ABSTRACT
Good pharmacokinetic (PK) behavior is a key prerequisite for sufficient efficacy of therapeutic monoclonal antibodies (mAbs). Fc glycosylation is a critical quality attribute (CQA) of mAbs, due to its impact on stability and effector functions. However, the effects of various IgG Fc glycoforms on antibody PK remain unclear. We used a combination of glycoengineering and glycoform-resolved PK measurements by mass spectrometry (MS) to assess glycoform effects on PK. Four differently glycoengineered mAbs, each still containing multiple glycoforms, were separately injected into rats. Rat models have been shown to be predictive of human PK. At different time points, blood was taken, from which the mAbs were purified and analyzed with a liquid chromatography-MS-based bottom-up glycoproteomics approach. This allowed us to follow changes in the glycosylation profiles of each glycoengineered mAb over time. Enzyme-linked immunosorbent assay measurements provided an absolute concentration in the form of a sum value for all glycoforms. Information from both readouts was then combined to calculate PK parameters per glycoform. Thereby, multiple glycoform kinetics were resolved within one mAb preparation. We confirmed increased clearance of high-mannose (Man5) and hybrid-type (Man5G0) glycoforms. Specifically, Man5 showed a 1.8 to 2.6-fold higher clearance than agalactosylated, complex glycans (G0F). Unexpectedly, clearance was even higher (4.7-fold) for the hybrid-type glycan Man5G0. In contrast, clearance of agalactosylated, monoantennary glycoforms (G0F-N) was only slightly increased over G0F (1.2 to 1.4-fold). Thus, monoantennary, hybrid-type and high-mannose glycoforms should be distinguished in CQA assessments. Strikingly, α2,3-linked sialylation did not affect clearance, contradicting the involvement of the asialoglycoprotein receptor in mAb clearance.
Introduction
Desirable pharmacokinetic (PK) behavior is one of the key features that determines the success of a drug candidate. With respect to biopharmaceuticals, their biological activity is critically dependent on exposure.1,Citation2 Slow clearance/long half-life reduces the necessary dose, decreases dosing intervals, and/or may enable subcutaneous administration.Citation3 These factors in turn reduce costs and may increase compliance.Citation3 Less frequent dosing also reduces patient discomfort, as biopharmaceuticals are often formulated as injectables.
Today, monoclonal antibodies (mAbs) are widely used in therapeutic agents.Citation4 They have revolutionized the treatment of cancer and autoimmune diseases in the past decades and continue to show great promise in these areas, as exemplified by the 2018 Nobel Prize in Physiology or Medicine.Citation5 In contrast to their importance, the biotransformation of mAbs is generally not well understood. Especially, the changes in post-translational modifications induced by metabolism and the relationship between post-translational modifications and clearance have not been extensively investigated.
mAbs are mainly cleared from the body via lysosomal degradation in endothelial cells and hematopoietic cells.Citation6 This happens either as part of the homeostatic protein turnover or in relation to immunological pathways.Citation1 After nonspecific pinocytosis, immunoglobulin G (IgG) is transported to endosomes.Citation3 A main factor influencing this clearance pathway of IgG-based mAbs is binding to the neonatal Fc receptor (FcRn) and consequent scavenging of IgG.Citation7 FcRn is responsible for the exceptionally long half-life of IgG. Other molecular interactions, mainly with lectins, are thought to increase the clearance of mAbs.Citation8 Mannose receptorCitation9,Citation10 and asialoglycoprotein receptor (also known as Ashwell-Morell receptor) have been implicated in this context.Citation8 In addition, anti-drug antibodies and target-mediated drug disposition may also contribute to an increase in clearance of mAbs.Citation11,Citation12
Glycosylation is a complex co- and post-translational modification and a critical quality attribute for mAbs.Citation13 It is critical to control glycosylation during the product life cycle and in development of biosimilars. Additionally, modulation of glycosylation has also led to glycoengineered mAbs with enhanced antibody-dependent cell-mediated cytotoxicity, such as obinutuzumab.Citation14 Many studies have investigated the influence of mAb glycosylation on effector functions, such as antibody-dependent cellular cytotoxicity.Citation13,Citation15,Citation16
The impact of mAb glycosylation on their PK remains controversial. Various influences have been reported.Citation3,Citation8 However, these seem to be more pronounced in antibodies with glycosylation within the antigen-binding fragment (Fab) and IgG fusion proteins where the non-Fc glycans dominate glycan-dependent PK effects.Citation17,Citation18 provides an overview of previously reported glycan effects in PK studies focusing on mAbs with only Fc glycosylation.
Table 1. Known and novel effects of Fc glycans on the pharmacokinetics of mAbs
Studies in which no effect of Fc-glycosylation was observed have been reported, but one difference now seems to be well established: High mannose leads to faster clearance compared to complex Fc-glycoforms.Citation3,Citation21,Citation23 Also, hybrid-type glycans, namely a mixture of Man5G0 and Man5G1, have been implicated in faster clearance.Citation19 In this report, we maintain a strict distinction between hybrid-type glycans (Man5G0), containing more than three mannoses, and monoantennary glycans (G0-N, G0F-N, and G1FS-N), containing only the three core mannoses ().
Figure 1. Monoantennary and hybrid-type glycans should be carefully distinguished, as they have a strongly differential impact on pharmacokinetics. Mono- and diantennary glycoforms, containing only core mannoses, have relatively low clearance. Hybrid (Man5G0) and high mannose (Man5) type glycoforms which both contain additional outer mannoses show high clearance. The further decoration of the glycoforms, meaning the presence or absence of fucoses, galactoses and N-acetylneuraminic acid was found to be of only minor influence. Low clearance is often a desirable pharmacokinetic trait, as it reduces amount and interval of dosing
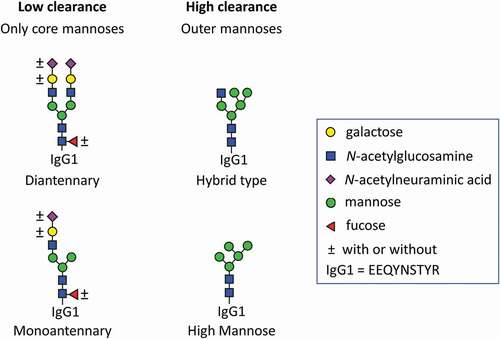
Sialylation is known to decrease clearance in other biopharmaceuticals, such as erythropoietin.Citation25 Furthermore, terminal N-acetylglucosamine residues have been implicated in increased clearance of Fab-glycosylated antibodies and IgG fusion-proteins.Citation17,Citation26 However, neither sialylation nor terminal N-acetylglucosamine was investigated in mAbs that only exhibit glycosylation in the Fc.
Differential FcRn binding could potentially explain clearance differences between glycoforms. Although oxidation at Met255 is reported to dominate FcRn binding with respect to the effects of post-translational modifications, small differences in FcRn binding have been reported for different glycoforms.Citation27–29
Clearance mediated by naturally-occurring lectins on the surface of mammalian cells could provide another potential explanation for why specific glycoforms are preferentially cleared. The mannose receptor is a C-type lectin expressed on subsets of myeloid and endothelial cells.Citation9 It has been demonstrated to cause internalization of mannosylated fusion proteins in mice into lysosomes of hepatic endothelial cells and splenic macrophages.Citation10 This has a strong effect on clearance of mannosylated mAbs and fusion proteins, as demonstrated by prolonged high plasma concentrations upon parallel mannose receptor inhibition.Citation10,Citation30
Immunogenic glycoforms, such as N-glycolylneuraminic acid or α1,3-linked galactose, may increase the immunogenicity risk, and consequently lead to hypersensitivity reactions.Citation13 Since mannose receptor is also involved in antigen presentation, the chance for anti-drug antibodies might be greater when the mAb is cleared via mannose receptor.Citation9,Citation10
Target-mediated drug disposition might also be affected by glycosylation. For example, afucosylated mAbs could be cleared faster through their increased antibody-dependent cell-mediated cytotoxicity.Citation31 However, the target of the herein used mAbs is not present in rats, hence excluding an impact of target-mediated drug disposition in the study.
Lastly, enzymatic activity of glycosidases and glycosyltransferase, to which the mAb may be exposed in serum or while transiently passing through blood cells, organs, or tissues, can alter the glycoform distribution of a therapeutic mAb. This is well established for large high-mannose glycoforms (Man6 to Man9), which are converted to Man5 via the action of natural mannosidase I.Citation19,Citation21,Citation23 The presence of other glycosidases, such as sialidases and galactosidases, in human circulation and their involvement in protein turnover have been proposed as well.Citation32
Rats have been used successfully in the past to predict non-target-mediated mechanisms of antibodies in humans. For example, the PK of CD4-IgG in humans could be predicted using data obtained in animals, including rats.Citation33 Also, PK data in mice, rats, and cynomolgus monkeys were used to predict the PK of a recombinant humanized mAb against vascular endothelial growth factor in humans using allometric scaling.Citation34
In order to study the differential impact of glycoforms on functional aspects, such as PK, of mAb therapeutics, mAbs enriched in individual glycoforms are needed. The production of these mAbs is referred to as glycoengineering. It is achieved either by altering the genetic makeup of cell lines, modifying culturing conditions, synthesizing the glycan, and adding it to the antibody or by chemoenzymatic modification of existing glycoforms. With the latter approach, we have produced herein three glycoengineered antibodies, one of which has not been reported before as accessible by chemoenzymatic in-vitro synthesis (see also Methods paragraph “Glycoengineering”; and S1). A fully galactosylated and α2,3-sialylated mAb with high fucosylation had been reported previously.Citation35 We also synthesized a high-mannose type with five mannosesCitation24 and a highly fucosylated, agalactosylated monoantennary mAb, in order to assess potential differences between high-mannose type and truncated complex type glycans in PK behavior. For clarification, our glycoengineering had no effect on the fucosylation of the antibodies.
Figure 2. Pharmacokinetic study workflow. Four differentially glycosylated antibodies were injected into six rats each. Serum was taken at the indicated time-points. ELISA analysis yielded the total therapeutic antibody concentration. LC-MS after tryptic cleavage was used to generate glycosylation profiles of the Fc N-glycosylation of the mAbs. By combining both results, PK parameters could be calculated for each glycoform in each experiment
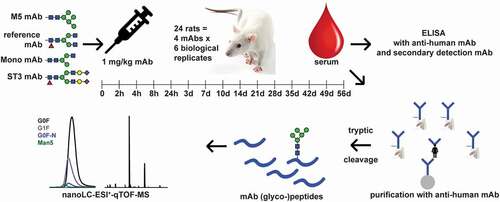
Next to approaching differential glycoform PK with glycoengineered antibodies and a classical quantitative enzyme-linked immunosorbent assay (ELISA), we also monitored clearance of mAbs in a glycoform-resolved manner by liquid chromatography-mass spectrometry (LC-MS). It allowed us to study a whole range of interesting minor glycoforms in parallel. Importantly, we were able to compare different glycoforms within one animal, giving better statistical power and consequently higher resolution for small effect sizes. With respect to classical mAbs, an MS-based glycoform-resolved PK has been performed before in mice, rabbit, and humans,Citation21,Citation23 but no glycan-resolved studies in rats have been reported. A brief comparison of our results to previous studies can be found in Table1. More importantly, glycoengineering and glycan-resolved monitoring during the PK study have not been combined before. Although MS analysis by itself allows individual glycoforms in a mAb preparation to be followed, glycoengineering increases the number of glycoforms that can be assessed. It also allows study of the same glycoform as a minor and major constituent of a mixture, highlighting potential analytical or PK effects of differential relative abundance of glycoforms.
Both ELISA and LC-MS can be operated at high sensitivities, which is essential for the low mAb concentrations encountered in the mechanistically interesting late time points of the PK curve. For the LC-MS approach, sensitivity, glycoprofiling precision, and antibody selectivity have been demonstrated previously, for example, in the glycoprofiling of antigen-specific antibodies. Measuring the mAbs against the background of natural antibodies of the model system is challenging. The glycopeptide-based approach for glycan-resolved PK monitoring greatly reduced the risk of interferences from contaminations during bioanalysis and allowed the selective monitoring of mAb glycoforms in the presence of a rat antibody background.
Results
Total antibody-based PK
Four groups, of six female Wistar rats each, were used in the PK study. Each group was injected with a single mAb preparation, which differed from each other in glycoform distribution, but shared the same primary sequence. The four mAb preparations () were neither mixed before injection nor did any animal receive more than one injection. The reference mAb showed an Fc glycosylation typical for Chinese hamster ovary cell production. The other mAbs had been glycoengineered (detailed in Figure S1) to have predominantly: 1) a high-mannose-type glycan with five mannoses (M5), 2) a fucosylated N-glycan core with a single N-acetylglucoseamine on the α1,3 arm (monoantennary; Mono), and 3) a fully galactosylated, fully α2,3-sialylated glycan (ST3) ( and Table S1 and S7). Rats were sampled at 15 time points between 5 min and 56 days ( and Table S8). The concentrations of the mAbs were followed over time by ELISA. depicts the PK values derived from these measurements. Only the M5 experiment showed a tendency to deviate from the reference in terms of area-under-the-curve (AUC), clearance (CL), and half-life (t1/2) (see also Figure S2). However, the maximum concentration (cmax) and the distribution volume (Vss) did not deviate.
Table 2. Total antibody-based PK values. Values in bold tend to show a difference with the wildtype (p < .05). However, none of the results are significant after multiple testing correction
Figure 3. Glycosylation profiles of the four mAbs used in the rat PK study. All species are depicted which appear in any mAb >1% relative intensity (0.5% cutoff for visualization). The depicted values are obtained from LC-MS analysis of standards (thus not from material captured from animal serum). Complete glycoprofiling results can be found in Table S7
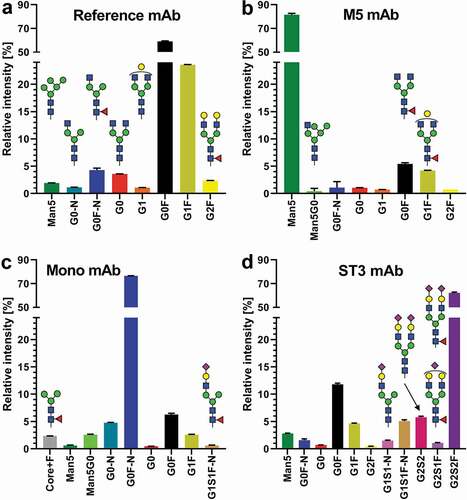
Glycoform-resolved PK
G0F is used throughout as a reference for the other glycoforms
We determined the mAb glycosylation profiles for each PK sample by LC-MS of tryptic Fc-glycopeptides (Table S8). We then combined the glycosylation profiles with the mAb concentrations determined by ELISA to obtain concentrations for each individual glycoform, which we used to calculate individual PK parameters for each glycoform. depicts the concentration changes over time for the various glycoforms within the M5 mAb samples. Man5 clearly showed a faster decrease in concentration than G0F, while G1F behaved similarly to G0F. Interestingly, the slope of the Man5 curve in was quite different from that of the total antibody curve of the M5 mAb in the late time points, despite Man5 comprising 86.6 ± 0.8% of the total glycosylation of the M5 mAb initially. The slope of the M5 mAb elimination phase is more similar to that of the G0F and G1F curves. An overview of the changes in relative concentration over time and the PK parameters organized by an individual antibody is provided in Figure S4 and Supplementary Tables S2 to S5.
Figure 4. Concentration changes over time for the major constituents present in the M5 mAb preparation. Total antibody concentration (black) as well as most abundant glycoforms (color) are depicted. The faster clearance of Man5 is visible, especially for the later time points. Means and standard errors are shown
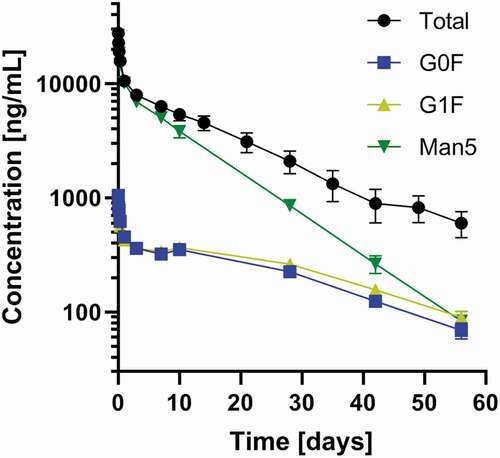
The Man5 high mannose and the Man5G0 hybrid glycan were cleared faster than the other glycoforms. When compared to G0F, clearance was strongly increased and half-life was strongly decreased for Man5, as shown in and Table S6. Relative effect sizes for Man5 were largely consistent between experiments, ranging from 1.8 to 2.6-fold change. The Man5G0 hybrid, observed in the Mono mAb, showed an extent of increased clearance (4.7-fold) similar to Man5.
Figure 5. Clearance values of individual glycoforms separately determined for each of the four mAbs. Furthermore, an indication of fold change and statistical significance of the difference compared to G0F is given. For nomenclature compare Figure S1 and Table S1. *p > .043; **p > .01; ***p > .001; ****p > .0001; 1not tested
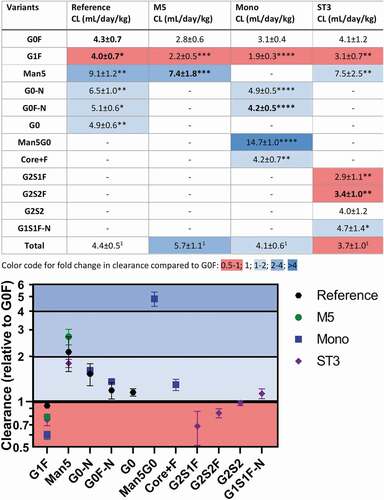
Galactosylation decreased clearance while additional sialylation did not. Relative to G0F, G1F showed a decreased clearance and increased half-life, although, with 0.6 to 0.9-fold change, the effect was smaller. With an 0.7 and 0.8-fold change, respectively, G2S1F and G2S2F behaved similarly to G1F showing decreased clearance and increased half-life.
All other findings, including afucosylation and truncation, were of increased clearance and decreased half-life. Mostly, effect sizes were significantly smaller than for Man5. Truncation of the 1,6-arm increased clearance and decreased half-life, as exemplified by G0F-N (1.2 to 1.4-fold). Afucosylation also increased clearance and decreased half-life, as observed for G0 (1.1-fold). The increased clearance due to 1,6-arm truncation and due to afucosylation seemed to be cumulative as suggested by the effect size order G0F-N≈ G0< G0-N. It must be noted that a corresponding decrease in half-life was only observed for G0F-N in the Mono mAb experiment and for G0-N (Table S6). However, these findings and consistent trends suggest an effect of 1,6-arm truncation and afucosylation also on half-life, the same as clearly demonstrated for clearance (). G2S1F-N showed slightly increased clearance (1.1-fold), but similar half-life as G0F. Hence, in comparison with G1F and G2S1F1, the effect of truncation seemed to be slightly larger than or equal to the effect of galactosylation. For G2S2, no differences in clearance or half-life to G0F were observed. The effects of galactosylation, sialylation, and afucosylation seemed to cancel each other out in G2S2. The composition H3N2F1 (core+F), which equals the fucosylated N-glycan core structure, showed an increased clearance and decreased half-life compared to G0F. Interestingly, the effect size was far more similar to G0F-N as opposed to Man5 or the Man5G0 hybrid. All these changes lead to changes in the relative glycan composition of the Ab over time (Figure S4).
Discussion
The glycoform-resolved analysis clearly demonstrated advantages over the classical approach. It allowed the pairing of results from the same individual rats, thereby increasing statistical power. Additionally, individual effects of glycans can be followed instead of having to rely on average effects. Levels of potential confounders, such as oxidation, are expected to be very similar for the different glycoforms of a mAb preparation, thereby omitting the need to monitor and correct for their impact on PK, e.g., through FcRn binding. This would allow an assessment of individual glycoforms from the reference antibody alone.
However, the glycoengineering provided additional key glycoforms at a level required for the glycoform-resolved approach. For example, it allowed the direct comparison of the monoantennary G0F-N and the hybrid-type Man5G0 species, which is crucial to the main findings of this study. Purity of the glycoengineered mAbs was very high. Thus, the N-acetylglucosaminyltransferase I-treated mAb and the α2,3-sialyltransferase-treated mAb would not have contained significant amounts of complex diantennary glycans. Therefore, the reference mAb was spiked at 10% relative concentration into these antibodies to obtain Mono and ST3 mAb prior to injection into the animals, facilitating the use of G0F for comparison across experiments when LC-MS readout was applied.
Previous publications have indicated the preferred clearance of high-mannose glycans.Citation19,Citation21,Citation23 The total antibody measurements yielded only tendencies of increased clearance, reduced half-life, and reduced AUC for the M5 experiment. Low statistical power results partially from the necessary exclusion of two animals of the reference group. These animals showed a strongly increased clearance for unknown reasons (Figure S3). An initially suspected involvement of anti-drug antibodies could be excluded. Two of the six rats given the reference mAb showed anti-drug antibodies, but not the two rats with increased clearance (data not shown). In addition, the total PK measurement is a sum of the clearance of the heterogenic glycovariants and subtle differences in clearance values may not be detectable. Fortunately, the glycoform-resolved PK calculations allowed a paired intra-individual comparison. Consequently, we could reproduce the strongly increased clearance of high-mannose-type glycoforms. A 1.8 to 2.6-fold increase was found for Man5 in the reference, M5, and ST3 mAb experiments (). Evidence has been presented linking this effect to interaction with mannose receptor.Citation30
Additionally, the Man5G0 hybrid-type glycoform led to a strongly increased clearance in the Mono mAb experiment. Though one publication also demonstrated the faster clearance of hybrid-type structures compared to complex biantennary structures, therein a smaller effect size was observed as compared to Man5.Citation19 In our study, the Man5G0 hybrid had an even higher clearance rate (4.7-fold increase) than Man5 (1.8 to 2.6-fold increase). However, the swainsonine-generated mAb used by Kanda et al. featured a mixture of Man5G0 and Man5G1 glycans.Citation19 Maybe the (opposing) effect of galactose on clearance (see below) is more pronounced in these hybrid-types than in complex glycans. In summary, it seems that additional mannoses on top of the N-glycan core mannoses strongly increase clearance, even in the context of a hybrid-type glycoform.
However, exposed core mannoses do not strongly increase clearance, as can be deduced from the behavior of the monoantennary glycans G0F-N, G0-N, and G1S1F-N, as well as the core+F structure. These exhibited only a 1.2 to 1.4-fold, 1.5 to 1.6-fold, 1.1-fold, and 1.4-fold increased clearance, respectively, significantly less than Man5 or the Man5G0 hydrid. Interestingly, the truncation effect is not enhanced by the removal of the second antennary N-acetylglucosamine in the core+F structure.
Our method proved very powerful in detecting glycosylation effects on clearance. We discuss several more minor effects below. However, due to the comparably large effects, high mannose (Man5), and hybrid-type glycoforms (Man5G0) will be the only minor glycoforms affecting the overall PK behavior of a mAb relevantly.
Like truncation, afucosylation leads to only a limited increase in clearance, as can be seen when comparing G0 to G0F (1.1-fold increase) in the reference mAb and G0-N to G0F-N (1.2 to 1.3-fold difference) in the reference and Mono mAbs. The very small clearance difference of afucosylation is unlikely to translate to a significant impact on the overall PK behavior of a regular mAb, such as the reference mAb. This means the effect will not be detectable with classical ELISA-based approaches. Thus, our findings are consistent with previous reports by Kanda et al. who did not report an effect when comparing an afucosylated (FUT8 knockout) and a fucosylated version of rituximab.Citation19 The faster clearance of afucosylated mAbs may be due to their strongly increased Fc gamma receptor IIIa affinity,Citation31 although the opsonization route required for a productive receptor involvement is unknown. Thus, an alternative explanation is that the relative contribution of Fc gamma receptor-mediated internalization and consequently clearance of afucosylated mAbs might be higher in (this specific dose of) the herein used mAb than in rituximab. The difference in model systems (rats versus mice) should also be considered.
The only glycosylation feature that we found to decrease clearance was galactosylation. G1F was consistently cleared slower than G0F in all experiments (0.9 to 0.6-fold change; 1.1 to 1.6-fold decrease), although the difference was significantly smaller than for Man5 (1.8 to 2.6-fold). Here, mannose receptor may play a role as well, since it has a significant affinity for terminal N-acetylglucosamine.Citation9 In fact, the effect has been observed for Fab glycosylation and the receptor glycans of a fusion protein, and was, in both cases, speculated to be the result of mannose receptor affinity of terminal N-acetylglucosamine.Citation17,Citation26 It is unclear why this effect was not observed in previous glycoform-resolved studies of Fc-only antibodies.Citation21–23 Probably some experimental and analysis choices may explain a lower sensitivity. Higel et al. compared each glycoform to the total antibody, instead of comparing two glycoforms directly, and used unpaired t-tests.Citation23 We followed the rats for longer and/or with a higher density of sampling than was chosen in other studies.Citation21,Citation22 All these aspects could be crucial for the detection of minor differences.
While sialylation could be expected to decrease clearance, we did not observe an effect of the α2,3-linked sialylation investigated in the ST3 mAb. G2S1F and G2S2F had the same clearance rate and neither behaved differently from G1F. Thus, their decreased clearance compared to G0F likely results from increased galactosylation. The findings with regard to sialylation discourage an involvement of the asialoglycoprotein receptor in the differential clearance of Fc-only mAbs. So far, its involvement has not been demonstrated for immunoglobulin G, mAbs, or other antibody-based formats directly. Furthermore, only low affinity toward the receptor was reported for diantennary glycans, which supports our findings.Citation36,Citation37
Noticeable differences in the calculated clearance values of the same glycoform between different experiments were observed ( and Table S6). Clearance pathways shared with the large excess of natural antibodies are unlikely to cause this variation. However, more specific, saturable pathways may explain how, for example, a large amount of Man5 glycoforms would further decrease the clearance of G0F and G1F glycoforms. Saturation might also explain why Man5 is cleared faster in the reference where it is not competing as strongly with itself as in the M5 sample.
underlines that the late elimination phase of the PK is dominated by the glycoforms with a slower clearance. Consequently, the whole PK behavior changes in the late elimination phase if there are minor glycoforms with slower clearance than the major glycoforms. Since the late elimination phase determines when the antibody concentration drops below the minimal therapeutic concentration and re-administration is necessary, special attention should probably be directed to glycoforms with relevantly slower clearance. This again stresses the importance to perform PK measurements in a glycoform-resolved way.
In our investigation, only galactosylation decreased clearance slightly compared to G0F (1.1 to 1.6-fold decrease). G1F is also a major glycan in the reference mAb, which is true for many mAbs from the most widespread production systems.Citation38 However, especially in the reference mAb, the effect on clearance was very small (1.1-fold decrease). Is the value from the reference mAb more reliable due to the higher abundance? Or is the difference from the other experiments more accurate, because G0F and G1F are paired with the same glycoform, instead of being preferentially paired with each other, thus resulting in an averaged apparent effect for both in the reference mAb? This is an important aspect that needs to be further studied in order to judge the potential impact on the overall PK behavior. Furthermore, the impact of a second galactose and/or the impact of α1,3 vs α1,6-antennary isomers will be important to conclude on the overall contribution of galactosylation.
Although FcRn has been reported to only have a small glycoform preference,Citation28,Citation29 its key importance in suppressing mAb clearance may well explain part of the differences observed. FcRn was reported to have a slightly higher affinity for galactosylated glycoforms. This is consistent with the reduced clearance of galactosylated glycoforms observed herein. Classically, the strong negative effect of Met255 oxidation on FcRn bindingCitation27 may lead to artifacts when comparing the PK of different antibodies, such as glycoengineered variants, with potentially different oxidation status. With the glycoform-resolved approach, this confounder is eliminated as PK values are compared within one batch of antibody.
Next to demonstrating the utility of our approach, we aimed at elucidating the influence of the exposed core mannose in monoantennary glycoforms. Traditionally, it has been assumed that monoantennary glycans without, and hybrid-type glycans with, outer mannoses would behave similarly to high-mannose glycans in PK. Consequently, these have often been grouped together. Interestingly, the monoantennary glycoforms showed PK behavior more similar to diantennary complex glycoforms, suggesting no strong interaction of these exposed core mannoses with clearance receptors. This is in stark contrast not only to the findings for Man5, but also the hybrid-type glycan Man5G0. The Man5G0 hybrid was also cleared 4.7-fold faster than G0F, confirming a previous report on faster clearance of hybrid-type structures.Citation19 Due to their very different impact on PK, it will be important to rigorously distinguish between monoantennary, hybrid-type, and high-mannose glycans in the characterization of mAbs. This may be especially challenging in human embryonic kidney cell-produced mAbs, since bisected hybrids and non-bisected complex glycans show compositional isomerism.
In the future, it may be interesting to further dissect the origin of differential PK of the glycoforms. For example, comparing mAbs with different levels of Fc gamma receptor affinity may make it possible to link the afucosylation effect to an involvement of this receptor or disprove this hypothesis. As discussed above, more research is necessary to judge whether galactosylation has to be viewed as a feature impacting overall antibody PK. Studies with differentially galactosylated antibodies, ideally including G0F, G1F(α1,3), G1F(α1,6), and G2F as pure glycoengineered antibodies, would likely provide the most reliable insights on this aspect. Additionally, alternative routes of administration may produce additional glycoform effects. Thus, it may be worthwhile investigating glycoform-resolved PK after subcutaneous application since there is an increasing focus on this administration route. Importantly, our approach could potentially reduce the number of animals needed to dissect glycosylation effects because multiple glycoforms of a mAb can be studied in the same animal simultaneously.
Materials and methods
Glycoengineering
Four different glycoform variants of an IgG1 mAb were prepared in house and used for the rat PK studies: 1) a variant with common Chinese hamster ovary cell glycosylation (reference mAb), 2) a variant containing mainly high-mannose glycans with five mannoses (M5 mAb), 3) a fully galactosylated and highly sialylated variant (ST3 mAb) and 4) a monoantennary glycan variant (Mono mAb); see Figure S1. The reference mAb was derived from a standard fermentation process. ST3 mAb and Mono mAb were prepared from the reference mAb, using a chemo-enzymatic approach with different glycosidases and glycosyltransferases. The M5 variant was obtained from a fermentation process with addition of kifunensine and subsequent enzymatic treatment. All enzymatic steps were followed by Protein A chromatography-based purification of the (intermediate) product. All final mAbs were sterilized with an 0.2 µm filter unit. M5 and Mono mAb were spiked with 10% reference mAb, so they would contain G0F in measurable quantities as a reference glycan for glycoform-resolved analysis by LC-MS.
To obtain the M5 mAb, 0.1 µg/mL kifunensine was added to the basal medium of the regular fermentation process. After harvest, 5.1 mg mannosidase A (in house) was added per 200 mg of antibody in 100 mM sodium acetate, 0.5 mM CaCl2, pH 5.0, and incubated at 37°C for 24 h.
For generation of the Mono mAb, the reference material was subjected to enzymatic degalactosylation of 45 mg antibody with 225 µL (0.45 U) β(1–4)-galactosidase (Prozyme, GKX-5014) for 22 hours at 37°C. Afterward, 30 mg of the gained G0F variant was further processed with 850 µl (255 U) N-acetylhexosaminidases in the recommended buffer (Prozyme, GKX-5023) for 24 h at 37°C. The resulting intermediate contained only the fucosylated N-glycan core (core+F). The final Mono mAb, containing mainly G0F-N (α1-3), was yielded by treatment with N-acetylglucosamine transferase I, N-acetylglucosaminyltransferase I (a gift from the Complex Carbohydrate Research Center, Athens, Georgia, USA) in order to specifically add N-acetylglucosamine to the α1,3-linked mannose branch. 200 µL (200 µg) N-acetylglucosaminyltransferase I were added to 30 mg of the core+F enriched antibody and incubated at 37°C for 17 hours in 25 mM MES buffer (pH 6.0), containing 10 mM MnCl2 and 4 mg/mL UDP-N-acetylglucosamine.
Enzymatic preparation of the ST3 mAb started with hyper-galactosylation of the reference mAb for 24 hours at 37°C. 400 mg IgG1 (c = 25 mg/ml) was mixed with 62.8 ml reaction buffer (10 mM UDP-Gal, 5 mM MnCl2, 100 mM MES, pH 6.5) and 2 mg β(1–4)-galactosyltransferase (Roche, cat. no. 08098182103). For the sialylation reaction, 145 mg CMP-NANA (10 mg/ml in water) and 29 mg α2,3-sialyltransferase, ST3 (Roche, cat. no. 07429916103), were added to 290 mg of the hyper-galactosylated intermediate. Furthermore, alkaline phosphatase (AP) and ZnCl2 were added to the sample (final concentration: 200 nM AP, 0.1 mM ZnCl2). The mixture was incubated for 24 hours at 37°C.
Single-dose PK study
The animal studies, described below, were performed in accordance with animal welfare laws and were approved by the Covance Harrogate ethical review committee. The reference mAb and the three glycovariants, M5, ST3, and Mono mAb, were studied in four independent experiments in a rat model with six biological replicates for each mAb. None of the mAbs is cross-reactive in rats. Female Wistar rats (Charles River, UK) received a single intravenous injection of either the reference mAb, the M5 mAb, the ST3 mAb, or the Mono mAb (); but never a mixture of multiple glycovariants, although each glycovariant does contain a mixture of glycoforms (). Antibodies were administered via a lateral tail vein, at a dose of 1 mg/kg, as a bolus. Before administration, antibodies were diluted in a solution of 5 mM histidine, 60 mM trehalose, 0.01% Tween 20 pH 6.0. Consecutive blood samples were taken from all six animals in each of the four groups at pre-dose, as well as 0.0833, 2, 4, 8, and 24 h, and days 3, 7, 10, 14, 21, 28, 35, 42, 49, and 56 post-dose, in each group. Each group consisted of six animals. Blood samples were taken from the tail vein of each animal and allowed to clot at room temperature prior to centrifuging at 3000 rpm for 10 min at 4°C. Thereafter, blood samples were stored at −20°C.
Concentration measurements
Wistar rat serum samples were analyzed with an ELISA method specific for human IgG or Fab CH1/kappa domain using a cobas e411 instrument. Briefly, test samples of M5 mAB, ST3 mAB, Mono mAb, and reference mAB, first detection antibody mAb <H-Fab(kappa)> IgG-Bi, second detection antibody mAb <H-Fab(CH1)> IgG-Ru, and Streptavidin (SA)-beads were added stepwise to a detection vessel and incubated for 9 minutes in each step. Finally, the SA-bead-bound complex was detected in a measuring cell counting the SA-beads in repeat. The counts are proportional to the analyte concentration in the test sample. The lower limit of quantitation was 14 ng/mL. The assay had a dynamic range of 14 to 30.000 ng/ml in 100% matrix.
Purification of mAbs from rat plasma and LC-MS glycopeptide analysis
The approach was similar to the analysis of human IgG from human plasma, as published previously.Citation39 10 µL rat plasma, 10 µL phosphate-buffered saline, and 5 µL CaptureSelect™ Fc (Hu) beads (agarose beads with immobilized anti-IgG antibody; ThermoFisher Scientific) were mixed, washed 3x each with 200 µL phosphate-buffered saline and water, and eluted at 500 x g for 60 s in 100 µL 100 mM formic acid (analytical grade; Sigma-Aldrich, Steinheim, Germany). After drying, samples were re-dissolved in 40 µL 25 mM ammonium bicarbonate containing 0.5 µg TCPK-treated trypsin (Sigma-Aldrich) and incubated overnight. Resulting tryptic glycopeptides were analyzed by RP-nanoLC-MS. An Acclaim PepMap 100 C18 column 150 × 0.075 mm with 3 µm particles was operated at 700 nL/min flow in an Ultimate 3000 RSLCnano LC system (ThermoFisher Scientific) and detected on a maXis™ quadrupole-time-of-flight mass spectrometer equipped with a nanoBooster™ nanoESI source (Bruker, Leiden, The Netherlands). Glycopeptides were ionized as reported elsewhere.Citation39 A binary gradient, with solvent A, consisting of 0.1% formic acid in water, and solvent B, consisting of 95% acetonitrile with 0.1% formic acid, was as applied as follows: After 5 min 3% B, linear gradient to 27% B at 20 min, followed by washing at 70% B from 21 to 23 min and re-equilibration at 3% B from 24 to 42 min. Acetonitrile was obtained as LC-MS grade (Biosolve, Valkenswaard, The Netherlands).
Data processing, non-compartmental PK analysis and statistics
Automated data (pre-)processing was done with LaCyTools version 1.0.1 build 8 similarly as previously described.Citation39,Citation40 Importantly, due to the higher resolution and mass accuracy of the quadrupole-time-of-flight mass spectrometer we used, the extraction window could be reduced to 0.065 Th (Thomson; unit describing m/z differences; defined as Dalton per elementary charge; for example, the difference between m/z 1000 and m/z 1001 is 1 thomson). Individual concentrations of each detected glycoform in each sample were created by multiplying the relative abundance obtained from the LC-MS measurements with the concentrations obtained by the ELISA measurements (furthermore referred to as glycoform-resolved). Sufficient linearity and lower limit of quantitation of this approach are exemplified in Figure S5.
The PK parameters were derived from the individual concentration data and were estimated by non-compartmental analysis, using the kinetic evaluation program Phoenix® WinNonlin (6.4 NY, USA). PK data were calculated for each rat using individual serum concentration-time data. Two rats in the wild-type group were excluded from the analysis due to the accelerated clearance observed in these animals. To determine the PK parameters of the measured glycans in serum, the dose was adjusted according to the composition of the glycans in the material pre-dose.
Visualization and statistical evaluation of the obtained PK parameters were performed in GraphPad Prism 8 (GraphPad Software, San Diego, US). ELISA-derived PK parameters were analyzed with a Welch’s test. For the glycoform-resolved PK parameters, glycoforms could be compared within the same animal by a paired t-test. Owing to the use of inbred rats, low biological variation and therefore normality was assumed. This was confirmed by a Shapiro–Wilk test and one exception was treated as a false-negative under the generally handled 5% false discovery rate. Multiple testing correction was applied separately to the ELISA-derived and glycoform-resolved PK parameter comparisons using the Benjamini-Hochberg approach with 5% false discovery rate.
Abbreviations
AUC | = | area-under-the-curve |
CQA | = | critical quality attribute |
ELISA | = | enzyme-linked immunosorbent assay |
Fab | = | antigen-binding fragment |
FcRn | = | neonatal Fc receptor |
IgG | = | immunoglobulin G |
LC-MS | = | liquid chromatography-mass spectrometry |
mAbs | = | monoclonal antibodies |
MS | = | mass spectrometry |
PK | = | Pharmacokinetic |
Author contributions
S.M. and M.T. coordinated and performed the glycoengineered mAbs preparation. M.T., M.L. and D.R. coordinated the animal experiments and ELISA measurements. C.J. supervised the ELISA measurements. C.K. measured and D.F. processed the glycopeptide data. M.L. calculated the PK parameters. Visualization and further statistical analyses were performed by D.F.; M.W. and D.R. conceptualized and supervised the study. All authors contributed to the preparation of the manuscript.
Competing interests
Marco Thomann, Martin Lechmann, Sebastian Malik, Cordula Jany and Dietmar Reusch are employees of Roche Diagnostics.
Supplemental Material
Download Zip (1.8 MB)Acknowledgments
Prof. Kelley Moremen from the Complex Carbohydrate Research Center (Athens, Georgia, USA) is acknowledged for generously providing the N-acetylglucosaminyltransferase I transferase. Tomasz Baginski from Genentech is acknowledged for providing the mannosidase. David Falck received support by the Dutch Research Council (NWO; Vernieuwingsimpuls Veni Project No. 722.016.008). We thank Florian Nossek, Roche Diagnostics, for his kind support with regard to sample purification. Further support was provided by Roche Diagnostics.
Data availability
mAb N-glycan profiles and ELISA-based mAb concentrations forming the basis of all (PK) analyses in this study are included in the supplementary information files (Table S8).
Supplementary material
Supplemental data for this article can be accessed on the publisher’s website.
Additional information
Funding
References
- Keizer RJ, Huitema AD, Schellens JH, Beijnen JH. Clinical pharmacokinetics of therapeutic monoclonal antibodies. Clin Pharmacokinet. 2010;49:493–11. doi:10.2165/11531280-000000000-00000.
- Brandse JF, Mathôt RA, van der Kleij D, Rispens T, Ashruf Y, Jansen JM, Rietdijk S, Löwenberg M, Ponsioen CY, Singh S, et al. Pharmacokinetic features and presence of antidrug antibodies associate with response to infliximab induction therapy in patients with moderate to severe ulcerative colitis. Clin Gastroenterol Hepatol. 2016;14:251–258 e251–252. doi:10.1016/j.cgh.2015.10.029.
- Leipold D, Prabhu S. Pharmacokinetic and pharmacodynamic considerations in the design of therapeutic antibodies. Clin Transl Sci. 2019;12:130–39. doi:10.1111/cts.12597.
- Ecker DM, Jones SD, Levine HL. The therapeutic monoclonal antibody market. MAbs. 2015;7:9–14. doi:10.4161/19420862.2015.989042.
- The Nobel Foundation.The nobel prize: nobel prizes & laureates: medicine; 2018. https://www.nobelprize.org/prizes/medicine/.
- Tabrizi MA, Tseng CM, Roskos LK. Elimination mechanisms of therapeutic monoclonal antibodies. Drug Discovery Today. 2006;11:81–88. doi:10.1016/S1359-6446(05)03638-X.
- Challa DK, Velmurugan R, Ober RJ, Sally Ward E. FcRn: from molecular interactions to regulation of IgG pharmacokinetics and functions. Curr Top Microbiol Immunol. 2014;382:249–72. doi:10.1007/978-3-319-07911-0_12.
- Higel F, Seidl A, Sorgel F, Friess W. N-glycosylation heterogeneity and the influence on structure, function and pharmacokinetics of monoclonal antibodies and Fc fusion proteins. Eur J Pharm Biopharm. 2016;100:94–100. doi:10.1016/j.ejpb.2016.01.005.
- Allavena P, Chieppa M, Monti P, Piemonti L. From pattern recognition receptor to regulator of homeostasis: the double-faced macrophage mannose receptor. Crit Rev Immunol. 2004;24:179–92. doi:10.1615/CritRevImmunol.v24.i3.20.
- Kogelberg H, Tolner B, Sharma SK, Lowdell MW, Qureshi U, Robson M, Hillyer T, Pedley RB, Vervecken W, Contreras R, et al. Clearance mechanism of a mannosylated antibody-enzyme fusion protein used in experimental cancer therapy. Glycobiology. 2007;17:36–45. doi:10.1093/glycob/cwl053.
- Brunn ND, Mauze S, Gu D, Wiswell D, Ueda R, Hodges D, Beebe AM, Zhang S, Escandon E. The role of anti-drug antibodies in the pharmacokinetics, disposition, target engagement, and efficacy of a gitr agonist monoclonal antibody in mice. J Pharmacol Exp Ther. 2016;356(3):574–86. doi:10.1124/jpet.115.229864.
- Datta-Mannan A, Lu J, Witcher DR, Leung D, Tang Y, Wroblewski VJ. The interplay of non-specific binding, target-mediated clearance and FcRn interactions on the pharmacokinetics of humanized antibodies. MAbs. 2015;7(6):1084–93. doi:10.1080/19420862.2015.1075109.
- Reusch D, Tejada ML. Fc glycans of therapeutic antibodies as critical quality attributes. Glycobiology. 2015;25:1325–34. doi:10.1093/glycob/cwv065.
- Golay J, Da Roit F, Bologna L, Ferrara C, Leusen JH, Rambaldi A, Klein C, Introna M. Glycoengineered CD20 antibody obinutuzumab activates neutrophils and mediates phagocytosis through CD16B more efficiently than rituximab. Blood. 2013;122:3482–91. doi:10.1182/blood-2013-05-504043.
- Kapur R, Einarsdottir HK, Vidarsson G. IgG-effector functions: “the good, the bad and the ugly”. Immunol Lett. 2014;160:139–44. doi:10.1016/j.imlet.2014.01.015.
- Kaneko Y, Nimmerjahn F, Ravetch JV. Anti-inflammatory activity of immunoglobulin G resulting from Fc sialylation. Science. 2006;313:670–73. doi:10.1126/science.1129594.
- Huang L, Biolsi S, Bales KR, Kuchibhotla U. Impact of variable domain glycosylation on antibody clearance: an LC/MS characterization. Anal Biochem. 2006;349:197–207. doi:10.1016/j.ab.2005.11.012.
- Jones AJ, Papac DI, Chin EH, Keck R, Baughman SA, Lin YS, Kneer J, Battersby JE. Selective clearance of glycoforms of a complex glycoprotein pharmaceutical caused by terminal N-acetylglucosamine is similar in humans and cynomolgus monkeys. Glycobiology. 2007;17:529–40. doi:10.1093/glycob/cwm017.
- Kanda Y, Yamada T, Mori K, Okazaki A, Inoue M, Kitajima-Miyama K, Kuni-Kamochi R, Nakano R, Yano K, Kakita S, et al. Comparison of biological activity among nonfucosylated therapeutic IgG1 antibodies with three different N-linked Fc oligosaccharides: the high-mannose, hybrid, and complex types. Glycobiology. 2007;17(1):104–18. doi:10.1093/glycob/cwl057.
- Chen X, Liu YD, Flynn GC. The effect of Fc glycan forms on human IgG2 antibody clearance in humans. Glycobiology. 2009;19:240–49. doi:10.1093/glycob/cwn120.
- Goetze AM, Liu YD, Zhang Z, Shah B, Lee E, Bondarenko PV, Flynn GC. High-mannose glycans on the Fc region of therapeutic IgG antibodies increase serum clearance in humans. Glycobiology. 2011;21(7):949–59. doi:10.1093/glycob/cwr027.
- Alessandri L, Ouellette D, Acquah A, Rieser M, LeBlond D, Saltarelli M, Radziejewski C, Fujimori T, Correia I. Increased serum clearance of oligomannose species present on a human IgG1 molecule. MAbs. 2012;4(4):509–20. doi:10.4161/mabs.20450.
- Higel F, Seidl A, Demelbauer U, Viertlboeck-Schudy M, Koppenburg V, Kronthaler U, Sörgel F, Frieß W. N-glycan PK profiling using a high sensitivity nanoLCMS work-flow with heavy stable isotope labeled internal standard and application to a preclinical study of an IgG1 biopharmaceutical. Pharm Res. 2015;32(11):3649–59. doi:10.1007/s11095-015-1724-0.
- Yu M, Brown D, Reed C, Chung S, Lutman J, Stefanich E, Wong A, Stephan J-P, Bayer R. Production, characterization and pharmacokinetic properties of antibodies with N-linked mannose-5 glycans. MAbs. 2012;4(4):475–87. doi:10.4161/mabs.20737.
- Hua S, Oh MJ, Ozcan S, Seo YS, Grimm R, An HJ. Technologies for glycomic characterization of biopharmaceutical erythropoietins. Trends Anal Chem. 2015;68:18–27. doi:10.1016/j.trac.2015.02.004.
- Keck R, Nayak N, Lerner L, Raju S, Ma S, Schreitmueller T, Chamow S, Moorhouse K, Kotts C, Jones A, et al. Characterization of a complex glycoprotein whose variable metabolic clearance in humans is dependent on terminal N-acetylglucosamine content. Biologicals. 2008;36(1):49–60. doi:10.1016/j.biologicals.2007.05.004.
- Gahoual R, Heidenreich A-K, Somsen GW, Bulau P, Reusch D, Wuhrer M, Haberger M. Detailed characterization of monoclonal antibody receptor interaction using affinity liquid chromatography hyphenated to native mass spectrometry. Anal Chem. 2017;89:5404–12. doi:10.1021/acs.analchem.7b00211.
- Wada R, Matsui M, Kawasaki N. Influence of N-glycosylation on effector functions and thermal stability of glycoengineered IgG1 monoclonal antibody with homogeneous glycoforms. MAbs. 2019;11:350–72. doi:10.1080/19420862.2018.1551044.
- Dashivets T, Thomann M, RuegL.er P, Knaupp A, Buchner J, Schlothauer T. Multi-angle effector function analysis of human monoclonal IgG glycovariants. PLoS One. 2015;10(12):e0143520. doi:10.1371/journal.pone.0143520.
- Wright A, Morrison SL. Effect of altered CH2-associated carbohydrate structure on the functional properties and in vivo fate of chimeric mouse-human immunoglobulin G1. J Exp Med. 1994;180:1087–96. doi:10.1084/jem.180.3.1087.
- Dekkers G, Treffers L, Plomp R, Bentlage AEH, de Boer M, Koeleman CAM, Lissenberg-Thunnissen SN, Visser R, Brouwer M, Mok JY, et al. Decoding the human immunoglobulin G-glycan repertoire reveals a spectrum of fc-receptor- and complement-mediated-effector activities. Front Immunol. 2017;8:877. doi:10.3389/fimmu.2017.00877.
- Yang WH, Aziz PV, Heithoff DM, Mahan MJ, Smith JW, Marth JD. An intrinsic mechanism of secreted protein aging and turnover. Proc Natl Acad Sci U S A. 2015;112(44):13657–62. doi:10.1073/pnas.1515464112.
- Mordenti J, Chen SA, Moore JA, Ferraiolo BL, Green JD. Interspecies scaling of clearance and volume of distribution data for 5 therapeutic proteins. Pharm Res. 1991;8:1351–59. doi:10.1023/A:1015836720294.
- Lin YS, Nguyen C, Mendoza JL, Escandon E, Fei D, Meng YG, Modi NB. Preclinical pharmacokinetics, interspecies scaling, and tissue distribution of a humanized monoclonal antibody against vascular endothelial growth factor. J Pharmacol Exp Ther. 1999;288:371–78.
- Thomann M, Schlothauer T, Dashivets T, Malik S, Avenal C, Bulau P, Rüger P, Reusch D. In vitro glycoengineering of IgG1 and its effect on Fc receptor binding and ADCC activity. PLoS One. 2015;10(8):e0134949. doi:10.1371/journal.pone.0134949.
- Stockert RJ. The asialoglycoprotein receptor: relationships between structure, function, and expression. Physiol Rev. 1995;75:591–609. doi:10.1152/physrev.1995.75.3.591.
- Grewal PK. The Ashwell-Morell receptor. Methods Enzymol. 2010;479:223–41.
- de Haan N, Falck D, Wuhrer M. Monitoring of immunoglobulin N- and O-glycosylation in health and disease. Glycobiology. 2019;30(4):226–240. https://doi.org/10.1093/glycob/cwz048.
- Falck D, Jansen BC, de Haan N, Wuhrer M. High-throughput analysis of IgG Fc glycopeptides by LC-MS. Methods Mol Biol. 2017;1503:31–47.
- Jansen BC, Falck D, de Haan N, Hipgrave Ederveen AL, Razdorov G, Lauc G, Wuhrer M. LaCyTools: a targeted liquid chromatography-mass spectrometry data processing package for relative quantitation of glycopeptides. J Proteome Res. 2016;15:2198–210. doi:10.1021/acs.jproteome.6b00171.