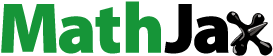
ABSTRACT
Coastal erosion presents a significant environmental threat globally, including in the Northern Java coastal area of Indonesia. As employing hard structures is not an environmentally sustainable practice, nature-based solutions, such as mangrove forests, have emerged as a global trend offering sustainable coastal protection. However, safeguarding young seedlings from wave and current forces remains challenging. Given that temporary structures, such as synthetic geo-bag dikes, considered for protection pose environmental challenges as they degrade slowly, the use of natural fibres such as ramie, unique to Indonesia as a strategic raw commodity, is a sustainable alternative. The study examined ramie fibres (0.28 mm thick) as an eco-friendly geo-bag material through laboratory tests, biodegradability burial tests, and comparisons with other natural fibres. Despite alkali treatment, the strength of ramie fibre did not meet standard geotextile parameters, and its degradability rendered it unsuitable for the expected service life. Ramie would need specific treatments to become a viable alternative for geo-bags. In conclusion, further research is needed to explore how ramie can serve as a sustainable geo-bag material.
1. Introduction
In recent years, coastal erosion has become the most significant hazard affecting human social life and natural ecosystems in coastal areas (Chang, Chu, and Chuang Citation2018). Indonesia, an archipelago country, is experiencing a coastal erosion crisis in the Northern Java coastal area, which bears the most significant erosion risks. Given that 58% of Indonesians live on Java Island, preventing erosion is very crucial (Ekaputri et al. Citation2014; Wahyudi and Adi Citation2018).
Engineering solutions available to address erosion are hard-structure solutions such as breakwaters, groins, dykes, and revetments made of stones, concrete, or sandbags, which have been employed worldwide. The use of natural components for coastal protection aligns with the concept of building with nature, which requires sustainability, multifunctionality, and stakeholder involvement, rather than single-function solutions that are no longer considered acceptable (De Vriend et al. Citation2015). In recent years, several engineers and scientists have considered eco-friendly and sustainable solutions. This approach involves the development of soil and water bioengineering, which has gained popularity worldwide over the years. Soil and water bioengineering is a technology that fosters collaboration between scientists and practitioners to manage ecosystems and achieve maximum benefits for humans and the natural environment. This approach utilises plants as living building materials for natural hazard control and ecological restoration or nature-based reintroduction of species on degraded lands, river embankments, and disturbed environments (Rey et al. Citation2019). Additionally, this approach, which is based on combining live plants and dead materials, is applied to achieve the dual goal of contrasting instability phenomena, both in riverine and earth constructions, while simultaneously limiting the impact on the environment (Bischetti, Di Fi Dio, and Florineth Citation2014). An example of this technique is the usage of mangrove forests for coastal protection.
In tropical and subtropical regions, mangrove forests offer essential ecosystem support for natural regeneration and biosystem processes. Additionally, they provide livelihoods for local communities while significantly contributing to natural resources. Mangroves create a complex ecosystem that boosts substrate stabilisation and dissipates wave energy, allowing the deposition of fine materials (Karimi et al. Citation2022). Studies have extensively explored the effectiveness of mangrove forests in protecting coastlines (Hashim and Catherine Citation2013; Husrin, Strusińska, and Oumeraci Citation2012; Strusinska-Correia, Husrin, and Oumeraci Citation2014). Nevertheless, this approach has its challenges. For example, waves can damage mangrove seedlings before they develop sufficient strength to reduce water level, necessitating protection for at least two years after planting. During storm surges, mangrove seedlings can be submerged under extreme conditions. Therefore, specific protection is necessary during their early stages. To address this challenge, a hybrid coastal-protection system that combines primary natural and temporary artificial structures has been recommended, which is the geo-bag dyke as shown in (Yuanita et al. Citation2020, Citation2021).
Geobags filled with sand or soil are stacked to form a temporary dyke. However, most marketed geo-bags are synthetic and do not degrade easily, becoming a potential waste that threatens the coastal environment. Decommissioning of the geo-bag dyke is necessary to prevent geo-bag marine debris. The geo-bag material, such as natural fibres, should be eco-friendly and easily degradable to offer a sustainable solution. An alternative natural fibre for substituting synthetic geo-bags in Indonesia is ramie fibre, considered the longest, toughest, and silkiest cellulosic fibre (Pandey Citation2008).
Recently, the Indonesian government has promoted ramie fibre as one of its strategic commodities since they can produce ramie fibre in large quantities in various locations in Indonesia. Ramie plants have been recognised in Indonesia since the Japanese occupation in 1942. Government and public interest in these plants with high potential has fluctuated over time. Nowadays, ramie production spans several areas in Indonesia, covering 1,353 hectares and yielding 997 tonnes in 2012. Additionally, tests conducted on Indonesia’s ramie fibre in Japan in 2000 confirmed its adherence to international quality standards, ensuring its acceptance in the global market. In the future, textile-based composites made from ramie fibre are expected to surpass several metal and polymer materials in multiple applications, including the automotive, aerospace, construction, and medical industries (Habibie et al. Citation2022).
The objective of this study is to investigate the feasibility of ramie fibre as an alternative material for a geo-bag in a temporary coastal dyke to protect mangrove seedlings. Additionally, this study is a continuation of previous studies (Gunawan et al. Citation2022; Herbenita et al. Citation2022; Yuanita et al. Citation2020, Citation2021). This study aims to evaluate the performance of ramie fibre by conducting mechanical properties tests in the laboratory, optimising strength with alkali treatment, performing biodegradability burial tests, and comparing the results with the previous studies both in terms of mechanical (Herbenita et al. Citation2022) and biodegradability properties (Gunawan et al. Citation2022).
2. Materials and methods
2.1. Methods
illustrates the methodology of this study. Two investigations were conducted in this study: the first was to study the mechanical properties of ramie before and after alkali treatment, and the second was to study the biodegradation of buried ramie.
Research workflow depicting the sequential process starting from literature review and sample preparation, followed by mechanical tests, alkali treatment, and biodegradation tests. Subsequently, laboratory result analysis and comparison for mechanical properties, biodegradation rate regression and prediction. The conclusion aims to identify the best alternative fabric for sustainable geo-bag material.
2.1.1. Mechanical properties testing
Testing of fabric properties was conducted between June and July, 2022, at the Textile Laboratory of the Center of Textile, Bandung, under the Indonesia Industry Ministry. The testing consisted of thickness and unit mass measurement and mechanical properties testing, such as tensile strength and tear strength. Five samples were tested for every parameter in the laboratory according to standards. shows the standards used in this testing.
Table 1. Laboratory test standard for material characteristics of Ramie Fabric.
Some tests examined ramie samples, which are woven fabrics, from two directions: the warp and the weft. Woven fabric is produced by interlacement at a right angle between two sets of yarns: warp and weft. The warp yarns run longitudinally along the length of the fabric, while the weft yarns run transversely through the fabric (Islam, Chowdhury, and Akter Citation2018). These directions are fundamental for fabric reproduction. illustrates the direction of warp and weft.
Figure 3. Definition of warp (vertical) and weft (horizontal) direction in fabric (Satish et al., Citation2018).
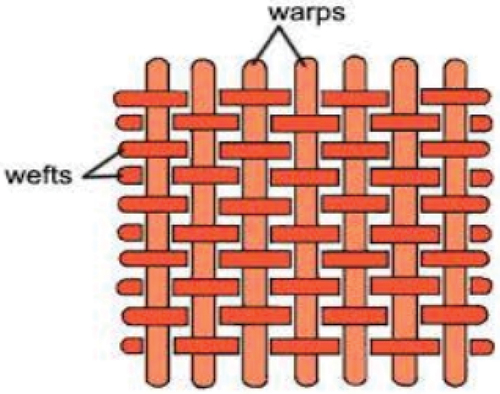
The test results represented the mean value of five samples tested for each trial. The standard error of the mean (SEM) was calculated to ensure the proximity of the mean value to the population. The SEM was determined by dividing the standard deviation by the square root of the sample size, as presented by Equation 1. A higher SEM value indicates a greater dispersion of means in hypothetical studies, while a low SEM value signifies closely clustered mean values within hypothetical studies (Andrade Citation2020).
where:
SE = Standard Error of Mean (SEM)
SD = Standard Deviation
n =Number of Samples
2.1.2. Alkali treatment
Fabric alkali treatments were conducted in the Material Laboratory of the Faculty of Mechanical and Aerospace Engineering, Institut Teknologi Bandung. Alkali treatment (NaOH) on natural fibres is a chemical process that increases cellulose content by removing hemicellulose and lignin (Shukla Citation2016). This treatment involves soaking the ramie sheet in NaOH solution, which increases the durability and ductility of the sheet.
This treatment process involves soaking the 20 cm × 30 cm fabric in NaOH solution at 26°C for 24 h. The NaOH solution was prepared in four concentration variations: 1%, 3%, 5%, and 17.5%. Lower concentrations are typically used as a standard for alkali treatment (Jamilah and Sujito Citation2021; Jin and Maekawa Citation2001; Munawar et al. Citation2008; Witono et al. Citation2014), while the highest concentration, 17.5%, is used as an extreme condition where cellulose has not dissolved yet. Higher concentrations could increase the solubility of cellulose (Wang and Deng Citation2008). Three fabric samples were soaked for each concentration.
2.1.3. Biodegradability testing
Biodegradability testing was conducted in the Ocean Engineering Laboratory of the Faculty of Civil and Environmental Engineering, Institut Teknologi Bandung, following ISO 11721–1: Citation2001 standards. The testing involved burying the ramie sample in soil for approximately 15 weeks, from 27 July 2022, to 9 November 2022.
The method had been utilised in previous similar studies (Gunawan et al. Citation2022; Sülar and Devrim Citation2019). Ramie samples sized 30 cm × 20 cm were prepared, and their mass of the sample was measured using an analytical balance and was assured to be approximately 6.62 grams. All samples were buried at three different depths: 10 cm at the bottom of the container, 5 cm at the centre, and a control sample that remained unburied. Therefore, there were fifteen containers, each containing three samples. During burial, the soil temperature was maintained at 25 ± 5°C and humidity at 95 ± 5%.
Every week, a container was disassembled, and the buried samples were cleaned with water and soaked in 70% alcohol for 30 min, while the control samples were soaked directly in water for the same duration. Subsequently, the samples were dried in an oven at 45 ± 5°C for 24 h and then conditioned at 20 ± 2°C and 65 ± 4% humidity for another 24 h. Finally, the mass of the sample was measured again to calculate the percentage of mass loss.
2.2. Materials
The ramie fabric used in this study was obtained from a textile factory in Bandung, West Java. The required fabric dimension was 10 m in length. The material had not undergone any treatment or bleaching to ensure better and more precise results. displays a photo of the ramie sample used in this study. The fabric was divided into several pieces: 6 m in length for laboratory properties testing and 4 metres for alkali treatment and biodegradability testing.
3. Results and discussions
3.1. Results
The results of this study consists of material properties from laboratory testing, strength and fibre properties after alkali treatment, and biodegradability rate after 15 weeks of testing.
3.1.1. Material properties based on laboratory test
The results of material properties testing in the Center of Textile, Bandung, are shown in
Table 2. Laboratory test results for material characteristics of ramie fabric before treatment.
3.1.2. Alkali treatment results
Based on the observation, the appearance of the detailed fibres after the alkali treatment changed, as shown in . The observed physical changes indicate that the alkali treatment can affect the length of the ramie fibres. Upon examining microscopic photographs, the spacing between the fibres becomes thinner, reducing the size of the fabrics and making them stiffer.
Table 3. Physical changes in ramie fabrics after alkali treatment.
Additionally, tensile strength tests were conducted on the material to measure the changes in tensile strength before and after alkali treatments. The material’s tensile test results are illustrated in , indicating that alkali treatment can impact the characteristics of ramie fibre materials.
Furthermore, for ramie fibres in the machine direction (MD/warp), the maximum tensile strength of the fabric treated with 17.5% NaOH concentration is 21.08% higher than that before treatment. Meanwhile, for the ramie fibres in the cross-machine direction (CD/weft), the highest tensile strength is found in fabrics treated with 1% NaOH concentration, with the strength being 16.9% higher than that before treatment. These findings result from mercerisation-induced swelling in ramie fibres caused by treatment with NaOH.
Four bar charts showing breaking force and elongation at break for different NaOH concentration, i.e. no treatment, 1%, 3%, 5%, and 17.5%, in warp and weft direction.
In addition to testing tensile and tear strength, tests were also conducted to assess changes in fabric grammage. The grammage test results are presented in . Fabric grammage refers to the fabric weight calculated from the length of the thread.
After the alkali treatment, fabrics generally experienced an increase in weight along with an increase in NaOH concentration during treatment. In other words, the higher the concentration of NaOH used, the thicker the fabric and the shorter the thread will be.
Bar chart of ramie grammation in gram/m2 for different NaOH concentration: no treatment, 1%, 3%, 5%, and 17.5%.
3.1.3. Biodegradability test results
The percentage of mass loss of ramie material over 15 weeks is presented in . Based on the biodegradability rate data, a prediction of the time for ramie material to decompose completely was determined. visualise the distribution of biodegradability rates and the corresponding regression curves for the top, middle, and bottom material positions. Linear, polynomial, logarithmic, and exponential equation regressions are employed for approximation.
Figure 7. Scatter plot of ramie mass loss percentage and five possible regression curves: linear, second order polynomial, third order polynomial, logarithmic, and exponential.
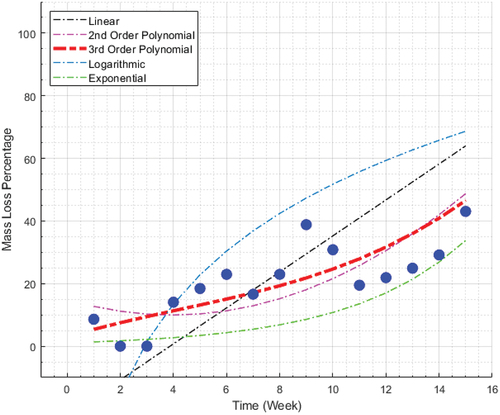
Figure 8. Scatter plot of ramie mass loss percentage and five possible regression curves: linear, second order polynomial, third order polynomial, logarithmic, and exponential.
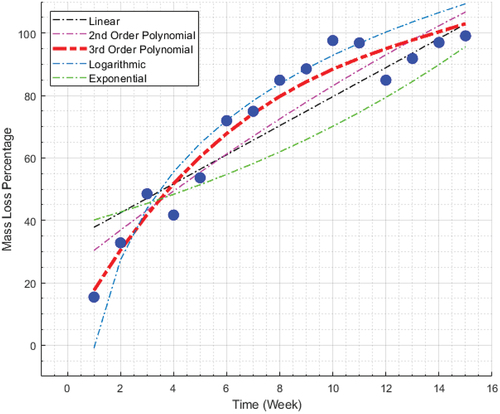
Figure 9. Scatter plot of ramie mass loss percentage and five possible regression curves: linear, second order polynomial, third order polynomial, logarithmic, and exponential.
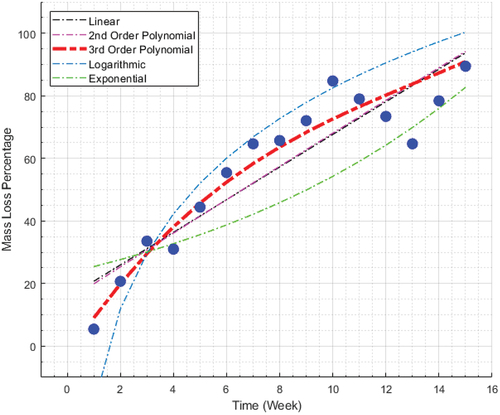
Table 4. Weekly percentage of ramie material mass loss for 15 weeks.
In this study, we observed weight fluctuations over several weeks owing to the use of different containers and samples. Each week, one container was disassembled for inspection; therefore, this study did not utilise continuous samples. Despite receiving the same treatment and conditioning, the results were different. External conditions may also have influenced the samples.
After determining the best-fit equation, the fully degraded time of the material is predicted based on the biodegradability rate and regression results. presents the results of the fully degraded time for each material condition and its corresponding R-squared value. As indicated in , the 3rd-order polynomial regression provides the best-fitted curve for ramie biodegradability rate data, having the highest R-squared value and being visually fitted.
Table 5. Regression results and predictions of the time for ramie material to decomposed completely.
3.2. Discussion
To utilise ramie fibre for geotextile fabric, each material characteristic must fall within the typical range of geotextile standard characteristics. Furthermore, considering that in this study, ramie will be used for temporary structures, the predicted service life of ramie should meet temporary criteria, requiring approximately 2–5 years before complete degradation.
3.2.1. Comparing the ramie properties with the other natural fibres and standard values of geotextile
The comparison of material characteristics was performed between ramie materials and other natural fibre materials previously tested in a study (Herbenita et al. Citation2022), including Tencel, Kenaf, Cotton, and Rayon, alongside typical values of geotextiles, as shown in . The material characteristics include thickness, unit mass, tensile strength (machine and cross direction), and tear strength (machine and cross direction).
Table 6. Strength comparison of natural fibres, Ramie, and typical Geotextile Geobags.
The comparison reveals that the ramie materials used in this study maintain a thickness and weight within the range of typical geotextile values. However, the tensile and tear strength of untreated natural ramie materials still fall below typical geotextile values. In this study, kenaf fabrics fulfil typical standard geotextile parameters due to kenaf’s higher unit mass compared to other natural fibre materials.
3.2.2. Comparing the properties of ramie fibres after alkali treatment with those reported in previous studies as well as with typical geotextile strength
According to the results, alkali treatment of the fibre yields improvements and changes, particularly in visual appearance and strength, by removing the cellulose from the fibre. Ramie fibre becomes denser and more ductile as its concentration increases, while its colour fades with increased concentration. The fibre strength improves in either the warp or weft direction. These findings have been demonstrated in previous studies with both similar and dissimilar fibres (Jamilah and Sujito Citation2021; Jin and Maekawa Citation2001; Munawar et al. Citation2008; Witono et al. Citation2014). A comparison of the results of this study with previous studies is displayed in .
Table 7. Alkali treatment results comparison with previous studies.
In addition, tensile strength tests were conducted on the material to measure the changes in tensile strength before (NT, Non-treatment) and after alkali treatment (1%, 3%, 5%, and 17.5%). presents the results of tensile strength tests before and after alkali treatment, compared to geotextile standard parameters. displays the results of the ramie tensile strength test for each NaOH concentration. It is evident from and that alkali treatment can affect the characteristics of ramie fibre materials. Specifically, for the machine direction (MD/warp), the maximum tensile strength after the fabric was treated with NaOH concentration of 17.5% is 21.08% higher than before treatment. Meanwhile, for the cross-machine direction (CD/weft), the ultimate tensile strength is found in fabrics treated with 1% NaOH concentration, increased by 16.9%. The tensile strength of the ramie sample in this study does not meet the geotextile standard parameters, but with the thicker and heavier ramie fabric, it will meet the standard.
Table 8. Ramie strength comparison of before and after the alkali treatment.
3.2.3. Comparison of the ramie biodegradability rate with other natural fibres
To determine the best alternative geo-bag material, especially for temporary dyke applications that will degrade over time, a comparative study was conducted by comparing the ramie biodegradability rate with other fibres tested in a previous study (Gunawan et al. Citation2022). illustrates the percentage of mass loss over the weeks for various natural fibres placed at the top, middle, and bottom positions.
Figure 10. Scatter plot of comparison between biodegradation rate of five natural fibre samples: cotton, rayon, tencel, kenaf, and ramie.
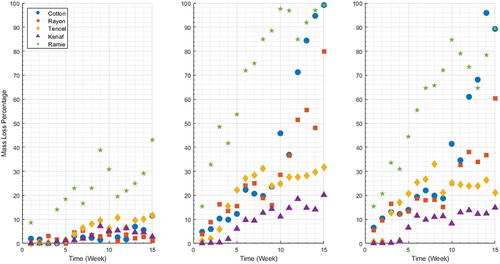
The comparison graph illustrates that ramie fibre exhibits the fastest biodegradability rate among all fibres considered in this study. This is attributed to the fact that the ramie sample was the thinnest compared to the other fibre samples. Sample thickness and mass density influence tensile and tear strength performance. Following this, regression analysis was conducted based on the biodegradability rate of each fibre to obtain the predicted fully degraded duration. The regression equations used in this study include linear, 2nd-order polynomial, 3rd-order polynomial, logarithmic, and exponential regressions. An R-squared value is calculated for each regression equation. presents the predicted fully degraded duration for various types of fibres.
Table 9. Comparison of biodegradability rate regression results of each fibre.
Generally, according to the results of the regression processes, kenaf takes the longest duration to be completely degraded, primarily because the kenaf sample is the thickest and heaviest. Evaluating the graph and R-squared value, the distribution of the biodegradability rate indicates that logarithmic regression provided the closest result. compares the duration predictions for complete degradation of each fibre from the regression results in years.
Table 10. Comparison of biodegradability rate best regression results of each fibre in years.
Based on the regression results in , ramie fibre will be fully degraded within 14 weeks or four months. Conversely, kenaf exhibits the slowest rate due to its thicker structures and double weave usage. However, it approaches the expected service life. To meet the expected service life, adjustments and treatments are necessary, such as using thicker fabric or incorporating additives into the fibre during the finishing process.
4. Conclusion
Ramie has been recognised as a superior eco-friendly alternative to typical geotextile used in geobag dykes for protecting mangrove seedlings from coastal erosion. Ramie holds strategic significance for Indonesia and the government has promoted it as a key commodity. To further explore the potential of ramie, this study aims to investigate its performance through mechanical tests, alkali treatment, and biodegradability tests, comparing the results with other natural fibres such as kenaf, cotton, rayon, and tencel.
Results indicate that ramie exhibits the lowest mechanical properties in tensile strength and the second lowest in tear strength compared to other fibres. Moreover, neither strength falls within the range of typical geotextile strength. However, thickness and unit mass meet the requirements. Despite this, the application of ramie-fibre textile as a geo-bag material remains promising.
Alkali (NaOH) treatment changes the length of ramie fibre and alters its mechanical properties. According to the tensile test results, the fibre length increases with NaOH concentration. The tensile strength of ramie fibres after NaOH treatment varies depending on the alkali concentration. Fibres treated with 17.5% NaOH concentration show the highest maximum warp tensile strength (MD), with a 21.08% increase compared to untreated fibres. In contrast, fibre treated with 1% NaOH concentration exhibit a 16.9% increase in maximum tensile strength in the weft direction (CD). However, this treatment is impractical for ramie fibres to meet typical geotextile standards.
Based on the biodegradability test results, ramie fibre degrades within 15 weeks, which is faster compared to other fibres. Additionally, samples positioned in the middle of the pot are predicted to degrade in approximately 14 weeks. These findings suggest that current ramie may not provide the intended protection for mangrove seedlings throughout their expected service life under the experiment condition. Further treatment is necessary for elaboration.
Laboratory tests of material characteristics demonstrate that, although specific characteristics fall below typical geotextile standard values, ramie fibres have the potential to be employed in constructing geo-bag temporary structures. To meet the mechanical and biodegradable criteria for a geo-bag temporary dyke, thicker and heavier ramie fabric is required.
Since research related to the use of natural fibre for coastal protection is still limited, the possibilities for future topics are vast. Future research topics include technical and construction management aspects, such as creating custom fabric that is thicker and heavier, finding treatments to increase biodegradability, and conducting cost and risk analysis.
Acknowledgments
This study was funded by the ITB Research and Community Service (P2MI) grant through the Offshore and Coastal Engineering Research Groups, Faculty of Civil and Environmental Engineering, Bandung Institute of Technology, Indonesia.
Disclosure statement
No potential conflict of interest was reported by the author(s).
Correction Statement
This article has been republished with minor changes. These changes do not impact the academic content of the article.
Additional information
Notes on contributors
Rildova
Rildova is an associate professor at the Ocean Engineering Program, Faculty of Civil and Environmental Engineering, Institut Teknologi Bandung (ITB), Indonesia. He has a PhD degree in Engineering Mechanics from Virginia Polytechnic Institute and State University, US. He is currently the head of the Offshore Engineering Research Group, Faculty of Civil and Environmental Engineering, ITB.
N Yuanita
Nita Yuanita is an associate professor at the Ocean Engineering Program, Faculty of Civil and Environmental Engineering, Institut Teknologi Bandung (ITB), Indonesia. She has a PhD degree in Civil Engineering from Asian Institute of Technology, Thailand. She is working under the Coastal Engineering Research Group within the Faculty of Civil and Environmental Engineering. She is currently the Vice Dean for Academic Affairs in the Faculty of Civil and Environmental Engineering, ITB.
A Kurniawan
Alamsyah Kurniawan is an assistant professor at the Ocean Engineering Program, Faculty of Civil and Environmental Engineering, Institut Teknologi Bandung (ITB), Indonesia. He has a PhD degree in Civil Engineering from National University of Singapore. He is working under the Coastal Engineering Research Group within the Faculty of Civil and Environmental Engineering. He is currently the Head of the Ocean Engineering Master Program, ITB.
K Kahdar
Kahfiati Kahdar is an associate professor at the Craft Program, Faculty of Art and Design, Institut Teknologi Bandung, Indonesia. She has a PhD in Design from ITB. She is working under the Craft and Tradition Research Group within the Faculty of Art and Design.
Mardiyati
Mardiyati is an assistant professor at the Material Engineering Program, Faculty of Mechanical and Aerospace Engineering, Institut Teknologi Bandung (ITB), Indonesia. She has a PhD degree from Johannes Guttenberg Universitat Mainz, Germany. She is working under the Material Science and Engineering Research Group within the Faculity of Mechanical and Aerospace Engineering. She is currently the Head of the Material Engineering Bachelor Program, ITB.
RMF Fagmi
Rifqi Muhammad Faiq Fagmi has a master degree in Ocean Engineering from Institut Teknologi Bandung (ITB), Indonesia. He is currently a research assistant at the Faculty of Civil and Environmental Engineering, ITB.
E Gunawan
Erika Gunawan has a master degree in Ocean Engineering from Institut Teknologi Bandung (ITB), Indonesia. She is currently working as a project control and planning engineer in an oil and gas consulting company in Jakarta.
R H Rabbani
Raudhy Humaer Rabbani has a master degree in Ocean Engineering from Institut Teknologi Bandung (ITB), Indonesia. He currently works as a project engineer for an oil and gas consulting company in Jakarta.
References
- Andrade, C. 2020. “Understanding the Difference Between Standard Deviation and Standard Error of the Mean, and Knowing When to Use Which.” Indian Journal of Psychological Medicine 42 (4): 409–410. https://doi.org/10.1177/0253717620933419.
- Bischetti, G. B., M. Di Fi Dio, and F. Florineth. 2014. “On the Origin of Soil Bioengineering.” Landscape Research 39 (5): 583–595. https://doi.org/10.1080/01426397.2012.730139.
- Chang, Y., K. W. Chu, and L. Z. H. Chuang. 2018. “Sustainable Coastal Zone Planning Based on Historical Coastline Changes: A Model from Case Study in Tainan, Taiwan.” Landscape and Urban Planning 174:24–32. https://doi.org/10.1016/j.landurbplan.2018.02.012.
- De Vriend, H. J., M. van Koningsveld, S. KAarninkhof, M. de Vries, and M. J. Baptist. 2015. “Sustainable Hydraulic Engineering Through Building with Nature.” Journal of Hydro-Environment Research 9 (2): 159–171. https://doi.org/10.1016/j.jher.2014.06.004.
- Ekaputri, D., W. Windupranata, W. Windupranata, and A. B. Harto. 2014. “The Calculation of Erosion and Sedimentation Rate in Coastal Zone Using Satellite Imageries (Case Study: Kecamatan Muara Gembong, Kabupaten Bekasi.” West Java 3 (2): 17–33.
- Gunawan, W., N. Rildova, N. Yuanita, A. Kurniawan, K. Kahdar, and V. Herbenita. 2022. “Analysis of Biodegradation Characteristics Based on Visual Observation and Mass Loss Percentage in Soil Burial Test of Sustainable Geo-Bag Materials As Temporary Structures in Natural Coastal Protection System.” IOP Conference Series 1065 (1): 012051–012051. https://doi.org/10.1088/1755-1315/1065/1/012051.
- Habibie, S., N. Suhendra, B. Setiawan, M. Hamzah, N. Aisah, D. Fitriani, R. Tasomara, and M. Anggaravidya. 2022. “Prospect of Ramie Fiber Development in Indonesia and Manufacturing of Ramie Fiber Textile-Based Composites for Industrial Needs, an Overview.” International Journal of Composite Materials 11:43–53. https://doi.org/10.5923/j.cmaterials.20211103.01.
- Hashim, A. M., and S. M. P. Catherine. 2013. “A Laboratory Study on Wave Reduction by Mangrove Forests.” APCBEE procedia 5:27–32. https://doi.org/10.1016/j.apcbee.2013.05.006.
- Herbenita, V., N. Yuanita, A. Kurniawan, K. Kahdar, W. Gunawan, and W. Gunawan. 2022. “Comparative of Material Properties Between Natural Fibers and Geo-Bag Synthetic Fibers As Sustainable Material of Temporary Structure in Natural Coastal Protection Systems.” IOP Conference Series 1065 (1): 012055–012055. https://doi.org/10.1088/1755-1315/1065/1/012055.
- Husrin, S., A. Strusińska, and H. Oumeraci. 2012. “Experimental Study on Tsunami Attenuation by Mangrove Forest.” Earth, Planets and Space 64 (10): 973–989. https://doi.org/10.5047/eps.2011.11.008.
- Islam, S., S. Chowdhury, and S. Akter. 2018. “The Experiential Analysis of Woven Fabric for Reproduction.” Journal of Textile Science and Technology 4 (1): 18–48. https://doi.org/10.4236/jtst.2018.41002.
- ISO 11721–1. 2001. Textiles – Determination of Resistance of Cellulose – Containing Textiles to Microorganisms – Soil Burial Test. Switzerland: Technical Committee ISO.
- Jamilah, U. L., and S. Sujito. 2021. “The Improvement of Ramie Fiber Properties as Composite Materials Using Alkalisation Treatment: NaOH Concentration.” Jurnal Sains Materi Indonesia 22 (2): 62. https://doi.org/10.17146/jsmi.2021.22.3.6182.
- Jin, C., and M. Maekawa. 2001. “Evaluating an Enzyme Treatment of Ramie Fabrics.” Textile Research Journal 71 (9): 779–782. https://doi.org/10.1177/004051750107100906.
- Karimi, Z., E. Abdi, A. Deljouei, A. Cislaghi, A. Shirvany, M. Schwarz, and T. C. Hales. 2022. “Vegetation-Induced Soil Stabilisation in Coastal Area: An Example from a Natural Mangrove Forest.” CATENA 216:106410. https://doi.org/10.1016/j.catena.2022.106410.
- Munawar, S. S., K. Umemura, F. Tanaka, and S. Kawai. 2008. “Effects of Alkali, Mild Steam, and Chitosan Treatments on the Properties of Pineapple, Ramie, and Sansevieria Fiber Bundles.” Journal of Wood Science 54 (1): 28–35. https://doi.org/10.1007/s10086-007-0903-y.
- Pandey, S. N. 2008. “Ramie Fibre: Part II. Physical Fibre Properties. A Critical Appreciation of Recent Developments.” Textile Progress 39 (4): 189–268. https://doi.org/10.1080/00405160701706049.
- Rey, F., F. Rey, C. Bifulco, G. B. Bischetti, F. Bourrier, G. De Cesare, F. Florineth, et al. 2019. “Soil and Water Bioengineering: Practice and Research Needs for Reconciling Natural Hazard Control and Ecological Restoration.” Science of the Total Environment 648:1210–1218. https://doi.org/10.1016/j.scitotenv.2018.08.217.
- Satish, A., K. Sobana, and S. B. 2018. “Fabric Texture Analysis and Weave Pattern Recognition by Intelligent Processing.” Journal of Telecommunication, Electronic and Computer Engineering 10 (1–13): 121–127. https://jtec.utem.edu.my/jtec/article/view/4136.
- Shukla, S. K. 2016. “An Introduction to Geosynthetic Engineering.” https://doi.org/10.1201/b21582.
- SNI 0276. 2009. Manual of the Tensile Strength and Elongation of Woven Fabric Tests. Indonesia: BSN.
- SNI ISO 13937–1. 2010. Textiles - Fabric Tear Strength - Part 1: Tear Strength Test Using the Pendulum Method (Elmendorf). Indonesia: BSN.
- SNI ISO 3801. 2010. Textiles – Woven Fabric – Manual of Fabric Weight per Unit Length and Weight per Unit Area Tests. Indonesia: BSN.
- SNI ISO 5084. 2010. Textiles – Manual of Textile and Textile Product Thickness Tests. Indonesia: BSN.
- Strusinska-Correia, A., S. Husrin, and H. Oumeraci. 2014. “ATTENUATION of SOLITARY WAVE by PARAMETERISED FLEXIBLE MANGROVE MODELS.” Coastal Engineering Proceedings 1 (34): 13. https://doi.org/10.9753/icce.v34.management.13.
- Sülar, V., and G. Devrim. 2019. “Biodegradation Behaviour of Different Textile Fibres: Visual, Morphological, Structural Properties and Soil Analyses.” Fibres & Textiles in Eastern Europe 27 (1(133)): 100–111. https://doi.org/10.5604/01.3001.0012.7751.
- Wahyudi, S., and H. P. Adi. 2018. “Evaluating Environment, Erosion and Sedimentation Aspects in Coastal Area to Determine Priority Handling (A Case Study in Jepara Regency, Northern Central Java.” Indonesia 140 (1): 012042. https://doi.org/10.1088/1755-1315/140/1/012042.
- Wang, Y., and Y. Deng. 2008. “Cellulose Fiber Dissolution in Sodium Hydroxide Solution at Low Temperature: Dissolution Kinetics and Solubility Improvement.” Biotechnology and Bioengineering 102 (5): 1398–1405. https://doi.org/10.1002/bit.22160.
- Witono, K., Y. S. Irawan, R. Soenoko, and H. Suryanto. 2014. “Pengaruh Perlakuan Alkali (NaOH) Terhadap Morfologi dan Kekuatan Tarik Serat Mendong.” Jurnal Rekayasa Mesin. 4 (3): 227–234.
- Yuanita, N., M. L. Hakim, A. Kurniawan, M. L. A. Hakim, K. R. Irawan, and N. W. Saputra. 2020. “Physical Model of Natural Coastal Protection System: Geobag-Dyke Performance to Effectiveness of Natural Coastal Protection System.” Journal of Sustainability Science and Management 15 (6): 85–99. https://doi.org/10.46754/jbsd.2020.08.008.
- Yuanita, N., A. Kurniawan, A. Kurniawan, I. M. Nurmansyah, and F. M. Rizaldi. 2021. “A Physical Model Simulation of Combination of a Geo-Bag Dike and Mangrove Vegetation As a Natural Coastal Protection System for the Indonesian Shoreline.” Applied Ocean Research 108:102516. https://doi.org/10.1016/j.apor.2020.102516.