ABSTRACT
Determination of the impact of individual antibody glycoforms on FcɣRIIIa affinity, and consequently antibody-dependent cell-mediated cytotoxicity (ADCC) previously required high purity glycoengineering. We hyphenated FcɣRIIIa affinity chromatography to mass spectrometry, which allowed direct affinity comparison of glycoforms of intact monoclonal antibodies. The approach enabled reproduction and refinement of known glycosylation effects, and insights on afucosylation pairing as well as on low-abundant, unstudied glycoforms. Our method greatly improves the understanding of individual glycoform structure–function relationships. Thus, it is highly relevant for assessing Fc-glycosylation critical quality attributes related to ADCC.
Fcɣ receptors (FcɣR) are key elements in many immunological responses. FcɣRIIIa has an important role in mediating antibody-dependent cell-mediated cytotoxicity (ADCC). Thus, investigating antibody–FcɣRIIIa interactions is highly relevant for fundamental and clinical research, as well as (bio)therapeutic innovations.Citation1,Citation2 The Fc glycosylation of immunoglobulin G (IgG) has large effects on its FcɣRIIIa interaction.Citation3 Most significantly, IgG fucosylation reduces FcɣRIIIa affinity by up to 100-fold. This is in part attributable to a unique glycan–glycan interaction between the IgG Fc and the FcɣRIIIa N162 glycan.Citation4 Knowledge of the effects of IgG glycosylation led to the successful development and approval of next-generation, glycoengineered therapeutic monoclonal antibodies (mAbs) with low fucose levels, such as Gazyva (obinutuzumab, Roche/Genentech).Citation5,Citation6 In contrast to the effects of fucosylation, galactosylation of the IgG Fc glycan slightly increases the FcɣRIIIa affinity.Citation7,Citation8 Several glycosylation traits are therefore critical quality attributes.Citation9
Analytical methods for assessing effector functions and Fc/Fc-receptor interactions in therapeutic antibodies were recently reviewed.Citation1,Citation10 Functional cellular activity assays have the advantage of high relevance towards the in vivo situation. The downstream signaling is determined by a complex interplay of immune complexes binding to activating and inhibitory FcɣR.Citation2 The interaction of FcɣRIIIa with the Fc portion occurs in an asymmetrical 1:1 stoichiometry that precludes cellular activation in vivo by monomeric antibodies.Citation2,Citation11 Thus, the translation of functional cellular assays is more straightforward compared to cellular or cell-free binding assays studying monomeric antibodies. However, the fundamental interactions of Fc and FcɣRIIIa do not depend on whether IgG is present in monomeric form or as an immune complex, and it is important to study them. Several cell-free physicochemical assays are well established and generally, the first choice for assessing the potential impact of monomeric IgG Fc attributes on receptor binding due to their increased analytical performance with regard to assay complexity, affinity resolution, and robustness.Citation10 Moreover, these binding assays strongly linked in vitro FcɣRIIIa affinity and ADCC activity.Citation7,Citation8,Citation12 Explicitly, retention time differences in FcɣRIIIa affinity liquid chromatography (AC) were linked to ADCC.Citation8
A common disadvantage of all previous methods is the averaged and potentially biased output because of the naturally occurring IgG glycoform heterogeneity. Therefore, unraveling the impact of individual IgG glycoforms on FcɣRIIIa affinity previously required laborious glycoengineering. The lack of molecular resolution of established in vitro affinity assessment techniques, such as surface plasmon resonance (SPR) or AC necessitated high IgG glycoform purity.Citation8,Citation12,Citation13
Here, we present the simultaneous assessment of FcɣRIIIa affinity of multiple IgG glycoforms of a therapeutic mAb. This was achieved by AC hyphenated to mass spectrometry (MS). MS allowed molecular resolution while the separation dimension provides FcɣRIIIa (V158) affinity. Advantageous features are: (1) affinity assessment of individual, previously unstudied glycoforms from biosynthetic mixtures, omitting the need for advanced glycoengineering; and (2) increased affinity differentiation compared to established in vitro techniques due to the simultaneous assessment.
MS has become an important technique for characterizing intact proteins.Citation14–Citation17 The combination with AC (AC-MS) proved its potential for functional characterization of therapeutic mAbs recently. AC-MS based on the fetal/neonatal Fc receptor (FcRn) showed decreased FcRn affinity, and by extension IgG half-life, for a mAb oxidized at M255.Citation18 However, FcRn affinity is only very weakly influenced by glycosylation.Citation12,Citation13 Therefore, resolving complex glycosylation heterogeneity molecularly on an intact protein level is necessary for FcɣRIIIa-AC-MS, which makes it more challenging and more powerful at the same time. For method development, a good balance between MS response and separation efficiency is crucial. In contrast to previous AC-UV studies,Citation13 we employed a simple ammonium acetate buffer. We optimized ammonium acetate concentration and linear pH gradient (Supplemental ), aiming at an at least equal separation efficiency compared to the previously reported FcɣRIIIa AC-UV conditions ()). This was achieved using 50 mM ammonium acetate and a pH gradient from pH 5 – pH 3 ()). We used the same column for which FcɣRIIIa AC retention times were previously linked to ADCC and obtained comparable profiles ().Citation8
Figure 1. FcɣRIIIa affinity chromatography for a therapeutic mAb. (a) UV chromatogram using reported non-MS compatible conditions. (b) AC-MS under MS-compatible conditions represented by extracted ion chromatograms of detected glycoforms. (c and d) Deconvoluted mass spectra and charge state distribution (inserts) of (c) 2x fucosylated (18– 34 min) and (d) remaining glycoforms (34– 42 min). In case of multiple possibilities (asterisk), the most probable glycoform is presented, based on reference data (Supplemental Table 7); all possible glycoforms and their structures are listed in Supplemental Table 1 and 4.
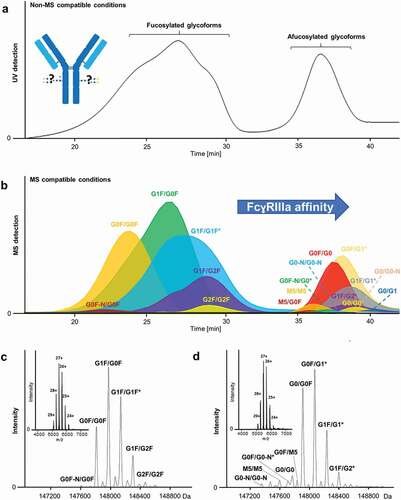
A 15 T Fourier transform ion cyclotron resonance (FT-ICR)-MS instrument provided high sensitivity and mass accuracy for determining even low-abundant glycoforms (), Supplemental Table 3 and 4). We detected 21 compositions, reflecting 27 partially isomeric glycoforms ( and Supplemental Table 4). Typical charge state distributions ([M + 24H]24+ to [M + 29H]29+) of folded protein conformations were observed for the entire pH gradient (inserts )). The method showed very good intra- and inter-day variability of relative peak areas for the resolved glycoforms of the mAb (Supplemental Figure 3 and Table 5). Further, relative peak areas of glycoforms of the intact mAb showed good agreement compared to the glycoform profile based on released glycan analysis (Supplemental Figure 5). This demonstrated the feasibility of performing relative quantitation and provides additional proof of correct glycoform assignment.
AC-MS enables the simultaneous in vitro comparison of FcɣRIIIa affinity of antibody glycoform mixtures, in contrast to SPR or AC-UV. Previously, FcɣRIIIa affinities of seven glycoengineered mAbs were assessed using AC-UV.Citation13 More recently, AC-UV and subsequent glycan analysis were used to assess the heterogeneity of Fc-glycosylation of a therapeutic antibody, using a non-glycosylated FcɣRIIIa.Citation19 However, glycosylation has been shown to be critical for differential recognition of IgG-glycoforms by wild-type FcɣRIIIa.Citation20 Thus, no significant differences for individual glycoforms, except for galactosylation levels, were observed. With our method, we can directly compare the affinity of 21 glycoforms present in a therapeutic mAb without the need for fractionation or glycoengineering. Herein, we use a human embryonic kidney (HEK) cell-produced FcɣRIIIa. This may lead to altered glycosylation and affect affinity/selectivity compared to the natural receptor. Currently, available natural FcɣRIIIa data is missing site-specificity or is inferred (and incomplete).Citation20,Citation21 While the majority of CHO and HEK cell glycoforms seems to qualitatively overlap with natural glycosylation, neither seems good at producing triantennary structures.Citation22 HEK cells can produce antennary fucosylation, but also unnatural LacDiNAc structures.Citation22 At the same time, the functional impact of individual FcɣRIIIa glycoforms is unclear.
Fucosylation is known to have the highest impact on FcɣRIIIa affinity.Citation3,Citation23 Single afucosylation is sufficient to drastically increase binding, owing to the asymmetric 1:1 binding between IgG and FcɣRIIIa.Citation11,Citation24 Consequently, glycoforms containing fucosylation on both heavy chains (2x fucosylated) populate the earlier eluting peak in the AC chromatogram ()). The 1x (a)fucosylated and 2x afucosylated species are found in the later eluting AC peak (). However, the AC-MS system achieves a much more detailed differentiation of affinity than AC-UV, because it can rank FcɣRIIIa affinity of multiple (partially) co-eluting glycoforms in one run (Supplemental Table 6). In contrast, AC-UV requires comparison of multiple runs, making retention time stability a limiting factor. Other assays only give an average value for all glycoforms present, resulting in a potentially significant impact of even low-abundant glycoforms of vastly higher affinity. As a result, we could demonstrate that 2x afucosylated complex glycoforms (G0(N)/G0(N), G0(N)/G1(N)) show an increased FcɣRIIIa affinity over 1x (a)fucosylated () and )). Furthermore, AC-MS can distinguish the positive influence of galactosylation on FcɣRIIIa affinity in all fucosylation variants (), ), Supplemental Figure 4). Previously, this was only observed for 2x fucosylated glycoforms by AC-UV and mainly 1x afucosylated glycoforms by AC-UV and SPR.Citation7,Citation8,Citation13 More recently, a comprehensive binding study applying AC-UV, SPR and ADCC analysis showed the positive influence of afucosylation and galactosylation for highly homogeneous 2x fucosylated glycoforms and 0x fucosylated glycoforms.Citation12
Figure 2. Extracted ion chromatograms of AC-MS with glycoengineered mAbs containing a high level of bisected and afucosylated glycoforms (a) and high level of bisected and fucosylated glycoforms (b). Increased affinity is observed for afucosylation compared to 1x (a)fucosylation. Bisected fucosylated glycoforms showed also increased affinity compared to non-bisected fucosylated glycoforms. Positive effect of galactosylation is represented for each glycosylation feature.
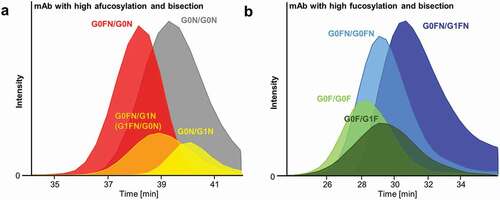
A further challenge in glycoengineering is biosynthetic interferences between the different glycosylation features.Citation7,Citation25 For example, bisection correlates with other glycosylation features depending on the expression system, which complicates independent affinity assessment.Citation25,Citation26 Furthermore, glycoengineered mAbs often still exhibit a high degree of glycosylation heterogeneity.Citation26 The high molecular resolution of our method allows the study of subtle FcɣRIIIa affinity differences by dissecting the contribution of individual glycosylation features. For example, we could demonstrate that bisection increases FcɣRIIIa affinity independent of afucosylation and galactosylation.Citation7,Citation26 This was observed regardless of the presence of galactoses ()).
In addition, FcɣRIIIa affinity of typically low-abundant glycoforms (≈0.1% relative abundance, Supplemental Table 5) could be studied ()). For example, mono-antennary glycoforms lacking N-acetylglucosamine (-N) showed decreased affinity compared to its complex bi-antennary glycoforms (e.g., G0F/G0F-N vs. G0F/G0F, ), Supplemental Figure 4 and Table 6). Glycoengineering, and therefore classical affinity assessment, of low-abundant species, is often deemed too tedious, a limitation efficiently addressed by our method.
High mannose species have been shown to increase ADCC activity via FcɣRIIIa binding that was attributed to the lack of core-fucose.Citation27 A minor amount of high mannose glycans, paired with either another high mannose (0.8% M5/M5) or the complex glycan (0.2% M5/G0F), was detected in the therapeutic mAb ()).Citation28 Largely, these showed higher affinity compared to 2x fucosylated glycoforms. However, the affinity of M5-containing glycoforms was reduced compared to 1x (a)fucosylated complex glycoforms. These findings are supported by previous studies describing increased binding affinitiesCitation12,Citation13,Citation27 and ADCC activitiesCitation12,Citation27 of high mannose glycoforms over 2x fucosylated complex glycoforms, and similar or decreased affinities and activities compared to afucosylated complex glycoforms.Citation12,Citation13,Citation27 Identity and affinity of the M5/M5 were confirmed by glycoengineering (Supplemental Figure 6 and 7). Moreover, the isolated analysis of the glycoengineered M5/M5 mAb showed comparable retention as observed in the therapeutic mAb, giving no indication for a measurable bias in retention behavior caused by competitive receptor binding of other glycoforms.
Glycoforms with one non-glycosylated heavy chain (X/NG) are normally also found to a low extent in therapeutic antibodies.Citation29 Decreased binding affinities and ADCC activities were observed for these glycoforms in previous studies.Citation30 We observed elution of fucosylated glycoforms (G0F/NG, G1F/NG, G2F/NG) already before the pH gradient (for initial pH of 5) started, whereas G0/NG showed the lowest affinity of the afucosylated glycoforms, but it exhibited a higher affinity compared to the 2x fucosylated glycoforms (Supplemental Table 6, Supplemental Figure 4).
In conclusion, FcɣRIIIa AC-MS represents a great advance in characterizing the impact of antibody Fc glycosylation on FcɣRIIIa affinity. It omits the need for high purity glycoengineering, which is notoriously difficult, especially for biosynthetic pathway intermediates,Citation24 and has hitherto complicated and, in part, prohibited the affinity assessment of various glycoforms. We were able to perform an FcɣRIIIa affinity assessment of individual glycoform pairings present in a therapeutic mAb, including so far unstudied, low-abundant glycoforms (e.g., G0F-N/G0F). Due to its high molecular and high FcɣRIIIa affinity resolution, the method allows independent assessment of individual glycosylation features. For example, we could show, for the first time, the increased affinity of afucosylated complex glycoforms over singly (a)fucosylated complex glycoforms and high mannose glycoforms. Furthermore, we could support the independent, positive influence of bisection. FcɣRIIIa AC-MS will allow a fast and reliable assessment of FcɣRIIIa affinity of novel (synthetic) glycoforms, even in the presence or excess of other glycoforms.
FcɣRIIIa AC-MS may be highly useful in other applications. For example, other post-translational modifications as well as protein backbone differences may be analyzed in addition to glycosylation, again separating independent effects. Thus, FcɣRIIIa AC-MS will be an enormously valuable tool in studying and tuning ADCC activation of (therapeutic) antibodies. Largely by the omission of high purity glycoengineering, proteoform-related critical quality attributes and their interactions could be defined earlier in therapeutic mAb development. In the future, glycoengineering of FcɣRIIIa towards natural glycosylation would be highly desirable. In general, AC-MS approaches have a high potential for studying biomolecular interactions in a proteoform-resolved manner and are extremely valuable in unraveling structure–function relationships.
Materials and methods
Chemicals
All chemicals were purchased from Sigma-Aldrich (Steinheim, Germany) and had at least analytical grade quality. Deionized water was used from a Purelab ultra (ELGA Labwater, Ede, The Netherlands). Ammonium acetate solution (7.5 M, Sigma-Aldrich) and glacial acetic acid (Fluka-Honeywell) were used for preparing MS-compatible mobile phases.
Antibodies
In this study, four different monoclonal antibodies (mAbs) differing in glycosylation were used. First, a standard therapeutic mAb covering a typical degree of glycosylation heterogeneity was investigated. In addition, a glycoengineered high mannose-containing variant of the first mAb and a mAb contained a high level of afucosylation and bisection were analyzed. Lastly, a highly fucosylated and highly bisected anti-D mAb were investigated (reported as +B).Citation7 The first three mAbs were provided by Roche Diagnostics (Penzberg, Germany) and the last one by Sanquin (Amsterdam, The Netherlands).
FcɣRIIIa column preparation
FcɣRIIIa affinity column was provided by Roche Diagnostics (Penzberg, Germany). The preparation of the FcɣRIIIa affinity column is reported elsewhere.Citation8 In brief, biotinylated human FcɣRIIIa_V158 (HEK cell-produced with glycosylation profile as reportedCitation22) was incubated with streptavidin sepharose for 2 h upon mild shaking and the receptor-derivatized sepharose was packed in a Tricorn 5/50 Column housing (inner diameter 5 mm x length 50 mm, GE Healthcare).
FcɣRIIIa affinity liquid chromatography
For FcɣRIIIa affinity liquid chromatography with non-MS compatible conditions and UV detection (absorbance measured at 280 nm), previously reported pH gradient and mobile phase composition were used.Citation13 Equilibration buffer/mobile Phase A consisted of 20 mM sodium citrate pH 6.0 and 150 mM NaCl. Mobile phase B contained 20 mM citrate pH 3.0 and 150 mM NaCl. Final MS-compatible conditions contained 50 mM ammonium acetate pH 5.0 (mobile phase A) and 50 mM ammonium acetate pH 3.0 (mobile phase B). All experiments were performed using a biocompatible Thermo Ultimate3000 instrument at 25 C. The samples were diluted with mobile phase A, if necessary (conc. 1–5 µg/µL). Injection volume was set to 10 µL. The flow rate was set to 500 µL/min. For hyphenation with MS, the flow rate was reduced from 500 µL/min to 30 µL/min via flow-splitting prior to the electrospray ionization (ESI) source. Upon injection, the column was washed with 5 column volumes (10.5 min) of mobile phase A, and the sample was eluted in a linear gradient of 15 column volumes (31.5 min) ending with 100% mobile phase B. In addition, a washing step using 5 column volumes (10.5 min) of mobile phase B and a linear pH gradient of 7.5 min ending with 100% mobile phase A was applied after the elution gradient. Re-equilibration using 15 column volumes (31.5 min) of mobile phase A was applied between runs.
Fourier transform ion cyclotron resonance mass spectrometry
Online ESI-MS coupling of FcɣRIIIa AC was performed on a Bruker 15 T solariX FT-ICR-MS (Bruker Daltonics, Bremen, Germany). Instrument tuning and calibration were performed using direct infusion (2 µL/min) of the therapeutic mAb (0.1 µg/µL in mobile phase A) (Supplemental Figure 2 and Table 2). The m/z of all charge states of the main glycoform (G0F/G1F) were used for calibration. By using the charge state envelope of the most abundant glycoform, the relevant mass range was sufficiently covered. External calibration was not needed for the intended purpose of the study because the masses and glycoforms of the analyzed mAbs were known. ESI capillary voltage was set to 4000 V and endplate offset to −500 V. Nebulizer gas pressure was set to 0.8 bar, dry gas to 3 L/min and source temperature to 200 C. The ion funnel 1 was operated at 150 V, radio frequency amplitude at 300 Vpp and skimmer 1 at 125 V. Spectra were acquired in an m/z-range of 506–20,000. Accumulation time was set to 1 s. The amount of data points was set to 128 k. Each spectrum in serial mode analysis resulted from the summation of 20 spectra. This resulted in the acquisition of 2.6 data points per minute in the chromatogram. This acquisition rate was chosen as a compromise of sensitivity (for low-abundant glycoforms) and resolution, and it was sufficient for the generally broad eluting peaks observed in this study. All mass spectra were visualized and processed using DataAnalysis 5.0 (Bruker Daltonics). For deconvolution, the Maximum Entropy tool was used (145,000–150,000 Da, datapoint spacing = 1, instrument resolving power = 3000). Further information regarding data processing are described in the Supplemental Material.
Supplemental Material
Download MS Word (405.2 KB)Acknowledgments
This research was supported by Roche Diagnostics, the European Union (Analytics for biologics project, Grant No. 765502), and the NWO (Vernieuwingsimpuls Veni Project No. 722.016.008 and SATIN, Project No. 731.017.202). We are grateful to Tilman Schlothauer from Roche Diagnostics for providing the FcɣRIIIa column.
Disclosure statement
A. Heidenreich, D. Reusch, and M. Haberger are employees of Roche Diagnostics, the manufacturer of the column and the therapeutic antibodies used in this research.
Supplemental material
Supplemental data for this article can be accessed on the publisher’s website.
Additional information
Funding
References
- Jiang XR, Song A, Bergelson S, Arroll T, Parekh B, May K, Chung S, Strouse R, Mire-Sluis A, Schenerman M. Advances in the assessment and control of the effector functions of therapeutic antibodies. Nat Rev Drug Discov. 2011;10:101–11. doi:10.1038/nrd3365.
- Nimmerjahn F, Ravetch JV. Fcγ receptors as regulators of immune responses. Nat Rev Immunol. 2008;8:34–47. doi:10.1038/nri2206.
- Shields RL, Lai J, Keck R, O’Connell LY, Hong K, Meng YG, Weikert SH, Presta LG. Lack of fucose on human IgG1 N-linked oligosaccharide improves binding to human FcγRIII and antibody-dependent cellular toxicity. J Biol Chem. 2002;277:26733–40. doi:10.1074/jbc.M202069200.
- Ferrara C, Grau S, Jäger C, Sondermann P, Brünker P, Waldhauer I, Hennig M, Ruf A, Rufer AC, Stihle M, et al. Unique carbohydrate–carbohydrate interactions are required for high affinity binding between FcγRIII and antibodies lacking core fucose. Proc Natl Acad Sci USA. 2011;108:12669–74. doi:10.1073/pnas.1108455108.
- Beck A, Reichert JM. Marketing approval of mogamulizumab: a triumph for glyco-engineering. mAbs. 2012;4:419–25. doi:10.4161/mabs.20996.
- Evans JB, Syed BA. Next-generation antibodies. Nat Rev Drug Discov. 2014;13:413–14. doi:10.1038/nrd4255.
- Dekkers G, Treffers L, Plomp R, Bentlage AEH, de Boer M, Koeleman CAM, Lissenberg-Thunnissen SN, Visser R, Brouwer M, Mok JY, et al. Decoding the human immunoglobulin G-glycan repertoire reveals a spectrum of Fc-receptor- and complement-mediated-effector activities. Front Immunol. 2017;8. doi:10.3389/fimmu.2017.00877.
- Thomann M, Schlothauer T, Dashivets T, Malik S, Avenal C, Bulau P, Rüger P, Reusch D, Chammas R. In vitro glycoengineering of IgG1 and its effect on Fc receptor binding and ADCC activity. PLoS One. 2015;10:e0134949. doi:10.1371/journal.pone.0134949.
- Reusch D, Tejada ML. Fc glycans of therapeutic antibodies as critical quality attributes. Glycobiology. 2015;25:1325–34. doi:10.1093/glycob/cwv065.
- Cymer F, Beck H, Rohde A, Reusch D. Therapeutic monoclonal antibody N-glycosylation–structure, function and therapeutic potential. Biologicals. 2018;52:1–11. doi:10.1016/j.biologicals.2017.11.001.
- Sondermann P, Huber R, Oosthuizen V, Jacob U. The 3.2-Å crystal structure of the human IgG1 Fc fragment–fcγRIII complex. Nature. 2000;406:267. doi:10.1038/35018508.
- Wada R, Matsui M, Kawasaki N. Influence of N-glycosylation on effector functions and thermal stability of glycoengineered IgG1 monoclonal antibody with homogeneous glycoforms. mAbs. 2019;11:350–72. doi:10.1080/19420862.2018.1551044.
- Dashivets T, Thomann M, Rueger P, Knaupp A, Buchner J, Schlothauer T, Karagiannis SN. Multi-angle effector function analysis of human monoclonal IgG glycovariants. PLoS One. 2015;10:e0143520. doi:10.1371/journal.pone.0143520.
- Li H, Nguyen HH, Loo RRO, Campuzano IDG, Loo JA. An integrated native mass spectrometry and top-down proteomics method that connects sequence to structure and function of macromolecular complexes. Nat Chem. 2018;10:139–48. doi:10.1038/nchem.2908.
- Rosati S, Yang Y, Barendregt A, Heck AJR. Detailed mass analysis of structural heterogeneity in monoclonal antibodies using native mass spectrometry. Nat Protoc. 2014;9:967–76. doi:10.1038/nprot.2014.057.
- Bailey AO, Han G, Phung W, Gazis P, Sutton J, Josephs JL, Sandoval W. Charge variant native mass spectrometry benefits mass precision and dynamic range of monoclonal antibody intact mass analysis. mAbs. 2018;10:1214–25. doi:10.1080/19420862.2018.1521131.
- Campuzano ID, Li H, Bagal D, Lippens JL, Svitel J, Kurzeja RJ, Xu H, Schnier PD, Loo JA. Native MS analysis of bacteriorhodopsin and an empty nanodisc by orthogonal acceleration time-of-flight, orbitrap and ion cyclotron resonance. Anal Chem. 2016;88:12427–36. doi:10.1021/acs.analchem.6b03762.
- Gahoual R, Heidenreich A-K, Somsen GW, Bulau P, Reusch D, Wuhrer M, Haberger M. Detailed characterization of monoclonal antibody receptor interaction using affinity liquid chromatography hyphenated to native mass spectrometry. Anal Chem. 2017;89:5404–12. doi:10.1021/acs.analchem.7b00211.
- Kiyoshi M, Caaveiro JM, Tada M, Tamura H, Tanaka T, Terao Y, Morante K, Harazono A, Hashii N, Shibata H, et al. Assessing the heterogeneity of the Fc-glycan of a therapeutic antibody using an engineered FcγReceptor IIIa-immobilized column. Sci Rep. 2018;8:3955. doi:10.1038/s41598-018-22199-8.
- Patel KR, Roberts JT, Subedi GP, Barb AW. Restricted processing of CD16a/Fc γ receptor IIIa N-glycans from primary human NK cells impacts structure and function. J Biol Chem. 2018;293:3477–89. doi:10.1074/jbc.RA117.001207.
- Washburn N, Meccariello R, Duffner J, Getchell K, Holte K, Prod’homme T, Srinivasan K, Prenovitz R, Lansing J, Capila I, et al. Characterization of endogenous human FcγRIII by mass spectrometry reveals site, allele and sequence specific glycosylation. Mol Cell Proteomics. 2019;18:534–45. doi:10.1074/mcp.RA118.001142.
- Zeck A, Pohlentz G, Schlothauer T, Peter-Katalinić J, Regula J. Cell type-specific and site directed N-glycosylation pattern of FcγRIIIa. J Proteome Res. 2011;10:3031–39. doi:10.1021/pr1012653.
- Jefferis R. Glycosylation as a strategy to improve antibody-based therapeutics. Nat Rev Drug Discov. 2009;8:226–34. doi:10.1038/nrd2804.
- Shatz W, Chung S, Li B, Marshall B, Tejada M, Phung W, Sandoval W, Kelley RF, Scheer JM. Knobs-into-holes antibody production in mammalian cell lines reveals that asymmetric afucosylation is sufficient for full antibody-dependent cellular cytotoxicity. mAbs. 2013;5:872–81. doi:10.4161/mabs.26307.
- Ferrara C, Brünker P, Suter T, Moser S, Püntener U, Umaña P. The carbohydrate at FcγRIIIa Asn-162: an element required for high affinity binding to non-fucosylated IgG glycoforms. Biotechnol Bioeng. 2006;93:851–61. doi:10.1074/jbc.M510171200.
- Dekkers G, Plomp R, Koeleman CA, Visser R, Von Horsten HH, Sandig V, Rispens T, Wuhrer M, Vidarsson G. Multi-level glyco-engineering techniques to generate IgG with defined Fc-glycans. Sci Rep. 2016;6:36964. doi:10.1038/srep36964.
- Yu M, Brown D, Reed C, Chung S, Lutman J, Stefanich E, Wong A, Stephan J-P, Bayer R. Production, characterization and pharmacokinetic properties of antibodies with N-linked Mannose-5 glycans. mAbs. 2012;4:475–87. doi:10.4161/mabs.20737.
- Liu YD, Flynn GC. Effect of high mannose glycan pairing on IgG antibody clearance. Biologicals. 2016;44:163–69. doi:10.1016/j.biologicals.2016.02.003.
- Yang Y, Wang G, Song T, Lebrilla CB, Heck AJ. Resolving the micro-heterogeneity and structural integrity of monoclonal antibodies by hybrid mass spectrometric approaches. MAbs. 2017;9:638–45. doi:10.1080/19420862.2017.1290033.
- Ha S, Ou Y, Vlasak J, Li Y, Wang S, Vo K, Du Y, Mach A, Fang Y, Zhang N. Isolation and characterization of IgG1 with asymmetrical Fc glycosylation. Glycobiology. 2011;21:1087–96. doi:10.1093/glycob/cwr047.