ABSTRACT
Forced degradation studies have become integral to the development of recombinant monoclonal antibody therapeutics by serving a variety of objectives from early stage manufacturability evaluation to supporting comparability assessments both pre- and post- marketing approval. This review summarizes the regulatory guidance scattered throughout different documents to highlight the expectations from various agencies such as the Food and Drug Administration and European Medicines Agency. The various purposes for forced degradation studies, commonly used conditions and the major degradation pathways under each condition are also discussed.
Abbreviations
CDR | = | Complementary-Determining Region |
CE-SDS | = | Capillary Electrophoresis Sodium Dodecyl Sulfate |
CQA | = | Critical Quality Attributes |
EMA | = | European Medicines Agency |
FDA | = | Food and Drug Administration |
ICH | = | International Conference on Harmonisation of Technical Requirements for Pharmaceuticals for Human Use |
LC-MS | = | Liquid Chromatography Mass Spectrometry |
mAb | = | Monoclonal Antibody |
SDS-PAGE | = | Sodium Dodecyl Sulfate Polyacrylamide Gel Electrophoresis |
SEC | = | Size-Exclusion Chromatography |
Forced degradation is an integral part of recombinant monoclonal antibody (mAb) therapeutics research and development. From early stage candidate selection to post-approval, forced degradation studies are frequently performed to support manufacturability assessments, formulation development, establishment of stability-indicating methods and comparability. Degradation of recombinant mAbs can negatively affect product quality, safety and efficacy and thus needs to be detected if it occurs. Forced degradation studies provide an opportunity to gain an in-depth understanding of the biochemical and biophysical properties of the molecules, including the major degradation pathways that are not observed from stability studies performed at real time and accelerated conditions. Even though forced degradation studies are performed at relatively harsh conditions within a short time period, the information gathered can provide highly relevant data to support real time environmental conditions.
Several review articles have previously addressed forced degradation of drugs.Citation1-5 This article differs in that it focuses specifically on recombinant mAb therapeutics. It discusses the objectives of forced degradation studies, commonly used conditions and the major degradation pathways under each condition. It further summarizes various types of forced degradation studies that are commonly used at different developmental stages for various objectives. Additionally, it extensively reviews the current guidance documents.
Purpose of forced degradation studies
Forced degradation studies have been commonly used by the industry to support the development of mAb therapeutics throughout the life-cycle of the products for various purposes (). It is also the expectation of agencies that forced degradation studies be used to understand the product degradation pathways, and establish stability indicating methods enabling monitoring degradation, if occurs, within the shelf life. In addition, forced degradation has been commonly used to evaluate manufacturability, method development, qualification and transfer, critical quality attributes (CQA) evaluation and identification of product variants. Forced degradation conditions, as discussed below, are highly relevant to mAb process and product development operations.
Table 1. Purposes and rationale of forced degradation studies.
Major degradation pathways under common forced degradation conditions
The commonly used forced degradation conditions include high temperature, freeze-thaw, agitation, high pH, low pH, light exposure, oxidation and glycation. Those conditions, though, relatively harsh compared with real-life storage and accelerated stability conditions, can generate relevant degradation trending and degradation products within a short-time period. The variety of conditions is also chosen based on the likelihood that the products are potentially exposed to those detrimental conditions during processing, packaging, shipping and handling.
The major degradation pathways are summarized in . The most commonly observed degradation pathways are aggregation, fragmentation, deamidation, and oxidation. Under specific conditions, limited degradation pathways could become dominant. For example, high pH conditions can trigger disulfide bond-related degradation pathways such as the formation of thioether or covalent aggregates due to disulfide bond scrambling. Incubation with reducing sugars results in glycation of mainly the surface-exposed lysine residues. The common as well as the unique degradation pathways under each condition are further discussed in the following sections.
Figure 1. Major degradation pathways of recombinant monoclonal antibodies. Arrows indicate the main degradation sites. The commonly used methods to detect the degradation products are shown in the parenthesis.
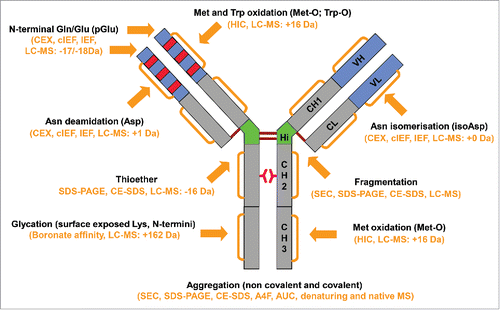
High temperature
Stability studies are routinely performed under normal storage, accelerated or intermediate temperature conditions. In comparison, forced degradation under thermal stress is generally performed at temperatures exceeding the nominal storage and accelerated stability conditions. For example, if the intended long-term storage condition for a mAb product is 2–8°C and the accelerated stability is 25°C, a thermal stress condition could be at 35°C or higher. The goal of using elevated temperature is to generate substantial levels of degradation within a short time period, such as a week. An appropriately high temperature may be selected by identifying the unfolding temperature measured through thermal stability techniques such as differential scanning calorimetry, or ideally by carrying out pre-screening studies. High temperature stress is one of the most relevant conditions for providing information about potential long-term degradation at the intended storage condition.
High temperature accelerates various degradation pathways. One of the major degradation pathways resulting from high temperature conditions is the formation of aggregates, including both insoluble (precipitates and particles) and soluble aggregates.Citation6-18 that are either covalent or non-covalent.Citation10,16 The mechanisms and types of aggregates vary with factors such as pHCitation12,17,19 and salt.Citation16 Another major high temperature-induced degradation pathway is fragmentation from peptide bond cleavage.Citation6,10,14-17,20-25 Fragmentation of antibody is mainly catalyzed by non-enzymatic reactions. It is rare for fragmentation to be catalyzed by contaminating proteases because drug substance is usually highly purified. CopperCitation26 and ironCitation27 can also catalyze fragmentation. The major fragmentation sites are around the hinge region,Citation6,14,20-25 and to a lesser degree around the domain-domain interfaces.Citation24 Fragmentation at other sites such as between two aspartate residuesCitation28 and two serine residuesCitation29 in the complementary-determining region 3 (CDR3) and between an aspartate and a proline residue in the CH2 domainCitation17 have also been reported. Interestingly, cleavage between aspartate and proline in the CH2 domain causes the formation of aggregates,Citation17 indicating dependency of chemical and physical modifications. Fragmentation is highly pH-dependent, with minimal levels of fragmentation around pH 6, whereas both low pHCitation23-25 and high pHCitation19,23-25 have been shown to accelerate fragmentation. Elevated temperature also accelerates asparagine (Asn) deamidation,Citation14,15,30-32 glutamine (Gln) deamidation,Citation14 N-terminal pyroglutamate formation (pGlu)Citation14 and methionine oxidation.Citation15,33 The sites of asparagine deamidation vary depending on the pH of the formulation buffer.Citation32 High temperature can accelerate aspartate isomerization, which leads to the formation of isoaspartate at slightly acidic pHCitation34-38 and the reaction intermediate, succinimide.Citation34-38 Analysis using charge-based methods led to the important observation that there is an increase in acidic species when recombinant mAbs are incubated at high temperature.Citation12,30,32 The increase in acidic species is likely caused by multiple degradation mechanisms, including modifications such as deamidation, N-terminal glutamine cyclization, and oxidation. High temperature at neutral and slightly basic conditions also accelerates disulfide bond degradation thorough β-elimination to form non-reducible cross-linked species due to the formation of thioether bonds.Citation12,30
Freeze-thaw
Freeze-thaw is often explored as a forced degradation condition to determine the susceptibility of a mAb to temperature cycling. Freezing and thawing are stresses to which mAb products are frequently exposed. For example, drug substance is often frozen to enable long-term storage. Drug product may be exposed to frozen temperature as part of lyophilization process development.
Chemical degradation is not the major degradation pathway for freeze-thaw stress because of the short time exposure of drug substance to room temperature. Instead, the major degradation pathway during freeze-thaw is the formation of various aggregates, including precipitates and particles, but consisting mainly of dimers and multimers.Citation11,16,18,19,39 Freeze-thaw-induced aggregation is mainly non-covalent in natureCitation19 and is highly dependent on pH,Citation19,39 buffer concentrationCitation19 excipientsCitation19 salt,Citation16 protein concentrationCitation39 and operating parameters such as cooling and warming rates.Citation39
Agitation
Another physical stress that mAbs encounter during production is agitation stress. Understanding the sensitivity of a mAb to agitation is important because it may be exposed to this condition as part of unit operations, including cell culture, purification, formulation, product filling, and shipping. The susceptibility of a mAb to agitation, such as stirring or shaking, is commonly evaluated to support formulation and process development. Agitation increases the likelihood of the mAb coming into contact with hydrophobic interfaces such as air-liquid or liquid-surface interfaces.
The major degradation pathway caused by shaking the antibody is the formation of insolubleCitation9,13,40-44 and soluble aggregates,Citation13,19,41,42 both of which can be covalent or non-covalent. The formation of non-native disulfide bonds is the major cause of reducible covalent aggregation.Citation40,45 Shake-induced aggregation is also highly dependent on the sample pH,Citation19 salts,Citation9 headspace,Citation42,46,47 excipients such as polysorbate,Citation40 shake rates, and container types and sizes.Citation47
Similar to shaking, the major degradation product caused by stirring is also aggregation (soluble and insoluble).Citation13,15,16,42,43,48 Factors such as salt and pH have a significant impact on the amounts and types of aggregates formed.Citation42 The insoluble aggregates caused by stirring are formed mainly due to non-native disulfide bonds.Citation42 Chemical modifications including oxidation of methionine and tryptophan, probably due to increased oxygen transfer from the headspace into the liquid, have also been reported.Citation15
Subtle differences in the degradation products and kinetics between stirring and shaking have been commonly observed. For example, one study demonstrated that both shaking and stirring generated precipitates, shaking generated soluble aggregates and particles, while stirring generated more particles, but fewer soluble aggregates.Citation42 Another study demonstrated that shaking resulted in both insoluble and soluble aggregates, but stirring resulted mainly in insoluble aggregates.Citation42 When designing agitation studies, it is important to consider both stirring and shaking, depending on the process relevance.
Low pH
Low pH is an important stress condition to evaluate because mAb purification processes typically involve exposure to acidic solution conditions, such as during protein A chromatography elution and the commonly used low pH virus inactivation. Forced degradation studies using low pH can thus use pH values from the purification process as reference points.
Low pH causes soluble,Citation12,19,49 and insoluble aggregation,Citation12,48,49 and accelerates fragmentation.Citation23,50 The major sites of fragmentation are in the hinge region, which results in the formation of fragments with molecular weights of ∼40–50 kDa under non-reduced conditions and ∼20–30 kDa under reduced conditions.Citation20,25 As discussed earlier, a study demonstrated that low pH induced cleavage between aspartate and proline occurred in the CH2 domain, which resulted in the formation of aggregates.Citation17 Low pH also resulted in the accumulation of succinimide from aspartate isomerization.Citation34,38,51
In practice, exposure of a mAb to low pH can result in precipitation, especially at the high protein concentrations found in drug substance. Although it is important to know if the product is susceptible to complete precipitation under specific low pH values, inclusion of a slightly higher pH condition that does not cause complete precipitation is recommended to effectively evaluate the effect of pH on product stability and to help identify the degradation pathways.
High pH and forced deamidation
Similar to low pH, it is important to evaluate high pH conditions for thorough process understanding and identification of degradation pathways. During purification, high pH elution buffer is used during operation of anion exchange chromatography. In addition, the mAb may be briefly exposed to high pH during the pH neutralization steps following protein A elution and low pH virus inactivation. High pH stress is typically performed using pH values such as 8 or 9. However, in some cases higher pH values, such as pH 10, may be needed to determine the deamidation susceptibility of asparagine residues located at different sites.
A signature indication of high pH stress is asparagine deamidation which is observed as an acidic shift of bands/peaks by charge-based methods.Citation12,14 The common deamidation sites of recombinant mAbs are located in the so-called “penny” peptide, SNGQPENNY, of the Fc.Citation52-55 Deamidation in the CDRs have also been reported.Citation56-58 The level of deamidation in the “penny” peptide provides a good reference point for comparing deamidation susceptibility of other asparagine residues.
High pH incubation often leads to aggregation, forming both insoluble and soluble aggregates,Citation12,13 and acceleration of fragmentation.Citation12,23,50 The major peptide bond hydrolysis sites are around the hinge and domain-domain interfaces.Citation14,19,22 High pH can also cause disulfide bond degradation, resulting in the formation of dehydroalanine, free sulfhydryl, thioether, and D-cysteine.Citation12,50
Photostability
Light exposure is a critical environmental factor that generally affects all stages of a product from cell culture to administration to patients. It is thus important to study the effect of light exposure on product quality. Unlike other forced degradation conditions, photostability is defined in the ICH guidance ICH Q1B. However, the conditions should be adjusted to achieve the appropriate levels of degradation for mAb. The amino acid residues tryptophan, tyrosine, phenylalanine and cysteine and peptide backbones are the most susceptible sites for photo-induced degradation.Citation59-61
The major degradation pathway due to light exposure is the formation of aggregates,Citation62 which are mainly covalent.Citation63 Light exposure resulting in fragmentation around the hinge region has also been commonly observed.Citation62,63 As expected, several residues including tryptophan,Citation62-65 methionine,Citation33,62,63,65,66 and histidineCitation63 are highly sensitive and become oxidized from light exposure. Oxidation of tryptophan residues results in the formation of a variety of products, with the major products having molecular weight increases of 16 Da and 32 Da.Citation65,67,68 Other modifications that have been reported include asparagine deamidationCitation63 and covalent cross-linking.Citation62 Oxidation and deamidation of residues in the CDR regions can correlate with the loss of activity.Citation63,65 Interestingly, photo-induced oxidation preferentially occurs on residues of the same heavy chain, whereas incubation with hydrogen peroxide results in random oxidation,Citation66,69 suggesting different mechanisms. One study reports that light exposure causes degradation of citric acid in the presence of Fe (II) and results in acetonation of recombinant antibody N-terminal and lysine side chain amine groups.Citation70
Oxidation
Recombinant mAbs are constantly exposed to oxidizing environments, including dissolved oxygen, oxygen in the air, and free radicals such as those generated by reactions with metals and impurities from raw materials (e.g., peroxides). Forced oxidation is used to probe residues that are susceptible to oxidation and to understand if there is a practical impact of this degradation pathway, e.g., oxidation of a residue in the CDR may affect binding and potency. The most commonly used approaches are incubation of recombinant mAbs with hydrogen peroxide or tert-butyl hydrogen peroxide for methionine residues,Citation71,72 and 2,2′-Azobis(2-amidinopropane) dihydrochloride for tryptophan.Citation72,73
The major degradation product of forced oxidation by incubating mAb with oxidizing reagents is oxidation of methionine residues to create methionine sulfoxide, with a molecular weight increase of 16 Da, and to a lesser degree, methionine sulfone, with a molecular weight increase of 32 Da.Citation15,31,74-77 Studies have demonstrated that one methionine residue in the CH2 domain (Met252, in the amino acid sequence of DTLMISR) and another (Met428, in the amino acid sequence of SVMHEA) in the CH3 domain are the most susceptible residues for oxidation under both photostability conditions and incubation with oxidizing reagents most likely because of their high levels of surface exposure.Citation66,69,78 While oxidation of methionine residues is the major degradation pathway, hydrogen peroxide can also result in mAb fragmentation.Citation79 Oxidation causes formation of insoluble and soluble aggregates,Citation13 which are probably due to oxidation-induced conformational changes.
It is worthwhile to mention that the commonly used forced oxidation conditions may not fully represent oxidation occurring under the real time conditions. Incubation with oxidizing regents causes the formation of methionine sulfoxides that are randomly distributed in the 2 heavy chains, while oxidation of the susceptible methionine residues on the same heavy chain is observed in accelerated stability samples.Citation69 These data suggest that different mechanisms are involved in the forced oxidation and accelerated conditions. Nevertheless, forced oxidation studies can reveal the susceptible residues and the degradation products, which are highly relevant in demonstrating the method capability to detect oxidation.
Glycation
Glycation is a non-enzymatic reaction between reducing sugars and the lysine side chain and N-terminal primary amine groups of the light and heavy chains of the antibody. The direct effect of glycation is modification of the protein's N-terminal amine and lysine side chains, with a molecular weight increase of 162 Da with each site of glycation.Citation8,80-83 The level of glycation is highly dependent on protein concentration, temperature, pH, sugar type, sugar concentration, exposure duration and free amino acid concentrations.Citation8,81,83 Glycation should be considered during forced degradation studies if there are exposed lysine residues (e.g., in the CDR), which could potentially affect binding and efficacy. Also, glycation can lead to aggregationCitation8 and can make the molecule more acidic.Citation82 The formation of advanced glycation end products can also result in coloration of the product and are associated with various disease-state proteins.Citation84
Recombinant mAbs are exposed to reducing sugars during many production steps, especially during cell culture, when sugars are used as nutrients.Citation82,85,86 Although non-reducing sugars are commonly used for formulation, it is possible for them to degrade into reducing sugars, and thus become reactive toward amine groups.Citation7,81,87 In addition, antibodies can become glycated during administration based on the diluent (e.g., dextrose solution) or after administration to patients due to reaction with sugars in the blood.Citation80
Pre-screening and application of forced degradation studies
Pre-screening
There are no procedures defined in regulatory guidance with regard to the specific conditions used in forced degradation, with the exception of photostability, which is defined in Q1B. However, for mAbs, the Q1B conditions are too severe, and therefore photostability forced degradation studies should be experimentally determined. The goal of the pre-screening is to identify optimal conditions that result in meaningful levels of degradation. Too little degradation cannot clearly demonstrate degradation pathways and kinetics, whereas too much degradation is not representative of real life scenarios.
Pre-screening is usually performed with fewer time points and using selected release and characterization assays, shown in . The selection of appropriate assays is important since each assay has its own benefits and limitations. Sensitive purity assays such as size-exclusion chromatography (SEC) and capillary electrophoresis-sodium dodecyl sulfate (CE-SDS) both measure monomer/main species, but SEC may be more sensitive to aggregates since it detects both covalent and noncovalent aggregates, while CE-SDS can only detect covalent aggregates. Both assays are reasonable choices to use during pre-screening. In contrast, a binding assay may not be sensitive in the characterization of non-significant changes in purity. Therefore, a binding assay may not be needed for pre-screening.
Table 2. Recommended pre-screening conditions and methods.
SEC is the method of choice to detect aggregation and to a lesser degree, fragmentation. Charge-based methods such as cation exchange chromatography or isoelectric focusing electrophoresis are very sensitive in the detection of overall changes to the molecule, mainly due to deamidation but from other modifications as well. Liquid chromatography-mass spectrometry (LC-MS) analysis of the intact or reduced molecular weight is also very useful for detection of modifications such as oxidation and glycation.
Samples evaluated during pre-screening should be as close as possible to the final formulated product. The rationale behind this requirement is multifold. First, degradation pathways and kinetics are highly dependent on sample properties such as concentration, pH, salt, and excipients. Second, data obtained from the forced degradation studies should be relevant to the final drug substance or drug product, and third, assays developed for a specific formulation matrix, such as ion exchange chromatography, may not be robust for different solution conditions. There could be exceptions if they are scientifically justifiable. In support of a comparability study, forced degradation studies that are performed using pre- and post-change lots with concentrations that differ from the drug substance are justifiable for relative comparison as long as the pre and post-change concentrations match each other.
Application of forced degradation studies
Forced degradation studies are designed based on the study objectives and the pre-screening outcomes. For example, agitation has been commonly used to determine the appropriate levels of polysorbate during pre-formulation to prevent aggregation. The study could be as simple as stressing samples with different levels of polysorbate and monitoring the generation of aggregates. On the other hand, to support comparability, multiple forced degradations could be used based on the potential impact of the process change on product quality and a prescreening study is most likely necessary to identify conditions resulting in a substantial level of degradation to allow the evaluation of degradation kinetics and degradation pathways. The areas of application are summarized in .
Table 3. Areas of applications, objectives and analytical tools of forced degradation studies throughout the lifecycle of mAb.
Manufacturability
Forced degradation studies are often used to evaluate the manufacturability of development candidates. The goal of manufacturability (also called “developability” or “molecule assessment”) of recombinant mAbs is to evaluate the candidate's biochemical and biophysical properties such as solubility, hydrophobicity and stability under the commonly used process, formulation and storage conditions. Such an evaluation is extremely useful for selection of one candidate to move forward in development. In the worst cases, this may trigger a re-design of the molecule to eliminate degradation “hot-spots.”
The forced degradation study explores the vulnerabilities of the candidates, which typically have already gone through an initial in-silico design analysis to correct obvious structural features that could cause problems such as free cysteines and degradation hotspots, as well as susceptible deamidation and oxidation sites in the CDR regions.Citation88-90 Additionally, some features can be eliminated from the molecule design, such as N-terminal glutamine and C-terminal lysine,Citation88,89 which have no known biologic functions but can cause heterogeneity.Citation91
The design of a manufacturability forced degradation study may be constrained due to limited material availability at this stage of development and the time allowed for such an evaluation. It is common to prioritize stress conditions and analytical methods based on prior knowledge of the molecule (e.g., in-silico analysis).Citation89,90 A wealth of information can be obtained from limited forced degradation studies such as heat treatment and oxidation.Citation90 It is suggested to use methods that are sensitive to degradation products, such as SEC for aggregates, charge-based methods for evaluation of the combined effects of several degradations including the commonly observed deamidation of mAb, and LC-MS for specific modifications such as oxidation. Low volume and high throughput analytical techniques are often used to screen multiple candidates. Examples include the use of CE-SDS to measure purity and capillary isoelectric focusing to measure changes in charge profile.
Pre-formulation development
Pre-formulation studies typically expand the knowledge of physico-chemical characteristic through measurement of solubility and thermodynamic stability in various buffers, pHs, salts, detergents and other excipients.Citation92,93 Forced degradation studies in multiple solution conditions are important to further evaluate the propensity of mAbs toward aggregation and overall chemical stability.Citation92,93 These data support the selection of appropriate buffers and pH ranges for further formulation development activities.
Degradation pathways
Establishment of the degradation pathways and profiles of a single representative lot is required for the Biologics License Application (BLA) or Marketing Authorisation Application (MAA). Both release and extended characterization methods are included to thoroughly characterize the degradation products generated under various forced degradation conditions. Characterization of degradation products from temperature stress may be helpful in identifying and understanding degradation products that are shown to occur in real time and under accelerated conditions.
In-depth characterization is straightforward for those conditions that result in specific reactions such as oxidation, glycation and deamidation. The sites and levels of those types of modifications can be readily determined using LC-MS. It is usually more challenging to characterize degradation products from more complex pathways such as those caused by high temperature, low pH, high pH and agitation. Multiple methods are usually used to thoroughly characterize those degradation products. Freeze/thaw is a unique condition, the major expected impact of which is the formation of aggregates, where methods to monitor particles and soluble aggregates can be useful, such as SEC, sodium dodecyl sulfate-polyacrylamide gel electrophoresis, particle counting, and mean fluorescence intensity.
Method development, qualification, validation and transfer
Forced degradation studies are an integral part of method development, qualification, validation and transfer. Release methods are designed to monitor and ensure the routine product quality and thus should have the capability to detect product degradation occurring in various real life conditions. Forced degradation materials generated under highly relevant conditions should be used in analytical method validation to establish the stability-indicating capabilities of the method so that degradation of products over time can be sufficiently monitored. Degradation products need to be relevant, meaning that they are predicted to occur during real time and accelerated or excursion conditions. In this sense, forced degradation conditions should be optimized to generate degradation products that are different only in the abundance, but not in species, as those formed during true processing and storage conditions.
The inclusion of forced degradation samples in method development can determine stability-indicating capabilities at an early stage. At that point, if methods do not indicate stability, there are still opportunities to improve them enough to do so. Using forced degradation samples can also help determine the critical method parameters. For a typical IgG1, the largest fragment is antibody without one Fab (about 100 kDa), which by SEC, elutes immediately after the monomer peak.Citation20 Therefore, detection of this peak is a minimum resolution requirement for the SEC method, if the capability to detect fragments is to be claimed.
Forced degradation samples usually contain relatively higher levels of product variants, including product-related substances and product-related impurities. Including these samples in method qualifications and transfers can thus build in additional robustness across a larger range of parameters.
Product-variant and degradation product identification
Forced degradation is often used to enrich the content of product variants in drug substance and degradation products in stability samples for in-depth characterization. Product variants are required to be thoroughly characterized to determine their chemical nature. Heterogeneity of recombinant mAbs is common due to various modifications including those caused by degradation resulting in product variants.Citation91,94 Likewise, degradation products that are present in stability samples are also required to be thoroughly characterized to understand the degradation pathways. However, it is challenging to collect low abundance variants or degradation products in the absence of forced degradation conditions.
It is a regulatory expectation that all peaks from chromatography release tests are identified. Forced degradation is used to enrich low abundant peaks observed in the drug substance/product. For chromatography methods, it is generally accepted that the peak retention time is correlated with specific modifications of the mAb. The same retention time indicates that variants detected in the drug substance are likely the same as those generated from stressing the samples.Citation95 However, there are also cases where the degradation products generated during the process are different from those generated from forced degradation studies. A study demonstrated that oxidation of a drug substance resulted in oxidation of methionine residues preferentially on the same heavy chain while incubation with hydrogen peroxide resulted in oxidation of methionine residues randomly on the two heavy chains.Citation69 Interestingly, photo-induced oxidation also resulted in oxidation of methionine residues preferentially on one heavy chain.Citation66 In another example, the sites of deamidation vary with the buffer pH,Citation32 therefore, using samples generated from incubation at high pH may not precisely predict deamidation under normal storage conditions if the formulation buffer pH is in the range of slightly acidic to neutral. Thus, the use of forced degradation samples to aid peak isolation and characterization of drug substance and stability samples should be scientifically justified.
Critical quality attribute evaluation support
ICH Q8(R2) defines a CQA as “a physical, chemical, biologic or microbiological property or characteristic that should be within an appropriate limit, range, or distribution to ensure the desired product quality.” Evaluation of whether or not a quality attribute is a CQA is based on its effect on potency, safety and immunogenicity while taking into consideration the degree of uncertainty.Citation96 In relation to the CQA concept, product variants can be classified as either product-related substance, with no impact to safety and efficacy, or as product-related impurities, with impact to product safety and efficacy. For some of the product variants found at low levels in drug substance, forced degradation conditions can be used to generate higher amounts of these variants so they can be used for the CQA evaluation.Citation97
Comparability support
Forced degradation studies can potentially provide strong evidence to support product comparability. Evaluation of pre-change lots and post-change lots under stress can reveal subtle changes that the routine release and characterization methods cannot detect. Comparability is established based on two criteria when using forced degradation data. The first criterion is the demonstration of the same degradation pathways. Generally, it can be assumed that degradation products with the same retention or migration times have the same degradation pathways. A unique species appearing in either the pre-change or post-change lots is required to be thoroughly characterized because it is indicative of a unique degradation pathway due to a subtle difference in the products. The second criterion is the demonstration of comparable degradation kinetics, as comparable lots will have the same trending. For degradation monitored by SEC, this is shown by comparable loss of monomer and simultaneous generation of aggregates and fragments over time.
The stringency of comparability is highly dependent on the phase of development recommended in Q5E. Accordingly, forced degradation studies including conditions, methods and number of lots can be selected based on risk assessment and scientific justification. Potential quality attribute changes can be reasonably predicted from internal experience and literature. Therefore, forced degradation conditions for comparability can be selected to include those with the highest potential to reveal differences between lots based on the pre-screening studies.
Regulatory guidance
Since forced degradation studies can serve a variety of purposes, aspects of forced degradation are found in various regulatory guidance documents. However, a single stand-alone document is lacking. Relevant guidance and the key points and application areas from the guidance are summarized in and further discussed in the following sections. Guidance documents related to biologicals are included, including documents from Food and Drug Administration (FDA) and European Medicines Agency (EMA). Guidance documents from International Conference on Harmonisation of Technical Requirements for Pharmaceuticals for Human Use (ICH) are also of particular importance because those documents reflect the harmonization between regulatory agencies and industry via sound scientific process.
Table 4. Relevant guidance documents on forced degradation.
ICH Q5C
International Conference on Harmonisation's Q5C document on stability testing of biotechnological/biologic products states that “The products are particularly sensitive to environmental factors such as temperature changes, oxidation, light, ionic content, and shear. To ensure maintenance of biologic activity and to avoid degradation, stringent conditions for their storage are usually necessary.” This paragraph recognizes that biologic products such as mAbs can be affected by many environmental factors that can cause degradation resulting in loss of biologic activity. Therefore, these conditions need to be studied to design appropriate formulation and storage conditions.
This guidance also states that “the applicant should develop the proper supporting stability data for a biotechnological/biologic product and consider many external conditions which can affect the product potency, purity and quality.” A stability program to establish product-shelf life is based on real time storage conditions with added assurance from accelerated or intermediate temperatures, if needed. The major environmental factor to be considered is temperature; however, the guidance recognizes that there are many additional factors that can affect drug potency, purity and quality. These factors are commonly included in a typical forced degradation study, such as exposure to extreme pH, light or agitation.
Q5C further states that “At the time of submission, applicants should have validated the methods that comprise the stability-indicating profile.” The applicants are required to understand the degradation pathways using relevant degraded samples to establish stability-indicating methods.
Lastly, Q5C states “Studies under stress conditions may be useful in determining whether accidental exposures to conditions other than those proposed (e.g., during transportation) are deleterious to the product and also for evaluating which specific test parameters may be the best indicators of product stability. Studies of the exposure of the drug substance or drug product to extreme conditions may help to reveal patterns of degradation; if so, such changes should be monitored under proposed storage conditions.” This paragraph suggests that exposure of product to extreme conditions can help identify the degradation pathways and establish methods that are capable of detecting these degradation products.
ICH Q1B
The Q1B document provides details for carrying out photostability studies. The entire study for drug substance photostability contains 2 parts, forced degradation and confirmatory studies. The goal of the forced degradation part is to determine the degradation pathways and support method development. For this part, different conditions can be used to generate appropriate amounts of degradation products. The goal of the confirmatory study is to provide information for appropriate processing and handling of drug product. The confirmatory study is critical in determining the appropriate procedures for handling, packaging and labeling of drug product. The guidance states that samples should be exposed to an overall total of not less than 1.2 million lux hours and an integrated near ultraviolet energy of not less than 200 Watt hours/square meter. The guideline suggests that the drug product photostability study be performed in a sequential manner, starting with fully exposed drug product, followed by drug product in the immediate pack and then in the marketing pack.
ICH Q6B
ICH document Q6B states “Degradation of drug substance and drug product, which may occur during storage, should be considered when establishing specifications. Due to the inherent complexity of these products, there is no single stability-indicating assay or parameter that profiles the stability characteristics. Consequently, the manufacturer should propose a stability-indicating profile. The result of this stability-indicating profile will then provide assurance that changes in the quality of the product will be detected.” This paragraph highlights the importance of establishing a stability-indicating profile for a given product, e.g., degradation pathways under various conditions and the appropriate methods. Forced degradation studies are therefore highly relevant because the conditions used are representative of the worst-case scenario of real life conditions. Degradation pathways identified are therefore representative of storage conditions and allow establishment of stability-indicating methods to detect those degradation products that may occur during storage.
ICH M4Q (R1)
Under the stability section 3.2.S.7, the guidance states “The types of studies conducted, protocols used, and the results of the studies should be summarized. The summary should include results, for example, from forced degradation studies and stress conditions, as well as conclusions with respect to storage conditions and retest date or shelf-life, as appropriate.” It further requires the data to be presented in an appropriate format. Understanding the degradation pathways under the forced degradation conditions is critical to ensuring product stability under storage conditions.
ICH Q1A (R2)
Q1A (R2) states that “Stress testing of the drug substance can help identify the likely degradation products, which can in turn help establish the degradation pathways and the intrinsic stability of the molecule and validate the stability indicating power of the analytical procedures used.” The degradation products should be used to validate the methods as stability-indicating, e.g., capability to detect relevant degradation products that occur under storage, transportation, and in-use conditions.
Q1A (R2) also states that “Stress testing is likely to be performed on a single batch of the drug substance. It should include the effect of temperatures (in 10°C increments (e.g., 50°C, 60°C, etc.) above that for accelerated testing), humidity (e.g., 75% RH or greater) where appropriate, oxidation, and photolysis on the drug substance. The testing should also evaluate the susceptibility of the drug substance to hydrolysis across a wide range of pH values when in solution or suspension.” The same document states that “Results from these studies will form an integral part of the information provided to regulatory authorities.” This document points out that understanding the degradation pathway is a requirement at the time of a license application submission. One representative batch can be used to thoroughly study the degradation pathways under forced degradation conditions including temperature, oxidation, photostability, low pH and high pH.
Q1A (R2) further states that “Examining degradation products under stress conditions is useful in establishing degradation pathways and developing and validating suitable analytical procedures. However, it may not be necessary to examine specifically for certain degradation products if it has been demonstrated that they are not formed under accelerated or long-term storage conditions.” Again, it points out that stress conditions are useful in establishing degradation pathways and thus develop and validate appropriate stability-indicating assays. It further specifies the requirement to identify degradation products that are actually present in long-term and accelerated stability conditions, while those degradation products which are unique to forced degradation conditions are not required to be identified.
Overall, at the time of BLA or MAA submission, forced degradation data from a single batch under various conditions should be included, demonstrating a good understanding of the degradation pathways determined from degradation product identification. These degradation products should have been used for stability-indicating method validations.
Food and drug administration guidance for industry investigational new drug applications for Phase 2 and 3 studies
This guidance states that “If not performed earlier, stress studies should be conducted during phase 3 to demonstrate the inherent stability of the drug substance, potential degradation pathways, and the capability and suitability of the proposed analytical procedures. The stress studies should assess the stability of the drug substance in different pH solutions, in the presence of oxygen and light, and at elevated temperature and humidity levels. These one-time stress studies on a single batch are not considered part of the formal stability program. The results should be summarized and submitted in an annual report.” The paragraph points out the requirement of using one representative batch to thoroughly study the degradation pathways under various stress conditions aside from the formal stability program to provide understanding of the intrinsic stability of the molecule. The samples from those studies should be used to demonstrate the capability and suitability of analytical methods to detect the degradation products.
This guidance further states that “For certain drug products, one-time stress testing can be warranted to assess the potential for changes in the physical (e.g., phase separation, precipitation, aggregation, changes in particular size distribution) and/or chemical (e.g., degradation and/or interaction of components) characteristics of the drug product. The studies could include testing to assess the effect of high temperature, humidity, oxidation, photolysis and/or thermal cycling. The relevant data should be provided in an annual report.” The degradation pathways of both drug substance and certain drug products should be included in the annual report during Phase 3 studies.
ICH Q5E
Q5E provides guidance on the use of forced degradation studies to support comparability of drug substance and drug product. For drug substance, the guidance highlights the importance of including stability in comparability studies through the following statements:
“The need for stability, including those generated from accelerated or stress conditions, to provide insight into potential product differences in the degradation pathways of the product and, hence, potential differences in product-related substances and product-related impurities.”
“Any change with the potential to alter protein structure or purity and impurity profiles should be evaluated for its impact on stability, since proteins are frequently sensitive to changes, such as those made to buffer composition, processing and holding conditions, and the use of organic solvents. Furthermore, stability studies might be able to detect subtle differences that are not readily detectable by the characterization studies.”
“Accelerated and stress stability studies are often useful tools to establish degradation profiles and provide a further direct comparison of pre-change and post-change product.”
For drug product, the guidance also adds that “even though all process changes occurred in the manufacture of the drug substance, in cases where the drug product could be impacted by the change, it might be appropriate to collect data on both the drug substance and the drug product to support the determination of comparability.”
The key point of using forced degradation studies to support comparability is the potential ability to reveal differences that may not be detected by release and extended characterization methods. The differences that are challenging to detect could be process-related impurities, product-related substance or product-related impurities, or subtle conformational difference of the product itself. For example, host cell proteins are typically detected by ELISA, which reports a numerical value. It is well known that not all host cell proteins are recognized by the method. Therefore, it is possible that two batches could have the same host cell protein amounts, but different species. If one of the missed host cell proteins is a protease, it can interfere with long-term stability. For drug-product related substances or drug product-related impurities, the specific modifications causing their formation may alter their long-term stability. For example, differences in the levels of glycation may cause differences in the amount of aggregates. It is challenging to detect subtle differences in conformation by release methods, as well as extended characterization methods, because of the complexity of recombinant mAbs and the limitations of the analytical methods used. However, subtle conformational differences may be exaggerated enough by the relatively harsh forced degradation conditions to be observed by differences in degradation products or kinetics.
ICH Q2(R1)
This document states “If impurity or degradation product standards are unavailable, specificity may be demonstrated by comparing the test results of samples containing impurities or degradation products to a second well-characterized procedure e.g., pharmacopoeial method or other validated analytical procedure (independent procedure). As appropriate, this should include samples stored under relevant stress conditions: light, heat, humidity, acid/base hydrolysis and oxidation.” This quote discusses the potential to use degraded samples to demonstrate method specificity, e.g., the methods should discriminate impurities or degradation products from the intended products.
FDA guidance: Analytical procedures and methods validation for drugs and biologics
This document states “If a procedure is a validated quantitative analytical procedure that can detect changes in a quality attribute(s) of the drug substance and drug product during storage, it is considered a stability-indicating test. To demonstrate specificity of a stability-indicating test, a combination of challenges should be performed. Some challenges include the use of samples spiked with target analytes and all known interferences; samples that have undergone various laboratory stress conditions; and actual product samples (produced by the final manufacturing process) that are either aged or have been stored under accelerated temperature and humidity conditions.” This paragraph discusses that stress conditions should be used to generate target degradation products to test stability-indicating method specificity. For example, higher levels of aggregates can be generated from stress conditions and then used to determine the specificity of a SEC method.
EMA guideline on the requirements for quality documentation concerning biologic investigational medicinal products in clinical trials
EMA's draft document states “Accelerated and stress condition studies are recommended as they may help understanding the degradation profile of the product and support an extension of the shelf-life.” In line with other regulatory guidance, it suggests use of accelerated and stress conditions to obtain knowledge of the degradation pathways. The same guideline also requires the inclusion of discussion of “stability-indicating properties of the analytical methods” in the stability programs. Such a discussion should be based on the understanding of the degradation pathways and evaluating analytical methods using representative degraded samples.
Conclusion
Forced degradation is a useful tool widely used during the development of recombinant mAb therapeutics, as well as related products such as antibody-drug conjugates. During the very early stages of development, a simple forced degradation study can provide useful information about the manufacturability of candidates and aid in the selection of those most appropriate to move forward. Forced degradation is an integral part of early formulation development in identifying appropriate conditions for long-term storage of the drug substance and drug product. For method development, inclusion of forced degradation samples as early as possible is suggested to facilitate understanding of method capabilities and limits. Generation of degradation products can help impurities isolation and characterization tremendously because these impurities are normally present at such low levels in drug substance. Isolation and characterization of different degradation products can provide the opportunity to evaluate the effect of degradation or modifications on product efficacy and thus define CQAs. When applied to comparability studies, forced degradation has the potential to reveal differences that are not detectable by routine analysis, thus providing further assurance of comparability. Data from forced degradation studies can be used to justify excursions that may occur during processing, shipping and handling. Overall, forced degradation provides in-depth understanding of the biochemical and biophysical nature of the molecules to ensure that products are well characterized in a timescale that enables rapid clinical and commercial development. Last but not least, as recently reported by Pisupati et al,Citation98 forced degradation are also key studies for antibody biosimilarity assessment.
Acknowledgment
The authors would like to thank Boxu Yan from Celgene for providing critical review and valuable comments to this manuscript.
References
- Blessy M, Patel R, Prajapati P, Agrawal Y. Develpment of forced degradation and stability indicating studies of drugs- a review. J Pharm Anal. 2014;4(3):159-65. doi:10.1016/j.jpha.2013.09.003
- Hawe A, Wiggenhorn M, van de Weert M, Garbe JH, Mahler HC, Jiskoot W. Forced degradation of therapeutic proteins. J Pharm Sci. 2012;101(3):895-913. doi:10.1002/jps.22812. PMID:22083792
- Kilck S, Muijselaar P, Waterval J, Eichinger T, Korn C, Gerding T, Debets A, Sanger-van de Griend C, Van den Beld C, Somsen G., et al. Toward a generic approach for stress testing of drug substances and drug products. Pharm Technol. 2005, February, 48-66
- Manning MC, Chou DK, Murphy BM, Payne RW, Katayama DS. Stability of protein pharmaceuticals: an update. Pharm Res. 2010;27(4):544-75. doi:10.1007/s11095-009-0045-6. PMID:20143256
- Tamizi E, Jouyban A. Forced degradation studies of biopharmaceuticals: Selection of stress conditions. Eur J Pharm Biopharm. 2016;98:26-46. doi:10.1016/j.ejpb.2015.10.016. PMID:26542454
- Alexander AJ, Hughes DE Monitoring of IgG antibody thermal stability by micellar electrokinetic capillary chromatography and matrix-assisted laser desorption/ionization mass spectrometry. Anal Chem. 1995;67(20):3626-32. doi:10.1021/ac00116a002. PMID:8644915
- Andya JD, Maa YF, Costantino HR, Nguyen PA, Dasovich N, Sweeney TD, Hsu CC, Shire SJ. The effect of formulation excipients on protein stability and aerosol performance of spray-dried powders of a recombinant humanized anti-IgE monoclonal antibody. Pharm Res. 1999;16(3):350-58. doi:10.1023/A:1018805232453. PMID:10213364
- Banks DD, Hambly DM, Scavezze JL, Siska CC, Stackhouse NL, Gadgil HS. The effect of sucrose hydrolysis on the stability of protein therapeutics during accelerated formulation studies. J Pharm Sci. 2009;98(12):4501-10. doi:10.1002/jps.21749. PMID:19388069
- Fesinmeyer RM, Hogan S, Saluja A, Brych SR, Kras E, Narhi LO, Brems DN, Gokarn YR. Effect of ions on agitation- and temperature-induced aggregation reactions of antibodies. Pharm Res. 2009;26(4):903-13. doi:10.1007/s11095-008-9792-z. PMID:19104916
- Franey H, Brych SR, Kolvenbach CG, Rajan RS. Increased aggregation propensity of IgG2 subclass over IgG1: Role of conformational changes and covalent character in isolated aggregates. Protein Sci. 2010;19(9):1601-15. doi:10.1002/pro.434. PMID:20556807
- Hawe A, Kasper JC, Friess W, Jiskoot W. Structural properties of monoclonal antibody aggregates induced by freeze-thawing and thermal stress. Eur J Pharm Sci. 2009;38(2):79-87. doi:10.1016/j.ejps.2009.06.001. PMID:19540340
- Jiskoot W, Beuvery EC, De Koning AA, Herron JN, Crommelin DJ. Analytical approaches to the study of monoclonal antibody stability. Pharm Res. 1990;7(12):1234-41.doi:10.1023/A:1015925519154. PMID:2095560
- Joubert MK, Luo Q, Nashed-Samuel Y, Wypych J, Narhi LO. Classification and characterization of therapeutic antibody aggregates. J Biol Chem. 2011;286(28):25118-33. doi:10.1074/jbc.M110.160457. PMID:21454532
- Liu H, Gaza-Bulseco G, Sun J. Characterization of the stability of a fully human monoclonal IgG after prolonged incubation at elevated temperature. J Chromatogr B Analyt Technol Biomed Life Sci. 2006;837(1–2):35-43. doi:10.1016/j.jchromb.2006.03.053. PMID:16644295
- Luo Q, Joubert MK, Stevenson R, Ketchem RR, Narhi LO, Wypych J Chemical modifications in therapeutic protein aggregates generated under different stress conditions. J Biol Chem. 2011;286(28):25134-44. doi:10.1074/jbc.M110.160440. PMID:21518762
- Telikepalli SN, Kumru OS, Kalonia C, Esfandiary R, Joshi SB, Middaugh CR, Volkin D B. Structural characterization of IgG1 mAb aggregates and particles generated under various stress conditions. J Pharm Sci. 2014;103(3):796-809. doi:10.1002/jps.23839. PMID:24452866
- Van BN, Rehder D, Gadgil H, Matsumura M, Jacob J. Elucidation of two major aggregation pathways in an IgG2 antibody. J Pharm Sci. 2009;98(9):3013-30. doi:10.1002/jps.21514. PMID:18680168
- Zhang A, Singh SK, Shirts MR, Kumar S, Fernandez EJ. Distinct aggregation mechanisms of monoclonal antibody under thermal and freeze-thaw stresses revealed by hydrogen exchange. Pharm Res. 2012;29(1):236-50. doi:10.1007/s11095-011-0538-y. PMID:21805212
- Paborji M, Pochopin NL, Coppola WP, Bogardus JB Chemical and physical stability of chimeric L6, a mouse-human monoclonal antibody. Pharm Res. 1994;11(5):764-71. doi:10.1023/A:1018948901599. PMID:8058650
- Cordoba AJ, Shyong BJ, Breen D, Harris RJ. Non-enzymatic hinge region fragmentation of antibodies in solution. J Chromatogr B Analyt Technol Biomed Life Sci. 2005;818(2):115-21. doi:10.1016/j.jchromb.2004.12.033. PMID:15734150
- Dillon TM, Bondarenko PV, Speed RM Development of an analytical reversed-phase high-performance liquid chromatography-electrospray ionization mass spectrometry method for characterization of recombinant antibodies. J Chromatogr A 2004;1053(1–2):299-305. doi:10.1016/S0021-9673(04)01410-4. PMID:15543996
- Dillon TM, Bondarenko PV, Rehder DS, Pipes GD, Kleemann GR, Ricci MS. Optimization of a reversed-phase high-performance liquid chromatography/mass spectrometry method for characterizing recombinant antibody heterogeneity and stability. J Chromatogr A. 2006;1120(1–2):112-20. doi:10.1016/j.chroma.2006.01.016. PMID:16448656
- Gaza-Bulseco G., Liu H. Fragmentation of a recombinant monoclonal antibody at various pH. Pharm Res. 2008;25(8):1881-90. doi:10.1007/s11095-008-9606-3. PMID:18473123
- Liu H, Gaza-Bulseco G, Lundell E Assessment of antibody fragmentation by reversed-phase liquid chromatography and mass spectrometry. J Chromatogr B Analyt Technol Biomed Life Sci. 2008;876(1):13-23. doi:10.1016/j.jchromb.2008.10.015. PMID:18993120
- Xiang T, Lundell E, Sun Z, Liu H Structural effect of a recombinant monoclonal antibody on hinge region peptide bond hydrolysis. J Chromatogr B Analyt Technol Biomed Life Sci. 2007;858(1–2):254-62. doi:10.1016/j.jchromb.2007.08.043. PMID:17901002
- Smith MA, Easton M, Everett P, Lewis G, Payne M, Riveros-Moreno V, Allen G. Specific cleavage of immunoglobulin G by copper ions. Int J Pept Protein Res. 1996;48(1):48-55. doi:10.1111/j.1399-3011.1996.tb01105.x. PMID:8844262
- Ouellette D, Alessandri L, Piparia R, Aikhoje A, Chin A, Radziejewski C, Correia I. Elevated cleavage of human immunoglobulin gamma molecules containing a lambda light chain mediated by iron and histidine. Anal Biochem. 2009;389(2):107-17. doi:10.1016/j.ab.2009.03.027. PMID:19318085
- Xiao G, Bondarenko PV. Identification and quantification of degradations in the Asp-Asp motifs of a recombinant monoclonal antibody. J. Pharm Biomed Anal. 2008;47(1):23-30. doi:10.1016/j.jpba.2007.11.050. PMID:18201853
- Li W, Yang B, Zhou D, Xu J, Li W, Suen WC. Identification and characterization of monoclonal antibody fragments cleaved at the complementarity determining region using orthogonal analytical methods. J Chromatogr B Analyt Technol Biomed Life Sci. 2017;1048:121-29. doi:10.1016/j.jchromb.2017.02.019. PMID:28242491
- Kroon DJ, Baldwin-Ferro A, Lalan P. Identification of sites of degradation in a therapeutic monoclonal antibody by peptide mapping. Pharm. Res. 1992;9(11):1386-93. doi:10.1023/A:1015894409623. PMID:1475223
- Yan B, Steen S, Hambly D, Valliere-Douglass J, Vanden Bos T, Smallwood S, Yates Z, Arroll T, Han Y, Gadgil H., et al. Succinimide formation at Asn 55 in the complementarity determining region of a recombinant monoclonal antibody IgG1 heavy chain. J Pharm Sci. 2009;98(10):3509-21. doi:10.1002/jps.21655. PMID:19475547
- Zhang YT, Hu J, Pace AL, Wong R, Wang YJ, Kao YH. Characterization of asparagine 330 deamidation in an Fc-fragment of IgG1 using cation exchange chromatography and peptide mapping. J Chromatogr B Analyt Technol Biomed Life Sci. 2014;965:65-71. doi:10.1016/j.jchromb.2014.06.018. PMID:24999246
- Lam XM, Yang JY, Cleland JL. Antioxidants for prevention of methionine oxidation in recombinant monoclonal antibody HER2. J Pharm Sci. 1997;86(11):1250-55. doi:10.1021/js970143s. PMID:9383735
- Chu GC, Chelius D, Xiao G, Khor HK, Coulibaly S, Bondarenko PV. Accumulation of succinimide in a recombinant monoclonal antibody in mildly acidic buffers under elevated temperatures. Pharm Res. 2007;24(6):1145-56. doi:10.1007/s11095-007-9241-4. PMID:17385019
- Huang HZ, Nichols A, Liu D Direct identification and quantification of aspartyl succinimide in an IgG2 mAb by RapiGest assisted digestion. Anal Chem. 2009;81(4):1686-92. doi:10.1021/ac802708s. PMID:19146457
- Yu XC, Joe K, Zhang Y, Adriano A, Wang Y, Gazzano-Santoro H, Keck RG, Deperalta G, Ling V. Accurate determination of succinimide degradation products using high fidelity trypsin digestion peptide map analysis. Anal Chem. 2011;83(15):5912-19. doi:10.1021/ac200750u. PMID:21692515
- Valliere-Douglass J, Jones L, Shpektor D, Kodama P, Wallace A, Balland A, Bailey R, Zhang Y. Separation and characterization of an IgG2 antibody containing a cyclic imide in CDR1 of light chain by hydrophobic interaction chromatography and mass spectrometry. Anal Chem. 2008;80(9):3168-74. doi:10.1021/ac702245c. PMID:18355059
- Cacia J, Keck R, Presta LG, Frenz J. Isomerization of an aspartic acid residue in the complementarity-determining regions of a recombinant antibody to human IgE: identification and effect on binding affinity. Biochemistry. 1996;35(6):1897-903. doi:10.1021/bi951526c. PMID:8639672
- Kueltzo LA, Wang W, Randolph TW, Carpenter JF. Effects of solution conditions, processing parameters, and container materials on aggregation of a monoclonal antibody during freeze-thawing. J Pharm Sci. 2008;97(5):1801-12. doi:10.1002/jps.21110. PMID:17823949
- Brych SR, Gokarn YR, Hultgen H, Stevenson RJ, Rajan R, Matsumura M. Characterization of antibody aggregation: role of buried, unpaired cysteines in particle formation. J Pharm Sci. 2010;99(2):764-81. doi:10.1002/jps.21868. PMID:19691118
- Ghazvini S, Kalonia C, Volkin DB, Dhar P. Evaluating the role of the air-solution interface on the mechanism of subvisible particle formation caused by mechanical agitation for an IgG1 mAb. J Pharm Sci. 2016;105(5):1643-56. doi:10.1016/j.xphs.2016.02.027. PMID:27025981
- Kiese S, Papppenberger A, Friess W, Mahler HC. Shaken, not stirred: mechanical stress testing of an IgG1 antibody. J Pharm Sci. 2008;97(10):4347-66. doi:10.1002/jps.21328. PMID:18240293
- Mahler HC, Muller R, Friess W, Delille A, Matheus S. Induction and analysis of aggregates in a liquid IgG1-antibody formulation. Eur J Pharm Biopharm. 2005;59(3):407-17. doi:10.1016/j.ejpb.2004.12.004. PMID:15760721
- Serno T, Carpenter JF, Randolph TW, Winter G. Inhibition of agitation-induced aggregation of an IgG-antibody by hydroxypropyl-beta-cyclodextrin. J Pharm Sci. 2010;99(3):1193-1206. doi:10.1002/jps.21931. PMID:19774651
- Huh JH, White AJ, Brych SR, Franey H, Matsumura M. The identification of free cysteine residues within antibodies and a potential role for free cysteine residues in covalent aggregation because of agitation stress. J Pharm Sci. 2013;102(6):1701-11. doi:10.1002/jps.23505. PMID:23559428
- Brady LJ, Martinez T, Balland A. Characterization of nonenzymatic glycation on a monoclonal antibody. Anal Chem. 2007;79(24):9403-13. doi:10.1021/ac7017469. PMID:17985928
- Eppler A., Weigandt M., Hanefeld A., Bunjes H. Relevant shaking stress conditions for antibody preformulation development. Eur J Pharm Biopharm. 2010;74(2):139-47. doi:10.1016/j.ejpb.2009.11.005. PMID:19922795
- Sharma DK, Oma P, Pollo MJ, Sukumar, M. Quantification and characterization of subvisible proteinaceous particles in opalescent mAb formulations using micro-flow imaging. J Pharm Sci. 2010;99(6):2628-42. doi:10.1002/jps.22046. PMID:20049937
- Arosio P, Rima S, Morbidelli M. Aggregation mechanism of an IgG2 and two IgG1 monoclonal antibodies at low pH: from oligomers to larger aggregates. Pharm Res. 2013;30(3):641-54. doi:10.1007/s11095-012-0885-3. PMID:23054090
- Cohen SL, Price C, Vlasak J. Beta-elimination and peptide bond hydrolysis: two distinct mechanisms of human IgG1 hinge fragmentation upon storage. J Am Chem Soc. 2007;129(22):6976-77. doi:10.1021/ja0705994. PMID:17500521
- Nowak C, Ponniah G, Neill A, Liu H. Characterization of succinimide stability during trypsin digestion for LC-MS analysis. Anal Biochem. 2017;526:1-8. doi:10.1016/j.ab.2017.03.005. PMID:28274724
- Liu YD, van Enk JZ, Flynn, GC. Human antibody Fc deamidation in vivo. Biologicals. 2009;37(5):313-22. doi:10.1016/j.biologicals.2009.06.001. PMID:19608432
- Wang L, Amphlett G, Lambert JM, Blattler W, Zhang W. Structural characterization of a recombinant monoclonal antibody by electrospray time-of-flight mass spectrometry. Pharm Res. 2005;22(8):1338-49. doi:10.1007/s11095-005-5267-7. PMID:16078144
- Chelius D, Rehder DS, Bondarenko PV. Identification and characterization of deamidation sites in the conserved regions of human immunoglobulin gamma antibodies. Anal Chem. 2005;77(18):6004-11. doi:10.1021/ac050672d. PMID:16159134
- Sinha S, Zhang L, Duan S, Williams TD, Vlasak J, Ionescu R, Top, EM. Effect of protein structure on deamidation rate in the Fc fragment of an IgG1 monoclonal antibody. Protein Sci. 2009;18(8):1573-84. doi:10.1002/pro.173. PMID:19544580
- Huang L, Lu J, Wroblewski VJ, Beals JM, Riggin RM. In vivo deamidation characterization of monoclonal antibody by LC/MS/MS. Anal Chem. 2005;77(5):1432-39. doi:10.1021/ac0494174. PMID:15732928
- Harris RJ, Kabakoff B, Macch FD, Shen FJ, Kwong M, Andya JD, Shire SJ, Bjork N, Totpal K, Chen AB. Identification of multiple sources of charge heterogeneity in a recombinant antibody. J Chromatogr B Biomed Sci Appl. 2001;752(2):233-45. doi:10.1016/S0378-4347(00)00548-X. PMID:11270864
- Vlasak J, Bussat MC, Wang S, Wagner-Rousset E, Schaefer M, Klinguer-Hamour C, Kirchmeier M, Corvaia N, Ionescu R, Beck A. Identification and characterization of asparagine deamidation in the light chain CDR1 of a humanized IgG1 antibody. Anal Biochem. 2009;392(2):145-54. doi:10.1016/j.ab.2009.05.043. PMID:19497295
- Creed D. The photophysics and photochemistry of the near-UV absorbing amino acids-I, Tryptophan and its simple derivatives. Photochem Photobiol. 1984;39(4):537-62. doi:10.1111/j.1751-1097.1984.tb03890.x
- Holt LA, Milligan B, Rivett DE, Stewart FH. The photodecomposition of tryptophan peptides. Biochim Biophys Acta. 1977;499(1):131-38. doi:10.1016/0304-4165(77)90235-5. PMID:889893
- Kerwin BA, Remmele RL, Jr. Protect from light: photodegradation and protein biologics. J Pharm Sci. 2007;96(6):1468-79. doi:10.1002/jps.20815. PMID:17230445
- Singh SR, Zhang J, O'Dell C, Hsieh MC, Goldstein J, Liu J, Srivastava A. Effect of polysorbate 80 quality on photostability of a monoclonal antibody. AAPS PharmSciTech. 2012;13(2):422-30. doi:10.1208/s12249-012-9759-6. PMID:22362139
- Qi P, Volkin DB, Zhao H, Nedved ML, Hughes R, Bass R, Yi SC, Panek ME, Wang D, Dalmonte P, et al. Characterization of the photodegradation of a human IgG1 monoclonal antibody formulated as a high-concentration liquid dosage form. J Pharm Sci. 2009;98(9):3117-30. doi:10.1002/jps.21617. PMID:19009595
- Nowak C, Ponniah G, Cheng G, Kita A, Neill A, Kori Y, Liu H. Liquid chromatography-fluorescence and liquid chromatography-mass spectrometry detection of tryptophan degradation products of a recombinant monoclonal antibody. Anal Biochem. 2016;496:4-8. doi:10.1016/j.ab.2015.12.004. PMID:26717898
- Wei Z, Feng J., Lin HY, Mullapudi S, Bishop E, Tous GI, Casas-Finet J, Hakki F, Strouse R, Schenerman MA. Identification of a single tryptophan residue as critical for binding activity in a humanized monoclonal antibody against respiratory syncytial virus. Anal Chem. 2007;79(7):2797-2805. doi:10.1021/ac062311j. PMID:17319649
- Liu H, Gaza-Bulseco G, Zhou L. Mass spectrometry analysis of photo-induced methionine oxidation of a recombinant human monoclonal antibody. J Am Soc Mass Spectrom. 2009;20(3):525-28. doi:10.1016/j.jasms.2008.11.011. PMID:19103498
- Li Y, Polozova A, Gruia F, Feng J. Characterization of the degradation products of a color-changed monoclonal antibody: tryptophan-derived chromophores. Anal Chem. 2014;86(14):6850-57. doi:10.1021/ac404218t. PMID:24937252
- Reubsaet JL, Beijnen JH, Bult A, van Maanen RJ, Marchal JA, Underberg WJ. Analytical techniques used to study the degradation of proteins and peptides: chemical instability. J Pharm Biomed Anal. 1998;17(6–7):955-78. doi:10.1016/S0731-7085(98)00063-6. PMID:9884187
- Chumsae C, Gaza-Bulseco G, Sun J, Liu H. Comparison of methionine oxidation in thermal stability and chemically stressed samples of a fully human monoclonal antibody. J Chromatogr B Analyt Technol Biomed Life Sci. 2007;850(1–2):285-94. doi:10.1016/j.jchromb.2006.11.050. PMID:17182291
- Valliere-Douglass JF, Connell-Crowley L, Jensen R, Schnier PD, Trilisky E, Leith M, Follstad B D, Kerr J, Lewis N, Vunnum S, et al. Photochemical degradation of citrate buffers leads to covalent acetonation of recombinant protein therapeutics. Protein Sci. 2010;19(11):2152-63. doi:10.1002/pro.495. PMID:20836085
- Keck RG The use of t-butyl hydroperoxide as a probe for methionine oxidation in proteins. Anal Biochem. 1996;236(1):56-62. doi:10.1006/abio.1996.0131. PMID:8619496
- Folzer E, Diepold K, Bomans K, Finkler C, Schmidt R, Bulau P, Huwyler J, Mahler HC, Koulov AV. Selective oxidation of methionine and tryptophan residues in a therapeutic igg1 molecule. J Pharm Sci. 2015;104(9):2824-31. doi:10.1002/jps.24509. PMID:26010344
- Ma Y, Chao C, Stadtman ER. Oxidative modification of glutamine synthetase by 2,2′-Azobis(2-amidinopropane) dihydrochloride. Arch Biochem Biophys. 1999;363(1):129-34. doi:10.1006/abbi.1998.1076. PMID:10049507
- Bertolotti-Ciarlet A, Wang W, Lownes R, Pristatsky P, Fang Y, McKelvey T, Li Y, Li Y, Drummond J, Prueksaritanont T, Vlasak J. Impact of methionine oxidation on the binding of human IgG1 to Fc Rn and Fc gamma receptors. Mol Immunol. 2009;46(8–9):1878-82. doi:10.1016/j.molimm.2009.02.002. PMID:19269032
- Pan H, Chen K, Chu L, Kinderman F, Apostol I, Huang G. Methionine oxidation in human IgG2 Fc decreases binding affinities to protein A and FcRn. Protein Sci. 2009;18(2):424-33. doi:10.1002/pro.45. PMID:19165723
- Wang W, Vlasak J, Li Y, Pristatsky P, Fang Y, Pittman T, Roman J, Wang Y, Prueksaritanont T, Ionescu R. Impact of methionine oxidation in human IgG1 Fc on serum half-life of monoclonal antibodies. Mol Immunol. 2011;48(6–7):860-66. doi:10.1016/j.molimm.2010.12.009. PMID:21256596
- Yang R, Jain T, Nobrega RP, Lu X, Boland T, Burnina I, Sun T, Caffry I, Brown M, Zhi X, et al. Rapid assessment of oxidation via middle-down LCMS correlates with methionine side-chain solvent-accessible surface area for 121 clinical stage monoclonal antibodies. MAbs. 2017;9(4):646-53. doi:10.1080/19420862.2017.1290753
- Liu D, Ren D, Huang H, Dankberg J, Rosenfeld R, Cocco MJ, Li L, Brems DN, Remmele RL, Jr. Structure and stability changes of human IgG1 Fc as a consequence of methionine oxidation. Biochemistry. 2008;47(18):5088-5100. doi:10.1021/bi702238b. PMID:18407665
- Yan B, Yates Z, Balland A, Kleemann GR. Human IgG1 hinge fragmentation as the result of H2O2-mediated radical cleavage. J Biol Chem. 2009;284(51):35390-402. doi:10.1074/jbc.M109.064147. PMID:19850927
- Goetze AM, Liu YD, Arroll T, Chu L, Flynn GC. Rates and impact of human antibody glycation in vivo. Glycobiology. 2012;22(2):221-34. doi:10.1093/glycob/cwr141. PMID:21930650
- Gadgil HS, Bondarenko PV, Pipes G, Rehder D, McAuley A, Perico N, Dillon T, Ricci M, Treuheit M. The LC/MS analysis of glycation of IgG molecules in sucrose containing formulations. J Pharm Sci. 2007;96(10):2607-21. doi:10.1002/jps.20966. PMID:17621682
- Quan C, Alcala E, Petkovska I, Matthews D, Canova-Davis E, Taticek R, Ma S. A study in glycation of a therapeutic recombinant humanized monoclonal antibody: where it is, how it got there, and how it affects charge-based behavior. Anal Biochem. 2008;373(2):179-91. doi:10.1016/j.ab.2007.09.027. PMID:18158144
- Zhang B, Yang Y, Yuk I, Pai R, McKay P, Eigenbrot C, Dennis M, Katta V, Francissen K C. Unveiling a glycation hot spot in a recombinant humanized monoclonal antibody. Anal Chem. 2008;80(7):2379-90. doi:10.1021/ac701810q. PMID:18307322
- Butko M, Pallat H, Cordoba A, Yu XC Recombinant antibody color resulting from advanced glycation end product modifications. Anal Chem. 2014;86(19):9816-23. doi:10.1021/ac5024099. PMID:25181536
- Miller AK, Hambly DM, Kerwin BA, Treuheit MJ, Gadgil HS. Characterization of site-specific glycation during process development of a human therapeutic monoclonal antibody. J Pharm Sci. 2011;100(7):2543-50. doi:10.1002/jps.22504. PMID:21287557
- Yuk IH, Zhang B, Yang Y, Dutina G, Leach KD, Vijayasankaran N, Shen AY, Andersen DC, Snedecor BR, Joly JC. Controlling glycation of recombinant antibody in fed-batch cell cultures. Biotechnol Bioeng. 2011;108(11):2600-10. doi:10.1002/bit.23218. PMID:21618472
- Fischer S, Hoernschemeyer J, Mahler H C. Glycation during storage and administration of monoclonal antibody formulations. Eur J Pharm. Biopharm. 2008;70(1):42-50. doi:10.1016/j.ejpb.2008.04.021. PMID:18583113
- Beck A, Wurch T, Bailly C, Corvaia N. Strategies and challenges for the next generation of therapeutic antibodies. Nat Rev Immunol. 2010;10(5):345-52. doi:10.1038/nri2747. PMID:20414207
- Yang X, Xu W, Dukleska S, Benchaar S, Mengisen S, Antochshuk V, Cheung J, Mann L, Babadjanova Z, Rowand J, et al. Developability studies before initiation of process development: improving manufacturability of monoclonal antibodies. MAbs. 2013;5(5):787-94. doi:10.4161/mabs.25269. PMID:23883920
- Jarasch A, Koll H, Regula JT, Bader M, Papadimitriou A, Kettenberger H. Developability assessment during the selection of novel therapeutic antibodies. J Pharm Sci. 2015;104(6):1885-98. doi:10.1002/jps.24430. PMID:25821140
- Liu H, Ponniah G, Zhang HM, Nowak C, Neill A, Gonzalez-Lopez N, Patel R, Cheng G, Kita AZ, Andrien B. In vitro and in vivo modifications of recombinant and human IgG antibodies. MAbs. 2014;6(5):1145-54. doi:10.4161/mabs.29883. PMID:25517300
- Rizzo JM, Shi S, Li Y, Semple A, Esposito JJ, Yu S, Richardson D, Antochshuk V, Shameem M. Application of a high-throughput relative chemical stability assay to screen therapeutic protein formulations by assessment of conformational stability and correlation to aggregation propensity. J Pharm Sci. 2015;104(5):1632-40. doi:10.1002/jps.24408. PMID:25757872
- Wang T, Kumru OS, Yi L., Wang YJ., Zhang J., Kim JH., Joshi SB., Middaugh CR., Volkin D B. Effect of ionic strength and pH on the physical and chemical stability of a monoclonal antibody antigen-binding fragment. J Pharm Sci. 2013;102(8):2520-37. doi:10.1002/jps.23645. PMID:23824562
- Liu H, Gaza-Bulseco G, Faldu D, Chumsae C, Sun J. Heterogeneity of monoclonal antibodies. J Pharm Sci. 2008;97(7):2426-47. doi:10.1002/jps.21180. PMID:17828757
- Ponniah G, Kita A, Nowak C, Neill A, Kori Y, Rajendran S, Liu H. Characterization of the acidic species of a monoclonal antibody using weak cation exchange chromatography and LC-MS. Anal Chem. 2015;87(17):9084-92. doi:10.1021/acs.analchem.5b02385
- Alt N, Zhang TY, Motchnik P, Taticek R, Quarmby V, Schlothauer T, Beck H, Emrich T, Harris RJ. Determination of critical quality attributes for monoclonal antibodies using quality by design principles. Biologicals. 2016;44(5):291-305. doi:10.1016/j.biologicals.2016.06.005. PMID:27461239
- Haberger M, Bomans K, Diepold K, Hook M, Gassner J, Schlothauer T, Zwick A, Spick C, Kepert JF, Hienz B, et al. Assessment of chemical modifications of sites in the CDRs of recombinant antibodies: Susceptibility vs. functionality of critical quality attributes. MAbs. 2014;6(2):327-39. doi:10.4161/mabs.27876. PMID:24441081
- Pisupati K, Benet A, Tian Y, Okbazghi S, Kang J, Ford M, Saveliev S, Sen KI, Carlson E, Tolbert TJ, et al. Biosimilarity under stress: a forced degradation study of Remicade® and Remsima™. MAbs. 2017;9(7): in press.