Abstract
Accurate measurement and functional characterization of antibody Fc domain N-linked glycans is critical to successful biosimilar development. Here, we describe the application of methods to accurately quantify and characterize the N-linked glycans of 2 IgG1 biosimilars with effector function activity, and show the potential pitfalls of using assays with insufficient resolution. Accurate glycan assessment was combined with glycan enrichment using lectin chromatography or production with glycosylation inhibitors to produce enriched pools of key glycan species for subsequent assessment in cell-based antibody-dependent cell-mediated cytotoxicity and complement-dependent cytotoxicity effector function assays. This work highlights the challenges of developing high-quality biosimilar candidates and the need for modern biotechnology capabilities. These results show that high-quality analytics, combined with sensitive cell-based assays to study in vivo mechanisms of action, is an essential part of biosimilar development.
Abbreviations
ACN | = | acetonitrile |
ADCC | = | antibody-dependent cell-mediated cytotoxicity |
AMBGs | = | afucosylated monoantennary and biantennary glycans |
AHGs | = | afucosylated hybrid glycans |
BGGs | = | β-galactosylated glycans |
CDC | = | complement-dependent cytotoxicity |
CHO | = | Chinese hamster ovary |
Con A | = | concanavalin A lectin |
CQA | = | critical quality attribute |
EIC | = | extracted ion current |
FT | = | flow-through |
HC | = | heavy chain |
HGs | = | hybrid glycans |
HMGs | = | high-mannose glycans |
HPLC | = | high performance liquid chromatography |
HILIC | = | hydrophilic interaction liquid chromatography |
LC | = | light chain |
mAb | = | monoclonal antibody |
MS | = | mass spectrometry |
MS/MS | = | tandem mass spectrometry |
PQA | = | product quality attribute |
SGs | = | sialylated glycans |
SM | = | starting material |
TRIS | = | tris(hydroxymethyl)aminomethane |
Introduction
Changes in drug regulations in recent years have opened pathways for approval of ‘near-copies’ of recombinant protein drugs, commonly referred to as biosimilars.Citation1 The development of biosimilar drugs is governed by regulations in the US and EU that allow for drug approval based on less extensive clinical trial data than typical of innovator drugs, but with expanded requirements for non-clinical data showing similarity in comparison to the originator product.Citation2 The need for more analytical data has led to a focus on the development of high-resolution analytical and biological characterization methods to support biosimilar product development.
Many of the monoclonal antibody (mAb) therapies approved in the late 1990s and early 2000s, some of which are now targets of biosimilar development, were of the IgG1 isotype.Citation3-5 When these drugs were first approved, aspects of the mechanisms of action (MOA) were not always fully understood. Since then, the MOAs for many of these drugs have been further elucidated.Citation5-7 Such studies have provided a deeper understanding of the effect of product quality attributes (PQAs), such as aggregated species, charge variants, and glycosylation isoforms, on biological function. The PQAs of IgG1 antibodies can affect both target bindingCitation8 and Fc domain-mediated effector function activity, if present.Citation5 At the same time, the past 2 decades have seen substantial improvements in mAb manufacturing.Citation9,10 Advancements in cell line development, bioreactor operation and downstream processing, as well as the implementation of chemically defined media that lack animal-derived materials, have resulted in increased titers and improved product purity and safety, while significantly reducing cost of goods. Biosimilar development requires an understanding of the effects these improved manufacturing processes have on the product quality profiles of biosimilar candidates in comparison to originator drugs. Determination of the criticality of PQAs of both originator and biosimilar candidates and the acceptable variation in critical quality attributes (CQAs) enables development of biosimilars with biochemical and biological properties equivalent to those of the innovator product.
Substantial progress has been made in recent years with regard to understanding the role of antibody effector function on the biology of IgG1 antibody drugs. This activity can take the form of antibody-dependent cell-mediated cytotoxicity (ADCC) or complement-dependent cytotoxicity (CDC) (). ADCC activity is primarily mediated by interaction of Fc with the receptor FcγRIIIa and can be enhanced by specific amino acid polymorphisms in the Fc domain primary sequence.Citation11 Amino acid mutations have also been shown to increase the affinity of Fc for complement in the case of CDC activity.Citation12 Fc glycans occupy the space between the 2 CH2 domains of the Fc dimer,Citation13 existing in a somewhat occluded space that limits the range of N-linked carbohydrates that are observed to an array of predominantly biantennary structures, and to a lesser extent monoantennary, high-mannose, and hybrid structures (). For ADCC activity, the presence of mono- or biantennary Fc glycans without a core fucose residue can dramatically increase affinity of the antibody for FcγRIIIaCitation14-16 and increase cell killing activity. High-mannose glycans include structures with between 5 to 9 mannose residues, and represent a class of less-processed glycans lacking a core fucose residue that have been shown to affect both ADCCCitation16 and CDC activity.Citation19 Hybrid glycans (HGs) are species with one complex arm and 4 or more core mannose residues, and the afucosylated forms have the potential to affect ADCC through increased FcγRIIIa binding. Sialylated glycans (SGs) have been reported to reduce ADCC.Citation20 For antibodies that possess CDC activity, the level of terminal β-galactoseCitation17 on Fc glycans increases cell cytotoxic activity through increased C1q binding.Citation18 β-galactosylated glycans can also increase FcγRIIIa binding,Citation17,21 which could increase ADCC activity if present. Understanding the type and distribution of Fc glycan species for a mAb therapeutic requires the use of analytical techniques that can resolve and quantify a range of forms, including minor species.
Figure 1. ADCC and CDC schematics of activity, key glycan types implicated in modulating Fc-mediated effector function and high-resolution glycan analysis and results. (a) A schematic view of ADCC, where a mAb binds to a target antigen on a tumor cell, binds Fcγ receptors and recruits effector cells (such as NK or macrophages) to kill the target cell; and CDC, where mAb binding to the target antigen and complement recruits a membrane attack complex to kill the target cell. (b) Key glycan groups implicated in effector function. Not all glycan species permutations are shown. For example, biantennary subtypes include A2G0, A2G1 or A2G2 with 0, 1, or 2 terminal β-galactose molecules attached to the GlcNAc, respectively. (c) A comparison of high and low-resolution HILIC glycan assays, with key co-eluting species indicated by the inset. (d) Glycan group % abundance values obtained for Antibody A and Antibody B originator samples. Squares denote Antibody A samples, circles denote Antibody B samples; EU-sourced originator lots are shown in red, US-sourced originator lots are shown in black. The right hand axis denotes BGG levels and the left hand axis denotes levels of the other 4 glycan species. Abbreviations: AMBGs, afucosylated monoantennary and biantennary glycans; AHGs, afucosylated hybrid glycans; HMGs, high-mannose glycans; SGs, sialylated glycans; BGGs, β-galactosylated glycans.
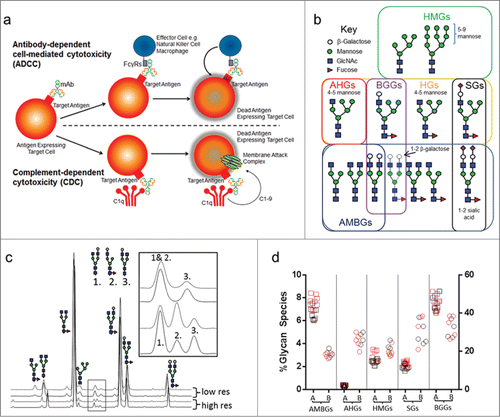
Here, we describe the characterization of glycan attributes for 2 marketed IgG1 antibodies, A and B, and their effect on effector function. Antibody B exhibits both ADCC and CDC activity while Antibody A only exhibits ADCC activity. We developed and applied highly resolving analytical assays in combination with cell-based tests of biological function to evaluate glycan attribute criticality of both biosimilar candidate and originator samples. Detailed analysis of structure/function relationships, as described here, are critical for guiding cell line and process development activities in order to produce a biosimilar with equivalent biological activity and optimal product attribute similarity.
Results
High resolution separation and quantification of N-linked glycans
Hydrophilic interaction liquid chromatography (HILIC) glycan analysis with mass spectrometry (MS) detection was used to accurately quantify and characterize the range of Fc-domain N-linked carbohydrates for Antibodies A and B, and correlate these species with the results of cell-based effector function assays. shows a comparison of 2 HILIC methods, one with typical resolution and one with high resolution, applied to Antibody A and the corresponding US- and EU-approved originator products. Initially, a standard HILIC method with typical resolution was employed, but characterization of the peaks by mass spectrometry showed that this method insufficiently resolved the biantennary afucosylated glycan species A2G1a from a co-eluting fucosylated mono-antennary glycan, A1G1F. Method resolution was improved significantly by increasing the column length and developing a shallower gradient. The improved method resolved a greater number of peaks and provided baseline separation of A2G1a and A1G1F species (, inset). Characterization of this map by assignment of the LC-MS and MS/MS data allowed identification of all of the major and minor peaks in the map, including all 6 possible core afucosylated monoantennary and biantennary glycans (AMBGs) (Fig. S1, upper panel). Accurate detection and quantification of these glycans is critical due to their effect on ADCC activity. A list of the glycan species identified by the method is shown in Table S1.
We applied this highly resolving method to a set of originator product samples for Antibodies A and B and calculated the levels of key glycan groups present on these samples (). To simplify the comparison of different samples and enable glycan structure / function correlations to be drawn from these antibodies, we divided the glycan species into 5 groups organized by glycan function and biosynthesis (): (1) afucosylated mono- and biantennary glycans (AMBGs); (2) high mannose glycans (HMGs); (3) hybrid glycans (HGs); (4) sialylated glycans (SGs); and (5) terminally β-galactosylated glycans (BGGs). BGGs were calculated by summing the level of any glycan with a terminal β-galactose, whether hybrid, monoantennary or biantennary. Triantennary glycans were not detected at significant levels in these antibodies. The levels of AMBGs, AHGs, HMGs and SGs are relatively low (below 10%) for both antibodies,Citation17 while the levels of BGGs are in the range of 30–50%.
Example of attribute criticality: Afucosylated glycans of Antibody A and originator product
Analysis of A1G1F showed that this minor glycan species was present at different levels in Antibody A than in the originator (). Co-elution caused the signal for A1G1F to be included in the AMBG estimate when a low resolution glycan map was used (), resulting in an overestimate in the level of AMBG that was as high as 1.4% for some originator product samples. In contrast, the high resolution glycan map resolved A1G1F from nearby afucosylated species, providing an accurate (and more similar) estimate of the afucosylation levels of all 3 sample sets.
Figure 2. Comparison of A1G1F, AMBG and HMG levels for Antibody A biosimilar candidate and EU- and US-sourced originator samples. (a) Levels of A1G1F. (b) Levels of AMBG determined using the low resolution and high resolution HILIC glycan method. (c) Levels of high mannose glycans. Abbreviations: AMBGs, afucosylated monoantennary and biantennary glycans; HMGs, high-mannose glycans.
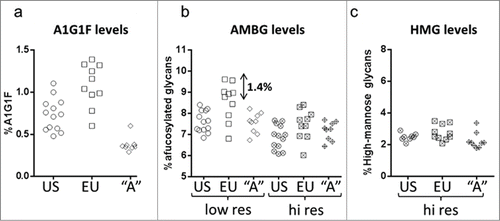
Since small changes in AMBG levels can affect ADCC activity, the accuracy of values provided for this group is essential to biosimilar development. Consistent ADCC results were observed for originator and Antibody A final process samples (), all of which had similar levels of AMBG and HMG species known to affect ADCC (levels were consistent with those shown in ). An experimental test sample, A2, produced with a 1% higher AMBG level showed higher ADCC activity (137%) compared that of the final process samples (range of 82–111%).
Figure 3. ADCC activity examples for Antibody A and originator. (a) ADCC results for Antibody A final process samples (A1) and an experimental sample (A2) compared to the originator samples (EU- and US-sourced). The ADCC activity for Antibody A and originator samples reported is relative to a single lot of Antibody A derived from a process that is representative of the final process used for Antibody A production which has ∼1% higher afucosylated mono and biantennary structures. (b) ADCC activity for Antibody B final process material compared against representative originator material. HMGs in the Antibody B sample were 3.0%, slightly lower than the 4.3% level in the originator product. (c) Representative ADCC dose response curves for Antibody B samples with different levels of afucosylation compared to representative originator material (d) A comparison of the ADCC activity based on EC50 ratios for the reference standard, which is a single lot of originator product, to Antibody B samples in .
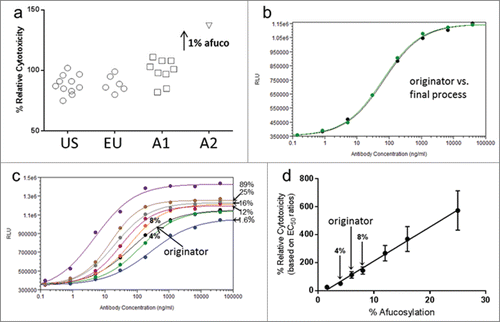
Example of unknown attribute impact: Afucosylated hybrid glycans of Antibody B and originator product
As shown in , the originator product for Antibody B was observed to have low but appreciable levels of AMBGs, HMGs and AHGs, all glycan groups lacking a core fucose with the potential to affect FcγRIIIa binding and ADCC activity. During development of Antibody B, we produced experimental samples of Antibody B with different levels of AMBGs that were assessed for ADCC activity ( and ). Comparison of the EC50 ratios for the samples in shows that a 1% change in% AMBG (% afucosylation) resulted in a 24% increase in ADCC activity (). Samples with 4% and 8% AMBGs had ADCC activity profiles that bracketed representative originator material ( and ). Production of Antibody B using the final process resulted in a sample with 5.8% AMBGs and a nearly identical match in ADCC potency to originator material, with nearly superimposable dose response curves (). As shown in , the final process material of Antibody B, with 5.8% AMBGs, had equivalent ADCC activity to an originator sample with 3.0% AMBGs and 4.5% AHGs, showing that the relative effect of AHGs on ADCC activity was lower than that of AMBGs for this antibody. No affect was observed on the CDC activity of Antibody B with different levels of AMBGs (data not shown), consistent with literature reportsCitation27 that AMBGs do not influence C1q binding for this molecule.
Table 1. Levels of glycans lacking core fucose in originator material and Antibody B 6% mixture comparison
Table 2. EC50 and maximal ADCC values for Antibody B samples with different levels of afucosylation compared to representative originator material
Example of attribute criticality: High mannose glycans of Antibodies A and B
For both Antibodies A and B, the levels of HMGs were within ranges comparable to those of the originator samples shown in . To produce samples with changed levels of HMGs for testing, we used concanavalin A (con A) lectin chromatography, which shows affinity for exposed mannose residues present on HMGs, HGs and mono-antennary glycans (MGs).Citation22
show the levels of AHGs, AMBGs and HMGs in starting material (SM), flow-through (FT) and elution pools from con A chromatography runs for both antibodies. AHGs and HMGs were nearly completely depleted in the FT pools for both samples, while the levels of AMBGs were reduced by no more than 0.8%, likely due to the binding of monoantennary glycans to con A. For Antibody A, the level of HMGs in the elution pool was enriched 5.5 fold, from 3.1% to 16.9%; and for Antibody B, 4.5-fold, from 2.2% to 10.0%. AHGs were also enriched in the elution pool but to a lesser degree: 0.6% to 2.9% for Antibody A and from 0.5% to 1.2% for Antibody B. A slight increase in AMBGs, of 0.4% and 1.0% for Antibody A and Antibody B, respectively, was also measured in the elution pool.
Figure 4. Enrichment of high-mannose glycans and effector function. (a) and (b) Levels of key glycan groups in starting material (SM), and flow-through (FT) and eluate (Elu) pools obtained from concanavalin A (con A) purifications of Antibody A and Antibody B. (c) ADCC results obtained for the starting material and con A pools of Antibody A and Antibody B. (d) CDC results obtained for the starting material and con A pools of Antibody B. In the case of Antibody B, the material used had a relatively low level of AMBGs and AHGs as compared to originator material shown in and . Abbreviations: AMBGs, afucosylated monoantennary and biantennary glycans; AHGs, afucosylated hybrid glycans; HMGs, high-mannose glycans
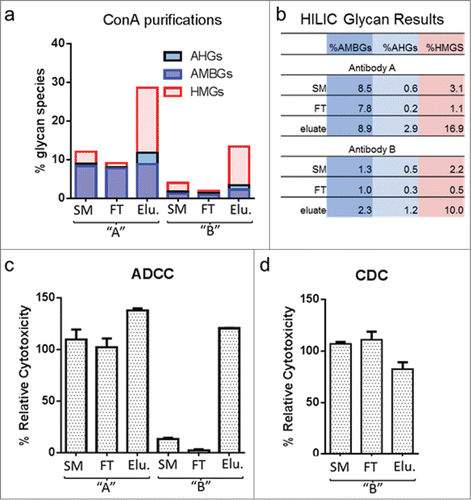
shows the ADCC results for the starting material, FT and elution pools for each antibody. In the case of Antibody A, the elution pool showed an increase in ADCC relative cytotoxicity from 110% to 138%. For the FT pool, where HMG species were depleted, the ADCC activity was similar to that obtained for the starting material when assay variation was considered. The elution sample showed an increase of 13.8% in HMGs, 0.4% in AMBGs, and 2.3% in AHGs compared to the starting material, suggesting that the effect of HMGs on ADCC activity of Antibody A is relatively small when compared to that of AMBGs (). This conclusion is supported by the minimal change in ADCC in the FT pool, where the HMG species were essentially absent. In this experiment, it was not possible to determine the individual effect of AHGs relative to HMGs in the elution pool, but, given that these species are very low in both Antibody A and the originator, the effect of AHGs on ADCC activity is likely less than that for HMGs.
In contrast, for Antibody B, a 4.5-fold increase in HMG species increased ADCC activity from 13% in the SM to 121% in the elution pool. The elution pool did not contain significant levels of AHGs, while the level of AMBGs increased from 1.3 to 2.3%. Given the absence of AHGs and the small change in AMBGs, the majority of the increase in ADCC activity can be attributed to the change in HMGs. (A 1% change in AMBGs resulted in approximately 24% increase in ADCC; see discussed above.) Consistent with this, the FT pool containing only 0.5% HMGs, along with slightly lower AMBGs and AHGs, showed a decrease in ADCC activity, from 13% to 2.4%. Antibody B also possesses CDC activity. The elution pool showed a decrease in CDC activity, from 103% to 83%; a larger data set would be required to confirm this effect, though the result is consistent with literature reports of HM effects on CDC.Citation16 The CDC activity of the FT pool was equivalent to that of the SM.
Example of attribute criticality: β-galactosylated glycans of Antibodies A and B
Glycans with a terminal β-galactose residue are well known to enhance the activity of antibodies with CDC activity through increased C1q binding. Enhanced FcγRIIIa binding has also been observed in samples with high levels of BGGs.Citation21 To examine the effect of BGGs on CDC and ADCC of Antibodies A and B,Citation21 we produced samples with differing levels of BGGs by using the enzyme β-galactosidase to remove terminal β-galactose and β-galactosyltransferase to add β-galactose. All other glycan species in the samples remained unchanged. Samples of enzyme-treated antibodies with high and low levels of BGGs were mixed to produce a defined level of BGGs, purified to remove potentially interfering buffer and enzyme reagents, and analyzed for ADCC and CDC activity.
A clear, linear increase in activity was obtained for Antibody B () for both ADCC and CDC activity when cytotoxicity was plotted against the level of BGGs measured in each sample. A similar dose response was observed with different BGG levels for Antibody A in the ADCC assay (), but dose response curves for samples with 46% or higher levels of BGGs failed the test for parallelism (F-test), thus ADCC activity values relative to the reference standard could not be determined. The larger effect of varying BGG levels on Antibody A ADCC dose response suggests a greater contribution of BGG species to the ADCC activity of Antibody A relative to Antibody B, although the different response could be also be attributed to inherent differences in the ADCC assays for the 2 antibodies (e.g., target cell type, effector:target coincubation times). These data are consistent with literature reports and demonstrate that β-galactose levels should be considered in the context of biosimilar development of antibodies that exhibit effector function. Further investigation of the effect of BGGs on the ADCC activity of Antibody A could be performed to understand the response of remodeled samples relative to the starting material.
Figure 5. Effect of β-galactosylated glycans on ADCC and CDC activity. (a) ADCC activity and (b) CDC activity for samples of Antibody B with differing levels of BGGs. Each point represents the mean ± standard deviation of % Relative Cytotoxicity from 3 independent experiments. All values showed a linear response in the assay and passed all acceptance criteria. (c) ADCC dose response curves for Antibody A samples with different levels of BGGs. For Antibody A, samples with BGG levels 46% and higher yielded ADCC values above the linear range of the assay, necessitating the use of dose response curves to compare activity. Abbreviations: BGGs, β-galactosylated glycans.
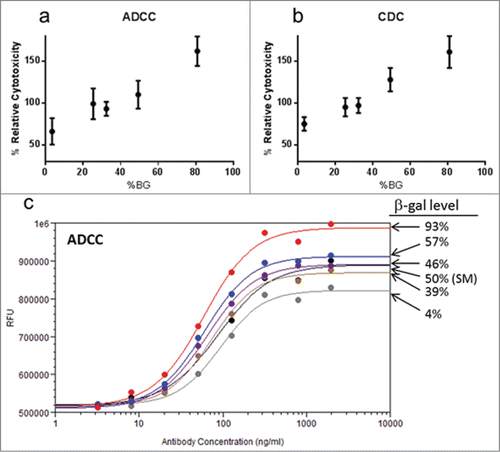
Example of attribute non-criticality: Sialylated glycans of Antibody B
The presence of sialic acid on IgG1 antibodies has been associated with reduced FcγRIIIa binding and ADCC activity.Citation23 Antibody B had levels of SGs ranging from 3.5 to 6.4%. Sialidase treatment of Antibody B to remove sialic acid, followed by re-purification and analysis for both CDC and ADCC activity, showed no impact of these species on activity (data not shown). These findings indicate that sialylation does not appear to play a role in effector function for antibody B, and thus may not be required for the biological function of Antibody B at the levels observed. In Antibody A, the levels of SGs were around 2%, and were not investigated to test the possible effects on Antibody A effector function.
Discussion
Characterization of a sufficient number of originator product lots, using high resolution analytical techniques to accurately determine and quantify PQAs, is necessary to understand the variation in PQAs of the innovator product. Acquisition of originator lots over a sufficient time period, from different regional jurisdictions, to detect changes in PQA ranges due to manufacturing changesCitation24 is also important. Here, we report a comprehensive assessment of the effect of glycan types on effector function for 2 biosimilar antibody therapeutics. Our experiments demonstrate the importance of using a high-resolution glycan assay to accurately define the level and ranges of each glycan species and glycan species group, especially those with known or potential biological impact (AMGBs, AHGs, HMGs, SGs and BGGs), in both originator and biosimilar development samples. Use of techniques with lower resolution, as shown in the example of AMBG quantification for Antibody A, could result in inaccurate determinations of CQA ranges for reference or candidate products, and faulty conclusions about equivalent biological activity and biosimilarity.
Once accurate ranges for originator attributes are established, a comprehensive assessment of the biological impact of these attributes must be conducted to determine if they are critical or non-critical quality attributes.Citation25 In our work, we leveraged the production of antibody samples with varying glycan levels to comprehensively test the effect of these attributes using representative cell-based models of biological function. This approach relies on the availability of relevant and predictive cell-based assays of in vivo activity; in some cases further assessment of the biological impact of a specific attribute in animal studies may be required. For example, differences in HMG glycan levels could affect the pharmacokinetics (PK) of antibody clearance;Citation26 animal PK studies may be needed to understand differences, when present, before studies in humans are initiated.
Attribute function must be tested on a molecule-by-molecule basis, as was demonstrated with our work on the effect of HMGs on the ADCC activity for Antibodies A and B, where the magnitude of the effect was much greater for Antibody B. Antibody B also had significantly lower levels of AMBGs than Antibody A, which may influence the magnitude of the HMG impact. Thus, a comprehensive understanding of the contribution of all glycan species affecting a particular function is important. Where molecules act through multiple mechanisms, as for Antibody B with both CDC and ADCC activities, assessment of attribute impact on both mechanisms of action should be made. In the case of Antibody B, HMGs increased ADCC activity but reduced CDC activity. Furthermore, the effect of multiple attributes on a single function, such as the effect of AMBGs, HMGs, AHGs and BGGs on ADCC activity, must be considered.
The strategy outlined above, if executed properly, should allow clear identification and assessment of the impact of glycan CQAs to be made on a molecule-specific basis. It should also enable construction of target product quality profiles of the reference and biosimilar products that can be used to guide process development decisions during development. These efforts may also reveal information about originator products not well reported in the literature, as was the case with our investigation of the impact of BGGs on the ADCC activity of antibodies A and B.Citation17,21
In conclusion, the work presented here demonstrates the necessity of using fit-for-purpose high resolution analytical assays in combination with cell-based assays of in vivo activity to identify CQAs and assess their importance during biosimilar development. These studies should be applied on a molecule-specific basis, with dose-ranging experiments performed, if possible, to test the effect of the attribute of interest in relevant biological assays. Analytical assays should be carefully assessed for inaccuracies that could result in erroneous assumptions about attribute ranges, which could lead to false conclusions about biosimilarity. The use of affinity chromatography, enzymatic remodeling or protein expression in the presence of glycosylation pathway inhibitors may be necessary to produce enriched pools of specific glycan species in sufficient quantities to allow characterization and bio-functional characterization. Ultimately, a molecule-specific, comprehensive picture of the criticality of Fc-domain N-linked carbohydrates on effector function can only emerge when high-resolution analytical assays are combined with cell-based testing applied to the appropriate test samples.
Materials and Methods
Samples of Antibody A and Antibody B were produced in-house as part of Amgen's biosimilar development program. Commercially-available originator samples were obtained to provide reference material for biosimilar development.
Hydrophilic interaction liquid chromatography analysis of N-linked glycans
100 μg of antibody was digested with PNGase F (2000 U) (New England Biolabs), and the released glycans were fluorescently labeled by addition of 50 μL of 12 mg/mL 2-aminobenzoic acid (Sigma-Aldrich) in 0.2 M sodium cyanoborohydride (EMD) in 4% acetate and 2% boric acid; this mixture was incubated at 80°C for 75 minutes. Labeled glycans were purified from reagents using an Oasis HLB μElution 96-well plate (Waters). The plate was first conditioned using 200 μL of Milli-Q water and then 2 × 200 μL applications of 90% acetonitrile (ACN) using a vacuum system. Labeled glycans are diluted in 600 μL of 100% ACN and then applied to the conditioned plate, and allowed to bind for at least 5 minutes prior to applying a vacuum. Bound glycans were washed with 2 × 200 μL of 90% ACN and then eluted into a collection plate in 2 × 100 μL volumes of 20% ACN. Eluted glycans were evaporated to dryness overnight in a SpeedVac and reconstituted in 15 μL of 50% ACN, before UPLC-HILIC analysis. For the low-resolution method, samples were injected onto a BEH glycan 1.7 μm column (2.1 × 100 mm, Waters) equilibrated with 76% solvent B: 24% solvent A and eluted over 16.2 minutes from 24% to 35.5% solvent A at a flow rate of 0.7 mL/min. For high-resolution HILIC analysis, 3 μg of glycans were bound to a BEH glycan 1.7 μm column (2.1 × 150 mm, Waters) in high organic conditions (78% ACN/22% solvent B:solvent A), where solvent A was 100 mM ammonium formate and solvent B was 100% ACN at a flow rate of 0.2 mL/min. Glycans were eluted over 111.2 minutes from 22% to 40.1% ammonium formate; total analysis time including column re-equilibration was 150 minutes.
Mass spectrometry
MS detection of glycans was performed for the high-resolution method by connection of the eluate of the column to an LTQ mass spectrometer operated in negative ion mode. ESI spray conditions were optimized by tuning on the A2G0F and S2A2G2F glycans. Where necessary, MS/MS fragmentation was used to aid in glycan identification.
Concanavalin A affinity chromatography
Antibody samples were purified on a HiTrap Con A 4B 5 mL column (GE Healthcare) using an Akta Explorer system flowing at 1 mL/min during loading and 3 mL/min during equilibration and elution. Approximately 4 mL of antibody at 18 mg/mL was loaded for each run on a column equilibrated in 10 mM TRIS, 1 mM MgCl2, 1 mM CaCl2, pH 7.2. The flow-through peak was collected after injection of the protein to the column. After loading, the sample was eluted in the equilibration buffer plus 200 mM α-methyl-mannoside (Sigma) and the elution peak collected.
Enyzmatic glycan remodeling
For sialidase A treatment, 100 mg of antibody were combined with 1200 μL of enzyme (≥5 U/mL) (Prozyme), 1200 μL of reaction buffer (250 mM sodium phosphate, pH 6.0), and 4200 μL of Milli-Q water. The enzyme-treated sample was then incubated overnight at 37°C (approx. 18 hours), followed by HILIC preparation as described. For β-galactosidase digestion, 150 mg of protein was digested with 600 μL of β-1,4 galactosidase (Sigma) diluted with supplied reaction buffer and digested overnight at 37°C (approx. 18 hours). For β-galactosyltransferase treatment, 600 μL of enzyme (Sigma) reconstituted in 10 mM TRIS, pH 7.4 was mixed with 150 μL of MnCl2, 3 mL of UDP-galactose (Sigma) and 150 mg of protein at 10 mg/mL. The solution was mixed and included overnight at 37°C (approx. 18 hours). Samples were purified after enzymatic remodeling by Protein A affinity chromatography using MabSelect SuRe (GE Healthcare). Samples were loaded on to a 3 mL self-packed gravity column (made in a Bio-Rad Poly-Prep chromatography disposable column) equilibrated in 25 mM TRIS, 100 mM NaCl, pH 7.4. Protein was adjusted to pH 7.2 using 2.0 M TRIS base, loaded to the column, washed with 15 mLs of equilibration buffer, and eluted in 100 mM acetate, pH 3.6. Samples were adjusted to pH 5.0 using 2.0 M TRIS base. Samples were buffer exchanged as appropriate into formulation buffer using dialysis cassettes. All enzymatic remodeling samples included mock-treated negative controls, which were processed, purified and buffer exchanged along with enzyme treated samples. No difference was observed when compared to untreated samples (data not shown).
Antibody-dependent cell-mediated cytotoxicity assay
ADCC assays were performed using FcγRIIIa (158V)-expressing NK92 (M1) cells as effector cells and appropriate antigen-expressing cells matched to the antibody under study as target cells. For Antibody A, target cells were first labeled with calcein-AM prior to incubating with increasing concentrations of antibody (3.277-2000 ng/ml). Effector cells were then added to opsonized target cells at an E:T ratio of 25:1 for approximately 2 hours. Calcein released from lysed target cells was determined by measuring the fluorescence of the supernatant. The reported cytotoxicity of Antibody A and corresponding originator samples reported is relative to a reference standard, which is a single lot of Antibody A derived from a process that is representative of the final process used for Antibody A production. For Antibody B, target cells were opsonised with increasing concentrations of antibody (0.143 to 40,000 ng/ml) prior to co-incubation with effector cells at an E:T ratio of 25:1 for approximately 3 hours. Adenylate kinase release from lysed target cells was then measured using the ToxiLightTM Bioassay Kit (Lonza). The reported cytotoxicity of Antibody B and corresponding originator samples is relative to a reference standard, which is a single lot of originator product.
For calculation of % relative cytotoxicity, a constrained 4-parameter logistic curve is fitted to the dose response data for the reference standard and for test samples and parallel line analysis is performed using SoftMax Pro software. The % relative cytotoxicity is then calculated as a ratio of the EC50 values for the reference standard to the test sample.
Complement-dependent cytotoxicity assay
The CDC activity of Antibody B was performed using antigen-expressing target cells and baby rabbit complement. Briefly, target cells were labeled with calcein-AM prior to incubating with antibody (1.56 to 200 ng/ml). Baby rabbit complement was then added to target cells for approximately 1 hour. Calcein released from lysed target cells was determined by measuring the fluorescence of the supernatant. The reported cytotoxicity of Antibody B is relative to a reference standard, which is a single lot of originator product. For calculation of % relative cytotoxicity, a constrained 4-parameter logistic curve is fitted to the dose response data for the reference standard and for test samples and parallel line analysis is performed using SoftMax Pro software. The % relative cytotoxicity is then calculated as a ratio of the EC50 values for the reference standard to the test sample.
Disclosure of Potential Conflicts of Interest
No potential conflicts of interest were disclosed.
Supplementary_Material.zip
Download Zip (551.7 KB)Acknowledgments
We gratefully acknowledge Victor Fung and Richard Markus for review and editorial assistance during preparation of the manuscript.
Supplementary Material
Supplemental data for this article can be accessed on the publisher's website.
References
- Kozlowski S, Woodcock J, Midthun K, Sherman RB. Developing the nation's biosimilars program. N Engl J Med 2011; 365:385-8; PMID:21812668; http://dx.doi.org/10.1056/NEJMp1107285
- Kálmán-Szekeres Z, Olajos M, Ganzler K. Analytical aspects of biosimilarity issues of protein drugs. J Pharm Biomed Anal 2012; 69:185-95; http://dx.doi.org/10.1016/j.jpba.2012.04.037
- Carter P, Presta L, Gorman CM, Ridgway JBB, Henner D, Wong WLT, Rowland AM, Kott C, Carver ME, Spepard HM. Humanization of an anti-p185Her2 antibody for human cancer therapy. Proc Natl Acad Sci U S A 1992; 89:4285-9; http://dx.doi.org/10.1073/pnas.89.10.4285
- Knight DM, Trinh H, Le J, Siegel S, Shealy D, McDonough M, Scallon B, Moore MA, Vilcek J, Daddona P, et al. Construction and intial characterization of a mouse-human chimeric anti-TNF antibody. Mol Immunol 1993; 30:1443-53; PMID:8232330; http://dx.doi.org/10.1016/0161-5890(93)90106-L
- Jian X-R, Song A, Bergelson S, Arroll T, Parehk B, May K, Chung S, Strouse R, Mire-Sluis A, Schenerman M. Advances in the assessment and aontrol of the effector functions of therapeutic antibodes. Nat Rev 2011; 10:101-10; PMID:21283105
- Mitoma H, Horiuchi T, Tsukamoto H, Tamimoto Y, Kimoto Y, Uchino A, To K, Harashima S-i, Hatta N, Harada M. Mechanisms of cytoxic effect of anti-tumor necrosis factor agens on transmembrane tumor necrosis factor alpha-expressing cells. Arthiritis Rheumatism 2008; 58:1248-57; http://dx.doi.org/10.1002/art.23447
- Hudis C. Trastuzumab – mechamism of action and use in clinical practice. N Engl J Med 2007; 357:39-51; PMID:17611206; http://dx.doi.org/10.1056/NEJMra043186
- Harris RJ, Kabakoff B, Macchi FD, Shen FJ, Kwong M, Andya JD, Shire SJ, Bjork N, Totpal K, Chen AB. Identification of multiple sources of charge heterogeneity in a recombinant antibody. J Chromatography B 2001; 752:233-45; http://dx.doi.org/10.1016/S0378-4347(00)00548-X
- Hacker DL, De Jesus M, Wurm FM. 25 years of recombinant proteins from reactor-grown cells — Where do we go from here? Biotechnol Adv 2009; 27:1023-7; PMID:19463938; http://dx.doi.org/10.1016/j.biotechadv.2009.05.008
- De Jesus M, Wurm FM. Manufacturing recombinant proteins in kg-ton quantities using animal cells in bioreactors. Eur J Pharm and Biopharm 2011; 78:184-8; PMID:21256214; http://dx.doi.org/10.1016/j.ejpb.2011.01.005
- Lazar G, Dang W, Karki S, Vafa O, Peng J, Hyun L, Chan C, Chung H, Elvazi A, Yoder S, et al. Engineered antibody Fc variants with enhanced effector function. Proc Natl Acad Sci 2006; 103:4005-10; http://dx.doi.org/10.1073/pnas.0508123103
- Moore G, Chen H, Karki S, Lazar G. Engineered Fc variant antibodies with enhanced ability to recruite complement and mediate effector funtions. mAbs 2010; 2:181-9; PMID:20150767; http://dx.doi.org/10.4161/mabs.2.2.11158
- Saphire EO, Stanfield R, Crispin MDM, Parren P, Rudd P, Dwek R, Burton D, Wilson I. Contrasting IgG structures reveal extreme asymmetry and flexibility. J Mol Biol 2002; 319:9-18; PMID:12051932; http://dx.doi.org/10.1016/S0022-2836(02)00244-9
- Kanda Y, Yamada T, Mori K, Okazaki A, Inoue M, Kitajima-Miyama K, Kuni-Kamochi R, Nakano R, Yano K, Kakita S, et al. Comparison of biological activity among nonfucosylated therapeutic IgG1antibodies with three different N-linked Fc oligosaccharides: the high-mannose,hybrid, and complex types. Glycobiology 2006; 17:104-18; PMID:17012310; http://dx.doi.org/10.1093/glycob/cwl057
- Niwa R, Natsume A, Uehara A, Wakitani M, Iida S, Uchida K, Satoh M, Shitara K. IgG subclass-independent improvement of antibody-dependent cellular cytotoxicity by fucose removal from Asn297-linked oligosaccharides. J Immunol Methods 2006; 306:151-60; http://dx.doi.org/10.1016/j.jim.2005.08.009
- Yu M, Brown D, Reed C, Chung S, Lutman J, Stefanich E, Wong A, Stefan J-P, Bayer R. Production, characterization and pharmacokinetic properties of antibodies with N-linked Mannose-5 glycans. mAbs 2012; 4:475-87; PMID:22699308; http://dx.doi.org/10.4161/mabs.20737
- Kumpel B, Rademacher T, Rook G, Williams P, Wilson I. Galactosylation of human IgG monoclonal anti-D produced by EBV-transformed B-lymphoblastoid cell lines is depedent on culture media and affects Fc receptor-mediated functional activity. Hum Antibodies Hybridomas 1994; 5:143-51; PMID:7756579
- Hodoniczky J, Zheng YZ, James D. Control of recombinant monoclonal antibody effector functions by Fc N-glycan remodeling in vitro. Biotechnol Prog 2005; 21:1644-52; PMID:16321047; http://dx.doi.org/10.1021/bp050228w
- Wright A, Morrison S. Effect of altered CH2-associated carbohydrate structure on the functional properties and in vivo fate of chimeric mouse-human immunoglobulin G1. J Exp Med 1994; 180:1087-96; PMID:8064227; http://dx.doi.org/10.1084/jem.180.3.1087
- Anthony R, Nimmerjahn F, Ashline D, Renhold V, Paulson J, Ravetch J. A recombinant IgG Fc that recapitulates teh anti-inflammitory activity of IVIG. Science 2008; 320:373-6; PMID:18420934; http://dx.doi.org/10.1126/science.1154315
- Houde D, Peng Y, Berkowitz S, Engen J. Post-translational modifications differentially effect IgG1 conformation and receptor binding. Mol Cell Proteomics 2010; 9:1716-28; PMID:20103567; http://dx.doi.org/10.1074/mcp.M900540-MCP200
- Malaise M, Franchimont P, Bouillene C, Houssier C, Mahieu P. Increased concanavalin A-binding capacity of immunoglobulin G purified from sera of patients with rheumatoid arthritis. Clin Exp Immunol 1987; 68:543-51
- Kaneko Y, Nimmerjahn F, Ravetch J. Anti-inflammatory activity of immunoglobulin G resulting from Fc sialylation. Science 2006; 313:670-3; PMID:16888140; http://dx.doi.org/10.1126/science.1129594
- Visser J, Feuerstein I, Stangler T, Schmiederer T, Fritsch C. Physicochemical and functional comparability between the proposed biosimilar rituximab GP2013 and originator rituximab. BioDrugs 2013; 27:495-507; PMID:23649935; http://dx.doi.org/10.1007/s40259-013-0036-3
- Rathore A, Winkle H. Quality by design for biopharmaceuticals. Nat Biotechnol 2009; 27:26-34; PMID:19131992; http://dx.doi.org/10.1038/nbt0109-26
- Goetze A, Liu Y, Zhang Z, Shah B, Lee E, Bondarenko P, Flynn G. High-mannose glycans on the Fc region of therapeutic IgG antibodies increase serum clearance in humans. Glycobiology 2011; 21:949-69; PMID:21421994; http://dx.doi.org/10.1093/glycob/cwr027
- Chung S, Quarmby V, Gao X, Ying Y, Lin L, Reed C, Fong C, Lau W, Qui Z, Shen A, et al. Quantitative evaluation of fucose reducing effects in a humanized antibody on FcGamma receptor biniding and antibody-dependent cell-mediated cytotoxicity activities. mAbs 2012; 4:326-40; PMID:22531441; http://dx.doi.org/10.4161/mabs.19941