ABSTRACT
Therapeutic antibodies (Abs) are emerging as major drugs to treat respiratory diseases, and inhalation may provide substantial benefits for their delivery. Understanding the behavior of Abs after pulmonary deposition is critical for their development. We investigated the pharmacokinetics of a nebulized Ab by continuous sampling in lung parenchyma using microdialysis in non-human primates. We defined the optimal conditions for microdialysis of Ab and demonstrated that lung microdialysis of Ab is feasible over a period of several days. The concentration-profile indicated a two-phase non-linear elimination and/or distribution of inhaled mAbX. Lung exposition was higher than the systemic one over a period of 33 hours and above MabX affinity for its target. The microdialysis results were supported by an excellent relationship with dosages from lung extracts.
Introduction
Since the market release of the first therapeutic antibody (Ab) in 1986, Ab-based therapeutics have experienced a remarkable expansion and demonstrated therapeutic success in various diseases. The overall clinical pipeline was estimated to include 470 Abs in 2016,Citation1 mostly full-length IgGs (Mw ≈ 150 kDa). Respiratory diseases continue to represent major unmet medical needs and are a growing area of use for therapeutic Abs, with >40 molecules (excluding those used in lung cancer) studied in clinical trials. While Abs for cancer treatment are mainly delivered through the intravenous (i.v.) route, other routes may be beneficial for respiratory diseases because Abs do not passively diffuse through the different compartments of the body. Among these other routes, inhalation is a promising non-invasive method of delivery for Abs to treat respiratory diseases, as it has allowed for therapeutic responses in various models and leads to high Ab concentration in the lungs, while limiting Ab passage into the systemic circulation.Citation2–Citation5
Prior to clinical trials, preclinical studies are mandatory to explore the pharmacodynamics, pharmacokinetics (PK) and toxicity profiles of Abs. More precisely, PK studies characterize the distribution and local concentrations of compounds and thereby guide toxicity studies; both study types generate critical data used to select the accurate doses and regimens for clinical trials. Classically, PK parameters are estimated by monitoring drug concentrations in the systemic circulation, from which the behavior of the drug at the target site is predicted after computation by mathematical compartmental models. However, this indirect estimation of tissue concentrations requires knowledge of intrinsic and extrinsic factors influencing Ab behavior, which should be previously determined experimentally.Citation6–Citation10 Additionally, scaling across animal species is questionable for Abs because differences in antigen-Ab and FcRn-Ab binding exist, and may drive significant differences in Ab distribution and clearance. Simplified allometric scaling across species, in particular from monkey data, is only predictive (in humans) when the Ab kinetic profile is linear. In other cases, interpretations and translation of the PK results remain speculative. In the case of inhalation as a delivery route, the limited understanding of the processes and molecular drivers involved in absorption, as well as considerable species differences, makes interpreting PK results even more challenging.
To follow the behavior of inhaled Abs in relevant tissues, i.e., the lungs, few methods are available, and each has specific strength and weakness. Iterative tissue sampling uses many animals and is ethically questionable. Broncho-alveolar lavage (BAL) cannot be repeated on the same animal and reflects only the airway compartment. Real time-imaging techniques rely on conjugation of Abs to isotopes/dyes that may modify Ab binding to their target antigen or Fc receptors. Alternatively, in vivo microdialysis appears to be an interesting semi-invasive PK sampling technique to measure unmodified drugs in the interstitial spaces of various tissues, including the lungs.Citation11,Citation12 Microdialysis enables continuous repetitive sampling of drugs in a single animal using a semi-permeable membrane inserted in the tissue and perfused with physiological buffer at constant flow rate. Although mainly described for small molecules, microdialysis of proteins and Abs has recently become possible due to the availability of large molecular weight cutoff membranes and the push-pull perfusion technique to avoid fluid loss.Citation13,Citation14 Herein, we attempted to use microdialysis for the dynamic quantification of an Ab administered by inhalation in non-human primates (NHP); the Ab targets a soluble antigen in the interstitial lung space (optimized mAb provided by Sanofi Genzyme, target undisclosed for confidential reasons).
Results
We first optimized the microdialysis flow to achieve a reliable and optimal diffusion rate of the antibody of interest, mAbX, by using the experimental setup illustrated in supplemental Figure S1. As previously reported, we observed an inverse relationship between the perfusion flow rate and the mAbX diffusion rate.Citation13,Citation14 The recovery rate, corresponding to the percentage of mAbX diffusing into the perfusion fluid ()), ranged from 15.5% (1 µL/min) to 40.25% (0.2 µL/min). A flow rate of 0.3 µL/min was selected based on an acceptable duration of time to collect 50 µL of microdialysate and achieve a median recovery rate value of 28.9% [4.5%], (3 probes, 30 h of observation). It is noteworthy that the recovery rate variability was very low over a two-day period (data not shown), which encompassed the duration of the PK study. In a second step, we qualified the reversibility of the probe in vitro using an irrelevant murine IgG1 and mAbX by retrodialysis. As shown in ), the murine IgG1 (35.67% [15.33%]) displayed similar reverse diffusion to mAbX (33.40% [3.95%]), and may be considered as standard tracer for in vivo retrodialysis. The recovery rate of the murine IgG1 was investigated in vivo by retrodialysis and was equal to 27.1% [16.9%] (median value of 34 measurements performed in two animals). Overall, the recovery rate obtained in vitro with mAbX was considered accurate for correcting calculation of mAbX concentration in vivo.
Figure 1. Setup for mAbX antibody microdialysis. a) The recovery rate of mAbX using a microdialysis probe, corresponding to its diffusion through a 1 MDa cut-off semi-permeable membrane (CMA 20), was assayed in vitro. The probe was incubated with antibody (mAbX 3.3 mg/mL) solution and was perfused continuously with ringer acetate buffer at different flow rates and maintained at 37°C. For each flow rate, the recovery rate is represented by histograms with error bars (left Y axis), and the sampling duration is indicated by an empty circle (right Y axis). The recovery rate corresponds to the percent of mAbX diffusing in the perfusion fluid. It was calculated as follows: (Cmicrodialysate/Creservoir) x 100, where C corresponds to the concentration of mAbX in the dialysate or reservoir. The optimal flow rate of 0.3 µl/min was chosen based on the recovery rate and the sampling duration (180 min) required to obtain 50 µL microdialysate and was used for the following experiments. b) The recovery rate of the internal control, an irrelevant murine IgG1 isotype used later for probe calibration in the in vivo experiments, was assessed by retrodialysis. The microdialysis probe was perfused with ringer acetate buffer containing the murine IgG1 (1µg/mL, Cperfusate). To ensure that the murine IgG1 mimicked mAbX, mAbX was added in the perfusion fluid and the probe was incubated in the buffer only in some experiments. The residual murine IgG1 or mAbX (Cresidual) concentrations were measured, and the recovery rate corresponded to the percentage of murine IgG1 or mAbX lost from the perfusion fluid (retrodialysis). The retrodialysis rate was calculated as follows: ((Cperfusate – Cresidual)/Cperfusate) x 100, where C corresponds to the concentration of the murine IgG1 or mAbX in the perfusate. Results are expressed as the median [IQR] from three independent experiments. n.s. non-significant (Kruskal-Wallis test).
![Figure 1. Setup for mAbX antibody microdialysis. a) The recovery rate of mAbX using a microdialysis probe, corresponding to its diffusion through a 1 MDa cut-off semi-permeable membrane (CMA 20), was assayed in vitro. The probe was incubated with antibody (mAbX 3.3 mg/mL) solution and was perfused continuously with ringer acetate buffer at different flow rates and maintained at 37°C. For each flow rate, the recovery rate is represented by histograms with error bars (left Y axis), and the sampling duration is indicated by an empty circle (right Y axis). The recovery rate corresponds to the percent of mAbX diffusing in the perfusion fluid. It was calculated as follows: (Cmicrodialysate/Creservoir) x 100, where C corresponds to the concentration of mAbX in the dialysate or reservoir. The optimal flow rate of 0.3 µl/min was chosen based on the recovery rate and the sampling duration (180 min) required to obtain 50 µL microdialysate and was used for the following experiments. b) The recovery rate of the internal control, an irrelevant murine IgG1 isotype used later for probe calibration in the in vivo experiments, was assessed by retrodialysis. The microdialysis probe was perfused with ringer acetate buffer containing the murine IgG1 (1µg/mL, Cperfusate). To ensure that the murine IgG1 mimicked mAbX, mAbX was added in the perfusion fluid and the probe was incubated in the buffer only in some experiments. The residual murine IgG1 or mAbX (Cresidual) concentrations were measured, and the recovery rate corresponded to the percentage of murine IgG1 or mAbX lost from the perfusion fluid (retrodialysis). The retrodialysis rate was calculated as follows: ((Cperfusate – Cresidual)/Cperfusate) x 100, where C corresponds to the concentration of the murine IgG1 or mAbX in the perfusate. Results are expressed as the median [IQR] from three independent experiments. n.s. non-significant (Kruskal-Wallis test).](/cms/asset/e1c65b2c-578b-4c7d-b7ae-f2edbfeb32ff/kmab_a_1556081_f0001_b.gif)
On the basis of the in vitro results, we designed the experimental setup ()) to carry out microdialysis sampling of mAbX in vivo. Non-human primates (macaques) were chosen because they are highly similar to humans in aspects relevant to aerosol therapy (lung anatomy, delivery method) and are recommended for preclinical studies of Ab development.Citation3 Immediately after delivery of mAbX by inhalation [3 mL at 10 mg/mL for 15–20 min to obtain an average 2.9% of the dose, i.e., 0.87 mg deposited in the lungs ())] in spontaneously breathing animals, animals were anesthetized, and the microdialysis probe was implanted in the lung parenchyma by thoracic surgery. Animals were thereafter maintained under prolonged anesthesia and mechanical ventilation for up to 2 days ()). The follow-up measurements of clinical parameters showed that all physiological parameters of the animals were maintained within normal levels throughout the experiments (). Next, the circulation of perfusion fluid was started, and microdialysate and blood were sampled on a preset schedule to quantify mAbX and endogenous/control markers. The stability of the blood/microdialysate urea ratio throughout the study (median [IQR] = 1.1 [0.3], n = 9 for each animal) underlined the correct permeability of the microdialysis probe ()). ) showed the concentration-time profiles of “unbound” mAbX in the microdialysate (corrected by the in vitro recovery rate) and in the blood, which represent the lung interstitial fluid concentration and transfer of inhaled mAbX from the airways into the bloodstream, respectively. Overall, we observed: 1) a two-phase disappearance of mAbX in the microdialysate, with a linear/continuous decrease for 33 hours followed by a pseudo-plateau thereafter; and 2) low and slow passage of mAbX into the bloodstream ()) as previously described.Citation3,Citation5 It is noteworthy that the disappearance profiles showed similar trends in each animal. Although heterogeneous between animals, the lung was more exposed than the systemic circulation to mAbX ()) during the experiment, and this was more remarkable during the first 33 hours (ratio AUCmicrodialysate over AUCserum = 22.37, 1.58 and 57.55 for each animal). As shown in ), the residual amount of “unbound” mAbX corresponded to 2.4% [1.3%] of the initial amount measured in the microdialysate. This value is consistent with the residual amounts of mAbX detected in the lungs after animal sacrifices (median [IQR] = 4.9% [2.6%]) and normalized to the deposited mass estimated from the scintigraphy imaging ()). Accordingly, mAbX was immunodetected in lung sections from animals after sacrifice up to 54 hours after the inhalation, and was mainly observed within airway epithelial cells and alveolar macrophages ().
Table 1. Clinical parameters of the macaques during the microdialysis experiment. Parameters were measured each hour. Results are expressed as the median [IQR] for each macaque over a period of up to 54 hours.
Figure 2. In vivo lung microdialysis of inhaled mAbX. a) Scheme of the experimental setup. Prior to microdialysis, pulmonary deposition in each animal was determined by scintigraphy imaging. Inhalation of mAbX (3 mL of 10 mg/mL solution) was done in conscious animals, and the microdialysis probe was then immediately inserted in the lung parenchyma by thoracic surgery. Animals were maintained under mechanical ventilation and prolonged anesthesia for up to 54 hours and were monitored continuously during this period. Blood and microdialysate were collected on a predefined schedule (every 6 and 3 hours, respectively) to analyze mAbX and urea (as a control of probe permeability). At the end of the experiment, animals were sacrificed by exsanguination and lungs were excised. b) Lung deposition with the customized mesh nebulizer in conscious macaques. Scintigraphic imaging of a representative animal that received the formulation without the antibody spiked with99mTc-DTPA as a tracer and the dose (in %, right) deposited in the different regions of interest (ear/nose and throat (ENT), lungs, stomach) determined from the digitalized images using lung tissue attenuation coefficients. All results are expressed as the percentage of the activity loaded in the nebulizer and correspond to the median [IQR] (n = 3 animals). c) Urea ratio to assess the probe permeability in vivo. Urea was measured in the microdialysate and in the blood throughout the experiment. Results are expressed as the ratio of urea in the microdialysate to blood urea and correspond to the median [IQR], n = 3 animals. n.s. non-significant (Friedman test).
![Figure 2. In vivo lung microdialysis of inhaled mAbX. a) Scheme of the experimental setup. Prior to microdialysis, pulmonary deposition in each animal was determined by scintigraphy imaging. Inhalation of mAbX (3 mL of 10 mg/mL solution) was done in conscious animals, and the microdialysis probe was then immediately inserted in the lung parenchyma by thoracic surgery. Animals were maintained under mechanical ventilation and prolonged anesthesia for up to 54 hours and were monitored continuously during this period. Blood and microdialysate were collected on a predefined schedule (every 6 and 3 hours, respectively) to analyze mAbX and urea (as a control of probe permeability). At the end of the experiment, animals were sacrificed by exsanguination and lungs were excised. b) Lung deposition with the customized mesh nebulizer in conscious macaques. Scintigraphic imaging of a representative animal that received the formulation without the antibody spiked with99mTc-DTPA as a tracer and the dose (in %, right) deposited in the different regions of interest (ear/nose and throat (ENT), lungs, stomach) determined from the digitalized images using lung tissue attenuation coefficients. All results are expressed as the percentage of the activity loaded in the nebulizer and correspond to the median [IQR] (n = 3 animals). c) Urea ratio to assess the probe permeability in vivo. Urea was measured in the microdialysate and in the blood throughout the experiment. Results are expressed as the ratio of urea in the microdialysate to blood urea and correspond to the median [IQR], n = 3 animals. n.s. non-significant (Friedman test).](/cms/asset/14ed9bd3-1b0f-4f63-bbfe-0f8d6c5a7b23/kmab_a_1556081_f0002_oc.jpg)
Figure 3. Fate of mAbX after inhalation in macaques. (a) Time-concentration of mAbX after inhalation in the lung interstitium (microdialysate, blue line) and serum (red line) in macaques. Results are expressed as the mean ± SEM and representative of three animals. (b) AUC values (µg.h.L−1) for microdialysate, serum and microdialysate to serum ratio. AUC, area under the curve.
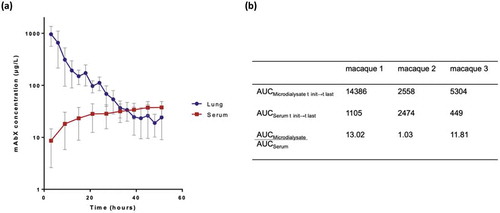
Figure 4. Immunodetection of residual mAbX in the lung tissue. Animals were sacrificed by exsanguination at the end of the microdialysis experiment, up to 54 hours after mAbX inhalation. High-magnification (×40, black scale = 20 μm) of hematoxylin staining and mAbX immunodetection in the lungs of an animal after inhalation of mAbX are represented and compared with control lung tissue from an animal that did not receive mAbX. AM: alveolar macrophages; BEC: bronchial epithelial cells.
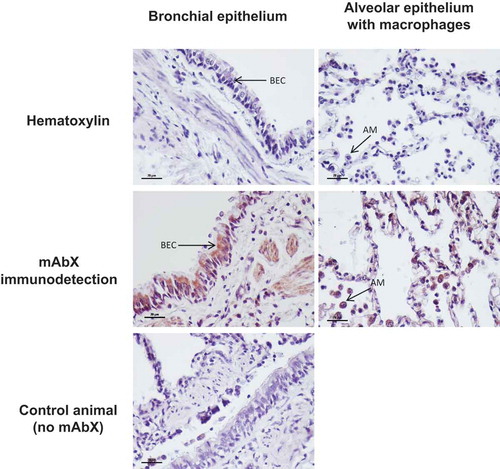
Discussion
Therapeutic Abs are emerging therapies for pulmonary diseases, including cancer and rare diseases like fibrosis. These pathologies would be better addressed with focused distribution of Ab, especially in diseases associated with marked rarefaction of blood vessels,Citation15,Citation16 which impedes the diffusion of systemic drugs into the lungs. Indeed, Abs are large macromolecules that exhibit limited distribution to the lungs after i.v. injection.Citation3,Citation17,Citation18 Because inhaled Ab passes slowly and poorly from the airways to the systemic circulation after pulmonary delivery,Citation2,Citation3 the lung interstitial concentration of inhaled mAbX is expected to be higher than that in the blood compartment. Consequently, the concentration-profile in the blood compartment may not reflect the true kinetics of inhaled mAbX in the lungs. Determining the concentration of the Ab in the lung interstitium is crucial for obtaining a true representation of the inhaled mAbX exposure in the target organ and for helping in the development of more predictive PK models. In this study, we reported for the first time the dynamic quantification of an inhaled Ab (mAbX) that targets a soluble antigen in the lung interstitium by continuous lung microdialysis in a large animal model.
In vivo microdialysis is a semi-invasive method and a powerful technique to continuously measure soluble molecules from the interstitial fluid of one animal. Although it is a widely used and well-established technique for small molecules, only a few successful examples of the technique has involved Ab microdialysis.Citation13,Citation14 Indeed, a major limitation of Ab microdialysis is the risk of low Ab recovery, mainly due to the high mass transport resistance and small aqueous diffusion coefficients typical of Abs. Herein, we used a large pore probe to favor Ab diffusion. After optimization, the selected parameters allowed a stable recovery rate of 28.9% during 30 hours of in vitro monitoring, which was remarkable for a high molecular weight protein.Citation13,Citation14 Another issue is the calibration of the microdialysis probe membrane, which should maintain its efficiency throughout the experiment to ensure the consistent appropriate measurement of the mAbX diffusion rate. As previously reported,Citation11–Citation14,Citation19 this can be achieved through different methods. Herein, we showed that measuring the disappearance of a murine IgG1, which was used as an internal standard with similar physico-chemical properties as mAbX, in vivo was in the same range of the recovery rate obtained in vitro, thus validating the use of in vitro results to calculate the accurate concentration of the molecule of interest in animals.Citation19 Besides, the measurement of an endogenous molecule, such as urea in different compartments (circulation and lung) may act as a surrogate for qualification of probe permeability during the in vivo experiment.
The dynamic quantification of inhaled mAbX in the lung interstitium by microdialysis was conducted in NHP. Inhalation therapy is probably one of the most challenging delivery routes in animal models because of the disparities between human and animal model anatomy, respiratory patterns and physiology.Citation20 Macaques are appropriate models for Ab inhalation because: 1) they allow accurate prediction of aerosol deposition in the lung and are comparable to human infants, since they are in the weight range of a full-term newborn human and display similar ventilation parameters;Citation21–Citation23 2) they can breathe spontaneously through a face mask connected to an aerosol device, although they are mainly nose breathers like infants; and 3) they are the species recommended by regulatory authorities for preclinical development of Abs. Herein, the average lung deposition of mAbX, as measured by scintigraphy, was low, since the device is not intended for infants. The dose predicted to deposit in the lungs by cascade impaction (inhalable fraction (expressed as the % of loaded mAbX): 47.1% ±3.4%; respirable fraction of mAbX > 0.5 µm and < 5 µm: 71.8% ±1.8%) is higher than the deposited dose in macaques and is compatible with aerosol therapy in humans.
The PK of inhaled mAbX was not linear. The concentration-profile indicated a two-phase absorption/elimination. This result may be explained by an alpha-phase distribution of mAbX from the lung to the systemic compartment, followed by pseudo-equilibrium of mAbX between the different compartments. The human antibody mAbX binds to a soluble protein: it cross-reacts with both the NHP antigen and endogenous neonatal Fc receptor (FcRn) recycling system. Thus, the first distribution and/or clearance phase may also be attributed to target mediated drug disposition (TMDD) Citation24 or active IgG intracellular catabolism due to saturation of FcRn. Of note, FcRn is restricted to alveolar macrophages and epithelial cells of the upper airways in NHP airways.Citation25 Additional experiments (e.g., with different doses) would be required to decipher further the mechanisms underlying non-linear PK profile of inhaled mAbX. After 33 hours, mAbX elimination was slower or null, and “soluble” mAbX was maintained at low but detectable concentrations in the lung interstitial fluid. We hypothesize that the low but sustained presence of mAbX in the interstitial fluid may be attributed to the continuous release of intracellular mAbX through FcRn recycling. This hypothesis is supported by the immunohistochemistry profile showing some staining within cells in the lungs at the end of the experiment. Of major importance from a therapeutic point of view, lung concentrations achieved during the first 24 h hours after inhalation were above the affinity constant of mAbX for its target. As anticipated,Citation3,Citation5 the amount of mAbX reaching the blood circulation was low and characterized by a slow passage. Based on the literature showing poor diffusion from the circulation to the lung compartment,Citation17,Citation18 it would have been necessary to administer a huge amount of mAbX via the i.v. route to achieve the equivalent lung exposition obtained by inhalation, thereby possibly raising toxicity concerns.
Overall, compared to classical PK studies, long-term lung microdialysis of Ab appears suitable to better understand the interstitial tissue distribution and behavior in situ of inhaled Abs targeting soluble antigens. Importantly, from an ethical point of view, this individual dynamic sampling and monitoring can reduce animal use by using each animal as its own control and follow-up. Nevertheless, even though microdialysis is considered a minimally invasive technique, complicated logistics are required, and a notably “human-like” surgical procedure is necessary to access the lungs and implant the probes without promoting tissue trauma and inflammation. In fact, the continuous lung microdialysis and the long half-life of Ab in the biological fluids necessitate expert anesthesiologist skills and dedicated animal facilities to maintain animals under adequate analgesia and sedation for prolonged mechanical ventilation. Altogether, these factors restrict the use of this promising technology to specialized teams with dedicated animal facilities.
To conclude, we achieved the dynamic quantification of an Ab in the interstitial lung space by microdialysis. By overcoming the technical, including zootechnical, challenges of microdialysis, we established, for the first time, the conditions for lung microdialysis in the lung parenchyma of NHP, thereby simultaneously evaluating the kinetics of an inhaled Ab in the lung compartment and in the bloodstream. Overall, we were able to quantify the local concentrations of a nebulized mAb and observed a complex non-linear elimination/distribution. Our dynamic results were highly supported by the excellent correspondence between the residual amounts of mAbX measured in the dialysate and the lungs after animal sacrifices more than 48 h after inhalation delivery. Altogether, our data open avenues for tests aiming to strengthen the local delivery, the residual/recycling concentration and the efficacy of therapeutic Abs.
Materials and methods
In vitro microdialysis
The mAbX was an optimized mAb provided by Sanofi Genzyme (target undisclosed for confidential reasons). A 1 MDa cut-off semipermeable membrane (CMA 20) was incubated with the antibody solution (mAbX 3.3 mg/mL) and was perfused continuously with ringer acetate buffer at different flow rates using a push-pull system (KDS Legato 272 push/pull pump) while maintained at 37°C. The recovery rate corresponds to the percent of mAbX diffusing in the perfusion fluid and was calculated as follows: (Cmicrodialysate/Creservoir) x100, where C corresponds to the concentration of mAbX in the dialysate (Cmicrodialysate) or reservoir (Creservoir) (Supplemental Figure S1). Results are representative of the recovery rate obtained with 3 probes and are expressed as median [IQR]. The recovery rate of an irrelevant murine IgG1 isotype was assessed for probe calibration in the in vivo experiments and measured by retrodialysis. In this case, the microdialysis probe was perfused with ringer acetate buffer containing the murine IgG1 (1μg/mL, Cperfusate). To ensure that the murine IgG1 mimicked mAbX, mAbX was added in the perfusion fluid and the probe was incubated in the buffer only in some experiments. The residual murine IgG1 or mAbX (Cresidual) concentrations were measured in the dialysate, and the recovery rates corresponded to the percentage of murine IgG1 or mAbX lost from the perfusion fluid (retrodialysis). The retrodialysis rate was calculated as follows: ((Cperfusate – Cresidual)/Cperfusate) x 100, where C corresponds to the concentration of the murine IgG1 or mAbX.
Animals and housing
Three healthy female cynomolgus macaques, weighing 3.6 to 4.5 kg and obtained from Bioprim®, were housed in accordance with the most recent European legislation (Directive 2010/63/UE). Animals were acclimatized to laboratory conditions and were trained to receive an aerosol before the first set of experiments. The experimental protocol was conducted according to NIH guidelines for the care and use of laboratory animals and was approved by the ethics committee of Val de Loire (n°04372.01).
Aerosol administration
Formulation and nebulization conditions as well post nebulization integrity of mAbX was previously characterized (data not shown). Macaques were placed on a restraint chair after being sedated via intramuscular (i.m.) injection of ketamine (10 mg/kg). Inhalation of mAbX (3 mL of 10 mg/mL solution) was done in conscious animals with a customized vibrating membrane eFlow® Technology nebulizer connected to the eFlow® PULSE controller at 50ms/50ms of ON/OFF associated with a baby face mask (Smartmask Baby), all from PARI Pharma GmbH. The volume mean diameter (VMD) was 3.3 ± 0.1 µm as measured by laser diffraction, and the respirable fraction (0.5µm < particles < 5µm) was 34.2% ± 6.5% with 0.9% NaCl solution. Aerosol administration lasted no longer than 15 min. In specific studies designed to evaluate pulmonary deposition in each animal with the device, a placebo solution traced with DTPA-TcCitation9, (3 mL) was delivered in the same conditions, and animals were imaged with a gamma camera (Ecam, Siemens) immediately after the aerosol session, as previously described.Citation2 Aerosol deposition in the lungs, stomach, ears, nose and throat was calculated taking into account the background, coefficient of tissue attenuation and radioactivity decay.
Implantation of the microdialysis probe and in vivo microdialysis
Immediately after aerosol delivery, animals were sedated with ketamine (10 mg/kg i.m.) then anesthetized with isoflurane. Animals were orotracheally intubated (tube internal diameter of 3.5 mm) and mechanically ventilated in a supine position with Datex Flexima ventilator. Ventilator settings were: tidal volume 70 ml, respiratory rate: 30/min, air and oxygen flows between 0.5 and 1L/min, oxygen inspiratory fraction: 50%, Pi Max: 25 cmH20, FeCO2: 35–40 mmHg. Equipment for anesthesia monitoring was set up (pulse oximetry, end-tidal carbon dioxide, non-invasive blood pressure, and temperature monitoring), as was a urinary catheter with an hourly diuresis unit, an electric warming blanket and pressure ulcer-reducing devices. After inserting two peripheral venous catheters, isoflurane was relayed by continuous injection of midazolam (0.9 to 5 mg.kg−1.h−1), ketamine (9 to 40 mg.kg−1.h−1) and buprenorphine (6 to 25 µg.kg−1.h−1) for up to 54 hours. Animals were supplemented with dextrose in normal saline solution (Glucidion G10%, injected at 2 mL/h).
The microdialysis probe was inserted in the lung parenchyma through right mini-thoracotomy under direct vision and was sutured on the lung. An intercostal pleural drain was inserted before closing the surgical wound. Arterial catheterization of the femoral artery was then performed to facilitate blood sampling and for continuous hemodynamic monitoring. The probe was continuously perfused with ringer acetate buffer at 0.3 µL/min. According to in vitro results, in some experiments a murine IgG1 (1µg/mL, Mouse IgG1 Isotype Control #MAB002, R&D System) was added to the perfusate to evaluate the in vivo recovery rate. Blood and microdialysate were collected on a predefined schedule (every 6 and 3 hours, respectively) to analyze murine IgG1 (retrodialysis), mAbX and urea (used as an additional control of probe permeability). The clinical parameters (body temperature, mean arterial pressure, arterial blood oxygen saturation, heart rate and respiratory rate) of the cynomolgus macaques were monitored continuously during this period (n = 54 for each animal). At the end of the experiment, animals were sacrificed by exsanguination, lungs were excised, and a part of each lobe was kept frozen at −80°C to measure mAbX in protein lysates.
Protein extraction and quantitative measurements of biological markers and Abs
Briefly, tissues (∼30 to 60 mg) were dissociated in RIPA buffer (1% Triton X-100, 1% sodium deoxycholate, 0.1% SDS, 150 mM NaCl, 25 mM Tri-HCl pH 7.6) with a gentleMACS Dissociator (Miltenyi M tubes, program Protein_01), using 1 mL RIPA/20 mg tissue. After 5 minutes of centrifugation at 4,000xg, supernatants were collected, and proteins were quantitated using a BCA Protein Assay Kit (Thermo Fisher Scientific). Urea was quantified by a quantitative colorimetric assay (Bioassay System) according to the manufacturer’s instructions. Murine IgG1 and mAbX were measured in the serum and/or the microdialysate by ELISA. The murine IgG1 was measured with the Mouse IgG1 Ready-SET-Go!® (affymetrix eBioscience) kit according to the manufacturer’s instructions. For mAbX detection, the home-made ELISA allowed a range of quantification from 100 ng/mL to 410 pg/mL, with intra-assay CV% (1.13 [1.10]%) and inter-assay CV% (5.81 [6.10]%).
Immunohistochemistry of mAbX in the lung tissue
For the detection of residual mAbX in the lung tissue (at the end of the experiment), the lungs were sampled and fixed in 10% neutral formalin for 24 hours. Tissue from an animal that did not receive Ab with Fc-human was used as a control. Inhaled mAbX was detected on lung sections (5 μm) with a secondary anti-human IgG (horseradish peroxidase-conjugated anti-human IgG, #2049–05, Southern Biotech), which do not cross-react with macaque IgGs (as confirmed by dot blot, data shown). The antigen was recovered by boiling the sections in a citrate-based buffer (10 mM sodium citrate, 0.05% Tween 20, pH 6.0). Endogen peroxidase activity was quenched by incubating the slides for 15 minutes in 3% H2O2 in methanol. For blocking, sections were incubated in diluted normal blocking serum (0.3% normal goat serum (#G9023, Sigma-Aldrich)) and 2.5% bovine serum albumin (#A7906, Sigma-Aldrich) in phosphate-buffered saline (PBS) 1X for 1 hour and washed twice. Next, sections were incubated with the diluted secondary peroxidase-conjugated anti-human IgG at 10 µg/mL in PBS-T (PBS 1X, 0.1% Tween 20) overnight at 4°C. They were then washed twice, and mAbX was then detected by incubation with DAB-peroxidase solution substrate (Dako, Les Ulis, France) until a satisfactory signal was obtained. The sections were counterstained with hematoxylin solution (Gill N°1, Sigma-Aldrich), cleared and mounted.
Statistical analysis
Results are expressed as median [IQR] for the indicated number of experiments performed independently. For the time-concentration profiles, results are expressed as mean ± SEM. Statistical significance between the different values was analyzed by the Kruskal-Wallis test for multi-group comparisons or by the Friedman test for non-parametric repeated measures. Statistical analyses were performed using GraphPad Prism5 software. A p value of <0.05 was considered to be significant.
Abbreviations
Abs | = | antibodies |
BAL | = | broncho-alveolar lavage |
CV | = | coefficients of variability |
Da | = | dalton |
DTPA | = | diethylenetriamine penta-acetic acid |
ELISA | = | enzyme-linked immunosorbent assay |
FcRn | = | neonatal Fc receptor |
FeCO2 | = | fraction of expired of CO2 |
IQR | = | interquartile range |
i.v. | = | intravenous |
i.m. | = | intramuscular |
NHP` | = | non-human primates |
Pi Max | = | maximal inspiratory pressure |
PBS | = | phosphate-buffered saline |
PK | = | pharmacokinetic |
RIPA buffer | = | radioimmunoprecipitation assay buffer |
SDS | = | sodium dodecyl sulfate |
SEM | = | mean standard error |
Tc99m | = | technetium-99m |
TMDD | = | target-mediated drug disposition |
VMD | = | volume mean diameter |
Authors’ contributions
AG, EG and NHV conceived and designed the experiments. AG, DM, JP, PL, CP, JM, MdM, CB and NHV performed the experiments. AG, JP, EG and NHV analyzed the data. AH, CJ, SH, PJ, RR, DM and EG contributed reagents/materials/analysis tools. AG, JP, EG and NHV wrote the paper. All authors revised and approved the manuscript.
Disclosure of Potential Conflicts of Interest
In accordance with Taylor & Francis policy and our ethical obligation as a researcher, we are reporting that PJ, CB and EG have business interests in a company (employees of Sanofi) that may be affected by the research reported in the enclosed paper. We have disclosed those interests fully to Taylor & Francis, and we have in place an approved plan for managing any potential conflicts arising from that involvement. No potential conflict of interest was reported by the other authors.
Correction Statement
This article has been republished with minor changes. These changes do not impact the academic content of the article.
Supplemental Material
Download Zip (428.4 KB)Acknowledgments
We would like to thank the members of the animal facility “PST Animaleries” of Tours (C. Aubert, B. Plante, G. Roseau, V. Schubnel), and those from Safety Pharmacology & In vivo PK supports PreClinical Safety Global Operations at Sanofi (A. Dudzicki, C. Battut, R.H Blanchard, F. Perrot), from CEPR-INSERM U1100 (E. Dalloneau, M. Cabrera and D. Le Pennec) for their technical assistance. We would like to thank the Department of the Biotherapeutic Formulation at Sanofi for providing the mAbX formulation for nebulization (S. Carayon) and the unit of molecular histopathology and bio-Imaging translational sciences (A.M. Lefebvre, R. Gorski and M. Classe) for their technical support with IHC, and the Laboratory of Biochemistry and Molecular Biology” CHRU of Tours (H. Blasco, C. Andrès) for their help in measuring urea. We acknowledge PARI Pharma GmbH (Germany) represented by M. Hahn for providing the eFlow Technology delivery system. We would like to thank Dr. B. Faivre d’Arcier and Franck Patin for their technical assistance. We thank Kimberly A. Barker for copy-editing assistance.
This work was supported by public grants overseen by the French National Research Agency (ANR) as part of the “Investissements d’Avenir” program (reference: ANR-10-LABX −53-01) and provided by Région Centre (MicroPulm).
Supplementary data
Supplemental data for this article can be accessed on the publisher’s website.
Additional information
Funding
References
- Reichert JM. Antibodies to watch in 2016. MAbs. 2016;8:197–204. doi: 10.1080/19420862.2015.1125583.
- Hervé V, Rabbe N, Guilleminault L, Paul F, Schlick L, Azzopardi N, Duruisseaux M, Fouquenet D, Montharu J, Redini F, et al. VEGF neutralizing aerosol therapy in primary pulmonary adenocarcinoma with K-ras activating-mutations. MAbs. 2014;6:1638–1648. doi:10.4161/mabs.34454.
- Guilleminault L, Azzopardi N, Arnoult C, Sobilo J, Hervé V, Montharu J, Guillon A, Andres C, Herault O, Le Pape A, et al. Fate of inhaled monoclonal antibodies after the deposition of aerosolized particles in the respiratory system. J Control Release. 2014;196:344–354. doi:10.1016/j.jconrel.2014.10.003.
- Respaud R, Marchand D, Pelat T, Tchou-Wong K-M, Roy CJ, Parent C, Cabrera M, Guillemain J, Mac Loughlin R, Levacher E, et al. Development of a drug delivery system for efficient alveolar delivery of a neutralizing monoclonal antibody to treat pulmonary intoxication to ricin. J Control Release. 2016;234:21–32. doi:10.1016/j.jconrel.2016.05.018.
- Respaud R, Vecellio L, Diot P, Heuzé-Vourc’h N. Nebulization as a delivery method for mAbs in respiratory diseases. Expert Opin Drug Deliv. 2015;12:1027–1039. doi:10.1517/17425247.2015.999039.
- Kamath AV. Translational pharmacokinetics and pharmacodynamics of monoclonal antibodies. Drug Discov Today Technol. 2016;21–22:75–83. doi:10.1016/j.ddtec.2016.09.004.
- Liu L. Pharmacokinetics of monoclonal antibodies and Fc-fusion proteins. Protein Cell. 2018;9:15–32. doi:10.1007/s13238-017-0408-4.
- Shah DK, Betts AM. Towards a platform PBPK model to characterize the plasma and tissue disposition of monoclonal antibodies in preclinical species and human. J Pharmacokinet Pharmacodyn. 2012;39:67–86. doi:10.1007/s10928-011-9232-2.
- Wang J, Iyer S, Fielder PJ, Davis JD, Deng R. Projecting human pharmacokinetics of monoclonal antibodies from nonclinical data: comparative evaluation of prediction approaches in early drug development. Biopharm Drug Dispos. 2016;37:51–65. doi:10.1002/bdd.v37.2.
- Ferl GZ, Theil F-P, Wong H. Physiologically based pharmacokinetic models of small molecules and therapeutic antibodies: a mini-review on fundamental concepts and applications. Biopharm Drug Dispos. 2016;37:75–92. doi:10.1002/bdd.v37.2.
- Elmquist WF, Sawchuk RJ. Application of microdialysis in pharmacokinetic studies. Pharm Res. 1997;14:267–288.
- Brunner M, Langer O. Microdialysis versus other techniques for the clinical assessment of in vivo tissue drug distribution. Aaps J. 2006;8:E263–271. doi:10.1208/aapsj080230.
- Jadhav SB, Khaowroongrueng V, Derendorf H. Microdialysis of large molecules. J Pharm Sci. 2016;105:3233–3242. doi:10.1016/j.xphs.2016.08.016.
- Jadhav SB, Khaowroongrueng V, Fueth M, Otteneder MB, Richter W, Derendorf H. Tissue distribution of a therapeutic monoclonal antibody determined by large pore microdialysis. J Pharm Sci. 2017;106:2853–2859. doi:10.1016/j.xphs.2017.03.033.
- Colombat M, Mal H, Groussard O, Capron F, Thabut G, Jebrak G, Brugière O, Dauriat G, Castier Y, Lesèche G, et al. Pulmonary vascular lesions in end-stage idiopathic pulmonary fibrosis: histopathologic study on lung explant specimens and correlations with pulmonary hemodynamics. Hum Pathol. 2007;38:60–65. doi:10.1016/j.humpath.2006.06.007.
- Hanumegowda C, Farkas L, Kolb M. Angiogenesis in pulmonary fibrosis: too much or not enough? Chest. 2012;142:200–207. doi:10.1378/chest.11-1962.
- Hart TK, Cook RM, Zia-Amirhosseini P, Minthorn E, Sellers TS, Maleeff BE, Eustis S, Schwartz LW, Tsui P, Appelbaum ER, et al. Preclinical efficacy and safety of mepolizumab (SB-240563), a humanized monoclonal antibody to IL-5, in cynomolgus monkeys. J Allergy Clin Immunol. 2001;108:250–257. doi:10.1067/mai.2001.117929.
- Dall’Acqua WF, Kiener PA, Wu H. Properties of human IgG1s engineered for enhanced binding to the neonatal Fc receptor (FcRn). J Biol Chem. 2006;281:23514–23524. doi:10.1074/jbc.M604292200.
- Chang H-Y, Morrow K, Bonacquisti E, Zhang W, Shah DK. Antibody pharmacokinetics in rat brain determined using microdialysis. MAbs. 2018;10:843–853. doi:10.1080/19420862.2018.1473910.
- Guillon A, Sécher T, Dailey LA, Vecellio L, de Monte M, Si-Tahar M, Diot P, Page CP, Heuzé-Vourc’h N. Insights on animal models to investigate inhalation therapy: relevance for biotherapeutics. Int J Pharm. 2018;536:116–126. doi:10.1016/j.ijpharm.2017.11.049.
- Pitance L, Vecellio L, Leal T, Reychler G, Reychler H, Liistro G. Delivery efficacy of a vibrating mesh nebulizer and a jet nebulizer under different configurations. J Aerosol Med Pulm Drug Deliv. 2010;23:389–396. doi:10.1089/jamp.2010.0816.
- Albuquerque-Silva I, Vecellio L, Durand M, Avet J, Le Pennec D, de Monte M, Montharu J, Diot P, Cottier M, Dubois F, et al. Particle deposition in a child respiratory tract model: in vivo regional deposition of fine and ultrafine aerosols in baboons. PLoS ONE. 2014;9:e95456. doi:10.1371/journal.pone.0095456.
- Réminiac F, Vecellio L, Loughlin RM, Le Pennec D, Cabrera M, Vourc’h NH, Fink JB, Ehrmann S. Nasal high flow nebulization in infants and toddlers: an in vitro and in vivo scintigraphic study. Pediatr Pulmonol. 2017;52:337–344. doi:10.1002/ppul.23526.
- Mager DE. Target-mediated drug disposition and dynamics. Biochem Pharmacol. 2006;72:1–10. doi:10.1016/j.bcp.2006.04.012.
- Roopenian DC, Akilesh S. FcRn: the neonatal Fc receptor comes of age. Nat Rev Immunol. 2007;7:715–725. doi:10.1038/nri2155.