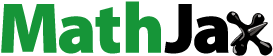
ABSTRACT
Today in Europe building-sector is responsible for the 50% of air pollution and for 70% of waste production. For this reason, researchers’ interest is focused on new eco-building materials that are sustainable, with low impact, renewable and recyclable. In this context, in the last few years, the use of cellulose-based fibres as potential reinforcements for bio-composite materials, instead of synthetic one, received worldwide growing attention. Among natural fibres, both cellulose and protein-based ones, in literature it was found that, due to its mechanical properties, artichoke fibre is suitable for this use. In this study, a Geographical Information System (GIS) – based model to locate and quantify the yearly amount of agricultural waste coming from Cynara cardunculus L. (CW) cultivation was put forward and was applied in a study area located in Southern Italy. As an additional protein-based fibre, sheep wool fibre was also taken into account due to the high presence of sheep. The estimated available amount of both considered fibres was reported in a GIS map in order to show their distribution at territorial level. The achieved results could represent basic information for both sustainable locating collection centre and analysing the environmental impact related to the logistics and supply phase.
1. Introduction
Nowadays, building sector is the most responsible for environmental degradation, global warming, and climate change (Ingrao et al. Citation2016). In fact, at a worldwide level, about 50% of carbon emissions, 20–50% of consumption of energy and natural resources, and 50% of total solid waste production (Vasilca et al. Citation2021; Barreca et al. Citation2018) are generated by construction industry. Ecological concerns such as environmental safety and recyclability have resulted in an increasing interest in green materials (Sain and Panthapulakkal Citation2004). Furthermore, discovering new alternatives for materials derived from non-renewable resources is urgently needed (Ingrao et al. Citation2019). In this regard, recently several studies are focused on new resources and sustainable materials that could be involved and integrated into building process (Sarasini and Fiore Citation2018).
With the aim of replacing traditional building materials, e.g. concrete, steel, plastics’ component, by using new eco-friendly materials, contributes significantly to reduce the impact on environment, by reducing CO2 emission, air and water pollution, waste solid production, and saving energy (Asdrubali, D’Alessandro, and Schiavoni Citation2015). Since the eco-friendly materials are generally obtained by using natural and renewable sources that are not commonly employed for construction (Moussa et al. Citation2018), they are totally recyclable, sustainable, non-toxic for human health, with a low carbon foot (Ingrao et al. Citation2015). Moreover, fully in accordance with the circular economy statements, eco-building materials could be also obtained by the reconversion of wastes (Aarhus Citation2015).
By considering this conversion to a green building approach, the interest in bio composites is constantly grown. A bio composite is a material composed of a matrix added by one or more distinct constituent materials, generally reinforcement fibres. These two or more constituents are combined to realise a new material with performant physical and mechanical behaviours (Fagone et al. Citation2019) or to improve the quality of recycled coarse concrete aggregates (Gonzalez and Etxeberria Citation2014; Singh et al. Citation2021).
Recently, the use of natural fibres as reinforcement for composite materials, instead of synthetic ones (e.g. glass fibre, polymeric fibres), is receiving increasing attention not only for their mechanical properties, but also for the low cost, the recyclability, the availability, and for health benefits that come from their use (Mathur Citation2006; Liuzzi, Sanarica, and Stefanizzi Citation2017; Fagone et al. Citation2019; Galan-Marin, Rivera-Gomez, and Garcia-Martinez Citation2016; Rojat et al. Citation2015). There is a wide variety of different natural fibres which can be applied as reinforcements. Furthermore, the use of agricultural residues provided also a solution to solve problems concerning solid waste disposal, which usually does not have any economic alternative (Vitrone et al. Citation2021). The most widely cellulose-based fibres used are flax, hemp, jute, kenaf and sisal, because of their properties and availability; only few recent scientific works have analysed the feasibility to use other cellulose-based fibres, such like okra (De Rosa et al. Citation2010) and isora (Mathew, Joseph, and Joseph Citation2006), as reinforcement for bio-composite materials. Three types of fibres, wheat straw, barley straw and wood shavings, were investigated by (Ashour and Wu Citation2010) as reinforcement on plaster materials that also are composed of cohesive soil and sand.
Worldwide, researchers are working to investigate the potential use of natural fibres as reinforcement composites by analysing their properties like tensile strength, compressive strength, toughness, thermal degradation temperature, low weight (Sanjay et al. Citation2018; Raja et al. Citation2017). Protein-based fibres are of animal origin (e.g. pig hair, sheep wool, silk) or vegetables (e.g. cotton, hemp, linen, jute, sisal, coconut, bamboo, cork), and differ by their structure. In detail, animals’ fibres have a protein structure (i.e. keratin protein); instead, plants’ fibres are composed by a lignocellulose structure (i.e. cellulose, lignin, hemicellulose, pectin, waxes, and water-soluble substances), which contributes at improving the tensile strength of vegetable fibres.
Among cellulose-based fibres, thanks to its mechanical characteristics, Cynara cardunculus L. fibres (CW) are well suited to building applications as reinforcement for adobe clay or cement mix. Several research studies were carried out on globe artichoke due to its relevant production and due to the several uses different from human food, i.e. fresh biomass, forage for livestock, feedstock for the preparation of alcoholic beverages, and a source of inulin (Lo Giudice et al. Citation2014).
Several research studies have revealed the possibility of using this kind of biomass (leaves, stems, flower heads and achenes) as solid biofuel, through direct combustion or pyrolysis (Damartzis et al., 2011; Ierna et al., 2012; Karampinis et al., 2012). Few studies have investigated the possibility of using CW as a new and alternative feedstock for anaerobic digestion for biogas production (Ferrero et al. Citation2020), by demonstrating that the chemical characteristics, in terms of tissue lignification, could affect biogas plant management at the farm scale (Foti et al. Citation1999) and, moreover, could arise environmental, social and economic concerns related to the competition between food and no-food products, i.e. the use of cardoon for human food, energy crops and for industrial purposes, by disregarding the Biogasdoneright© concept (Dale et al. Citation2016; Valenti et al. Citation2016; Selvaggi et al. Citation2018; Selvaggi and Valenti Citation2021).
Instead, in order to investigate the possibility of using globe artichoke cellulose-based fibres as reinforced composites, Fiore, Valenza, and Di Bella (Citation2011) carried out an experimental trial for the identification of microstructure, chemical composition and mechanical properties of cardoon stem fibres, with the aim of evaluating their potential use as reinforcements for polymer composites. By single fibre tensile tests, tensile strength and Young’s modulus of cardoon stem fibre were evaluated and the results were analysed through a statistical Weibull distribution, already used for other cellulose-based fibres (Fagone et al. Citation2019; Thyavihalli Girijappa et al. Citation2019). Using obtained results comparable to those of other cellulose-based fibres, i.e. banana and sisal fibres (Elanchezhian et al. Citation2018), it has been demonstrated that artichoke fibres, coming from the recovery of cardoon stems, are suitable to replace synthetic fibres as reinforcement in composite structures.
Nowadays, data on the exact amount of these fibres are very limited. This lack of official data related to the amount of recyclable fibres in terms of volume, and especially to the spatial localisation of the sites where these are produced, are the main factors that have over the years limited their reuse and exploitation. For this reason, this research study aims, by using Geographic Information System (GIS) tools, to fill the gap in the knowledge of the production, quantification and above all the localisation of CW as potential reinforcement cellulose-based fibres by its recycling process and to support new eco-building material production.
In detail, this research study focused on the first step of post-cultivation management of Cynara cardunculus L. wastes, i.e. stems, which is crucial in order to put forward a method for a sustainable recovery management and extracting process of fibres. Worldwide, Italy is the higher producer of cardunculus, and Sicily is the region with the yearly higher cardunculus production, followed by Apulia (Istat Citation2021). A large amount of waste, about 80–85% of this cultivation ground biomass was produced (Pandino, Lombardo, and Mauromicale Citation2013). Stems and leaves are the most abundant wastes regarding cardoon crops (Barbosa et al. Citation2020), and represent the 40% and the 60% of these wastes, respectively (Esposito et al. Citation2016).
Since GIS tools are a suitable platform for environmentally related issues and have been applied for both assessing, quantifying and site-location analyses, in this study a GIS-based model was developed for collecting, organising, analysing, and visualising geographical data related to CW (Zubaryeva et al. Citation2012; Höhn et al. Citation2014; Valenti et al. Citation2018; Selim et al. Citation2018; Abbasi, Pishvaee, and Bairamzadeh Citation2020; Zolfaghary, Zakerinia, and Kazemi Citation2021; Parlato, Valenti, and Porto Citation2020; Selvaggi et al. Citation2021).
Data recorded by statistical database were elaborated and applied for quantifying the amount of CW. The obtained results were adopted for computing, through a suitable index, the amount of available cardoon stems and developing tailored heatmaps for showing its distribution at territorial level.
Moreover, cardoon stem fibres yearly amount was also compared with sheep wool fibres yearly amount production. In a recent paper (Parlato and Porto Citation2020) sheep wool fibre was investigated as new resource for building construction, so it was interesting combining the localisation and the availability of these two different cellulose and protein-based fibres.
The GIS-based model results could provide basic information for the analysis of the environmental impact related to the logistics and supply phase due to the transportation of the estimated amount of fibres from both cardoon stems and sheep wool to a future collection centre for reusing them.
In addition, the use of both cellulose and protein-based fibres as a potential reinforcement in composite materials could also bring important benefits in terms of new jobs, in a region where the unemployment rate is quite high, within the context of circular economy.
2. Materials and methods
2.1. Cynara cardunculus L
Cynara cardunculus L. is a perennial plant belonging to the family Asteraceae, which is native to the Mediterranean area, commonly known as cardoon. Fibres of cardoon are generally extracted by stems through a maceration process. Stems of cardoon have a complex structure consisting mainly of cellulose, hemicellulose, lignin, pectin, and other compounds. This anatomical structure is typical of dicotyledon angiosperm non-woody plant with a central pith surrounded by a cortex and with an irregular thin epidermis (Gominho et al., 2018). Fibres are located on a fibro-vascular bundle that surround the pith. Helically wound microfibrils of cellulose are the constituent of each fibre that are bounded together by an amorphous lignin matrix. In is reported a typical chemical composition of cardoon fibre (Fiore, Valenza, and Di Bella Citation2011):
Table 1. Chemical composition of cardoon fibres
Mechanical and physical behaviours of cardoon fibres were found in literature. In detail, tensile strength is about 200 MPa, Young’s modulus is about 11.62 GPa, diameter is in the range between 150 mm to 320 mm, and density is about 1.579 gr/cm3 (Fiore, Valenza, and Di Bella Citation2011). These values are comparable, as shown in , with those of other vegetable fibres commonly employed in green building sector.
Table 2. Mechanical properties of most common vegetable fibres (Thyavihalli Girijappa et al. Citation2019)
For this reason, cardoon fibres are suitable to be used as reinforcement fibres for bio-composites (Sarasini and Fiore Citation2018; Turco et al. Citation2021).
To locate and quantify the yearly amount of waste coming from Cynara cardunculus L. a GIS-based model has been developed.
2.2. Study area
Sicily is a Southern region of Italy divided into nine provinces with the Tyrrhenian Sea to the North, the Ionian Sea to the East and the Mediterranean on the remaining coasts. It extends for 25,707 km2 and, in addition to being the largest Island in the Mediterranean, it is the largest Italian region (). Sicily is also the Italian region with the largest extension of agricultural land (), and as reported by statistical database (Istat Citation2021) it is the region with the highest production of Cynara cardunculus L. ().
Table 3. Italian cultivated area for horticultural crops and Cardunculus cultivation (ha)
Table 4. Italian production of horticultural crops and Cardunculus cultivation (t)
In Sicily, as in the rest of the South, employment in agriculture is much higher than the national average. Despite the lowest wages, in Sicily agriculture has maintained its predominant position within regional economy (Valenti et al. Citation2017), with the highest Utilised Agricultural Area (UAA), equal to over 1.387 million hectares which correspond to about 10.8% of the national UAA (Badami, Caracci, and Costanzo Citation2017).
Data related to the last 5 years (from 2016 to 2020), with reference to horticultural open field crops (both cultivated area and production) and by paying attention to the Cynara cardunculus L., were elaborated at regional level and reported in , respectively, for cultivated area and production.
From the data elaboration, as reported in , in Italy an average of about 380,000 ha/y of cultivated surface area are dedicated to horticultural crops cultivation, while in Sicily the cultivated surfaces are a little more than 60,000 ha/y (about 15%). Moreover, within the selected time-interval, the horticultural open field crops cultivated areas registered in Sicily a slight increase (+1.1%) by rising from about 59,000 ha/y to 67,000 ha/y.
By paying attention to the Cardunculus cultivation, about the 90% of the Italian cardunculus cultivated area coming from only three regions. Sicily is the region with the highest cultivated area, an average of about 40,000 ha/y, which corresponds to 37% of the Italian cardunculus cultivated area, followed by Apulia and Sardinia with about an average of 12,000 ha/y (30%) and 9,000 ha/y (23%), respectively. By considering the trend during the selected time-interval in Sicily a slight increase (+1.1%) was registered, by rising from about 14,000 ha/y to 15,200 ha/y.
However, Lombardy region contributes to the horticultural cultivated area with an average of about 16,000 ha/y, it is possible to notice that almost no areas were dedicated to Cardunculus cultivation, as well as for Piedmont, Friuli-Venezia Giulia, Valle d’Aosta, and Trentino Alto-Adige regions ().
Data related to the horticultural open field crop production were elaborated and reported for each Italian region in , by considering the same time-interval (2016–2020) and focusing on the cardunculus production.
By considering the production, as it shown in , again Apulia, Emilia-Romagna, and Sicily are those regions with the highest horticultural open field crop production, by representing more than 50% of the total production (about 12 million t/y), with, respectively, an average of about 3 million t/y, 2.4 million t/y and little less than 1 million t/y.
Instead, by focusing on the cardunculus production, Sicily, Apulia and Sardinia regions represent more than 80% of the Italian production (about 390,000 t/y). In detail, in Sicily more than 150,000 t (41% of the total production) were yearly produced. Furthermore, within the selected time-interval, the horticultural field crops registered in Sicily had a slight increase (+1.2%) by rising from about 950,000 t/y to more than 1 million t/y, while the production of cardunculus was kept almost unchanged ().
As concerning other Italian regions by taking into account the cardunculus production, no general trend was observed, in fact, as shown in , a first decrease until 2018 and then an increase in the cardunculus production was recorded for Umbria, Tuscany and Veneto regions; an increase over the years was registered for the region of Marche, Molise and Lazio, instead of a production decrease was observed for the region of Campania, Sardinia, Liguria and Lombardy, which starts its production from 2018.
Since, as reported in both , related to Cynara cardunculus L. cultivation, Sicily is the region with both the highest cultivated area (i.e. 14,955 ha/y) and, above all, the highest production (i.e. 159,721 t/y), it was selected as study area.
2.3. Data analysis
In this study, an extensive database was improved according to statistical sources, i.e. ISTAT (2016–2020), in order to quantify both the Cardunculus cultivation surface areas and its production for evaluating their distribution at territorial level by GIS analyses.
The base maps used in the GIS software included the Regional Technical Maps (RTM 2008) as the base map for carrying out both thematic maps and heatmaps.
By analysing available data on the agricultural sector, i.e. horticultural cultivation, the provinces with the highest surface areas dedicated to Cardunculus cultivation and with the highest amount of its production were identified and localised on GIS software.
In detail, the QGis software (ver. 3.10.11), an open-source GIS software, was used since it is a decision support tool appropriate for collecting, organising, analysing, and localising geographical data. QGis software was used to perform all the GIS analyses; by combining data provided by the base maps, data acquired from the database, and by adopting the Jenks tool available in the QGIS software, several maps were produced.
Firstly, the statistical data base of National Institute of Statistic (ISTAT) was used to acquire data relating to the horticultural cultivations by selecting a five-year time interval (2016–2020).
In detail, data concerning cardunculus cultivation and its production were selected and analysed in order to identify both the trend of surface areas and production. Then, by using spatial analysis GIS tools, the acquired data were elaborated and applied to provide distribution of both cultivation surface areas and cardunculus production within the study area. In detail, a territorial distribution of both surfaces areas and production areas were obtained, by classifying the Sicilian provinces through the application of Jenks tool plug-in, with the aim of maximising the differences among the classes.
A tailored methodology was developed in order to quantify the CW amount suitable as potential reinforcements for bio-composite materials, i.e. cardoon stem fibres.
The next step of the methodology was the computation of the index () for describing the amount of cardoons stems availability by applying the following equation:
where
CW represents the total amount of wastes, which correspond to about the 80–85% of the artichoke ground biomass (Pandino, Lombardo, and Mauromicale Citation2013); the 40% is the percentage of stems useful for extracting vegetal fibres (Esposito et al. Citation2016).
The obtained results were reported in GIS and, by using the Jenks tool, were elaborated for assessing those territorial areas highly characterised by this kind of potential recyclable cellulose-based fibres. Then, with the aim of estimating those areas, within each province, characterised by the highest density of cardoon stems, before applying the Heatmap plugin available in QGis software, the centroids for each province were computed. Therefore, firstly the Polygon centroid plugin was applied; then, the produced base map was used for carrying out the heatmap based on the amount of available cardoon stems. In detail, during the Heatmap plugin application, the discrete-interpolation method was set and, in order to increase the influence certain features, have on the resultant heatmap, the input feature was weighted by the attribute related to the stem availability.
Finally, the amount of cardoon stem fibres was combined with that of sheep wool fibres, previously investigated by (Parlato and Porto Citation2020), in order to highlight the territorial areas with the highest availability of these two different cellulose and protein-based fibres, both suitable for new eco-friendly materials. The selected areas could be useful for localising future collection centres by reducing environmental impact due to the logistic and supply phase.
3. Results and discussion
Data recorded from ISTAT database (2016–2020-time interval) were elaborated and deeply analysed in order to consider only data related to Cardunculus cultivation and production. Data were organised to show at territorial level the distribution of this cultivation within Sicilian provinces by developing a GIS map.
As first step, the acquired data were used for providing the distribution of cultivated areas dedicated to cardunculus production, by showing them at territorial level. Then, by using Jenks tool, available in QGIS software, the Sicilian provinces were grouped in three different classes based on their cardunculus cultivated areas. As shown in Caltanissetta province, followed by Agrigento and Catania are those ones with the main surface area dedicated to the Cardunculus cultivation.
In detail respectively, about an average of 6000, 4000 and 2000 ha of surface area are yearly cultivated, which correspond to about the 80% of the total cardunculus cultivated surface area in Sicily.
Since the areas with the highest surface of cultivated area do not always correspond to those with the highest production, the cardunculus production was analysed by considering averaged data belonging to the same time interval (2016–2020).
As reported in , the results of the elaborations showed a different distribution within Sicilian provinces and confirmed Caltanissetta and Agrigento as most productive provinces, with about 47,000 tons and 42,400 tons of yearly production, respectively, which correspond to 60% of the total Sicilian production.
Furthermore, the distribution of the cultivated areas allows the identification of the highly representative area for this cultivation located in the south of the Island.
Since the research paper was focused on estimating the amount and localisation of cellulose-based fibres that could be reuse as potential reinforcements for bio-composite materials, i.e. cardunculus fibre, firstly among the cardunculus production only the cardoon stems, which represent, on average, about 40% of total cardoon waste were considered with the aim of quantifying the available cellulose-based fibres.
Therefore, by applying EquationEquation 1(1)
(1) , data related on the available cardoon stems were obtained and reported on GIS map ().
Data shown in confirms the provinces of Caltanissetta and Agrigento as those ones with the highest availability of potential recyclable cellulose-based fibres, i.e. cardoon stems, with about 15,000 and 13,000 ton/y of produced cardoon stems, respectively.
With the aim of producing a tailored heatmap based on the computed amount of cardoon stems firstly, the polygon centroids were computed () and applied for developing the heatmap ().
Figure 5. Cardoon stems distribution. (a) Polygon centroid for developing heatmaps. (b) Heatmap distribution based on the computed cardoon stem production.
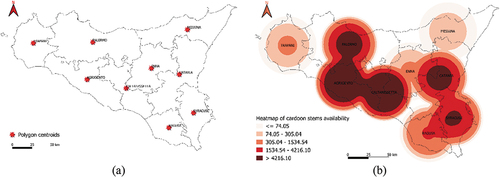
The heatmap results, as reported in , highlighted two big areas where cardoon stems are highly concentrated, i.e. the central area and southeast one. In this regard, the provinces of Caltanissetta, Agrigento, Ragusa and Catania are the provinces where more than 4000 tons/year of cardoon stems are yearly available.
The results reported in concerning the amount and location of cardoon stem fibres, recyclable as potential reinforcements for bio-composite materials, are essential for a better management of collection centres by considering the existing infrastructures in order to optimise the collection process and therefore reduce CO2 emissions that is the major responsible for the global warming.
The use of these fibres in Sicily, where the cardunculus production is huge, can produce important benefits in terms of new jobs (i.e. green jobs) in a region with high unemployment (Fiore, Valenza, and Di Bella Citation2011).
Since, as reported by several authors in literature, (Picuno, Citation2016; Wang et al. Citation2018; Parlato and Porto Citation2020), a sustainable building process could be improved by using natural insulation materials instead of those commonly used (e.g. polyurethane foam, polystyrene (EPS), fibreglass, mineral wool), and cellulose and protein-based fibres, i.e. fibre extracted by cardoon stems and sheep wool fibre (Galan-Marin, Rivera-Gomez, and Garcia-Martinez Citation2016), as reinforcements for bio-composite materials instead of synthetic ones. In this context, several research studies successfully demonstrated the possibility of using sheep wool fibres, not only as natural insulation material (Parlato and Porto Citation2020), but also within different composite matrices, such as unfired clay adobe or cement mortar (Galán-Marín, Rivera-Gómez, and Petric-Gray Citation2010a; Galán-Marín, Rivera-Gómez, and Petric-Gray, Citation2010b; Mounir et al. Citation2015).
Furthermore, as reported by (Parlato and Porto Citation2020), Sicily is the second region in Italy for number of sheep, with more than 900,000 heads that correspond to about an annual production of almost 1,000 tons of greasy wool (nowadays considered a special waste). In this context, data related to the quantity of sheep wool as recyclable fibres were elaborated and reported in GIS. Firstly, in order to show at territorial level, the distribution of those areas where sheep wool is highly available, by taking into account the number of Sicilian sheep, the amount of sheep wool was quantified and reported in GIS. shows at a territorial level the distribution of the computed available sheep wool, by highlighting the inner area of the Island as the most suitable for this kind of by-products.
Figure 6. Distribution within Sicily region of the recyclable sheep wool protein-based fibres as potentially reinforcements for bio-composite material.
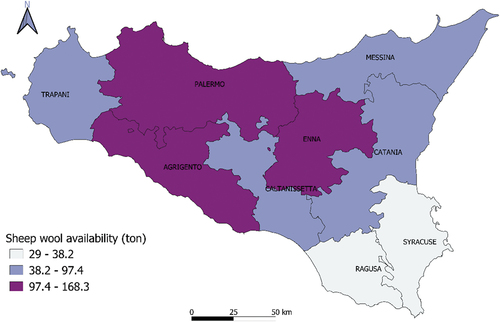
By overlying results reported in related to sheep wool availability areas, with those reported in , related instead to the cardoon stems distribution, a map was produced in order to define the most representative area by combining these two cellulose and protein-based fibres ().
Figure 7. Distribution within Sicily region of both recyclable sheep wool and cardoon stem cellulose-based fibres as potentially reinforcements for bio-composite material.
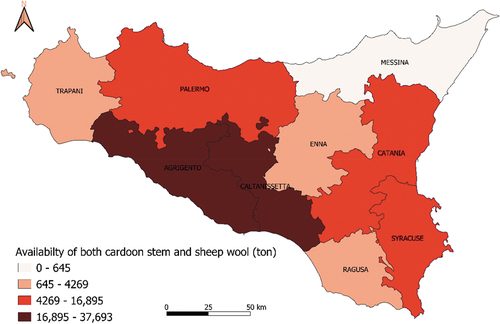
Form the provinces of Caltanissetta and Agrigento resulted as the most proper for locating collection centres due to the high concentration of both the considered fibres.
Therefore, in view of obtaining new eco-friendly materials, by using fibres from both cardoon stems and sheep wool and with the aim of reducing the transport cost for transferring them to the recycling plants (Díaz-Palacios-Sisternes, Ayuga, and García Citation2014; Osmani and Zhang Citation2017), the optimisation of the location of new collection centres is required. In this context, the results obtained in this study by developing the GIS-based model could represent a first step to achieve the objective and could be also used to plan in detail the location of such collection centre by using the developed heatmap ().
4. Conclusions
This research study was carried out by combining data recorded from a statistic database (ISTAT) and GIS-based maps, fulfiling the proposed aim of the research. In detail, the horticultural production, by focusing on Cardunculus cultivation, was deeply investigated by elaborating the data related to both the cultivation surface area and its production for producing GIS maps. The achieved results by the developed GIS-based model contribute to fill the gap in the knowledge of the production and localisation of cardoon stems as potential reinforcement cellulose and protein-based fibres by its recycling process and to support new eco-building material production. A new industrial chain could be created, which could generate potential source of value to be used and exploited through new production cycles, according to the principles of circular economy.
In this regard, the obtained results could be useful for planning tailored collected centres as near as possible to those areas where these by-products are highly produced. This condition is relevant for a sustainable valorisation by paying attention to the transport costs during the logistics and supply phase (i.e. from the cultivated fields to the collection centres), with an advantage in terms of environmental and social impacts, and costs. Finally, in this study also sheep wool fibre localisation was considered for the same purpose above-mentioned, by highlighting, through the overlay of both (i.e. cardoon stems and sheep wool fibres) the obtained territorial distributions, those areas most suitable for localising collection centres. After the identification of the most suitable areas, the future goal of this research work is to investigate the suitability of the combination of these kind of fibres for new kind of composites by analysing their mechanical properties.
Author contributions
Parlato Monica C.M.: Conceptualisation, Methodology, Writing – Original draft preparation, Writing – Reviewing and Editing. Valenti Francesca: Methodology, Formal analysis, Data curation, Software, Writing – Reviewing and Editing, Supervision. Lanza Elisa: Writing – Original draft preparation. Porto Simona M.C.: Visualization
Acknowledgments
This research was carried out by both Francesca Valenti within her project entitled “Sostenibilità dell’agricoltura e dell’industria agro-alimentare Mediterranea”—Programma Operativo Nazionale (PON) FSE–FESR “Research and Innovation 2014–2020, D.D. 407/2018” Attraction and Mobility (AIM)–Proposal: AIM1848200, Line 1, CUP: E64I19002440007 supported by Italian Ministry of Education, University and Research (MIUR), and by Monica C.M. Parlato PhD student in the “International Doctorate in Agricultural, Food and Environmental Science – Di3A – University of Catania (Italy)” and was supported by Simona M.C. Porto advisor and vice-coordinator of the above mentioned doctorate. Furthermore, it is in line with the research project funded by the University of Catania: (PIAno di inCEntivi per la RIcerca di Ateneo 2020/2022 (D.R. N. 1208 dell’11 Maggio 2020) -“Engineering solutions for sustainable development of agricultural buildings and land” (ID: 5A722192152) -WP3: Ottimizzazione e valorizzazione dell’uso delle risorse naturali, sottoprodotti e scarti.
Disclosure statement
No potential conflict of interest was reported by the authors.
Additional information
Notes on contributors
Monica C.M. Parlato
Monica C.M. Parlato is Architectural and Building Engineer, PhD student in Agricultural, Food and Environmental Science, at the Department of Agriculture, Food and Environment (Di3A), University of Catania (Italy). Her research activity mainly focuses on sustainable bio-based building materials and technologies, agricultural waste valorization, GIS-based analyses. During her research activity she was experienced on mechanical analyses, physical analyses, GIS models, production process of reinforced bio composites, laboratory activities. She has a teaching experience of 7 years as math teacher at high school.
Francesca Valenti
Francesca Valenti is Architectural and Building Engineer, PhD in Agricultural, Food and Environmental Science, Assistant Professor at the Department of Agriculture, Food and Environment (Di3A) of the University of Catania (Italy), and she has got the Italian scientific qualification for the role of full professor. Her research activity mainly focuses on GIS-based analyses; modelling and planning; food security in agro-industrial buildings; building systems and infrastructures for animal breeding; evaluation of the environmental sustainability of innovative production processes through LCA. During her research activity she was experienced on GIS models, CFD models, LCA tools, laboratory activities, chemical analyses, BMP tests and simulation of the continuous anaerobic digestion process. She is a member of the editorial board of the journal Applied System Innovation and Guest Editor for Special Issue “Reuse, Recycle and Reduce: Smart Innovative Treatments of Agricultural Waste and By-Products” on Applied System Innovation and the Special Issue ”Water–Food–Energy Nexus for a Sustainable Use and Management of Natural Resources, Byproducts and Wastes in Agri-Food Systems” on Energies.
Elisa Lanza
Elisa Lanza is an Architect, PhD student in Agricultural, Food and Environmental Science at the Department of Agriculture, Food and Environment (Di3A), University of Catania (Italy). Her research interests mailnly focus on green materials, technical solutions for nZEB (nearly Zero Energy Building) construction, environmental sustainability, landscape safeguard, and LCA Life Cycle Assessment (LCA) analyses.
Simona M.C Porto
Simona M.C. Porto is associate professor at the University of Catania (Italy) in the scientific sector “Rural Buildings and Agro-Forest Land Planning.” In the same institution, she is president of the master degree course “Safeguarding the territory, the environment and landscape,” vice coordinator of the PhD course in “agricultural, food and environmental science,” and a member of the related academic board. She is a member of the Italian Association of Agricultural Engineering (AIIA), technical section “agricultural constructions, installation techniques and related technologies.” She is also a member of the International Commission of Agricultural Engineering (CIGR), technical section “farm buildings, equipment, structures and environment.” She is the principal investigator in important national and international research projects. She is a member of the editorial board of the journal Sustainability. The studies that she has carried out have been published in over 100 full papers, more than half of them being indexed in the Scopus database and Web of Science.
References
- Aarhus, A. 2015. “Building Materials: From Waste to Resource”. Conference: Young Scientists Towards the Challenges of Modern Technology, Warsaw.
- Abbasi, M., M. S. Pishvaee, and S. Bairamzadeh. 2020. “Land Suitability Assessment for Paulownia Cultivation Using Combined GIS and Z-number DEA: A Case Study.” Computers and Electronics in Agriculture 176: 105666. doi:10.1016/j.compag.2020.105666.
- Asdrubali, F., F. D’Alessandro, and S. Schiavoni. 2015. “A Review of Unconventional Sustainable Building Insulation Materials.” Sustain Mater Technol 4: 1–17.
- Ashour, T., and W. Wu. 2010. “An Experimental Study on Shrinkage of Earth Plaster with Natural Fibres for Straw Bale Buildings.” International Journal of Sustainable Engineering 3 (4): 299–304. doi:10.1080/19397038.2010.504379.
- Badami, G., M. Caracci, and D. Costanzo. 2017. “.” In Le Filiere Agroalimentari Siciliane. Analisi Puntuale eTendenze del Settore ad uso Delle Imprese Agricole eDell’utenza Pubblica. Lausanne, Switzerland: Editions Antipode.
- Barbosa, C. H., M. A. Andrade, F. Vilarinho, I. Castanheira, A. L. Fernando, M. R. Loizzo, and A. Sanches Silva. 2020. “A New Insight on Cardoon: Exploring New Uses Besides Cheese Making with A View to Zero Waste.” Foods 9 (5): 564. doi:10.3390/foods9050564.
- Barreca, F., G. D. Cardinali, C. R. Fichera, and P. Praticò. 2018. “Utilization of Cork Residues for High Performance Walls in Green Buildings.” Agricultural Engineering International: CIGR Journal 20 (1): 47–55.
- Dale, B. E., F. Sibilla, C. Fabbri, M. Pezzaglia, B. Pecorino, E. Veggia, A. Baronchelli, et al. 2016. “An Innovative New System Is Commercialized in Italy.” Biofuels Bioprod Biorefin 10 (4): 341–345. DOI:10.1002/bbb.1671.
- De Rosa, I. M., J. M. Kenny, D. Puglia, C. Santulli, and F. Sarasini. 2010. “Morphological, Thermal and Mechanical Characterization of Okra (Abelmoschus Esculentus) Fibres as Potential Reinforcement in Polymer Composites.” Compos Sci Technol 70 (1): 116–122. doi:10.1016/j.compscitech.2009.09.013.
- Díaz-Palacios-Sisternes, S., F. Ayuga, and A. I. García. 2014. “A Method for Detecting and Describing Land Use Transformations: An Examination of Madrid’s Southern Urban–rural Gradient between 1990 and 2006.” Cities 40 (Part A): 99–110. doi:10.1016/j.cities.2014.03.010.
- Elanchezhian, C., B. V. Ramnath, G. Ramakrishnan, M. Rajendrakumar, V. Naveenkumar, and M. K. Saravanakumar. 2018. “Review on Mechanical Properties of Natural Fibre Composites.” Mater Today Proc 5 (1): 1785–1790. doi:10.1016/j.matpr.2017.11.276.
- Esposito, M., P. Di Pierro, W. Dejonghe, L. Mariniello, and R. Porta. 2016. “Enzymatic Milk Clotting Activity in Artichoke (Cynara Scolymus) Leaves and Alpine Thistle (Carduus Defloratus) Flowers. Immobilization of Alpine Thistle Aspartic Protease.” Food Chem 204: 115–121. doi:10.1016/j.foodchem.2016.02.060.
- Fagone, M., H. Kloft, F. Loccarini, and G. Ranocchiai. 2019. “Jute Fabric as a Reinforcement for Rammed Earth Structures.” Compos Part B Eng 175: 107064. doi:10.1016/j.compositesb.2019.107064.
- Ferrero, F., E. Dinuccio, L. Rollé, E. Tabacco, and G. Borreani. 2020. “Suitability of Cardoon (Cynara Cardunculus L.) Harvested at Two Stages of Maturity to Ensiling and Methane Production.” Biomass and Bioenergy 142 (1): 105776. doi:10.1016/j.biombioe.2020.105776.
- Fiore, V., A. Valenza, and G. Di Bella. 2011. “Artichoke (Cynara Cardunculus L.) Fibres as Potential Reinforcement of Composite Structures.” Composites Science and Technology 71 (8): 1138–1144. doi:10.1016/j.compscitech.2011.04.003.
- Foti, S., G. Mauromicale, S. A. Raccuia, B. Fallico, F. Fanella, and E. Maccarone. 1999. “Possible Alternative Utilization of Cynara Spp. I. Biomass, Grain Yield and Chemical Composition of Grain.” Ind Crops Prod 10 (3): 219–228. doi:10.1016/S0926-6690(99)00026-6.
- Galan-Marin, C., C. Rivera-Gomez, and A. Garcia-Martinez. 2016. “Use of Natural-fibre Bio-composites in Construction versus Traditional Solutions: Operational and Embodied Energy Assessment.” Materials 9 (6): 465. doi:10.3390/ma9060465.
- Galán-Marín, C., C. Rivera-Gómez, and J. Petric-Gray. 2010a. “Effect of Animal Fibres Reinforcement on Stabilized Earth Mechanical Properties.” J. Biobased Mater. Bioenergy 4 (2): 121–128. doi:10.1166/jbmb.2010.1076.
- Galán-Marín, C., C. Rivera-Gómez, and J. Petric-Gray. 2010b. “Animal Fibres and Seaweed Polymers to Improve Unfired Clay Blocks Characteristics.” J. Biobased. Mater. Bioenergy 4: 1–8.
- Gonzalez, A., and M. Etxeberria. 2014. “Experimental Analysis of Properties of High Performance Recycled Aggregate Concrete.” Construction and Building Materials 52: 227–235. doi:10.1016/j.conbuildmat.2013.11.054.
- Höhn, J., E. Lehtonen, S. Rasi, and J. Rintala. 2014. “A Geographical Information System (GIS) Based Methodology for Determination of Potential Biomasses and Sites for Biogas Plants in Southern Finland.” Appl Energy 113: 1–10. doi:10.1016/j.apenergy.2013.07.005.
- Ingrao, C., C. Arcidiacono, A. Bezama, G. Ioppolo, K. Winans, A. Koutinas, and A. Gallego-Schmid. 2019. “Sustainability Issues of By-product and Waste Management Systems, to Produce Building Material Commodities: A Comprehensive Review of Findings from A Virtual Special Issue.” Resources, Conservation and Recycling 146: 358–365. doi:10.1016/j.resconrec.2019.04.001.
- Ingrao, C., A. Lo Giudice, J. Bacenetti, C. Tricase, G. Dotelli, M. Fiala, V. Siracusa, and C. Mbohwa. 2015. “Energy and Environmental Assessment of Industrial Hemp for Building Applications: A Review.” Renewable and Sustainable Energy Reviews 51: 29–42. doi:10.1016/j.rser.2015.06.002.
- Ingrao, C., F. Scrucca, C. Tricase, and F. Asdrubali. 2016. “A Comparative Life Cycle Assessment of External Wall-compositions for Cleaner Construction Solutions in Buildings.” Journal of Cleaner Production 124: 283–298. doi:10.1016/j.jclepro.2016.02.112.
- Istat, 2021. “ISTAT - Italian Institute of Statistics Several Years”. Available online: www.dati.istat.it (accessed on 25 June 2021).
- Liuzzi, S., S. Sanarica, and P. Stefanizzi. 2017. “Use of Agro-wastes in Building Materials in the Mediterranean Area: A Review.” Energy Procedia 126: 242–249. doi:10.1016/j.egypro.2017.08.147.
- Lo Giudice, A., C. Mbohwa, M. T. Clasadonte, and C. Ingrao. 2014. “Life Cycle Assessment Interpretation and Improvement of the Sicilian Artichokes Production.” International Journal of Environmental Research 8 (2): 305–316.
- Mathew, L., K. U. Joseph, and R. Joseph. 2006. “Isora Fibre: Morphology, Chemical Composition, Surface Modification, Physical, Mechanical and Thermal Properties – A Potential Natural Reinforcement.” J Nat Fibres 3 (4): 13–27. doi:10.1300/J395v03n04_02.
- Mathur, V. K. 2006. “Composite Materials from Local Resources.” Constr Build Mater 20 (7): 470–477. doi:10.1016/j.conbuildmat.2005.01.031.
- Mounir, S., A. Khabbazi, A. Khaldoun, Y. Maaloufa, and Y. El Hamdouni. 2015. “Thermal Inertia and Thermal Properties of the Composite Material Clay-wool.” Sustain. Cities Soc 19: 191–199. doi:10.1016/j.scs.2015.07.018.
- Moussa, T., C. Maalouf, C. Ingrao, F. Scrucca, G. Costantine, and F. Asdrubali. 2018. “Bio-based and Recycled-waste Materials in Buildings: A Study of Energy Performance of Hemp-lime Concrete and recycled-PET Façades for Office Facilities in France and Italy.” Sci. Technol. Built Environ 24 (5): 492–501. doi:10.1080/23744731.2018.1438664.
- Osmani, A., and J. Zhang. 2017. “Multi-period Stochastic Optimization of A Sustainable Multi-feedstock Second Generation Bioethanol Supply Chain − A Logistic Case Study in Midwestern United States.” Land Use Policy 61: 420–445. doi:10.1016/j.landusepol.2016.10.028.
- Pandino, G., S. Lombardo, and G. Mauromicale. 2013. “Globe Artichoke Leaves and Floral Stems as a Source of Bioactive Compounds.” Industrial Crops and Products 44: 44–49. doi:10.1016/j.indcrop.2012.10.022.
- Parlato, M. C. M., and S. M. C. Porto. 2020. “Organized Framework of Main Possible Applications of Sheep Wool Fibres in Building Components.” Sustainability 12 (3): 761. doi:10.3390/su12030761.
- Parlato, M. C. M., F. Valenti, and S. M. C. Porto. 2020. “Covering Plastic Films in Greenhouses System: A GIS-based Model to Improve Post Use Sustainable Management.” Journal of Environmental Management 263: 110389. doi:10.1016/j.jenvman.2020.110389.
- Picuno, P. 2016. “Use of Traditional Material in Farm Buildings for a Sustainable Rural Environment.” Int. J. Sustain. Built Environ 5 (2): 451–460. doi:10.1016/j.ijsbe.2016.05.005.
- Raja, T., P. Anand, M. Karthik, and M. Sundaraj. 2017. “Evaluation of Mechanical Properties of Natural Fibre Reinforced Composites - A Review.” Int J Mech Eng Technol 8: 915–924.
- Rojat, F., M. Olivier, A. Mesbah, and B. Xiao. 2015. “Mechanical Characterization of Natural Fibre-Reinforced Earth Plasters.” In ICBBM 2015 - First Int. Conf. Bio-based Build. Mater, edited by Amziane, Sofiane, Sonebi, Mohammed, and Charlet, Karine, 64-71. Clermont-Ferrand, France: RILEM Publications S.A.R.L.
- Sain, M., and S. Panthapulakkal. 2004. “Green Fibre Thermoplastic Composites.” In Woodhead Publishing Series in Composites Science and Engineering, edited by Ansell, Martin P, 181–206. Sawston, Cambridge, UK: Green Composites, Woodhead Publishing.
- Sanjay, M. R., P. Madhu, M. Jawaid, P. Senthamaraikannan, S. Senthil, and S. Pradeep. 2018. “Characterization and Properties of Natural Fiber Polymer Composites: A Comprehensive Review.” Journal of Cleaner Production 172: 566–581. doi:10.1016/j.jclepro.2017.10.101.
- Sarasini, F., and V. Fiore. 2018. “A Systematic Literature Review on Less Common Natural Fibres and Their Biocomposites.” Journal of Cleaner Production 195: 240–267. doi:10.1016/j.jclepro.2018.05.197.
- Selim, S., D. Koc-San, C. Selim, and B. Taner San. 2018. “Site Selection for Avocado Cultivation Using GIS and Multi-criteria Decision Analyses: Case Study of Antalya, Turkey.” Computers and Electronics in Agriculture 154: 450–459. doi:10.1016/j.compag.2018.09.038.
- Selvaggi, R., and F. Valenti. 2021. “Assessment of Fruit and Vegetable Residues Suitable for Renewable Energy Production: GIS-based Model for Developing New Frontiers within the Context of Circular Economy.” Applied System Innovation 4 (1): 1–15. doi:10.3390/asi4010010.
- Selvaggi, R., F. Valenti, G. Pappalardo, L. Rossi, S. Bozzetto, B. Pecorino, B. E. Dale, et al. 2018. “Sequential Crops for Food, Energy, and Economic Development in Rural Areas: The Case of Sicily.” Biofuels Bioprod Bioref 12 (1): 22–28. DOI:10.1002/bbb.1844.
- Selvaggi, R., F. Valenti, B. Pecorino, and S. M. C. Porto. 2021. “Assessment of Tomato Peels Suitable for Producing Biomethane within the Context of Circular Economy: A GIS-based Model Analysis.” Sustainability 13 (10): 5559. doi:10.3390/su13105559.
- Singh, M., D. A. B. Roy, S. Waseem, and H. Singh. 2021. “Feasibility and Performance Analysis of Carbonated Recycled Aggregate Concrete.” International Journal of Sustainable Engineering 14 (4): 761–775. doi:10.1080/19397038.2020.1856967.
- Thyavihalli Girijappa, Y. G., S. Mavinkere Rangappa, J. Parameswaranpillai, and S. Siengchin. 2019. “Natural Fibers as Sustainable and Renewable Resource for Development of Eco-Friendly Composites: A Comprehensive Review.” Frontiers in Materials 6: 226. doi:10.3389/fmats.2019.00226.
- Turco, R., D. Zannini, S. Mallardo, G. Dal Poggetto, R. Tesser, G. Santagata, M. Malinconico, and M. Di Serio. 2021. “Biocomposites Based on Poly(lactic Acid), Cynara Cardunculus Seed Oil and Fibrous Presscake: A Novel Eco-friendly Approach to Hasten PLA Biodegradation in Common Soil.” Polymer Degradation and Stability 188: 109576. doi:10.1016/j.polymdegradstab.2021.109576.
- Valenti, F., S. M. Porto, G. Chinnici, G. Cascone, and C. Arcidiacono. 2016. “A GIS-based Model to Estimate Citrus Pulp Availability for Biogas Production: An Application to A Region of the Mediterranean Basin.” Biofuels Bioprod Biorefin 10 (6): 710–721. doi:10.1002/bbb.1707.
- Valenti, F., S. M. C. Porto, G. Chinnici, R. Selvaggi, G. Cascone, C. Arcidiacono, and B. Pecorino. 2017. “Use of Citrus Pulp for Biogas Production: A GIS Analysis of Citrus-growing Areas and Processing Industries in South Italy.” Land Use Policy 66: 151–161. doi:10.1016/j.landusepol.2017.04.041.
- Valenti, F., S. M. C. Porto, B. E. Dale, and W. Liao. 2018. “Spatial Analysis of Feedstock Supply and Logistics to Establish Regional Biogas Power Generation: A Case Study in the Region of Sicily.” Renewable and Sustainable Energy Reviews 97: 50–63. doi:10.1016/j.rser.2018.08.022.
- Vasilca, I. S., M. Nen, O. Chivu, V. Radu, C. P. Simion, and N. Marinescu. 2021. “The Management of Environmental Resources in the Construction Sector: An Empirical Model.” Energies 14 (9): 2489. doi:10.3390/en14092489.
- Vitrone, F., D. Ramos, F. Ferrando, and J. Salvad´o. 2021. “Binderless Fiberboards for Sustainable Construction. Materials, Production Methods and Applications.” Journal of Building Engineering 44: 102625. doi:10.1016/j.jobe.2021.102625.
- Wang, H., P. C. Chiang, Y. Cai, C. Li, X. Wang, T. L. Chen, S. Wei, and Q. Huang. 2018. “Application of Wall and Insulation Materials on Green Building: A Review.” Sustainability 10 (9): 3331. doi:10.3390/su10093331.
- Zolfaghary, P., M. Zakerinia, and H. Kazemi. 2021. “A Model for the Use of Urban Treated Wastewater in Agriculture Using Multiple Criteria Decision Making (MCDM) and Geographic Information System (GIS).” Agricultural Water Management 243: 106490. doi:10.1016/j.agwat.2020.106490.
- Zubaryeva, A., N. Zaccarelli, C. Del Giudice, and G. Zurlini. 2012. “Spatially Explicit Assessment of Local Biomass Availability for Distributed Biogas Production via Anaerobic Co-digestion - Mediterranean Case Study.” Renew Energy 39 (1): 261–270. doi:10.1016/j.renene.2011.08.021.