous, S. P., R. Shafaghat, R. Alamian, M. S. Shadloo, and M. Khosravi. 2019. “Feasibility Study of Wave Energy Harvesting along the Southern Coast and Islands of Iran.” Renewable Energy 135: 502–514.
- Zejli, D., R. Benchrifa, A. Bennouna, and K. Zazi. 2004. “Economic Analysis of Wind-powered Desalination in the South of Morocco.” Desalination 165: 219–230.
- Zhang, B., and Z. He. 2013. “Improving Water Desalination by Hydraulically Coupling an Osmotic Microbial Fuel Cell with a Microbial Desalination Cell.” Journal of Membrane Science 441: 18–24.
- Zhang, F., K. S. Brastad, and Z. He. 2011. “Integrating Forward Osmosis into Microbial Fuel Cells for Wastewater Treatment, Water Extraction and Bioelectricity Generation.” Environmental Science & Technology 45 (15): 6690–6696.
- Zhou, Y., and R. S. J. Tol. 2005. “Evaluating the Costs of Desalination and Water Transport.” Water Resources Research 41 (3): 1–10. doi:https://doi.org/10.1029/2004WR003749.
- Zirakrad, A., S. J. Hashemian, and M. T. Ghaneian. 2013. Performance Study of Reverse Osmosis Plants for Water Desalination in Bandar-lengeh, Iran.Iran/JOURNAL OF COMMUNITY HEALTH RESEARCH.