ABSTRACT
The neonatal Fc receptor (FcRn) is a homeostatic receptor responsible for prolonging immunoglobulin G (IgG) half-life by protecting it from lysosomal degradation and recycling it to systemic circulation. Tissue-specific FcRn expression is a critical parameter in physiologically-based pharmacokinetic (PBPK) modeling for translational pharmacokinetics of Fc-containing biotherapeutics. Using online peptide immuno-affinity chromatography coupled with high resolution mass spectrometry, we established a quantitative FcRn tissue protein expression profile in human FcRn (hFcRn) transgenic mice, Tg32 homozygous and hemizygous strains. The concentration of hFcRn across 14 tissues ranged from 3.5 to 111.2 pmole per gram of tissue. Our hFcRn quantification data from Tg32 mice will enable a more refined PBPK model to improve the accuracy of human PK predictions for Fc-containing biotherapeutics.
Abbreviations
PK | = | Pharmacokinetics |
FcRn | = | neonatal Fc receptor |
hFcRn | = | human FcRn |
IgG | = | immunoglobulin G |
IA-LC-HRMS | = | immuno-affinity liquid chromatography high resolution mass spectrometry |
PBPK model | = | physiologically-based pharmacokinetic model |
The neonatal Fc receptor (FcRn) is a heterodimer composed of an α-chain and β2-microglobulin (β2m), known to be involved in various important biological functions throughout human life.Citation1 One critical function is the FcRn-mediated recycling process, occurring mainly in haematopoietic cells, endothelial and epithelial cells, which maintains serum IgG and albumin homeostasis and influences the circulation half-life of Fc-containing therapeutic proteins.Citation2,3 Physiologically-based pharmacokinetic (PBPK) models estimate the PK of drug after its administration. However, a current limitation of using a PBPK model for large molecule PK predictions is the lack of information of localized FcRn concentrations and FcRn recycling rates.Citation4 Using measured concentrations of therapeutic antibody in plasma and tissues, several recent PBPK reports estimated average mouse FcRn concentrations to range from 20.1 to 49.8 µM.Citation5-8 Concurrent with the development of a physiologically-based mechanistic FcRn model, the accuracy of PK projections for monoclonal antibodies and antibody-directed enzyme prodrug therapy have improved.Citation9,10 Furthermore, the above model can be extended to include the pharmacodynamic (PD) effect and efficacy following drug administration in order to integrate their prediction with the estimation of PK of therapeutic antibodies.Citation11,12 In this study, we experimentally determined tissue-specific hFcRn concentrations in Tg32 transgenic mice, a key missing parameter to be integrated into PBPK models. This data now provides the needed resolution of the PBPK modeling system to the individual tissue level, which is anticipated to improve prediction accuracy and to enable a better a priori understanding of Fc-containing biotherapeutics in vivo.
A quantitative hFcRn tissue assay was developed in Tg32 transgenic mice.Citation13 The workflow includes tissue extraction, protein precipitation, pellet digestion with trypsin and measurement of a hFcRn tryptic surrogate peptide by peptide immuno-affinity liquid chromatography coupled with high resolution mass spectrometry (IA-LC-HRMS). A polyclonal antibody was generated in rabbits against the hFcRn immunogen peptide, GDDTGVLLPTPGEAQDADLK, derived from the cytosolic domain of hFcRn protein. Tg32 mice lack mouse FcRn α-chain and are transgenic for either one (hemizygous) or 2 copies (homozygous) of the hFcRn α-chain under the control of human endogenous promotor.Citation14 Though sequence similarity between human and mouse β2m is relatively high (77%), the effect of β2m on the PK of a human IgG was investigated in Tg32 mice expressing transgenic hFcRn and mouse endogenous β2m (Tg32) or Tg32 mice expressing both transgenic hFcRn and human β2m in the presence of mouse endogenous β2m (Tg32/hβ2m).Citation15 The results showed no detectable differences in half-lives between Tg32 and Tg32/hβ2m mice, indicating functional human-mouse hybrid FcRn complexes were formed in the Tg32 transgenic mice systems.Citation15 PK studies in Tg32 hemizygous mice also showed high correlation of antibody clearance to human (Pearson r = 0.99, p< 0.001), suggesting that Tg32 could serve as an animal model for translational PK of Fc-containing biotherapeutics.Citation16 Therefore, establishing a quantitative hFcRn protein tissue expression profile in Tg32 will provide a key parameter for translational PBPK models for human PK predictions.
We analyzed a panel of 14 different tissues from hemizygous and homozygous strains of Tg32 transgenic mice (15-18 weeks of age, male, N = 5 each). All procedures were executed within accordance of the Animal Use Protocol (AUP) and adherence to Pfizer institutional animal care and use committees (IACUC) regulations. Tissues were collected 7 weeks after dosing of an IgG at 5 mg/kg. Epididymal fat, spleen, brain, heart, mesenteric lymph node, kidney, liver, lung, pancreas, skeletal muscle of quadriceps, skin, stomach, small intestine, and large intestine were collected, weighted, and snap frozen in liquid nitrogen. The content in stomach, small and large intestine was removed prior to tissue collection. Details of sample preparation, analytical approaches, and assay qualification were previously described.Citation13 In brief, the assay was qualified with a quantification range of 1.76 – 900 ng/ml, equivalent to 0.89 – 452.70 pmol/g tissue, and a calibration curve was prepared using full length recombinant hFcRn (MW 39.74 kDa) spiked into control mouse liver lysate as an analyte-free, surrogate tissue matrix.Citation13 A standard curve was obtained by plotting peak area ratio against hFcRn concentration (Fig. S1).
In order to establish extraction reproducibility and understand tissue heterogeneity of hFcRn expression, selected single tissues (lung, muscle, small and large intestine) were dissected into 3-4 pieces for independent lysate preparation. shows total protein concentration measured by absorbance at 280 nm, and the coefficients of variation (%CVs) were found to be less than 20% from all dissected tissues from both homozygous and hemizygous Tg32 mice. Human FcRn concentrations quantified by IA-LC-HRMS in the lysate from the dissected pieces of each tissue showed little variability in lung (%CV < 20%), whereas muscle showed slightly higher variability (%CV < 30%) (). Tissues are composed of different cell types, which constitute the tissue architecture and can result in location-dependent heterogeneity of protein expression, leading to variable protein quantification data in dissected tissue samples. FcRn levels in human intestine, measured by ligand binding assay, were reported to be higher in proximal colon compared to jejunum.Citation17 We also observed differences in hFcRn concentration in dissected pieces of small and large intestine (), suggesting a higher degree of tissue heterogeneity of hFcRn expression in intestine. In addition to extraction reproducibility, we measured extraction efficiency by digesting with trypsin any tissue debris that remained following the bead homogenization step. Human FcRn peptides recovered from debris were 4.8%, 0.8%, and 0.1%, for lung, liver, and small intestine respectively, compared to hFcRn peptides measured in the extracts from the same tissue. Thus, the hFcRn extraction efficiency was more than 95% from solid tissues into tissue lysate.
Figure 1. Tissue extraction reproducibility and heterogeneity from independent lysate preparation of dissected tissue pieces resulting from the same lung, muscle, small and large intestine samples from both homozygous and hemizygous mice showing (A) protein concentration and (B) hFcRn expression. Protein concentration was determined by absorbance at 280 nm.
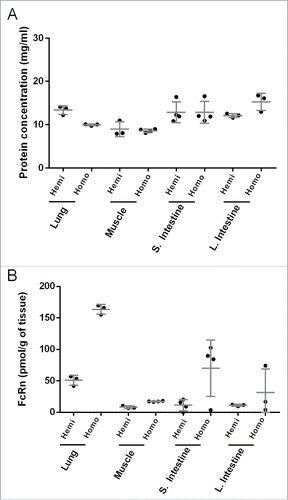
With the understanding of quantitative assay performances,Citation13 including the demonstration of tissue extraction efficiency, reproducibility and bias, a hFcRn tissue expression profile in Tg32 transgenic mice was generated. Human FcRn concentrations obtained from Tg32 homozygous and hemizygous mice are shown in and , respectively. The average of hFcRn tissue expression normalized by tissue weight, and the ratio between homozygous and hemizygous are shown in , where tissues with significant differences in hFcRn expression between both strains are indicated by asterisks. Using Mann-Whitney test to compare the hFcRn concentration obtained in Tg32 hemizygous and homozygous mice, tissues with statistical significant differences of p < 0.05 were lymph node, lung, skin, stomach, heart, kidney, skeletal muscle and brain, indicated by double asterisks (**), whereas tissues with statistical significant differences of p < 0.1 were liver and large intestine, indicated by single asterisks (*). No significant difference was observed in spleen, small intestine and epididymal fat. The average hFcRn expression ratio between homozygous and hemizygous Tg32 mice strains was 1.95 fold. Human FcRn expression in Tg32 mice pancreas was below the limit of quantification, but detectable from tissue matrices (), suggesting hFcRn concentration in pancreas was less than 0.89 pmol/g of tissue. Human FcRn tissue expression normalized by total protein concentration is shown in Table S1, and tissue protein concentration determined by absorbance at 280 nm is shown in Table S2. Though there are 2 copies of hFcRn α-chain genes in the homozygous mouse strain, the expression quantity of endogenous mouse β2m (mβ2m) may become a limiting factor in the formation of an active FcRn complex. The functionality of IgG binding to hybrid FcRn complex in transgenic mouse carrying multiple copies of bovine FcRnCitation18 or human FcRnCitation16 suggested active FcRn complex formation with mβ2m. Quantitative evidence of β2m expression level came from the study of human trophoblast-derived cells, which total protein expression of endogenous β2m increased by 120% comparing hFcRn overexpression cells and the parental cells using immunoblotting analysis.Citation19 It will be of interest to pursue and understand the expression level of tissue-specific mβ2m as well as other factors that result in the hFcRn expression differences in the 2 genotypes of the Tg32 strains.
Figure 2. Human FcRn tissue expression profile in (A) Tg32- homozygous and (B) Tg32-hemizygous mice.
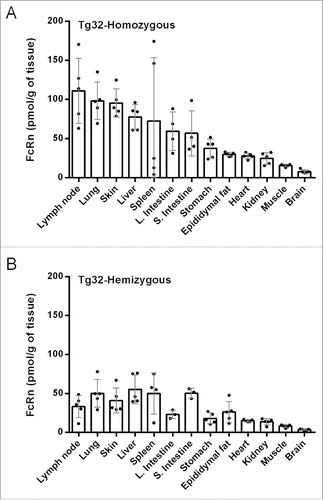
Table 1. FcRn tissue expression of human FcRn transgenic mice, Tg32, normalized by tissue weight. (N = 5) Using Mann-Whitney test, tissues with p < 0.05 are indicated by double asterisks (**); p < 0.1 are indicated by single asterisks (*).
In the measurement of hFcRn expression across 14 tissues, lymph node, lung and skin showed highest expression of hFcRn (range of 33.3 – 111.2 pmol/g tissue). The high expression of hFcRn in lung has been considered a key factor for investigating a pulmonary delivery route for Fc-fusion proteins or monoclonal antibodies.Citation20 Delivery of erythropoietin-Fc (EpoFc) fusion protein by inhalation in a human Phase 1 clinical study showed ∼70% of the Epo-Fc was delivered safely and effectively to the central lung region.Citation21 Furthermore, a mouse FcRn-incorporated PBPK model predicted that the skin could account for 33% of the total IgG elimination.Citation6 Our data support this prediction by taking into account that skin takes a large percentage of body weight.Citation22,23 Unlike other tissues, FcRn expression in the brain has been in dispute. Studies from blood and brain tissue analysis of 125I-labeled IgG intravenously administered into WT and FcRn KO mice showed no significant differences in the AUC (area under the curve of IgG concentration) ratio between brain and blood.Citation24-26 Interestingly, studies using methods to localize dosed antibodies via immunohistochemistry and confocal microscopy of antibody against rat FcRn α-chain in rat brain seemed to suggest that FcRn enabled the transport of monoclonal antibodies across the blood-brain barrier. The likely mechanism is reverse transcytosis in brain microvascular endothelial cells that form the blood brain barrier, enabling the efflux of IgG from the brain.Citation27,28 We used our IA-LC-HRMS approach to measure hFcRn expression in the Tg32 mice brain. Although hFcRn concentrations were relatively low compared to other tissues, they were clearly quantifiable in hemizygous (3.5 pmol/g of tissue) and homozygous (8.0 pmol/g of tissue) mice. In future studies, it will be of interest to establish whether FcRn expression in the human brain is comparable to the transgenic mice. In a systemic administration study, the contribution of hFcRn on IgG tissue distribution has been reported by co-dosing 125I-labeled IgG and 111In-labeled IgG indicating that liver, spleen, skin and muscle play important roles in IgG catabolism.Citation29 Our report and previous studies show important connections between FcRn expression and the understanding of biodistribution for therapeutic monoclonal antibodies, antibody-drug conjugates, Fc-fusion and albumin fusion proteins.
FcRn measurements in some selected tissues have previously been carried out via ligand binding assay,Citation17 protein immunoblotting,Citation17,30 tissue staining,Citation31 or transcriptional analysis from various cultured cell types.Citation32 However, to the best of our knowledge, systematic quantification of FcRn across tissues has not been reported, which is likely due to the technical challenges associated with tissue-based bioanalysis of membrane proteins. The challenges for endogenous membrane protein quantification across various tissue types include maximizing tissue extraction efficiency, ensuring high assay sensitivity, avoiding tissue matrices interference, and establishing rigorous quantification performances of the assay.Citation33,34 Our quantification strategy by protein pellet digestion and online peptide immuno-affinity enrichment is suitable for endosomal and cell-surface hFcRn, i.e., total hFcRn quantification, bypassing challenges of protein enrichment in a membranous environment using anti-FcRn antibodies. Such an approach coupled with high resolution mass spectrometry has enabled this tissue-based hFcRn assay to be highly selective and sensitive with robust quantification performance.
There are broad clinical applications for biotherapeutic drug development that involve targeting FcRn due to its function in the half-life extension of albumin or Fc-fusion proteins, and monoclonal antibodies,Citation35,36 including delivery of therapeutic modalities via pulmonary adsorption.Citation37 Other applications that have been extensively studied include intravenous immunoglobulin administration for autoimmune disease by saturating FcRn to reduce the level of circulating autoantibodies,Citation38 and evaluation of the risk on off-target toxicities of antibody-drug conjugate by antigen-independent internalization via FcRn into lysosomes.Citation39 To accurately understand hFcRn tissue concentrations is fundamental in these application areas and this study provided experimentally verified hFcRn concentrations in a translatable Tg32 transgenic mice system that has potential to be employed to provide PK estimations for humans.
Materials and methods
Material, methods and associated references are available as supplementary material.
Disclosure of potential conflicts of interest
No potential conflicts of interest were disclosed.
Supplementary_Data.docx
Download MS Word (297.3 KB)References
- Roopenian DC, Akilesh S. FcRn: the neonatal Fc receptor comes of age. Nat Rev Immunol 2007; 7:715-25; PMID:17703228; http://dx.doi.org/10.1038/nri2155
- Junghans RP, Anderson CL. The protection receptor for IgG catabolism is the beta2-microglobulin-containing neonatal intestinal transport receptor. Proc Natl Acad Sci U S A 1996; 93:5512-6; PMID:8643606; http://dx.doi.org/10.1073/pnas.93.11.5512
- Challa DK, Velmurugan R, Ober RJ, Sally Ward E. FcRn: from molecular interactions to regulation of IgG pharmacokinetics and functions. Curr Top Microbiol Immunol 2014; 382:249-72; PMID:25116104; http://dx.doi.org/10.1007/978-3-319-07911-0_12
- Jones HM, Chen Y, Gibson C, Heimbach T, Parrott N, Peters SA, Snoeys J, Upreti VV, Zheng M, Hall SD. Physiologically based pharmacokinetic modeling in drug discovery and development: a pharmaceutical industry perspective. Clin Pharmacol Ther 2015; 97:247-62; PMID:25670209; http://dx.doi.org/10.1002/cpt.37
- Ferl GZ, Wu AM, DiStefano JJ, 3rd. A predictive model of therapeutic monoclonal antibody dynamics and regulation by the neonatal Fc receptor (FcRn). Ann Biomed Eng 2005; 33:1640-52; PMID:16341929; http://dx.doi.org/10.1007/s10439-005-7410-3
- Garg A, Balthasar JP. Physiologically-based pharmacokinetic (PBPK) model to predict IgG tissue kinetics in wild-type and FcRn-knockout mice. J Pharmacokinet Pharmacodyn 2007; 34:687-709; PMID:17636457; http://dx.doi.org/10.1007/s10928-007-9065-1
- Shah DK, Betts AM. Towards a platform PBPK model to characterize the plasma and tissue disposition of monoclonal antibodies in preclinical species and human. J Pharmacokinet Pharmacodyn 2012; 39:67-86; PMID:22143261; http://dx.doi.org/10.1007/s10928-011-9232-2
- Xiao JJ. Pharmacokinetic models for FcRn-mediated IgG disposition. J Biomed Biotechnol 2012; 2012:282989; PMID:22665983; http://dx.doi.org/10.1155/2012/282989
- Urva SR, Yang VC, Balthasar JP. Physiologically based pharmacokinetic model for T84.66: a monoclonal anti-CEA antibody. J Pharm Sci 2010; 99:1582-600; PMID:19774657; http://dx.doi.org/10.1002/jps.21918
- Fang L, Sun D. Predictive physiologically based pharmacokinetic model for antibody-directed enzyme prodrug therapy. Drug Metab Dispos 2008; 36:1153-65; PMID:18356268; http://dx.doi.org/10.1124/dmd.107.019182
- Suzuki T, Ishii-Watabe A, Tada M, Kobayashi T, Kanayasu-Toyoda T, Kawanishi T, Yamaguchi T. Importance of neonatal FcR in regulating the serum half-life of therapeutic proteins containing the Fc domain of human IgG1: a comparative study of the affinity of monoclonal antibodies and Fc-fusion proteins to human neonatal FcR. J Immunol 2010; 184:1968-76; PMID:20083659; http://dx.doi.org/10.4049/jimmunol.0903296
- Chetty M, Li L, Rose R, Machavaram K, Jamei M, Rostami-Hodjegan A, Gardner I. Prediction of the Pharmacokinetics, Pharmacodynamics, and Efficacy of a Monoclonal Antibody, Using a Physiologically Based Pharmacokinetic FcRn Model. Front Immunol 2014; 5:670; PMID:25601866; http://dx.doi.org/10.3389/fimmu.2014.00670
- Fan YY, Neubert H. Quantitative analysis of human neonatal Fc receptor (FcRn) tissue expression in transgenic mice by online peptide immuno-affinity LC-HRMS. Anal Chem 2016; 88(8):4239-47; http://dx.doi.org/10.1021/acs.analchem.5b03900
- Roopenian DC, Christianson GJ, Sproule TJ, Brown AC, Akilesh S, Jung N, Petkova S, Avanessian L, Choi EY, Shaffer DJ, et al. The MHC class I-like IgG receptor controls perinatal IgG transport, IgG homeostasis, and fate of IgG-Fc-coupled drugs. J Immunol 2003; 170:3528-33; PMID:12646614; http://dx.doi.org/10.4049/jimmunol.170.7.3528
- Proetzel G, Roopenian DC. Humanized FcRn mouse models for evaluating pharmacokinetics of human IgG antibodies. Methods 2014; 65:148-53; PMID:23867339; http://dx.doi.org/10.1016/j.ymeth.013.07.005
- Tam SH, McCarthy SG, Brosnan K, Goldberg KM, Scallon BJ. Correlations between pharmacokinetics of IgG antibodies in primates vs. FcRn-transgenic mice reveal a rodent model with predictive capabilities. MAbs 2013; 5:397-405; PMID:23549129; http://dx.doi.org/10.4161/mabs.23836
- Hornby PJ, Cooper PR, Kliwinski C, Ragwan E, Mabus JR, Harman B, Thompson S, Kauffman AL, Yan Z, Tam SH, et al. Human and non-human primate intestinal FcRn expression and immunoglobulin G transcytosis. Pharm Res 2014; 31:908-22; PMID:24072267; http://dx.doi.org/10.1007/s11095-013-1212-3
- Bender B, Bodrogi L, Mayer B, Schneider Z, Zhao Y, Hammarstrom L, Eggen A, Kacskovics I, Bosze Z. Position independent and copy-number-related expression of the bovine neonatal Fc receptor alpha-chain in transgenic mice carrying a 102 kb BAC genomic fragment. Transgenic Res 2007; 16:613-27; PMID:17594529; http://dx.doi.org/10.1007/s11248-007-9108-9
- Ellinger I, Reischer H, Lehner C, Leitner K, Hunziker W, Fuchs R. Overexpression of the human neonatal Fc-receptor alpha-chain in trophoblast-derived BeWo cells increases cellular retention of beta2-microglobulin. Placenta 2005; 26:171-82; PMID:15708118; http://dx.doi.org/10.1016/j.placenta.2004.04.014
- Low SC, Nunes SL, Bitonti AJ, Dumont JA. Oral and pulmonary delivery of FSH-Fc fusion proteins via neonatal Fc receptor-mediated transcytosis. Hum Reprod 2005; 20:1805-13; PMID:15817590; http://dx.doi.org/10.1093/humrep/deh896
- Dumont JA, Bitonti AJ, Clark D, Evans S, Pickford M, Newman SP. Delivery of an erythropoietin-Fc fusion protein by inhalation in humans through an immunoglobulin transport pathway. J Aerosol Med 2005; 18:294-303; PMID:16181004; http://dx.doi.org/10.1089/jam.2005.18.294
- Davies B, Morris T. Physiological parameters in laboratory animals and humans. Pharm Res 1993; 10:1093-5; PMID:8378254; http://dx.doi.org/10.1023/A:1018943613122
- Brown RP, Delp MD, Lindstedt SL, Rhomberg LR, Beliles RP. Physiological parameter values for physiologically based pharmacokinetic models. Toxicol Ind Health 1997; 13:407-84; PMID:9249929; http://dx.doi.org/10.1177/074823379701300401
- Abuqayyas L, Balthasar JP. Investigation of the role of FcgammaR and FcRn in mAb distribution to the brain. Mol Pharm 2013; 10:1505-13; PMID:22838637; http://dx.doi.org/10.1021/mp300214k
- Garg A, Balthasar JP. Investigation of the influence of FcRn on the distribution of IgG to the brain. AAPS J 2009; 11:553-7; PMID:19636712; http://dx.doi.org/10.1208/s12248-009-9129-9
- Chen N, Wang W, Fauty S, Fang Y, Hamuro L, Hussain A, Prueksaritanont T. The effect of the neonatal Fc receptor on human IgG biodistribution in mice. MAbs 2014; 6:502-8; PMID:24492305; http://dx.doi.org/10.4161/mabs.27765
- Schlachetzki F, Zhu C, Pardridge WM. Expression of the neonatal Fc receptor (FcRn) at the blood-brain barrier. J Neurochem 2002; 81:203-6; PMID:12067234; http://dx.doi.org/10.1046/j.1471-4159.2002.00840.x
- Cooper PR, Ciambrone GJ, Kliwinski CM, Maze E, Johnson L, Li Q, Feng Y, Hornby PJ. Efflux of monoclonal antibodies from rat brain by neonatal Fc receptor, FcRn. Brain Res 2013; 1534:13-21; PMID:23978455; http://dx.doi.org/10.1016/j.brainres.2013.08.035
- Yip V, Palma E, Tesar DB, Mundo EE, Bumbaca D, Torres EK, Reyes NA, Shen BQ, Fielder PJ, Prabhu S, et al. Quantitative cumulative biodistribution of antibodies in mice: effect of modulating binding affinity to the neonatal Fc receptor. MAbs 2014; 6:689-96; PMID:24572100; http://dx.doi.org/10.4161/mabs.28254
- Shah U, Dickinson BL, Blumberg RS, Simister NE, Lencer WI, Walker WA. Distribution of the IgG Fc receptor, FcRn, in the human fetal intestine. Pediatr Res 2003; 53:295-301; PMID:12538789; http://dx.doi.org/10.1203/00006450-200302000-00015
- Akilesh S, Christianson GJ, Roopenian DC, Shaw AS. Neonatal FcR expression in bone marrow-derived cells functions to protect serum IgG from catabolism. J Immunol 2007; 179:4580-8; PMID:17878355; http://dx.doi.org/10.4049/jimmunol.179.7.4580
- Cauza K, Hinterhuber G, Dingelmaier-Hovorka R, Brugger K, Klosner G, Horvat R, Wolff K, Foedinger D. Expression of FcRn, the MHC class I-related receptor for IgG, in human keratinocytes. J Invest Dermatol 2005; 124:132-9; PMID:15654966; http://dx.doi.org/10.1111/j.0022-202X.2004.23542.x
- Xue YJ, Gao H, Ji QC, Lam Z, Fang X, Lin ZJ, Hoffman M, Schulz-Jander D, Weng N. Bioanalysis of drug in tissue: current status and challenges. Bioanalysis 2012; 4:2637-53; PMID:23173797; http://dx.doi.org/10.4155/bio.12.252
- Smith KM, Xu Y. Tissue sample preparation in bioanalytical assays. Bioanalysis 2012; 4:741-9; PMID:22452264; http://dx.doi.org/10.4155/bio.12.19
- Sleep D, Cameron J, Evans LR. Albumin as a versatile platform for drug half-life extension. Biochim Biophys Acta 2013; 1830:5526-34; PMID:23639804; http://dx.doi.org/10.1016/j.bbagen.2013.04.023
- Rath T, Baker K, Dumont JA, Peters RT, Jiang H, Qiao SW, Lencer WI, Pierce GF, Blumberg RS. Fc-fusion proteins and FcRn: structural insights for longer-lasting and more effective therapeutics. Crit Rev Biotechnol 2015; 35:235-54; PMID:24156398; http://dx.doi.org/10.3109/07388551.2013.834293
- Bitonti AJ, Dumont JA, Low SC, Peters RT, Kropp KE, Palombella VJ, Stattel JM, Lu Y, Tan CA, Song JJ, et al. Pulmonary delivery of an erythropoietin Fc fusion protein in non-human primates through an immunoglobulin transport pathway. Proc Natl Acad Sci U S A 2004; 101:9763-8; PMID:15210944; http://dx.doi.org/10.1073/pnas.0403235101
- Low SC, Mezo AR. Inhibitors of the FcRn:IgG protein-protein interaction. AAPS J 2009; 11:432-4; PMID:19499344; http://dx.doi.org/10.1208/s12248-009-9120-5
- Perez HL, Cardarelli PM, Deshpande S, Gangwar S, Schroeder GM, Vite GD, Borzilleri RM. Antibody-drug conjugates: current status and future directions. Drug Discov Today 2014; 19:869-81; PMID:24239727; http://dx.doi.org/10.1016/j.drudis.2013.11.004