ABSTRACT
Phage display technology in combination with next-generation sequencing (NGS) currently is a state-of-the-art method for the enrichment and isolation of monoclonal antibodies from diverse libraries. However, the current NGS methods employed for sequencing phage display libraries are limited by the short contiguous read lengths associated with second-generation sequencing platforms. Consequently, the identification of antibody sequences has conventionally been restricted to individual antibody domains or to the analysis of single domain binding moieties such as camelid VHH or cartilaginous fish IgNAR antibodies. In this study, we report the application of third-generation sequencing to address this limitation. We used single molecule real time (SMRT) sequencing coupled with hairpin adaptor loop ligation to facilitate the accurate interrogation of full-length single-chain Fv (scFv) libraries. Our method facilitated the rapid isolation and testing of scFv antibodies enriched from phage display libraries within days following panning. Two libraries against CD160 and CD123 were panned and monitored by NGS. Analysis of NGS antibody data sets led to the isolation of several functional scFv antibodies that were not identified by conventional panning and screening strategies. Our approach, which combines phage display selection of immune libraries with the full-length interrogation of scFv fragments, is an easy method to discover functional antibodies, with a range of affinities and biophysical characteristics.
Introduction
Display technologies, such as phage, yeast and ribosome display are important tools in the identification of therapeutic antibodies.Citation1 In particular, phage display is widely used at several stages of the drug discovery process. Phage library diversity can be derived from naïve human B cells,Citation2 synthetic engineered antibody sequencesCitation3 or from B cell-rich tissue of immunized animals;Citation4 libraries generated by the latter method are often referred to as immune phage display libraries. Immune phage display offers several advantages over naïve or synthetic display approaches, increasing the likelihood of isolating stable, high-affinity specific antibodies due to pre-enrichment and in-vivo affinity maturation.Citation5
Traditional isolation of antibodies from phage display libraries involves multiple rounds of panning followed by screening and sequencing of positive clones. A key limitation when identifying clones is the screening step following enrichment. Selecting clones can be technically challenging and time consuming, and the process is often hampered by dominant clones within the enriched population, which makes it difficult to identify alternative binders with lower representation within the pool.
Despite recent advances in next-generation sequencing (NGS) technologies, sequence read length is a limitation in their application to phage display screening. The majority of NGS platform technologies allow a maximum read length of up to 500 base pair (bp),Citation6 which has extensively been used for the analysis of immunoglobulin (Ig)-repertoires in several species. This length, however, is not sufficient to cover a full single-chain variable fragment (scFv) sequences.Citation7,Citation8 Currently, sequencing of the entire variable heavy (VH) and variable light (VL) fragment of a single chain is not routinely carried out and is not possible using conventional NGS platforms. Single-molecule real-time (SMRT) sequencing allows the contiguous sequencing of fragments of up to 8500 bp,Citation9 but read length comes at the expense of sequence fidelity.Citation10 A recent advance in SMRT sequencing is the addition of hairpin loops to double-stranded DNA fragments.Citation11 Consensus sequencing of adaptor ligated fragments can increase the sequence accuracy of an 850 bp fragment (the approximate length of an average scFv) to 99.99%.Citation12 Hemadou et al. first demonstrated this approach to be effective at accurately identifying full-length scFvs in an enriched fully human, recombinant scFv antibody library.Citation13 A separate group has also used it to investigate more in-depth the polyclonal response in phage libraries from simian immunodeficiency virus-infected Rhesus macaque.Citation14 In both these studies, the analysis was limited to the evaluation of the variable genes repertoire to identify paired VH-VL clones present in their phage display libraries, and no insights on the binding characteristics of the libraries are provided.
Sequencing of the entire scFv in one read identifies paired, functional scFv sequences, without time-consuming screening. Alternative methods of antibody discovery, such as single-cell cloning of antigen-specific B cells, have improved the ability to identify rare antigen specificities. However, these methods are time and resource consuming, and are limited to analysis of a small fraction of the antibody repertoire generated in an animal.Citation15,Citation16 Here, we have expanded the combination of phage display panning with SMRT sequencing and open source computational tools to interrogate more in-depth the scFv sequences contained in phage display-selected libraries. This method enables the rapid isolation of functional and specific antibodies from selected phage display libraries, allowing the identification of diverse antibody clones with a broad range of affinities, epitopes and biophysical characteristics.
Results
SMRT sequencing illuminates the diversity obtained in a scFv library against CD160 before and after enrichment
We analyzed the enrichment of a phage display library against CD160 derived from rats immunized with DNA encoding full-length protein. The library was subjected to three rounds of panning against recombinant antigen. We looked at clonal evolution within the library after each round. Data sets were aligned against the Rattus norvegicus germline database.Citation17 A total of 9434, 9336, 22660, and 14368 functional reads were obtained from the sequencing of pan 0, 1, 2 and 3, respectively. We investigated the IGHV/IGHJ and IGKV-KJ usage in clones to give an indication of changes in library diversity through rounds of panning. The frequencies of variable and joining regions were visualized using circular correlation analysis (). In pan 0, 519 clones used IGHV1-43 paired with IGHJ2 correlating to 5.5% of the unpanned library. After three rounds of selection, this pairing represented 0.37% of the library, while there was a concomitant increase in the presence of clones using the IGHV5S13 and IGHJ2 gene segments from 2.6% to 60.1%. This demonstrates that the library approached clonality after the third selection round. A similar pattern was observed with kappa chain frequency, where there was an increase from 1.1% to 33% of clones using the pairing IGKV4S9 IGKJ2. A more modest enrichment of IGKV-J pairs is indicative of the ability of antibody heavy chains to pair promiscuously with multiple light chains while retaining function.
Figure 1. Analysis of CD160 library diversity after phage display enrichment
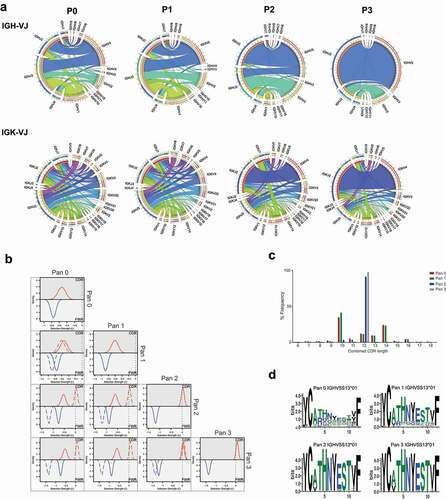
To further understand the depth and diversity of sequence, we performed a Bayesian analysis of the VH regions within the library at each round of panning.Citation18,Citation19 Mutations across IGHV regions of a group of sequences were compared to the closest parent germline sequence and assigned a selection score Σ. For example, ΣFWR of −1, 0 and 1 indicate strongly negative, neutral, and strongly positive selection, respectively, to the framework regions (FWR) of the sequences (). In pan 0, the highest frequency germline used was IGHV1-43*01, which occurred 945 times (10% of the total sequence). Selection scores of −0.536 and −0.795 were obtained in complementarity-determining region (CDR) and FWR, respectively, suggesting high variability of the library at this stage. At pan 3, analysis of the IGHV5S13 aligned clones demonstrated ΣCDR and ΣFWR of 0.08 and −1.357, respectively (Supplementary Table 1). Thus, at pan 3 the FWR regions show strong evidence of clonality, while there is still variability in the CDRs. This interesting observation suggests a high proportion of the library at pan 3 contains related clones that may represent stages of clonal evolution.
Next, we assessed CDR length distribution within the families. At pan 0 a broad distribution of CDR lengths is observed, and by pan 3 the frequency of clones containing 12 amino acid-length HCDR3 sequences was increased from 18.4% to 97.6% (). The consensus amino acid sequence at these positions clearly showed bias towards a prominent HCDR3, which was already prevalent within antibodies from the same germline before selection ().
Understanding the prevalence of heavy and light chain pairing during phage display selection
Analysis of heavy and light chain pairing during library generation and selection is not routinely carried out due to sequence length limitations in many NGS platforms. Here we have characterized heavy and light chain pairs through rounds of panning.
We first investigated the pairing of heavy and light chains and visualized the overall frequency with circular correlation analysis (). As expected, the pairing between heavy and light chains prior to selection was stochastic. The highest heavy-light chain pairing was the combination of IGHV1-43-IGHJ3 with IGKV3S10-IGKJ5, which made up 0.36% of the unpanned library. Unique heavy and light chain combinations constituted 67.74% of the unpanned library. Conversely, at pan 3, 19.5% of the library consisted of clones combining IGHV5S13-IGHJ2 IGKV4S9-IGKJ2 segments and only 16% were unique pairing combinations. The highest frequency heavy and light chains in the library at each round were further represented as a heat map (Supplementary Figure 1). This representation showed that the highly ranked heavy chains paired with many light chains in the unpanned library, but the top-heavy chains only linked to a few specific light chains in pan 2 and 3, highlighting the advantage of sequencing the entire scFv to obtain the correct pairing.
Figure 2. Heavy and light chain pairing in phage libraries
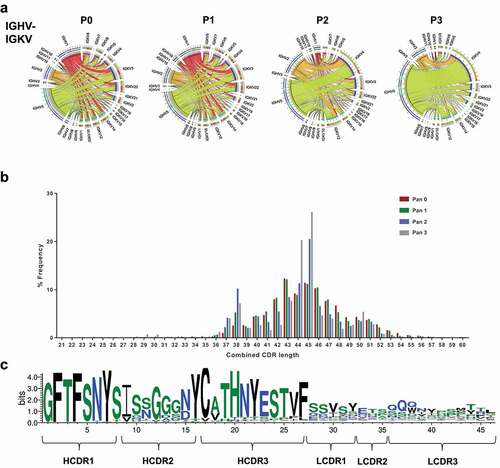
Somatic mutation in library sequences
We sought to examine somatic mutations across the entire scFv by analyzing the sequence across the six CDRs. The combined CDR length distribution showed an increase in antibodies with a peak combined CDR length of 46 amino acids as the selection progressed (). The sequence logo analysis for the antibodies with the same combined length highlighted a canonical CDR structure for the highest represented IGHV-IGHK pairing. The same HCDR3 sequence observed in the heavy chain only analysis demonstrated high diversity across the CDRs in the light chain in addition to diversity in HCDR2 (). We further looked at the variability in amino acid sequence across the six CDRs in the top 10 most abundant heavy and light chains families observed in the library after selection (Supplementary Figure 2). Variability was observed primarily in HCDR2, suggesting the selection had enriched clones from the same germline with somatic mutations.
Identification, generation, and biophysical characterization of clones based on NGS data analysis
We sought to determine the quality and diversity of CD160-binding antibodies obtained through NGS analysis. We devised a simple workflow for the identification and testing of scFv antibodies (). Initially, to identify binders, we based our analysis on the frequency of heavy chains and further searched for unrelated clones based on unique IGHV usage. The strategy was applied to data from pan 3 of the CD160 library. Here, we sorted for antibody clonotypes, defined as clones with identical HCDR3 sequence. Within each clonotype family several variants existed with substitutions in HCDRs 1 and 2. A single clone within each clonotype family was selected, based on the distance of HCDRs 1 and 2 from germline, for further characterization. In previous experiments we identified 5 clonotypes through conventional phage display selection and screening of over 200 phage clones.Citation20 All clonotypes found through conventional screening methods were observed in the NGS data set.
Figure 3. Overview of strategy to generate antibodies from deep-sequenced scFv libraries
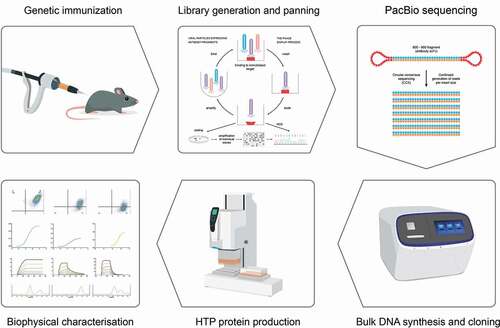
We observed that for each HCDR3 clonotype family, between 1 and 77 kappa variable (VK) clonotypes were present (). We carried out functional testing on antibodies using the top ranked IGVK clonotype by frequency for each HCDR3.
Figure 4. Identification and characterization of scFv clones
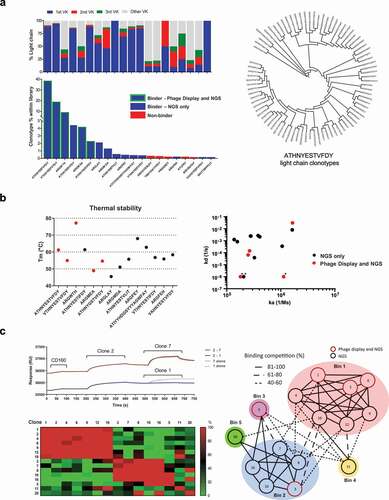
The top 20 clones were placed into scFv-Fc format and screened by flow cytometry; from these, 15 demonstrated binding to cells expressing CD160 in flow cytometry screening and 5 had previously been identified through conventional screening (). The thermal stability of the derived antibodies was next investigated using differential scanning fluorimetry (). The stability (Tm) of the scFv portion of the different antibodies ranged from 45–78oC. Of the 15 identified binders, one scFv (EHITTSPPFLIT) did not exhibit typical thermal denaturation properties despite the specific binding on CD160. Binding kinetics of the scFv antibodies to recombinant CD160 were determined by surface plasmon resonance (SPR) (Supplementary Figure 3). Antibodies with binding kinetics in the pM to nM range were obtained, with a broad range of kinetic profiles summarized in . Clone 1, 3 and 12 had unmeasurable off rates in this experiment and were artificially limited to 1 × 10–6 (1/s) to enable comparison. Taken together, the clones identified solely by NGS demonstrated comparable biophysical properties to the clones derived by traditional phage display selection. The most represented 5 clones from the NGS sequence analysis were further tested in a poly-reactivity ELISA assay against an array of diverse antigens,Citation21 showing minimal non-specific interactions for one of the scFv tested (Supplementary Figure 4). The scFvs obtained were grouped into epitope bins (). The antibodies fell into one of 5 unique bins. Antibodies obtained through conventional phage screening largely clustered into one epitope bin, with two antibodies in a second and third independent bin. Antibodies obtained through NGS analysis demonstrated binding to two further unique epitopes.
Table 1. Summary of the top 20 CD160 scFv identified
Mining NGS data to obtain binders with altered biophysical properties
NGS data analysis identified several clones with identical HCDR3 sequences but containing mutations in HCDR1 and HCDR2. As per our previous analysis, we maintained a single light chain for each HCDR3 clone based on the highest frequency pair identified after PacBio sequencing. We chose clones with a greater distance from the germline sequences of HCDRs 1 and 2 for characterization. We hypothesized that clones with alternative HCDR1 and HCDR2 sequences represented diverse antibodies at various stages of affinity maturation, and thus attempted to generate a panel of antibodies with unique binding kinetics based on a conserved binding epitope. Starting from an antibody clone with high thermal stability in our top 20 clones (ATIYYHDGSYYYAGWFAY), we identified alternative sequences containing the same HCDR3 but associated with different HCDRs 1 and 2. Sequences were compared to the identified germline antibody sequence (). The scFv antibodies that were generated bound with a range of affinities, from the micromolar to nanomolar range (). Differences in affinity were mainly driven by a change in the dissociation rate of the antibody, which varied 2 Logs (1.29 × 10−3 to 2.82 × 10–1). Amongst the modified scFv antibodies, clone 40880 lost the capability to bind, the substitution of G for W in CDR1 is clearly not accepted in the binding interface and was the furthest from germline sequence. It is tempting to speculate that this might represent an unproductive step in the clonal evolution of this antibody, but the possibility of sequencing errors cannot be discounted. Importantly, all of the antibodies generated maintained high thermal stability, and, as expected, competed for the same epitope on the protein ().
Figure 5. Identification of alternative binders in the NGS data set
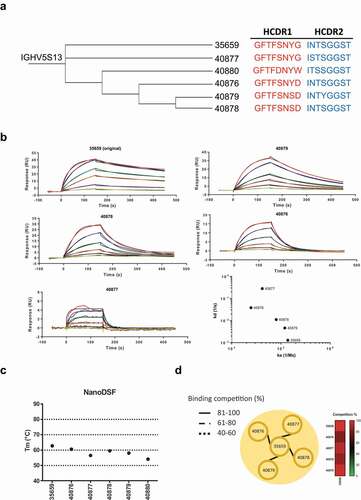
Identification of alternative binding in highly clonal libraries
We applied PacBio sequencing technology to a second library generated from rats immunized with the target antigen CD123. CD123 is a cell surface receptor that transmits signal from the cytokine interleukin 3 and has been identified as a possible target in the treatment of acute myeloid leukemia.Citation22,Citation23 The library was chosen for analysis because the generation of antibodies using standard clone selection and expression had yielded few unique hits. Of 200 screened clones, only 6 unique clones that showed specificity toward CD123-positive SupT1s by flow cytometry were identified. We analyzed the clonal distribution of the CD123 library after three rounds of selection. A total of 5193 IGH and 1361 IGK clonotypes were identified. IGHV1 and IGHV5 were observed to be the most abundant v-genes, with pairing to IGHJ3 and IGHJ2 predominantly favored. Again, V gene utilization for IGK was more diverse, with IGKV4 (20%), IGKV22 (13%), IGKV3 (11%), IGKV10 (11%), IGKV12 (9%), IGKV14 (8%), IGKV8 (7%), IGKV6 (6%) and IGKV2 (4%) all present within the library. IGKJ1 (44%) was the most abundant, closely followed by IGKJ5 (36%) and IGKJ2 (20%) (Supplementary Figure 5a). Analysis of IGHV-IGKV pairing showed that IGHV1 appeared to favor pairing with IGKV12 (60%), while IGHV5 favored pairing with IGKV4 (50%) following enrichment.
In order to select antibodies for synthesis and testing, candidate HCDR3 sequences were again chosen based on library abundance. In this instance, however, closely related sequences were avoided to increase the diversity of selected candidates (Supplementary Figure 5b). A total of 37 IGH clonotypes were selected. Each heavy chain clonotype was also expressed with three candidate IGK sequences, excluding the clones derived from phage display manual selection. Clones were transiently expressed and tested for binding to cells expressing CD123. Flow cytometry staining showed CD123-specific binding with 30 of 37 selected clonotypes (Supplementary Figure 5c). For 12 of the 24 NGS-selected clonotypes, we observed that all 3 selected IGVK chains were functional in the context of the scFv, in 5 cases 2 IGVK chains were functional and in the remaining 7 a single functional IGVK chain was observed. In 16 of the 24 cases, we observed that the most prevalent VK chain generated a functional scFv, further supporting the strategy previously used of selecting the highest represented light chain within the heavy chain clonotypes.
Discussion
NGS technologies have been used in combination with phage display in a number of applications.Citation24,Citation25 While NGS allows in-depth analysis of phage display libraries, its widespread use has been hampered by limitations in read lengthCitation26,Citation27 and signal integrityCitation16 of several sequencing technologies. These limitations have meant that most sequencing applications have only covered a single variable domain within a library, showing the greatest utility in the sequencing of single domain antibody libraries.Citation28,Citation29 Yang and colleagues recently described the use of phage display data obtained using MiSeq sequencing to isolate antibodies from scFv libraries that had undergone multiple rounds of panning. The data sets obtained had high sequence depth and allowed generation of antibodies against the target antigen of interest; however, a key limitation to the method was the use of limited read length within the sequencing data that required additional PCR amplification to retrieve the full sequence of the clones.Citation30 Here, we used the PacBio sequencing platform combined with SMRTbell technology to generate highly accurate full-length scFv sequence repertoires from immune libraries selected through conventional phage display biopanning targeting the cell surface antigens CD160 and CD123. Mining these two independent libraries enabled the identification of a large and diverse panel of high-quality antibodies.
Hemadou et al. demonstrated that the combination of SMRT sequencing with circular consensus adapter ligation allowed the identification of full-length scFvs from an enriched phage display library. By tracking a manually selected clone, they identified its presence in the top 100 of the pool of reads and they were able to determine an accuracy of >99.9% for complete sequences of inserts approaching 1,000 bp. Although this supports the robustness of the combinatorial method, it does not give insights on the functionality and diversity of the scFvs contained in the phage library.Citation13 Similarly, Han and colleagues used this approach to investigate phage display libraries that had shown high clonality using traditional screening methods and, although they identified several new clones, they did not investigate the specificity and functionality of the phage display-selected antibodies.Citation14
In our study, we used long-read sequencing to expand the screening of selected phage display libraries. This combination not only provides additional knowledge on the antibody repertoire of immunized animals but greatly enhances the screening power of immune phage display libraries for the identification of functional and diverse scFv fragments. As 5% of the immunoglobulin repertoire in rodents expresses the lambda light chain, we have considered the kappa chain locus only.Citation31 We analyzed data from two immune libraries against CD160 and CD123 based on HCDR3 abundance within the enriched libraries, and selected clones either based on frequency or phylogenetic distance to allow the isolation of more diverse binders. An interesting observation was that not all clones enriched by phage display panning bound to target antigen, but we observed a success rate of 75% and 81% when we analyzed the most frequent clones prevalent after CD160 and CD123 selection, respectively. This strategy consists of conventional phage display biopanning followed by SMRT sequencing and open source NGS analysis to identify an expansive repertoire of antigen-specific antibody clones, with virtually no screening required.
A disadvantage of the system deployed here, relative to second-generation systems such as the Illumina platform, is the far lower sequencing depth obtained. Typically yields of 80,000 reads for a 1 Kb sequence fragment can be generated, as compared to over 5 × 106 reads for Illumina Hiseq sequencing. Clearly, the read depths obtained in our experiments were insufficient to cover the theoretical diversity of a typical phage display library. It is possible to modify the sequencing protocol by sequencing the same prepared library on multiple SMRT cells of the sequencer. As library preparation and instrument run time are a substantial proportion of the sequencing cost, this approach could enable sequencing depth to be increased 10-fold with a modest increase in sequencing costs. For the purpose of this study and using an approach in which libraries are first enriched against target antigen, we surmise that depth of sequencing obtained in a single run is sufficient to sample our immune scFv phage display libraries, which typically have reduced diversity and clonal redundancy. This is clearly the case for libraries after multiple rounds of enrichment. However, for libraries on which limited enrichment have been performed or where a broader view of an immune repertoire is desired, the depth of sequencing may become limiting.
Increasingly, antibody discovery has become a high throughput empiric endeavor. Antibodies with desired properties, such as receptor agonism or the appropriate binding kinetics, within a population may be rare. Thus, it is often necessary to sample highly diverse populations in order to obtain the antibody of interest. Our library sequencing approach allows the isolation of large numbers of antibodies that have been affinity matured in-vivo and are thus likely to retain excellent specificity and biophysical properties.Citation32
Single cell screening technologies such as SLAMCitation33 and microfluidic-basedCitation34 platforms similarly offer an excellent source of in-vivo matured antibodies, but themselves suffer limitations. In B cell binding strategies, screening of the antibody repertoire often requires live cells to be manipulated and sorted for antigen binding, limiting the antibody repertoire to memory B cells that express surface IgG. Microfluidic-based platforms allow the real-time interrogation of plasma cells that secrete IgG, but these technologies are technically complex and require specialized instrumentation. The strategy that we describe is relatively low cost, simple and easily adoptable by most labs while offering a depth and diversity of antigen specific antibodies similar to single cell methods. Single B cell methods have a clear advantage in the native pairing of heavy and light chains, but they are limited to a small numbers of antigen-specific B cell that can be efficiently sorted and recovered before the sequence identification.Citation15,Citation35
Here we have described a simple method using SMRT sequencing to interrogate diversity from enriched phage display libraries to identify antigen-specific scFvs. The interrogation of the full-length sequences of each clone provides the advantageous information of functionally paired VH-VL selected during the phage display biopanning rounds. A logical next step for this technology will be to incorporate techniques such as single cell emulsion PCRCitation36 at the library generation stage. Combining these methods will enable the generation and full screening of natively paired heavy and light chain libraries, thus offering all the advantages of phage display and B-cell screening methods.
Materials and methods
Library construction and bio-panning
Three Wistar rats were immunized using genetic vaccinations with DNA encoding for CD160 and CD123, the DNA plasmids of interest were coated on gold nanoparticles and administered intramuscularly using a GeneGun™ (Biorad). Vaccinations were carried out at Aldevron, GmBH, Freiburg. Following vaccination, total RNA was extracted from the spleen of animals using an RNAeasy™ (QIAGEN) extraction kit. RNA obtained from multiple animals was reverse transcribed to cDNA, which was used to generate phage display libraries cloned into the phagemid vector pHEN1.Citation37 Libraries were generated as previously described with a size of 1 × 106 and 1 × 108 for CD160 and CD123, respectively.Citation20 Bio panning was carried out on Strep-Tactin® (IBA) magnetic beads using in-house generated proteins that incorporated a streptagII tag for direct capture from transfected HEK293T cells. After three rounds of biopanning, antigen binding tests from individual bacterial colonies were performed as previously described.Citation20
PacBio SMRTbell™ library assembly
DNA from various libraries was amplified using primers specific for the phagemid vector pHEN1 backbone (M13 Rev caggaaacagctatgac and M13 Fd3 gtcgtctttccagacgttagt).
PCR amplification was carried out on 1 ug of plasmid DNA using 20 cycles of amplification to reduce the risk of PCR bias. PCR products were purified using a Mini amp Kit (QIAGEN) according to the manufacturer’s instructions. The amplified DNA libraries were prepared following the PacBio guidelines and sequenced on an SMRT cell using Pacific Biosciences RS sequencing technology (Pacific Biosciences) at GATC GMBH (GATC). Input DNA quality and concentrations were measured using a Qubit Fluorometer dsDNA Broad Range assay (Life Technologies, p/n 32850). The SMRT bell was produced using the DNA Template Prep Kit 1.0 (Pacific Biosciences; p/n 100-259-100). A Bioanalyzer 2100 12 K DNA Chip assay (Agilent Technologies, p/n 5067-1508) was used to assess the fragment size distribution. A blunt end ligation reaction followed by exonuclease treatment was performed to create the SMRTbell template.
NGS analysis, ranking and clone selection
The selected libraries were quality inspected and quantified on the Agilent Bioanalyzer. Ready-to-sequence SMRTbell-polymerase Complex was created using the P6 DNA/Polymerase binding kit 2.0 (Pacific Biosciences, p/n 100-236-500) according to the manufacturer’s instructions. The Pacific Biosciences RS2 instrument was programed to load and sequence the sample on a single SMRT cell v3.0 (Pacific Biosciences, p/n100-171-800), taking one movie of 120 min. The MagBead loading method (Pacific Biosciences, p/n 100–133-600) was chosen in order to improve enrichment of the longer fragments. At the end of the run, a sequencing report was generated for every cell, via the SMRT portal. Thereby, the adapter dimer contamination, the sample loading efficiency, the obtained average read length and the number of filtered sub-reads were assessed.
ScFv libraries were sequenced by PacBio sequencing. A total of 15395, 10044, 26802 and 19499 reads were generated from biopanning rounds 0, 1, 2 and 3, respectively. Total reads were filtered to contain between 900 and 1200 bp fragments that include phage PelB leader sequence and myc tag to obtain full-length sequences. These were then processed using one of two methods: sequence was either analyzed using IMGT/HighV-QUESTCitation17 or by a custom pipeline to obtain in frame paired antibody heavy and light chains. The resulting functional reads represented 61.3%, 93.0%, 84.5% and 73.7%, for each of the biopanning round dataset.
In the custom pipeline, the linker sequence was used to identify binders, long read sequences were first split into VH and VL sequences and each were annotated using a standalone version of IgBlastn.Citation38 The rat immunoglobin germline gene sequences from the international ImMunoGeneTics (IMGT) database (http://www.imgt.org) were used for numbering and annotation. Each VL sequence was annotated by IGK genes, and the final light annotation was designated as that with the higher identity to the corresponding V gene. The alignments from IgBLAST were processed and summarized by Change-O,Citation39 which provides a tabular output for all CDR elements to enable ranking.
Sequences were further processed with ClustalOmega-1.2.2 using a full distance matrix and the Kimmura correction. The intermittent tree files were visualized using Interactive Tree Of Life (iTOL) v3 (https://itol.embl.de/about.cgi).Citation40
Bayesian analysis of immunoglobulin sequences was carried out using Baseline v1.3 (http://selection.med.yale.edu/baseline). A focused selection was used as the statistical framework. FWR/CDR boundaries were defined based on IMGT numbering.
Generation of scFv constructs
ScFv antibody sequences were generated through DNA synthesis. DNA sequences, as retrieved from the phage display library, were synthesized as gBlocks (Integrated DNA Technologies) with flanking restriction sites AgeI and BamHI. The scFv fragments were cloned into a modified version of the expression vector AbVec2.0Citation41 containing an in-frame mIgG2a Fc region.
Surface plasmon resonance
SPR experiments were performed with a Biacore T200 instrument using HBS-P+ as the running and dilution buffer (GE Healthcare, p/n BR100671). Biacore Insight Evaluation software v3.0 (GE Healthcare) was used for data processing. For determination of binding kinetics, goat anti-mouse IgG (GE Healthcare, p/n BR100838) was covalently coupled to a CM5 Sensor Chip (GE Healthcare, p/n 29149603) according to manufacturer recommendations (approximately 9000 RU). ScFvs with a murine IgG2a Fc were captured (level range 200–400 RU), and concentrations of interaction partner protein from 200 nM with 2-fold serial dilutions, were injected over the flow cell at a flow rate of 30 µl/minute. A double reference subtraction was performed using buffer alone. Kinetic rate constants were obtained by curve fitting according to a 1:1 Langmuir binding model. KD for clones 1, 3 and 12 were calculated using an artificially limited kd of 1 × 10–6 (1/s) due to instrument limitations.
Differential scanning fluorimetry
Protein stability was analyzed on a Prometheus NT.48 (NanoTemper). The emission of fluorescent radiation with the wavelengths of 330 nm and 350 nm was measured with the temperature changes from 20°C to 95°C, at a rate of increase of 1°C min−1. The fluorescence curves obtained were differentiated, and the ratio of differentials was used to determine the melting temperature of the proteins.
Cross-reactivity ELISA
The ELISA was performed in-house using a standard set of six antigens used to test antibodies cross-reactivity.Citation21 Human Insulin (Sigma, p/n I9278-5 ML), dsDNA (Sigma, p/n D4522-1 MG), ssDNA (Sigma, p/n D8899-1 MG), Cardiolipin (Sigma, p/n C0563-10 MG), LPS (Invivogen, p/n tlrl-eblps) and KLH (Sigma, p/n H8283-50 MG) were coated at 1 μg/ml in phosphate-buffered saline (PBS) on Nunc MaxiSorp plates. After 2% bovine serum albumin block step, the top 5 anti-CD160 clones in scFv-murine IgG2a Fc format were used as primary antibodies at 1 µg/ml in duplicate and compared to specific positive control antibodies for each antigen (anti-insulin Abcam, p/n ab8304; anti-dsDNA Abcam, p/n ab27156; anti-ssDNA and cross-reactive for Cardiolipin, Sigma, p/n MAB3868; anti-LPS Abcam, p/n ab35654; anti-KLH Abcam, p/n ab34607). Plates were washed 4 times in PBS 0.05% Tween20, to remove unbound antibodies, prior to incubation with secondary anti-mouse IgG-HRP antibody (Jackson Laboratories, p/n 115-005-062). Positive interaction was revealed using 1-Step Ultra TMB substrate (ThermoFisher Scientific, p/n 34028). Reaction was stopped with a solution of 1 M sulfuric acid and absorbance read at 450 nm using Multiskan plate reader (ThermoFisher Scientific).
Flow cytometry
SupT1 cells were engineered to overexpress the human CD160 or PSMA. Cloned scFv antibodies were used as the primary antibody for staining 1 × 105 antigen positive supT1 cells followed by 1 µl of anti-mouse secondary antibody (Jackson ImmunoResearch, p/n 115-116-146). Cells were acquired on a MACSQuant analyzer flow cytometer.
Epitope binning
The assay was performed with a Biacore T200 instrument using HBS-P+ as the running and dilution buffer (GE Healthcare, p/n BR100671). BIAevaluation software version 3.0 (GE Healthcare) was used for data processing. Soluble recombinant His-tagged CD160 (Sino Biological, p/n 12191-H08H) was captured on a NiNTA Series S chip at 0.5 µg/ml and then saturated with a first anti-CD160 antibody at 150 nM concentration. To determine binding competition, a second anti-CD160 antibody (150 nM) was injected over the flow cells. Percentage of binding competition for the second antibody was determined by normalization of same antibody pair to adjust for dissociation, and then compared with the Rmax obtained in the absence of the first competing antibody.
In silico analysis
3D structure of heavy and light chain variable domains was obtained via structure prediction and homology modeling on BioLuminate 3.8 (Schrodinger). Surface charge analysis was performed via the Protein Surface Analyzer tool on BioLuminate 3.8 (Schrodinger). Area for charge and hydrophobic patches was calculated for the regions encompassing the CDR loops.
Abbreviations
Ig | = | immunoglobulin |
CDR | = | complementarity-determining region |
FWR | = | framework region |
NGS | = | next-generation sequencing |
scFv | = | Single-chain variable fragment |
SMRT | = | single molecule real-time |
SPR | = | surface plasmon resonance |
Tm | = | melting temperature |
VH | = | variable heavy |
VK | = | variable kappa |
VL | = | variable light |
PBS | = | phosphate-buffered saline |
BSA | = | bovine serum albumin |
Contributions
FN constructed the libraries. SO and FN devised the sequencing strategy. FN, LS and BM prepared the samples for sequencing. FP produced and analyzed the alternative libraries. SO, FN and KK analyzed sequences. YB and XH performed the bioinformatic analysis. JS and RB produced the constructs and the proteins. AK generated thermal stability data. MF generated biophysical characterization data. SO, MP and FN wrote the manuscript.
Supplemental Material
Download Zip (23.1 MB)Acknowledgments
This work was funded by Cancer Research UK, grant 515707. MP is supported by the University College London Hospital Biomedical Research Centre funded by the UK National Institute of Health Research.
Supplementary material
Supplemental data for this article can be accessed on the publisher’s website.
Additional information
Funding
References
- Bradbury ARM, Sidhu S, Dübel S, McCafferty J. Beyond natural antibodies: the power of in vitro display technologies. Nat Biotechnol. 2011;29(3):245–12. doi:10.1038/nbt.1791.
- Pershad K, Pavlovic JD, Gräslund S, Nilsson P, Colwill K, Karatt-Vellatt A, Schofield DJ, Dyson MR, Pawson T, Kay BK, et al. Generating a panel of highly specific antibodies to 20 human SH2 domains by phage display. Protein Engineering, Design and Selection. 2010;23(4):279–88. doi:10.1093/protein/gzq003.
- Shim H. Synthetic approach to the generation of antibody diversity. BMB Rep. 2015;48(9):489–94. doi:10.5483/BMBRep.2015.48.9.120.
- Krebber A, Bornhauser S, Burmester J, Honegger A, Willuda J, Bosshard HR, Plückthun A. Reliable cloning of functional antibody variable domains from hybridomas and spleen cell repertoires employing a reengineered phage display system. . Journal of Immunological Methods. 1997;201(1):35–55. doi:10.1016/S0022-1759(96)00208-6.
- Nguyen TTH, Lee JS, Shim H. Construction of rabbit immune antibody libraries. Methods Mol Biol. 2018;1701:133–46.
- Lövgren J, Pursiheimo J-P, Pyykkö M, Salmi J, Lamminmäki U. Next generation sequencing of all variable loops of synthetic single framework scFv—Application in anti-HDL antibody selections. N Biotechnol. 2016;33(6):790–96. doi:10.1016/j.nbt.2016.07.009.
- Waltari E, Jia M, Jiang CS, Lu H, Huang J, Fernandez C, Finzi A, Kaufmann DE, Markowitz M, Tsuji M, et al. 5′ rapid amplification of cDNA ends and illumina MiSeq reveals B cell receptor features in healthy adults, adults with chronic HIV-1 infection, cord blood, and humanized mice. Front Immunol. 2018;9. doi:10.3389/fimmu.2018.00628
- Miho E, Roškar R, Greiff V, Reddy ST. Large-scale network analysis reveals the sequence space architecture of antibody repertoires. Nat Commun. 2019;10(1):1321. doi:10.1038/s41467-019-09278-8.
- Teng JLL, Yeung ML, Chan E, Jia L, Lin CH, Huang Y, Tse H, Wong SSY, Sham PC, Lau SKP, et al. PacBio but not illumina technology can achieve fast, accurate and complete closure of the high GC, complex burkholderia pseudomallei two-chromosome genome. Front Microbiol. 2017;8:1448. doi:10.3389/fmicb.2017.01448.
- Eid J, Fehr A, Gray J, Luong K, Lyle J, Otto G, Peluso P, Rank D, Baybayan P, Bettman B, et al. Real-time DNA sequencing from single polymerase molecules. Science. 2009;323(5910):133–38. doi:10.1126/science.1162986.
- Travers KJ, Chin C-S, Rank DR, Eid JS, Turner SW. A flexible and efficient template format for circular consensus sequencing and SNP detection. Nucleic Acids Res. 2010;38(15):e159–e159. doi:10.1093/nar/gkq543.
- Rhoads A, Au KF. PacBio sequencing and its applications. Genomics Proteomics Bioinformatics. 2015;13(5):278–89. doi:10.1016/j.gpb.2015.08.002.
- Hemadou A, Giudicelli V, Smith ML, Lefranc M-P, Duroux P, Kossida S, Heiner C, Hepler NL, Kuijpers J, Groppi A, et al. Pacific biosciences sequencing and IMGT/HighV-QUEST analysis of full-length single chain fragment variable from an in vivo selected phage-display combinatorial library. Frontiers in Immunology. 2017;8. doi:10.3389/fimmu.2017.01796
- Han SY, Antoine A, Howard D, Chang B, Chang WS, Slein M, Deikus G, Kossida S, Duroux P, Lefranc M-P, et al. Coupling of single molecule, long read sequencing with IMGT/HighV-QUEST analysis expedites identification of SIV gp140-specific antibodies from scFv phage display libraries. Front Immunol. 2018;9. doi:10.3389/fimmu.2018.00329
- Lei L, Tran K, Wang Y, Steinhardt JJ, Xiao Y, Chiang C-I, Wyatt RT, Li Y. Antigen-specific single B cell sorting and monoclonal antibody cloning in guinea pigs. . Frontiers in Microbiology. 2019;10. doi:10.3389/fmicb.2019.00672.
- Waltari E, McGeever A, Friedland N, Kim PS, McCutcheon KM. Functional enrichment and analysis of antigen-specific memory B cell antibody repertoires in PBMCs. Frontiers in Immunology. 2019;10. doi:10.3389/fimmu.2019.01452.
- Brochet X, Lefranc M-P, Giudicelli V. IMGT/V-QUEST: the highly customized and integrated system for IG and TR standardized V-J and V-D-J sequence analysis. Nucleic Acids Res. 2008;36(Web Server):W503–508. doi:10.1093/nar/gkn316.
- Yaari G, Uduman M, Kleinstein SH. Quantifying selection in high-throughput Immunoglobulin sequencing data sets. Nucleic Acids Res. 2012;40(17):e134. doi:10.1093/nar/gks457.
- Uduman M, Yaari G, Hershberg U, Stern JA, Shlomchik MJ, Kleinstein SH. Detecting selection in immunoglobulin sequences. Nucleic Acids Res. 2011;39(suppl):W499–504. doi:10.1093/nar/gkr413.
- Nannini F, Parekh F, Wawrzyniecka P, Mekkaoui L, Righi M, Dastjerdi FV, Yeung J, Roddie C, Bai Y, Ma B, et al. A primer set for the rapid isolation of scFv fragments against cell surface antigens from immunised rats. Sci Rep. 2020;10(1):19168. doi:10.1038/s41598-020-76069-3.
- Jain T, Sun T, Durand S, Hall A, Houston NR, Nett JH, Sharkey B, Bobrowicz B, Caffry I, Yu Y, et al. Biophysical properties of the clinical-stage antibody landscape. PNAS. 2017;114(5):944–49. doi:10.1073/pnas.1616408114.
- Tettamanti S, Marin V, Pizzitola I, Magnani CF, Giordano Attianese GMP, Cribioli E, Maltese F, Galimberti S, Lopez AF, Biondi A, et al. Targeting of acute myeloid leukaemia by cytokine-induced killer cells redirected with a novel CD123-specific chimeric antigen receptor. Br J Haematol. 2013;161(3):389–401. doi:10.1111/bjh.12282.
- Arcangeli S, Rotiroti MC, Bardelli M, Simonelli L, Magnani CF, Biondi A, Biagi E, Tettamanti S, Varani L. Balance of anti-CD123 chimeric antigen receptor binding affinity and density for the targeting of acute myeloid leukemia. Molecular Therapy. 2017;25(8):1933–45. doi:10.1016/j.ymthe.2017.04.017.
- Hu D, Hu S, Wan W, Xu M, Du R, Zhao W, Gao X, Liu J, Liu H, Hong J, et al. Effective optimization of antibody affinity by phage display integrated with high-throughput DNA synthesis and sequencing technologies. PloS One. 2015;10(6):e0129125. doi:10.1371/journal.pone.0129125.
- Turner KB, Naciri J, Liu JL, Anderson GP, Goldman ER, Zabetakis D. Next-generation sequencing of a single domain antibody repertoire reveals quality of phage display selected candidates. PLoS One. 2016;11(2):e0149393. doi:10.1371/journal.pone.0149393.
- Matochko WL, Chu K, Jin B, Lee SW, Whitesides GM, Derda R. Deep sequencing analysis of phage libraries using Illumina platform. Methods. 2012;58(1):47–55. doi:10.1016/j.ymeth.2012.07.006.
- Ito Y. 網羅的配列解析による多様な候補抗体の迅速同定手法の開発. Yakugaku Zasshi. 2017;137(7):823–30. doi:10.1248/yakushi.16-00252-2.
- Miyazaki N, Kiyose N, Akazawa Y, Takashima M, Hagihara Y, Inoue N, Matsuda T, Ogawa R, Inoue S, Ito Y, et al. Isolation and characterization of antigen-specific alpaca (Lama pacos) VHH antibodies by biopanning followed by high-throughput sequencing. J Biochem. 2015;158(3):205–15. doi:10.1093/jb/mvv038.
- Deschaght P, Vintém AP, Logghe M, Conde M, Felix D, Mensink R, Gonçalves J, Audiens J, Bruynooghe Y, Figueiredo R, et al. Large diversity of functional nanobodies from a camelid immune library revealed by an alternative analysis of next-generation sequencing data. Front Immunol. 2017;8. doi:10.3389/fimmu.2017.00420
- Yang W, Yoon A, Lee S, Kim S, Han J, Chung J. Next-generation sequencing enables the discovery of more diverse positive clones from a phage-displayed antibody library. Exp Mol Med. 2017;49(3):e308. doi:10.1038/emm.2017.22.
- Haughton G, Lanier LL, Babcock GF. The murine kappa light chain shift. Nature. 1978;275(5676):154–57. doi:10.1038/275154a0.
- Ledsgaard L, Kilstrup M, Karatt-Vellatt A, McCafferty J, Laustsen AH. Basics of antibody phage display technology. Toxins (Basel). 2018;10(6):236. doi:10.3390/toxins10060236.
- Lightwood DJ, Carrington B, Henry AJ, McKnight AJ, Crook K, Cromie K, Lawson ADG. Antibody generation through B cell panning on antigen followed by in situ culture and direct RT-PCR on cells harvested en masse from antigen-positive wells. . Journal of Immunological Methods. 2006;316(1–2):133–43. doi:10.1016/j.jim.2006.08.010.
- Mocciaro A, Roth TL, Bennett HM, Soumillon M, Shah A, Hiatt J, Chapman K, Marson A, Lavieu G. Light-activated cell identification and sorting (LACIS) for selection of edited clones on a nanofluidic device. Commun Biol. 2018;1(1):41. doi:10.1038/s42003-018-0034-6.
- Winters A, McFadden K, Bergen J, Landas J, Berry KA, Gonzalez A, Salimi-Moosavi H, Murawsky CM, Tagari P, King CT, et al. Rapid single B cell antibody discovery using nanopens and structured light. MAbs. 2019;11(6):1025–35. doi:10.1080/19420862.2019.1624126.
- DeKosky BJ, Kojima T, Rodin A, Charab W, Ippolito GC, Ellington AD, Georgiou G. In-depth determination and analysis of the human paired heavy- and light-chain antibody repertoire. . Nature Medicine. 2015;21(1):86–91. doi:10.1038/nm.3743.
- Hoogenboom HR, Griffiths AD, Johnson KS, Chiswell DJ, Hudson P, Winter G. Multi-subunit proteins on the surface of filamentous phage: methodologies for displaying antibody (Fab) heavy and light chains. Nucleic Acids Res. 1991;19(15):4133. doi:10.1093/nar/19.15.4133.
- Ye J, Ma N, Madden TL, Ostell JM. IgBLAST: an immunoglobulin variable domain sequence analysis tool. Nucleic Acids Res. 2013;41(W1):W34–40. doi:10.1093/nar/gkt382.
- Gupta NT, Vander Heiden JA, Uduman M, Gadala-Maria D, Yaari G, Kleinstein SH. Change-O: a toolkit for analyzing large-scale B cell immunoglobulin repertoire sequencing data: Table 1. Bioinformatics. 2015;31(20):3356–58. doi:10.1093/bioinformatics/btv359.
- Letunic I, Bork P. Interactive tree of life (iTOL) v3: an online tool for the display and annotation of phylogenetic and other trees. Nucleic Acids Res. 2016;44(W1):W242–245. doi:10.1093/nar/gkw290.
- Tiller T, Meffre E, Yurasov S, Tsuiji M, Nussenzweig MC, Wardemann H. Efficient generation of monoclonal antibodies from single human B cells by single cell RT-PCR and expression vector cloning. J Immunol Methods. 2008;329(1–2):112–24. doi:10.1016/j.jim.2007.09.017.