ABSTRACT
The human IgG3 subclass is conspicuously absent among the formats for approved monoclonal antibody therapies and Fc fusion protein biologics. Concern about the potential for rapid degradation, reduced plasma half-life, and increased immunogenicity due to marked variation in allotypes has apparently outweighed the potential advantages of IgG3, which include high affinity for activating Fcγ receptors, effective complement fixation, and a long hinge that appears better suited for low abundance targets. This review aims to highlight distinguishing features of IgG3 and to explore its functional role in the immune response. We present studies of natural immunity and recombinant antibody therapies that elucidate key contributions of IgG3 and discuss historical roadblocks that no longer remain clearly relevant. Collectively, this body of evidence motivates thoughtful reconsideration of the clinical advancement of this distinctive antibody subclass for treatment of human diseases.
Abbreviations: ADCC - Antibody-Dependent Cell-mediated CytotoxicityADE – Antibody-dependent enhancementAID – Activation-Induced Cytidine DeaminaseCH – Constant HeavyCHF – Complement factor HCSR – Class Switch RecombinationEM - Electron MicroscopyFab – Fragment, antigen bindingFc – Fragment, crystallizableFcRn – Neonatal Fc ReceptorFcγR – Fc gamma ReceptorHIV – Human Immunodeficiency VirusIg - ImmunoglobulinIgH – Immunoglobulin Heavy chain geneNHP – Non-Human Primate
Introduction
As both understanding of antibody sequence-structure-function relationships and the number of antibody therapeutics in development and clinical use increase each year, it is surprising that the unique attributes of the immunoglobulin gamma 3 (IgG3) subclass have not supported its translation into clinical practice by now. While the absence of IgG3 antibodies for therapy can be attributed to historical concerns about the ability to manufacture IgG3 in large scale as well as its in vivo half-life, stability, and immunogenicity, new data suggest that these exclusion criteria should be reconsidered. Here, we summarize the unique structural characteristics of human IgG3, including its extended hinge architecture, which offers both Fab–Fab and Fab–Fc distances and domain flexibilities not observed for other subclasses, and its unique functional attributes, which have yet to be fully leveraged in antibody design. We also explore associations between IgG3 responses in immunization and infection to disease outcomes to elucidate potential immunological significance, and consider subclass switching experiments to provide mechanistic insights into the potential relevance of these associations. We revisit prior studies that have provided inferences to suggest challenges to translational development, and describe recent efforts to stimulate new interest in exploring the range of human IgG diversity for therapeutic purposes.
Human IgG subclasses: diversity in specificity, structure, and function
The four human IgG subclasses are named according to serum prevalence and exhibit relatively high sequence homology (~95%); however, amino acid variation at key positions results in incredible functional diversity.Citation1,Citation2 IgG1 and IgG3 subclasses are generally elicited in response to protein antigens associated with viral infections, IgG2 typically targets polysaccharide antigens induced by bacterial infections, and the IgG4 response is associated with repeated exposure to allergens in the absence of an infectious agent.Citation3–5 Such diversity among target antigens is reflected in functional differences between subclasses. Whereas IgG1 and IgG3 are potent activators of innate immune effector cells, IgG2 and IgG4 possess more subdued effector activity. The existence of these distinct types of IgGs and their association with divergent immunologic stimuli suggests that human subclass diversity results from evolutionary pressures applied over long time periods. However, the immune system also leverages this diversity over short timescales – displaying rapidly divergent activity profiles of isotypes and subclasses when individuals are repeatedly exposed to foreign antigens, in which multiple rounds of class switch recombination (CSR) can occur.
Structurally, the principal differences among the human IgG subclasses relate to their hinge compositions, i.e., the sequence linking the CH1 and CH2 regions that comprise parts of the Fab and Fc domains, respectively (). Following reports of full-length human IgG 1, 2, and 4 sequences in the early 1980s,Citation7 the basis for the most striking distinction of IgG3 was apparent from its sequence, reported a few years later.Citation8 IgG3 possesses a unique extended hinge, typically composed of quadruplicate proline-rich repeated motifs with extensive interchain disulfide bonds, as well as a slightly extended upper hinge adjoining the CH1 (totaling 62 amino acids), which is encoded by four exons. In contrast, IgG4 has reduced hinge length (12 amino acids, as compared to 15 for IgG1) and can also undergo a process known as Fab arm exchange,Citation9,Citation10 in which the heavy-chain dimer can dissociate and re-associate in serum. This process leads to functional monovalency in antigen recognition based on the resultant swapping of Fab specificities. Lastly, IgG2 contains a 12 amino acid hinge with four disulfide bonds. Based on studies of IgG2 disulfide bond isomers,Citation11,Citation12 it is known that even subtle structural changes in the hinge can affect hydrodynamic radius, antigen binding, ligand blocking, and in vivo potency.Citation12–17
Figure 1. Flexibility and other features of human IgG subclasses. Top: Representation of the Fab domain flexibility with respect to the hinge regions of the human IgG subclasses in which positions for Fab domains are represented by spheres in purple and teal. Figure adapted from Hansen et al.Citation6 with permission. Bottom: Measured values for various physical attributes of IgG3 compared to other human subclasses
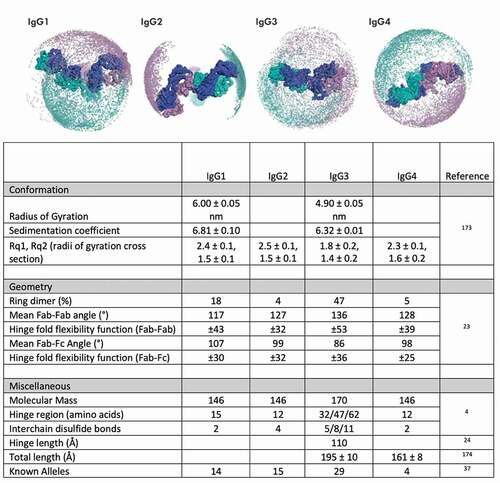
Collectively, these distinctions among hinges result in a rich structural and conformational landscape (usefully visualized in Hayes et al.Citation18). While the hinge contains many constrained proline and disulfide-bonded cysteine residues, its superstructure has been demonstrated to be quite flexible. Only a few antibodies have been crystallized as whole molecules,Citation19 sometimes requiring perturbation γ correction to gain clarified density for the hinge, carbohydrate, and solvent-exposed areas. Among IgG subclasses, circular dichroism has shown that the IgG3 hinge has a high degree of secondary structure,Citation20 and significantly extended Fab–Fc distance. Each core repeat adds an estimated 25 Å between the Fab and Fc.Citation18,Citation21,Citation22 Electron microscopy (EM), including cryogenic EM, have been used to define IgG hinge flexibility and range of motionCitation19,Citation23 and enable visualization of multiple conformation states. Aside from Fab–Fc distance, Fab–Fab distance and flexibility, as defined by permissible and mean Fab–Fab angles (), are also greater in IgG3 compared to the other IgG subclassesCitation24 and have been found to directly correlate with upper hinge length.Citation22 This flexibility results in distinctly different conformational compositions,Citation6 which could affect epitope accessibility and binding valency.
Such differences in flexibility and reach between Fab arms and the Fc domains among human IgG subclasses suggest that factors beyond intrinsic antigen and Fc receptor binding affinities can affect antibody functionality. Specifically, differences in bivalent antigen-binding capacity have been suggested by a number of studies.Citation25–27 Toward this end, the most intriguing evidence of the biological consequences of differing hinge composition can be found in the superior HIV-1 neutralization activity of polyclonal, serum-derived bivalent Fab fragments of different subclasses. Whereas equivalent neutralization potency was observed for monovalent IgG1 and IgG3 Fab fragments, suggesting equivalent intrinsic Fab neutralization potency, bivalent IgG3 Fab’2 demonstrated potentiated neutralization relative to IgG1 Fab’2, pointing toward a key role for distinctions in hinge architecture in activities that do not require the Fc domain.Citation28 Notably, multiple broadly neutralizing HIV-specific antibodies were discovered as IgG3s, particularly those recognizing relatively poorly accessible epitopes on the envelope glycoprotein that are proximal to the membrane,Citation29–31 and subclass switching experiments have demonstrated enhanced neutralization potency of IgG3 forms in a number of cases.Citation25,Citation32–34 Together, these isotype switching experiments suggest that factors other than Fab affinity contribute to neutralization activity: the unique structure and sequence aspects that differentiate IgG3 from the other subclasses are thought to potentially affect avid viral recognition, a factor shown to be very important to neutralization potency in experiments with non-native linkers between Fab domains and with “unzipped” hinges in which disulfide bond cysteines have been deleted.Citation33,Citation35
In sum, Fab–Fab and Fab–Fc flexibility along with variable Fab-Fc length distinguish IgG3. Evidence suggests that the IgG3 hinge may allow targeting of antigens or epitopes less suited to ligation by other IgG types, with the potential to more effectively stimulate cellular activity via Fcɣ receptors.
Allotypic IgG3 diversity in humans
With 29 reported allelic variants () defined to date, IgG3 is the most polymorphic of the human IgG subclasses ().Citation36,Citation37 Unlike other subclasses that vary principally in terms of isolated amino acid substitutions, IgG3 also has structural allotypes that vary in the number of exon repeats (from 1 to 3) in the core hinge.Citation38–41 While there is little to suggest direct functional relevance of most allotypic variation among IgG subclasses,Citation38 associations between IgG allotypes and a wide variety of infections, malignancies, and autoimmune conditions have been observed.Citation42–48 In contrast, while IgG3 allotypes have likewise been associated with differences in other aspects of the immune response,Citation49 both structure and sequence distinctions among allotypes of this subclass are known to affect antibody function.
Table 1. Human IgG3 allotypes. Individual allelic positions are mapped against IGHG3*01 sequence by EU position number. The first listed accession number in the IMGT database is listed for each given allelic variant. Although several alleles contain synonymous polymorphisms, only non-synonymous SNPs are listed
Figure 2. Sites of amino acid polymorphisms in IgG3 CH2 and CH3 domains. Red amino acids indicate sites of amino acid sequence diversity among G3m allotypes. Brackets indicate recognition footprints of various human Fc receptors
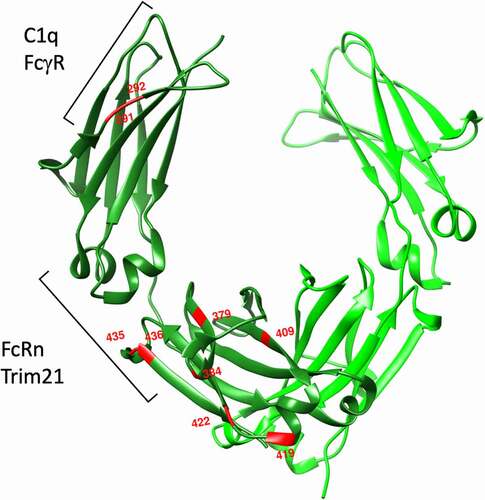
The best-known example of a highly impactful IgG polymorphism is perhaps the R435H polymorphism observed among several G3m allotypes. This polymorphism alters pH-dependent binding to the neonatal Fc receptor (FcRn), which functions as a mucosal transport and systemic recycling receptor. Whereas allotypes bearing an arginine at this position exhibit rapid clearance with a half-life of approximately 1 week, substitution with histidine results in an extended 21-day IgG1-like half-life.Citation50 This allotypic variant is quite prevalent in South Asian (10–25%) and African populations (30–60%),Citation38,Citation51 and has been associated with better transplacental transport of maternal antibody and resulting improvements in neonatal protection from malaria.Citation51
Hinge length is a second functional consequence of allotypic variation in IgG3. Polymorphisms in hinge length have been observed primarily in sub-Saharan populations and can occur concurrently with other point mutations.Citation4 For example, in the context of an HIV-neutralizing antibody, the IgG3*17 allotype, with a 47 rather than 62 amino acid-long hinge has been demonstrated to exhibit reduced neutralization potency and effector function.Citation34 Beyond the shorter hinge, this allotype also has a lysine at position 392, which eliminates a glycosylation motif at a site that has been reported to contribute to molecular stability and affect interactions with FcɣRIIIa and antibody-dependent cell-mediated cytotoxicity (ADCC).Citation34,Citation52,Citation53 Hinge exon deletions that mimic naturally occurring allotypes demonstrate an enhancing effect on complement activation and complement-mediated lysis,Citation54,Citation55 whereas extensions have been reported to enhance phagocytosis.Citation56 Collectively, the impact of these genetic differences and potentially others that influence induction, persistence, biodistribution, and the function of IgG3s merits further study in the context of responses to infection or vaccination, as well as in antibody engineering.
IgG3 Glycosylation
Despite accounting for only 2–3% of the mass of an IgG molecule, glycans affect critical antibody functions such as Fc receptor binding.Citation57 N-glycosylation of a conserved site in the CH2 domain is required for antibody binding to Fc receptors, and the specific composition of the glycoform incorporated alters some antibody activities by more than an order of magnitude.Citation58 While the human subclasses show diverse inherent effector function, the impact of different Fc glycoforms appears to be consistent across subclasses. For example, the enhanced FcγRIII binding imparted by afucosylated glycans is maintained for IgG3.Citation59 Among naturally derived antibodies, IgG1 and IgG3 N-glycosylation patterns are similar in whole blood as well as among antigen-specific antibody subpopulations,Citation60 but can differ when produced recombinantly using different cell lines.Citation61
In contrast to Fc domain-N-glycosylation, the effect of antibody O-glycosylation on function is less well documented, despite being observed within the hinge region. Only a few antibody classes contain O-glycosylation sites within their hinge regions, including human IgA1,Citation62 human IgD,Citation63 human IgG3,Citation64 and mouse IgG2b.Citation65 The presence of the O-glycan has been hypothesized to provide proteolytic resistance, but glycosylation sites are only partially occupied in both serum and recombinant IgG3.Citation64 Whether the presence of O-glycans in the hinge affect structure or function has yet to be thoroughly studied, but may represent another important means to modify antibody bioactivity.
Subclass and hinge diversity across species
IgG subclasses are not clearly conserved in terms of arrangement, sequence, structure, or activity between humans, non-human primates (NHP), or mice (). In fact, because the subclass naming convention typically follows serum prevalence rather than sequence or functional homology, subclass numbers between species have highly divergent properties. For instance, both mouse IgG2 and IgG3 are capable of activating complement,Citation66 but mouse IgG2 and its isoforms can additionally activate the immune system through FcγR signaling, and IgG1 lacks or shows dramatically reduced effector function.Citation67–69 Mice also lack an IgG4 or an analogue, which in humans is capable of undergoing Fab arm exchange. In humans, IgG1 and IgG3 are capable of both potent FcɣR signaling as well as complement activation, and IgG2 and IgG4 tend to be more functionally inert. Such broad, overarching differences in IgG subclass characteristics are then compounded by species-specific differences in FcɣR properties and expression profiles, which pose substantial challenges when trying to recapitulate the human immune response in animal models.
Figure 3. Human IgG responses over time and IgH locus arrangement in human, mouse, and rhesus. Top: Schematic of subclass composition over time for human IgG. IgG3 appears early and wanes over time. IgG1 additionally increases early and titer remains high. IgG2 and IgG4 appear later in infection. Affinity increases over time during infection due to somatic hypermutation. Bottom: IGHC locus arrangement across species. Subclass naming convention is based on serum prevalence rather than genetic similarity
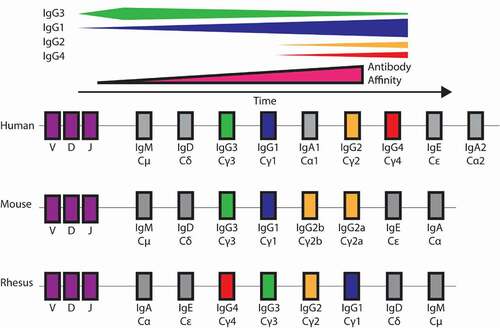
Activity and receptor affinity differences are more limited among macaque IgG subclasses compared to human and mouse IgG subclasses, whose activity and FcR ligation properties vary by orders of magnitude. In rhesus macaques, all four IgG types have high sequence homology to human IgG1.Citation70 Neither mice nor NHP possess a subclass with an extended human IgG3-like hinge, and differences in Fab arm exchange of IgG4 also exist between species.Citation71
Beyond these fundamental differences in subclass profiles between species, the considerably extended four-exon IgG3 hinge is a feature that is only currently known to exist in humans (). Neither rhesus macaques (Macaca mulatta), nor chimpanzees (Pan troglodytes), nor baboons (Papio cynocephalus anubis) have IgGs with extended hinges,Citation70,Citation72,Citation73 though the chimpanzee IgG3 amino acid hinge, which has only one core hinge region, aligns perfectly with the human IgG3 upper hinge. Among other species, camelid IgG2a contains a 35 amino acid long hinge that has only three inter-chain disulfide bonds, which are situated toward the CH2 domain resulting in a more “open” configuration.Citation74–76 While the impact of this hinge extension on camelid IgG function is unclear, its existence suggests that length polymorphisms can arise in other species, and may be an example of convergent evolution, as diversification of the Ig locus results in sampling of different sequence-function profiles. Overall, the unique attributes of the human IgG3 hinge in antigen recognition and effector function suggest the existence of a blind spot that may arise from trying to model human responses in animal models.
Table 2. IgG hinges of various species. Red lettering indicates disulfide bond positions
The IgH locus is variably arranged across species ()Citation77,Citation78 (IMGT Repertoire (IG and T cell receptors) http://www.imgt.org/IMGTrepertoire/). Direct comparison across species is much more complex than simply comparing antibodies with similar functions. Certain subclasses share similar effector activity, such as mouse IgG2a and human IgG1, but human Igs do not have the same splicing complexity as mouse. While the exact same process in different species generates similar molecules, each animal has a unique fingerprint to their Ig repertoires in terms of composition, concentration, and timing of Ig molecules.
Subclass switching over the course of an immune response
Class switching is a DNA recombination process characterized by double-strand breaks and deletion between “switch” regions driven by activation-induced cytidine deaminase (AID). As a result, while repeated recombination events can occur, they are restricted to downstream subclasses in a process linked to cell division and regulated by cytokines.Citation79–82 The first IgG subclass in the human IGH locus, IgG3 is associated with potent acute responses, with waning levels often observed over time.Citation83 Indeed, anti-HIV IgG3 responses peak before 4-weeks post-infectionCitation84 and subsequently decline along with effector function.Citation85 However, IgG3 responses can be persistently elevated, such as in leprosyCitation86 and tuberculosis.Citation87 In contrast, IgG4, the least abundant IgG subclass, is often associated with tolerance and has reduced hinge length and functional monovalency in antigen recognition, as described above. In the context of HIV vaccines, repetitive protein boosting has been associated with increasing prevalence and levels of IgG4 responses,Citation88–90 a characteristic observed exceedingly rarely in natural infection.Citation91 Given that sequential switching reactions are associated with greater mutation rates, relatively higher affinity and mutational loads are associated with distal IgG types.Citation92–96 Reconstructed histories of CSR among B cell Ig lineages based on variable region sequencing have offered a view of commonly observed patterns of CSR.Citation97 Among other observations, this work suggests that the proportion of direct switching from IgM or IgD follows position in the IgH locus, with all IgG3s resulting from direct switching, a majority of IgG1s, approximately half of IgG2s, and a minority of IgG4s.Citation97 Further, consistent with linked induction observed in in vitro B cell-stimulation experiments,Citation98,Citation99 such sequence-based repertoire studies have shown that CSR to IgG3 is preferentially associated with subsequent switching to IgG1.Citation97
Interestingly, human IgG subclasses are generally arranged from greater to lesser inflammatory potential (). This ordering suggests the hypothesis that evolutionary pressures have resulted in an arrangement that supports rapid and potent initial responses that can be dampened by subsequent switching – a scheme that may balance the need for inflammatory acute responses with a means to reduce the consequences of autoimmune antibodies, while still providing long-term protection. Early IgG3 antibodies might be key for enhancing opsonization of pathogenic particles and trafficking to germinal centers, where subsequent B cell somatic hypermutation and affinity maturation feedback loops then allow for the generation of antibodies with greater potency in antigen recognition if not effector function.Citation100,Citation101 Mature memory B cells tend to skew toward IgG and IgG4 expression, while naïve memory B cells tend toward more IGHM proximal subclasses IgG1 and IgG3.Citation96 While this locus arrangement – coupled with the low serum proportion of IgG3, its rapid clearance, and waning prevalence after acute responses – may suggest possible downsides to the inflammatory potential of IgG3, there are many human observational studies that suggest its beneficial contributions to protection from infection.
Table 3. FcγR binding affinities of human IgG Subclasses. Some interactions were not measurable (n.m)
Natural infection histories
Classically, IgG3 has been grouped with IgG1 in terms of immune function because both bind-activating FcɣR well and primarily target protein antigens.Citation1,Citation102–104 As might be expected given their linked induction in in vitro CSR experiments,Citation98,Citation99 IgG1 and IgG3 responses have been observed to be correlated.Citation105,Citation106 Between them, however, IgG3 has often been reported to demonstrate enhanced phagocytosisCitation2,Citation56,Citation107–109 and complement deposition, particularly 108, 109, 110in the context of low-density target antigen.Citation110–112
Consistent with this enhanced functional potency, induction of IgG3 can be a key marker for protection, whereas its absence is associated with infectious disease susceptibility. Adults and children with otherwise normal total IgG levels and functional B cell compartments but IgG3 subclass deficiency tend to present with recurrent upper respiratory tract infections.Citation113–116 These individuals tend to respond well to intravenous Ig treatment,Citation117–120 suggesting that their antibody repertoires are relatively functionally naïve toward common pathogens despite multiple encounters. Whether IgG3 is directly responsible for pathogen sequestration or contributes to the development of other protective antibodies is unclear and merits further consideration.
In support of potential mechanistic relevance, IgG3 responses have been associated with better outcomes across diverse infectious pathogens in the setting of both natural infection and vaccination. For instance, IgG3 responses against the HIV V1/V2 loop regions in the RV144 HIV vaccine trial correlated with decreased infection risk.Citation88,Citation89,Citation121 Early IgG3 responses against chikungunya virus infection are associated with more rapid viral clearance and a reduction in long-term arthralgic sequelae,Citation122 and in clearance of acute hepatitis C virus infection.Citation123 A number of studies have reported that IgG3 responses induced by infection are associated with reduced risk of malaria disease. Interestingly, IgG3 responses increase with age, as does clinical immunity.Citation124 Associations between IgG3 and better outcomes are also observed in children.Citation125–127 In the context of the IgG3-H435 polymorphism that leads to better transport and recycling by FcRn, IgG3 responses have even been linked to infant protection through enhanced placental transfer of maternal antibody.Citation51 In contrast, immunity to influenza from vaccination wanes with age,Citation128 along with a corresponding reduction in the prevalence and magnitude of flu-specific IgG3,Citation129–131 among many other parameters.
In contrast, in other settings, antibodies can increase infection risk or disease severity by facilitating viral uptake into host cells that express antibody receptors. In these cases, the enhanced ability of IgG3 to ligate antigen and interact with FcɣR has the potential to exacerbate disease. As examples, IgG3 antibodies against Ebola, Zika, and enterovirus have been shown to exhibit higher levels of antibody-dependent enhancement (ADE) of infection in vitro than other subclasses.Citation132–134 The most compelling evidence of the relevance of ADE to infection in vivo comes from dengue, in which severity of secondary infection is associated with levels and types of virus-specific antibodies present in serum. Subneutralizing quantities,Citation135 but more concerningly, effective FcγR ligation associated with relatively high IgG1/IgG2 ratios and afucosylationCitation136,Citation137 have been associated with disease enhancement, though correlation between IgG3 responses and severe disease has not been described.
In summary, it is clear that the potentiated effector function profile of IgG3 can be associated with enhanced protection, suggesting the contributions this unique subclass could make in the context of antibody therapeutics. In cases where IgG3 responses may be causally linked to different infectious disease outcomes, diverse mechanisms are possible. Beyond expected differences in effector function, previous studies have linked this subclass to potentiated neutralization activity in polyclonal responsesCitation28 and among monoclonal antibodies.Citation25,Citation32,Citation33,Citation138 Importantly, the neutralization enhancements observed for subclass-switched monoclonal antibodies imply that, beyond the well-known impact of subclass and glycosylation on FcR binding and effector function, structural differences between subclasses can affect other potentially protective antibody activities.
Lost in translation
Subclass selection represents a modular and predictable means to control antibody function, making it a key aspect of therapeutic antibody development.Citation139 Despite being perhaps the most functional subclass,Citation1,Citation53,Citation140,Citation141 there is a surprising lack of IgG3 antibodies among approved therapeutics, as well as a paucity among early clinical and pre-clinical candidates under evaluation. This absence can be attributed to four historical factors: 1) greater allotypic variation among IgG3s, raising concern about potential immunogenicity;Citation142 2) the reduced plasma half-life typical of IgG3, increasing the frequency or level of dose required to achieve a given antibody concentration relative to IgG1;Citation143 3) the absence of binding to protein A, eliminating this standard approach to antibody purification and requiring alternative and more costly approaches;Citation144 and 4) the potential of proteolytic susceptibility of the extended hinge, raising concern about in vivo stability;Citation139 However, as discussed below, these perceived challenges now have simple solutions or new data suggest that they may not be sufficiently scientifically backed to cause concern.
As mentioned previously, a single, naturally polymorphic amino acid substitution improves the half-life and mucosal transport properties of IgG3 to those observed for IgG1.Citation50 Given the success of non-native Fc domains in the clinic, such as those engineered for extended half-life,Citation145–147 selection of a H435-bearing IgG3 allotype to impart persistent plasma pharmacokinetics seems a simple and low risk means to eliminate what has likely been the single biggest barrier to clinical translation. Allotypic variability has not been associated with anti-drug antibody responses.Citation148–150 In fact, a number of the polymorphic positions in IgG3 are “isoallotypes”, meaning that these substitutions are present in other subclasses and thus may not have a propensity to be immunogenic.
As to challenges in purification, other purification methods, such as protein G resinCitation151 and CaptureSelect FcXL affinity matrix are available, and have been used for commercial purification. Alternatively, the use of the same H435 IgG3 allotype that extends half-life also allows for protein A binding,Citation152 enabling the use of current standard industrial purification methods. Like other subclasses, IgG3 is susceptible to aggregation at low pH.Citation153 IgG3 potentially has somewhat elevated susceptibility in this regard, including aggregation during expression, as compared to IgG1, but recent work has used sequence swapping to reduce this profile.Citation154 In terms of thermal stability, IgG3 has been omitted from some studies given its absence from the clinic.Citation155 However, it is likely that further improvements to expression, purification, and storage schemes will also provide value and help to smooth the path toward broader in vivo and clinical investigation.
Lastly, despite greater sensitivity to enzymatic cleavage in vitro in intentional proteolysis experiments,Citation156–158 hinge cleavage has not been reported to pose a barrier in vitro or in vivo. Further, as gene therapy tools advance, the prospects for vectored deliveryCitation159,Citation160 of IgG3 could bypass concerns about clearance and stability. Collectively, new evidence and clinical experience suggest that the absence of clinically advanced IgG3 is a historical artifact, and lack of precedent need not be considered a barrier to their future use. Gaining regulatory agency and industry buy-in for development of the first IgG3 monoclonal treatment may be more a problem of changing minds than overcoming scientific barriers.
Forward toward the clinic: evaluation in animal models and the first human IgG3s tested in humans
A limited number of subclass switching experiments have been carried out that enable comparison of the in vitro and in vivo activities between subclasses. One study in mice described similar biodistribution but did not test in vivo efficacy.Citation56 In another study, despite lower serum titers, the passive transfer of an HIV envelope glycoprotein variable loop-specific IgG3 antibody was at least equally effective in preventing viral infection as IgG1 in an NHP model of HIV infection.Citation161 In contrast, in a mouse model of melanoma, IgG3 against the TA99 target antigen was not superior to IgG1 at protecting against metastasis.Citation162 Though generally supportive of the feasibility to clinically translate IgG3, these case studies do not reflect settings in which IgG3 antibodies were superior in their in vitro functions: the TA99-specific IgG3 was not superior to IgG1 in vitro, and the HIV envelope variable loop-specific IgG3 showed similar binding activity and phagocytic activity as compared to IgG1.109 This latter experiment was performed instead to investigate the basis of IgG3 responses to this epitope being correlated with reduced risk of infection in the RV144 vaccine trial.
The strongest evidence in support of enhanced activity of IgG3 in vivo comes from the setting of antibodies to pneumococcus. Here, a longer half-life allotype of IgG3 was shown to provide improved protection against pneumococcal pneumonia, as defined by colony-forming units in lung, as compared to IgG1.Citation50 Other contexts in which IgG3 is clearly more potent than IgG1, such as in the bactericidal activity of antibodies specific to meningococcal factor h binding protein,Citation163,Citation164 may provide further opportunity to better establish the ability of in vitro functional enhancements to improve in vivo protective activity. To this end, an anti-Protein A IgG2 antibody fragment isolated from a human donor via phage display was reformatted as an IgG3 and demonstrated strong activity against antibiotic sensitive and methicillin-resistant Staphylococcus aureus in vitro and was able to provide 60% protection from fatal S. aureus challenge in a mouse model.Citation165 Other subclasses, however, were not evaluated in comparison. Nonetheless, the promising results of these limited in vivo studies and the more prevalent observations from natural infection correlates and in vitro studies in which subclass switching from IgG1 to IgG3 has enhanced desirable antibody activities suggest that further investigation in vivo is merited.
To our knowledge, two monoclonal IgG3 antibodies have been investigated clinically. A Phase 1/2 clinical trial (NCT02357966) evaluated the Protein A-specific IgG3 monoclonal (omodenbamab or 514G3) described above in the setting of Staphylococcus aureus bacteremia with promising results. The IgG3 backbone was specifically chosen to avoid Fc interactions with protein A (SpA). Concluded in 2017, this clinical trial found that the IgG3 treatment was well tolerated and generated few severe adverse events.Citation166 Efficacy data was self-reported by the sponsoring company (Xbiotech Inc.), but as of this time not peer-reviewed. The use of IgG3 to avoid protein A interaction is a good demonstration of utilizing subclass selection for therapeutic design, and its tolerability is encouraging from a safety standpoint.
In the oncology space, one recent study of lung cancer patients found an IgG3 autoantibody against complement factor H (CFH) that was associated with early-stage disease and no evidence of metastasis. Single B cells producing the CFH-specific response were isolated from these patients, the Ig gene sequenced, and a series of preclinical studies then demonstrated tumor cell killing with modulation of the adaptive immune response using the molecule GT103.Citation167 A recombinant CFH-specific monoclonal antibody, originally identified and formulated as an IgG3, has now been successfully manufactured on a large scale for clinical trial NCT04314089, which was initiated in June 2020. This human-derived antibody will be the first monoclonal therapeutic IgG3 to be tested in humans against cancer and will certainly provide essential information about IgG3 as a viable therapeutic option.
Beyond natural Ig diversity
Cumulatively, natural histories and subclass switch experiments also point to the potential value of IgG3-inspired engineering of other subclasses. To this end, substantial work has already been invested in innovating on natural forms. Considering developability, IgG3 has been engineered for reduced aggregation and better tolerance of pH stress.Citation154
Beyond correction of undesirable physicochemical attributes, chimeric IgG3s have been advanced as molecules with enhanced functionality. For example, such chimeras have shown enhanced complement-dependent cytotoxicity, ADCC function, and target cell depletion in vivo in NHP.Citation53,Citation168 One such molecule is GSK2849330,Citation169 an anti-HER3 glycoengineered IgG1/IgG3 chimera investigated in two Phase 1 clinical studies to date: NCT02345174, initiated in 2015 and completed in 2016; and NCT01966445, initiated in 2013 and completed in 2017. This molecule was engineered for enhanced ADCC and complement-mediated cytotoxicity by utilizing the IgG3 Fc, although the longer half-life amino acid allotype/point mutation was not used. An imaging study of a radiolabeled form of this antibody showed good tumor uptake,Citation170 and in a separate study of 29 individuals, one exceptional responder was noted.Citation171 Accordingly, these and other such strategies may hold further promise in combination with existing glycan engineering and the wealth and diversity of amino acid point mutations developed to alter FcR and C1q interactions.Citation172–174
Based on studies suggesting the significance of the IgG3 hinge to its unique properties, non-native hinges have also been investigated. Constructs exploring alternative hinge topologies have included substitution with traditional, conformationally flexible linkers such as repeating Gly4Ser subunits;Citation175 “unzipped” native hinges, in which cysteines have been eliminated in an effort to increase the span between Fab domains;Citation33 and hinge-length extension by repeating natural hinge exon subunits an unnatural number of times.Citation56 These approaches, which can enhance antibody function by orders of magnitude, are thought to work by enhancing binding to low abundance, poorly exposed, or distantly spaced target epitopes. Collectively, these results suggest that beyond translation of natural IgG3 constant domains, antibody engineering based on the unique structural and functional attributes of IgG3 holds promise in improving the potency or activity of IgG1-based therapies.
Conclusion
In summary, considerable epidemiological data suggest that relative to their serum prevalence, IgG3 antibodies make an important contribution to effective humoral immune responses. Extensive subclass switching experiments in vitro point to the potential mechanistic relevance of associations in these cohort studies, with IgG3 often inducing elevated phagocytosis and greater complement activation. Albeit limited, the in vivo studies conducted to date comparing IgG3 and IgG1 antibodies suggest that IgG3 results in equivalent or superior outcomes, supporting further exploration of this subclass in early preclinical studies for applications where its structural and functional distinctions may prove advantageous. These applications include those where antibody effector function is desirable, where antigenic epitopes may be sparse or difficult to access, and in the settings in which cohort studies have associated IgG3 responses with improved outcomes. That IgG1 allotypic diversity does not appear to lead to increased immunogenicity risk and non-native Fcs are now commonly employed in the clinic suggest that a high level of concern about elevated anti-drug antibodies against IgG3 is unwarranted. With the ability to extend the half-life, and produce, purify and manufacture IgG3 on a large scale, fundamental arguments against clinical translation of IgG3-based or IgG3-inspired interventions have been successfully resolved. Further experimental data to support IgG3 as a potent therapeutic antibody in cases where enhance effector function and its altered structural characteristics might provide therapeutic advantages, and results from clinical trials to show efficacy could open the door toward additional development of this subclass as an important tool to treat a spectrum of human diseases.
Disclosures
EFP is an inventor on a patent/application(s) relating to a novel IgG3, is co-founder, CEO, and a shareholder of Grid Therapeutics, a company conducting a phase I clinical trial of a human IgG3. EFP has in place an approved plan for managing any potential conflicts arising from these interests.
Acknowledgments
The authors thank Chanc VanWinkle Orzell for copyediting the manuscript and Dr. Janice Reichert for important content suggestions.
Additional information
Funding
References
- Bruhns P, Iannascoli B, England P, Mancardi DA, Fernandez N, Jorieux S, et al. Specificity and affinity of human Fcgamma receptors and their polymorphic variants for human IgG subclasses. Blood. 2009;113(16):3716–15. doi:10.1182/blood-2008-09-179754.
- Goh YS, Grant AJ, Restif O, McKinley TJ, Armour KL, Clark MR, et al Human IgG isotypes and activating Fcgamma receptors in the interaction of Salmonella enterica serovar Typhimurium with phagocytic cells. Immunology. 2011;133(1):74–83. doi:10.1111/j.1365-2567.2011.03411.x.
- Irani V, Guy AJ, Andrew D, Beeson JG, Ramsland PA, Richards JS. Molecular properties of human IgG subclasses and their implications for designing therapeutic monoclonal antibodies against infectious diseases. Mol Immunol. 2015;67(2):171–82. doi:10.1016/j.molimm.2015.03.255.
- Vidarsson G, Dekkers G, Rispens T. IgG subclasses and allotypes: from structure to effector functions. Front Immunol. 2014;5:520. doi:10.3389/fimmu.2014.00520.
- Ferrante A, Beard LJ, Feldman RG. IgG subclass distribution of antibodies to bacterial and viral antigens. Pediatr Infect Dis J. 1990;9(Supplement):S16–24. doi:10.1097/00006454-199008001-00004.
- Hansen K, Lau AM, Giles K, McDonnell JM, Struwe WB, Sutton BJ, et al. A Mass-Spectrometry-Based Modelling Workflow for Accurate Prediction of IgG Antibody Conformations in the Gas Phase. Angewandte Chemie. 2018;57(52):17194–99. doi:10.1002/anie.201812018.
- Ellison J, Hood L Linkage and sequence homology of two human immunoglobulin gamma heavy chain constant region genes. Proceedings of the National Academy of Sciences of the United States of America 1982; 79: 1984–88.
- Huck S, Fort P, Crawford DH, Lefranc MP, Lefranc G. Sequence of a human immunoglobulin gamma 3 heavy chain constant region gene: comparison with the other human Cγgenes. Nucleic Acids Res. 1986;14(4):1779–89. doi:10.1093/nar/14.4.1779.
- Broug E, Bland-Ward PA, Powell J, Johnson KS. Fab-arm exchange. Nat Biotechnol. 2010;28(2):123–25. author reply 125-126. doi:10.1038/nbt0210-123.
- Barrett DJ, Ayoub EM. IgG2 subclass restriction of antibody to pneumococcal polysaccharides. Clin Exp Immunol. 1986;63:127–34.
- Lilie H, Rudolph R, Buchner J. Association of antibody chains at different stages of folding: prolyl isomerization occurs after formation of quaternary structure. J Mol Biol. 1995;248(1):190–201. doi:10.1006/jmbi.1995.0211.
- Wypych J, Li M, Guo A, Zhang Z, Martinez T, Allen MJ, et al Human IgG2 antibodies display disulfide-mediated structural isoforms. J Biol Chem. 2008;283(23):16194–205. doi:10.1074/jbc.M709987200.
- Lightle S, Aykent S, Lacher N, Mitaksov V, Wells K, Zobel J, et al. Mutations within a human IgG2 antibody form distinct and homogeneous disulfide isomers but do not affect Fc gamma receptor or C1q binding. Protein Science: A Publication of the Protein Society. 2010;19(4):753–62. doi:10.1002/pro.352.
- White AL, Chan HT, French RR, Willoughby J, Mockridge CI, Roghanian A, et al. Human IgG2 antibody disulfide rearrangement in vivo. J Biol Chem. 2008;283(43):29266–72. doi:10.1074/jbc.M804787200.
- White AL, Chan HT, French RR, Willoughby J, Mockridge CI, Roghanian A, et al. Conformation of the human immunoglobulin G2 hinge imparts superagonistic properties to immunostimulatory anticancer antibodies. Cancer Cell. 2015;27(1):138–48. doi:10.1016/j.ccell.2014.11.001.
- Liu X, Zhao Y, Shi H, Zhang Y, Yin X, Liu M, et al. Human immunoglobulin G hinge regulates agonistic anti-CD40 immunostimulatory and antitumour activities through biophysical flexibility. Nat Commun. 2019;10(1):4206. doi:10.1038/s41467-019-12097-6.
- Konitzer JD, Sieron A, Wacker A, Enenkel B. Reformatting Rituximab into Human IgG2 and IgG4 Isotypes Dramatically Improves Apoptosis Induction In Vitro. PLoS One. 2015;10(12):e0145633. doi:10.1371/journal.pone.0145633.
- Hayes JM, Wormald MR, Rudd PM, Davey GP. Fc gamma receptors: glycobiology and therapeutic prospects. J Inflamm Res. 2016;9:209–19. doi:10.2147/JIR.S121233.
- Saphire EO, Parren PW, Pantophlet R, Zwick MB, Morris GM, Rudd PM, et al. Crystal structure of a neutralizing human IGG against HIV-1: a template for vaccine design. Science. 2001;293(5532):1155–59. doi:10.1126/science.1061692.
- Johnson PM, Michaelsen TE, Scopes PM. Conformation of the hinge region and various fragments of human IgG3. Scand J Immunol. 1975;4(2):113–19. doi:10.1111/j.1365-3083.1975.tb02607.x.
- Shakib F. The Human IgG subclasses: molecular analysis of structure, function, and regulation. 1st ed. Oxford: New York, USA: Pergamon Press. 1990. 316. xi
- Roux KH, Strelets L, Brekke OH, Sandlie I, Michaelsen TE. Comparisons of the ability of human IgG3 hinge mutants, IgM, IgE, and IgA2, to form small immune complexes: a role for flexibility and geometry. Journal of Immunology. 1998;161:4083–90.
- Ryazantsev S, Tishchenko V, Vasiliev V, Zav’yalov V, Abramov V. Structure of human myeloma IgG3 Kuc. European Journal of Biochemistry. 1990;190(2):393–99. doi:10.1111/j.1432-1033.1990.tb15588.x.
- Roux KH, Strelets L, Michaelsen TE. Flexibility of human IgG subclasses. Journal of Immunology. 1997;159:3372–82.
- Cavacini LA, Emes CL, Power J, Desharnais FD, Duval M, Montefiori D, et al. Influence of heavy chain constant regions on antigen binding and HIV-1 neutralization by a human monoclonal antibody. Journal of Immunology. 1995;155:3638–44.
- Wang P, Yang X. Neutralization Efficiency Is Greatly Enhanced by Bivalent Binding of an Antibody to Epitopes in the V4 Region and the Membrane-Proximal External Region within One Trimer of Human Immunodeficiency Virus Type 1 Glycoproteins. J Virol. 2010;84(14):7114–23. doi:10.1128/JVI.00545-10.
- Dimmock NJ, Hardy SA. Valency of antibody binding to virions and its determination by surface plasmon resonance. Rev Med Virol. 2004;14(2):123–35. doi:10.1002/rmv.419.
- Scharf O, Golding H, King LR, Eller N, Frazier D, Golding B, et al Immunoglobulin G3 from polyclonal human immunodeficiency virus (HIV) immune globulin is more potent than other subclasses in neutralizing HIV type 1. J Virol. 2001;75(14):6558–65. doi:10.1128/JVI.75.14.6558-6565.2001.
- Kunert R, Wolbank S, Stiegler G, Weik R, Katinger H. Characterization of Molecular Features, Antigen-Binding, and in Vitro Properties of IgG and IgM Variants of 4E10, an Anti-HIV Type 1 Neutralizing Monoclonal Antibody. AIDS Res Hum Retroviruses. 2004;20(7):755–62. doi:10.1089/0889222041524571.
- Kunert R, Steinfellner W, Purtscher M, Assadian A, Katinger H. Stable recombinant expression of the anti HIV-1 monoclonal antibody 2F5 after IgG3/IgG1 subclass switch in CHO cells. Biotechnol Bioeng. 2000;67(1):97–103. doi:10.1002/(SICI)1097-0290(20000105)67:1<97::AID-BIT11>3.0.CO;2-2.
- Cavacini LA, Kuhrt D, Duval M, Mayer K, Posner MR. Binding and neutralization activity of human IgG1 and IgG3 from serum of HIV-infected individuals. AIDS Res Hum Retroviruses. 2003;19(9):785–92. doi:10.1089/088922203769232584.
- Miranda LR, Duval M, Doherty H, Seaman MS, Posner MR, Cavacini LA. The Neutralization Properties of a HIV-Specific Antibody Are Markedly Altered by Glycosylation Events Outside the Antigen-Binding Domain. The Journal of Immunology. 2007;178(11):7132–38. doi:10.4049/jimmunol.178.11.7132.
- Bournazos S, Gazumyan A, Seaman MS, Nussenzweig MC, Ravetch JV. Bispecific Anti-HIV-1 Antibodies with Enhanced Breadth and Potency. Cell. 2016;165(7):1609–20. doi:10.1016/j.cell.2016.04.050.
- Richardson SI, Lambson BE, Crowley AR, Bashirova A, Scheepers C, Garrett N, et al. IgG3 enhances neutralization potency and Fc effector function of an HIV V2-specific broadly neutralizing antibody. PLoS Pathog. 2019;15(12):e1008064. doi:10.1371/journal.ppat.1008064.
- Galimidi RP, Klein JS, Politzer MS, Bai S, Seaman MS, Nussenzweig MC, et al. Intra-spike crosslinking overcomes antibody evasion by HIV-1. Cell. 2015;160(3):433–46. doi:10.1016/j.cell.2015.01.016.
- de Lange GG. Polymorphisms of human immunoglobulins: gm, Am, Em and Km allotypes. Exp Clin Immunogenet. 1989;6:7–17.
- Richardson SI, Lambson BE, Crowley AR, Bashirova A, Scheepers C, Garrett N, et al. Unveiling the Diversity of Immunoglobulin Heavy Constant Gamma (IGHG) Gene Segments in Brazilian Populations Reveals 28 Novel Alleles and Evidence of Gene Conversion and Natural Selection. Front Immunol. 2019;10:1161. doi:10.3389/fimmu.2019.01161.
- Lefranc M-P, Lefranc G. Human Gm, Km, and Am Allotypes and Their Molecular Characterization: A Remarkable Demonstration of Polymorphism. In: Christiansen FT, Tait BD, editors. Immunogenetics: methods and Applications in Clinical Practice. Totowa: NJ, USA: Humana Press; 2012. p. 635–80.
- Huck S, Lefranc G, Lefranc MP. A human immunoglobulin IGHG3 allele (Gmb0,b1,c3,c5,u) with an IGHG4 converted region and three hinge exons. Immunogenetics. 1989;30(4):250–57. doi:10.1007/BF02421328.
- Dard P, Huck S, Frippiat J-P, Lefranc G, Langaney A, Lefranc M-P, et al. The IGHG3 gene shows a structural polymorphism characterized by different hinge lengths: sequence of a new 2-exon hinge gene. Hum Genet. 1996;99(1):138–41. doi:10.1007/s004390050328.
- Dard P, Lefranc M-P, Osipova L, Sanchez-Mazas A. DNA sequence variability of IGHG3 alleles associated to the main G3m haplotypes in human populations. European Journal of Human Genetics. 2001;9(10):765. doi:10.1038/sj.ejhg.5200700.
- Dugoujon JM, Cambon-Thomsen A. Immunoglobulin allotypes (GM and KM) and their interactions with HLA antigens in autoimmune diseases: a review. Autoimmunity. 1995;22(4):245–60. doi:10.3109/08916939508995322.
- Granoff DM, Boies E, Squires J, Pandey JP, Suarez B, Oldfather J, et al. Interactive effect of genes associated with immunoglobulin allotypes and HLA specificities on susceptibility to Haemophilus influenzae disease. J Immunogenet. 1984;11(3–4):181–88. doi:10.1111/j.1744-313X.1984.tb01055.x.
- Ilic V, Milosevic-Jovcic N, Markovic D, Petrovic S, Stefanovic G. A biased Gm haplotype and Gm paraprotein allotype in multiple myeloma suggests a role for the Gm system in myeloma development. Int J Immunogenet. 2007;34(2):119–25. doi:10.1111/j.1744-313X.2007.00673.x.
- Kagnoff MF, Weiss JB, Brown RJ, Lee T, Schanfield MS. Immunoglobulin allotype markers in gluten-sensitive enteropathy. Lancet. 1983;1(8331):952–53. doi:10.1016/S0140-6736(83)92080-9.
- Nakao Y, Matsumoto H, Miyazaki T, Watanabe S, Mukojima T, Kawashima R, et al. Immunoglobulin G heavy-chain allotypes as possible genetic markers for human cancer. J Natl Cancer Inst. 1981;67(1):47–50.
- Pandey JP, Astemborski J, Thomas DL. Epistatic effects of immunoglobulin GM and KM allotypes on outcome of infection with hepatitis C virus. J Virol. 2004;78(9):4561–65. doi:10.1128/JVI.78.9.4561-4565.2004.
- Pandey JP, Goust JM, Salier JP, Fudenberg HH. Immunoglobulin G heavy chain (Gm) allotypes in multiple sclerosis. J Clin Invest. 1981;67(6):1797–800. doi:10.1172/JCI110220.
- Grubb R, Hallberg T, Hammarstrom L, Oxelius VA, Smith CI, Soderstrom R, et al. Correlation between deficiency of immunoglobulin subclass G3 and Gm allotype. Acta Pathol Microbiol Immunol Scand C. 1986;94(5):187–91. doi:10.1111/j.1699-0463.1986.tb02110.x.
- Stapleton NM, Andersen JT, Stemerding AM, Bjarnarson SP, Verheul RC, Gerritsen J, et al. Competition for FcRn-mediated transport gives rise to short half-life of human IgG3 and offers therapeutic potential. Nat Commun. 2011;2(1):599. doi:10.1038/ncomms1608.
- Dechavanne C, Dechavanne S, Sadissou I, Lokossou AG, Alvarado F, Dambrun M, et al. Associations between an IgG3 polymorphism in the binding domain for FcRn, transplacental transfer of malaria-specific IgG3, and protection against Plasmodium falciparum malaria during infancy: A birth cohort study in Benin. PLoS Med. 2017;14(10):e1002403. doi:10.1371/journal.pmed.1002403.
- Rispens T, Davies AM, Ooijevaar-de Heer P, Absalah S, Bende O, Sutton BJ, et al. Dynamics of inter-heavy chain interactions in human immunoglobulin G (IgG) subclasses studied by kinetic Fab arm exchange. J Biol Chem. 2014;289(9):6098–109. doi:10.1074/jbc.M113.541813.
- Natsume A, In M, Takamura H, Nakagawa T, Shimizu Y, Kitajima K, et al. Engineered antibodies of IgG1/IgG3 mixed isotype with enhanced cytotoxic activities. Cancer Res. 2008;68(10):3863–72. doi:10.1158/0008-5472.CAN-07-6297.
- Michaelsen TE, Aase A, Westby C, Sandlie I. Enhancement of complement activation and cytolysis of human IgG3 by deletion of hinge exons. Scand J Immunol. 1990;32(5):517–28. doi:10.1111/j.1365-3083.1990.tb03192.x.
- Sandlie I, Aase A, Westby C, Michaelsen TE. C1q binding to chimeric monoclonal IgG3 antibodies consisting of mouse variable regions and human constant regions with shortened hinge containing 15 to 47 amino acids. Eur J Immunol. 1989;19(9):1599–603. doi:10.1002/eji.1830190912.
- Chu TH, Crowley AR, Backes I, Chang C, Tay M, Broge T, et al. Hinge length contributes to the phagocytic activity of HIV-specific IgG1 and IgG3 antibodies. PLoS Pathog. 2020;16(2):e1008083. doi:10.1371/journal.ppat.1008083.
- Arnold JN, Wormald MR, Sim RB, Rudd PM, Dwek RA. The Impact of Glycosylation on the Biological Function and Structure of Human Immunoglobulins. Annu Rev Immunol. 2007;25(1):21–50. doi:10.1146/annurev.immunol.25.022106.141702.
- Dekkers G, Treffers L, Plomp R, Bentlage AEH, de Boer M, Koeleman CAM, et al. Decoding the Human Immunoglobulin G-Glycan Repertoire Reveals a Spectrum of Fc-Receptor- and Complement-Mediated-Effector Activities. Front Immunol. 2017;8:877. doi:10.3389/fimmu.2017.00877.
- Niwa R, Natsume A, Uehara A, Wakitani M, Iida S, Uchida K, et al. IgG subclass-independent improvement of antibody-dependent cellular cytotoxicity by fucose removal from Asn297-linked oligosaccharides. J Immunol Methods. 2005;306(1–2):151–60. doi:10.1016/j.jim.2005.08.009.
- Sonneveld ME, Koeleman CAM, Plomp HR, Wuhrer M, van der Schoot CE, Vidarsson G. Fc-Glycosylation in Human IgG1 and IgG3 Is Similar for Both Total and Anti-Red-Blood Cell Anti-K Antibodies. Front Immunol. 2018;9:129. doi:10.3389/fimmu.2018.00129.
- Vestrheim AC, Moen A, Egge-Jacobsen W, Bratlie DB, Michaelsen TE. Different glycosylation pattern of human IgG1 and IgG3 antibodies isolated from transiently as well as permanently transfected cell lines. Scand J Immunol. 2013;77(5):419–28. doi:10.1111/sji.12046.
- Mattu TS, Pleass RJ, Willis AC, Kilian M, Wormald MR, Lellouch AC, et al. The glycosylation and structure of human serum IgA1, Fab, and Fc regions and the role of N-glycosylation on Fcalpha receptor interactions. J Biol Chem. 1998;273(4):2260–72. doi:10.1074/jbc.273.4.2260.
- Takahashi N, Tetaert D, Debuire B, Lin LC, Putnam FW. Complete amino acid sequence of the delta heavy chain of human immunoglobulin D. Proc Natl Acad Sci U S A. 1982;79(9):2850–54. doi:10.1073/pnas.79.9.2850.
- Plomp R, Dekkers G, Rombouts Y, Visser R, Koeleman CA, Kammeijer GS, et al. Hinge-Region O-Glycosylation of Human Immunoglobulin G3 (IgG3). Molecular & Cellular Proteomics: MCP. 2015;14(5):1373–84. doi:10.1074/mcp.M114.047381.
- Kim H, Yamaguchi Y, Masuda K, et al. O-glycosylation in hinge region of mouse immunoglobulin G2b. J Biol Chem. 1994;269(16):12345–50. doi:10.1016/S0021-9258(17)32722-9.
- Neuberger MS, Rajewsky K. Activation of mouse complement by monoclonal mouse antibodies. Eur J Immunol. 1981;11(12):1012–16. doi:10.1002/eji.1830111212.
- Klaus GG, Pepys MB, Kitajima K, Askonas BA. Activation of mouse complement by different classes of mouse antibody. Immunology. 1979;38:687–95.
- Michaelsen TE, Kolberg J, Aase A, Herstad TK, Hoiby EA. The four mouse IgG isotypes differ extensively in bactericidal and opsonophagocytic activity when reacting with the P1.16 epitope on the outer membrane PorA protein of Neisseria meningitidis. Scand J Immunol. 2004;59(1):34–39. doi:10.1111/j.0300-9475.2004.01362.x.
- Yuan R, Clynes R, Oh J, Ravetch JV, Scharff MD. Antibody-mediated modulation of Cryptococcus neoformans infection is dependent on distinct Fc receptor functions and IgG subclasses. J Exp Med. 1998;187(4):641–48. doi:10.1084/jem.187.4.641.
- Scinicariello F, Engleman CN, Jayashankar L, McClure HM, Attanasio R. Rhesus macaque antibody molecules: sequences and heterogeneity of alpha and gamma constant regions. Immunology. 2004;111(1):66–74. doi:10.1111/j.1365-2567.2004.01767.x.
- Warncke M, Calzascia T, Coulot M, Balke N, Touil R, Kolbinger F, et al. Different adaptations of IgG effector function in human and nonhuman primates and implications for therapeutic antibody treatment. Journal of Immunology. 2012;188(9):4405–11. doi:10.4049/jimmunol.1200090.
- Attanasio R, Jayashankar L, Engleman CN, Scinicariello F. Baboon immunoglobulin constant region heavy chains: identification of four IGHG genes. Immunogenetics. 2002;54(8):556–61. doi:10.1007/s00251-002-0505-1.
- Giudicelli V, Duroux P, Ginestoux C, Folch G, Jabado-Michaloud J, Chaume D, et al. IMGT/LIGM-DB, the IMGT comprehensive database of immunoglobulin and T cell receptor nucleotide sequences. Nucleic Acids Res. 2006;34(90001):D781–784. doi:10.1093/nar/gkj088.
- Tillib SV, Vyatchanin AS, Muyldermans S. Molecular analysis of heavy chain-only antibodies of Camelus bactrianus. Biochemistry (Mosc). 2014;79(12):1382–90. doi:10.1134/S000629791412013X.
- Hamers-Casterman C, Atarhouch T, Muyldermans S, Robinson G, Hamers C, Songa EB, et al. Naturally occurring antibodies devoid of light chains. Nature. 1993;363(6428):446–48. doi:10.1038/363446a0.
- Daley LP, Kutzler MA, Bennett BW, Smith MC, Glaser AL, Appleton JA. Effector functions of camelid heavy-chain antibodies in immunity to West Nile virus. Clinical and Vaccine Immunology: CVI. 2010;17(2):239–46. doi:10.1128/CVI.00421-09.
- Ramesh A, Darko S, Hua A, Overman G, Ransier A, Francica JR, et al. Structure and Diversity of the Rhesus Macaque Immunoglobulin Loci through Multiple De Novo Genome Assemblies. Front Immunol. 2017;8:1407. doi:10.3389/fimmu.2017.01407.
- Lefranc MP. IMGT, the international ImMunoGeneTics database. Nucleic Acids Res. 2001;29(1):207–09. doi:10.1093/nar/29.1.207.
- D’Addabbo P, Scascitelli M, Giambra V, Rocchi M, Frezza D. Position and sequence conservation in Amniota of polymorphic enhancer HS1.2 within the palindrome of IgH 3ʹRegulatory Region. BMC Evol Biol. 2011;11(1):71. doi:10.1186/1471-2148-11-71.
- Stavnezer J, Guikema JE, Schrader CE. Mechanism and regulation of class switch recombination. Annu Rev Immunol. 2008;26(1):261–92. doi:10.1146/annurev.immunol.26.021607.090248.
- Valenzuela NM, Schaub S. The Biology of IgG Subclasses and Their Clinical Relevance to Transplantation. Transplantation. 2018;102:S7–S13. doi:10.1097/TP.0000000000001816.
- Tangye SG, Ferguson A, Avery DT, Ma CS, Hodgkin PD. Isotype switching by human B cells is division-associated and regulated by cytokines. Journal of Immunology. 2002;169(8):4298–306. doi:10.4049/jimmunol.169.8.4298.
- Santana SS, Silva DA, Vaz LD, Pirovani CP, Barros GB, Lemos EM, et al. Analysis of IgG subclasses (IgG1 and IgG3) to recombinant SAG2A protein from Toxoplasma gondii in sequential serum samples from patients with toxoplasmosis. Immunol Lett. 2012;143(2):193–201. doi:10.1016/j.imlet.2012.02.008.
- Yates NL, Lucas JT, Nolen TL, Vandergrift NA, Soderberg KA, Seaton KE, et al. Multiple HIV-1-specific IgG3 responses decline during acute HIV-1: implications for detection of incident HIV infection. AIDS (London, England). 2011;25(17):2089–97. doi:10.1097/QAD.0b013e32834b348e.
- Dugast A-S, Stamatatos L, Tonelli A, Suscovich TJ, Licht AF, Mikell I, et al. Independent evolution of Fc- and Fab-mediated HIV-1-specific antiviral antibody activity following acute infection. Eur J Immunol. 2014;44(10):2925–37. doi:10.1002/eji.201344305.
- Hussain R, Kifayet A, Chiang TJ. Immunoglobulin G1 (IgG1) and IgG3 antibodies are markers of progressive disease in leprosy. Infect Immun. 1995;63(2):410–15. doi:10.1128/IAI.63.2.410-415.1995.
- Hussain R, Dawood G, Abrar N, Toossi Z, Minai A, Dojki M, et al. Selective increases in antibody isotypes and immunoglobulin G subclass responses to secreted antigens in tuberculosis patients and healthy household contacts of the patients. Clin Diagn Lab Immunol. 1995;2(6):726–32. doi:10.1128/CDLI.2.6.726-732.1995.
- Chung AW, Ghebremichael M, Robinson H, Brown E, Choi I, Lane S, et al. Polyfunctional Fc-effector profiles mediated by IgG subclass selection distinguish RV144 and VAX003 vaccines. Sci Transl Med. 2014;6(228):228ra238. doi:10.1126/scitranslmed.3007736.
- Yates NL, Liao HX, Fong Y, deCamp A, Vandergrift NA, Williams WT, et al. Vaccine-induced Env V1-V2 IgG3 correlates with lower HIV-1 infection risk and declines soon after vaccination. Sci Transl Med. 2014;6(228):228ra239. doi:10.1126/scitranslmed.3007730.
- Karnasuta C, Akapirat S, Madnote S, Savadsuk H, Puangkaew J, Rittiroongrad S, et al. Comparison of Antibody Responses Induced by RV144, VAX003, and VAX004 Vaccination Regimens. AIDS Res Hum Retroviruses. 2017;33(5):410–23. doi:10.1089/aid.2016.0204.
- Brown EP, Dowell KG, Boesch AW, Normandin E, Mahan AE, Chu T, et al. Multiplexed Fc array for evaluation of antigen-specific antibody effector profiles. J Immunol Methods. 2017;443:33–44. doi:10.1016/j.jim.2017.01.010.
- Jackson KJ, Wang Y, Collins AM. Human immunoglobulin classes and subclasses show variability in VDJ gene mutation levels. Immunol Cell Biol. 2014;92(8):729–33. doi:10.1038/icb.2014.44.
- Collins AM, Jackson KJ. A Temporal Model of Human IgE and IgG Antibody Function. Front Immunol. 2013;4:235. doi:10.3389/fimmu.2013.00235.
- van Zelm MC. B cells take their time: sequential IgG class switching over the course of an immune response? Immunol Cell Biol. 2014;92(8):645–46. doi:10.1038/icb.2014.48.
- Kitaura K, Yamashita H, Ayabe H, Shini T, Matsutani T, Suzuki R. Different Somatic Hypermutation Levels among Antibody Subclasses Disclosed by a New Next-Generation Sequencing-Based Antibody Repertoire Analysis. Front Immunol. 2017;8:389. doi:10.3389/fimmu.2017.00389.
- Berkowska MA, Driessen GJ, Bikos V, Grosserichter-Wagener C, Stamatopoulos K, Cerutti A, et al. Human memory B cells originate from three distinct germinal center-dependent and -independent maturation pathways. Blood. 2011;118(8):2150–58. doi:10.1182/blood-2011-04-345579.
- Horns F, Vollmers C, Croote D, Mackey SF, Swan GE, Dekker CL, et al. Lineage tracing of human B cells reveals the in vivo landscape of human antibody class switching. Elife. 2016;5.
- Briere F, Servet-Delprat C, Bridon JM, Saint-Remy JM, Banchereau J. Human interleukin 10 induces naive surface immunoglobulin D+ (sIgD+) B cells to secrete IgG1 and IgG3. J Exp Med. 1994;179(2):757–62. doi:10.1084/jem.179.2.757.
- Fujieda S, Zhang K, Saxon A. IL-4 plus CD40 monoclonal antibody induces human B cells gamma subclass-specific isotype switch: switching to gamma 1, gamma 3, and gamma 4, but not gamma 2. Journal of Immunology. 1995;155:2318–28.
- Zhang L, Ding Z, Heyman B. IgG3-antigen complexes are deposited on follicular dendritic cells in the presence of C1q and C3. Sci Rep. 2017;7(1):5400. doi:10.1038/s41598-017-05704-3.
- Zhang L, Ding Z, Xu H, Heyman B. Marginal zone B cells transport IgG3-immune complexes to splenic follicles. Journal of Immunology. 2014;193(4):1681–89. doi:10.4049/jimmunol.1400331.
- Butler NR, Barr M, Glenny AT. Immunization of young babies against diphtheria. Br Med J. 1954;1(4860):476–81. doi:10.1136/bmj.1.4860.476.
- Osborn JJ, Dancis J, Julia JF. Studies of the immunology of the newborn infant. II. Interference with active immunization by passive transplacental circulating antibody. Pediatrics. 1952;10:328–34.
- Terry WD, Matthews BW, Davies DR. Crystallographic studies of a human immunoglobulin. Nature. 1968;220(5164):239–41. doi:10.1038/220239a0.
- Lai JI, Licht AF, Dugast AS, Suscovich T, Choi I, Bailey-Kellogg C, et al. Divergent antibody subclass and specificity profiles but not protective HLA-B alleles are associated with variable antibody effector function among HIV-1 controllers. J Virol. 2014;88(5):2799–809. doi:10.1128/JVI.03130-13.
- Hjelholt A, Christiansen G, Sorensen US, Birkelund S. IgG subclass profiles in normal human sera of antibodies specific to five kinds of microbial antigens. Pathog Dis. 2013;67(3):206–13. doi:10.1111/2049-632X.12034.
- Tay MZ, Liu P, Williams LD, McRaven MD, Sawant S, Gurley TC, et al. Antibody-Mediated Internalization of Infectious HIV-1 Virions Differs among Antibody Isotypes and Subclasses. PLoS Pathog. 2016;12(8):e1005817. doi:10.1371/journal.ppat.1005817.
- Musich T, Li L, Liu L, Zolla-Pazner S, Robert-Guroff M, Gorny MK. Monoclonal Antibodies Specific for the V2, V3, CD4-Binding Site, and gp41 of HIV-1 Mediate Phagocytosis in a Dose-Dependent Manner. J Virol. 2017;91(8). doi:10.1128/JVI.02325-16.
- Bruggeman CW, Dekkers G, Bentlage AEH, Treffers LW, Nagelkerke SQ, Lissenberg-Thunnissen S, et al. Enhanced Effector Functions Due to Antibody Defucosylation Depend on the Effector Cell Fcgamma Receptor Profile. Journal of Immunology. 2017;199(1):204–11. doi:10.4049/jimmunol.1700116.
- Rosner T, Lohse S, Peipp M, Valerius T, Derer S. Epidermal growth factor receptor targeting IgG3 triggers complement-mediated lysis of decay-accelerating factor expressing tumor cells through the alternative pathway amplification loop. Journal of Immunology. 2014;193(3):1485–95. doi:10.4049/jimmunol.1400329.
- Rosner T, Derer S, Kellner C, Dechant M, Lohse S, Vidarsson G, et al. An IgG3 switch variant of rituximab mediates enhanced complement-dependent cytotoxicity against tumour cells with low CD20 expression levels. Br J Haematol. 2013;161(2):282–86. doi:10.1111/bjh.12209.
- Giuntini S, Granoff DM, Beernink PT, Ihle O, Bratlie D, Michaelsen TE. Human IgG1, IgG3, and IgG3 Hinge-Truncated Mutants Show Different Protection Capabilities against Meningococci Depending on the Target Antigen and Epitope Specificity. Clinical and Vaccine Immunology: CVI. 2016;23(8):698–706. doi:10.1128/CVI.00193-16.
- Kim JH, Park S, Hwang YI, Jang SH, Jung KS, Sim YS, et al. Immunoglobulin G Subclass Deficiencies in Adult Patients with Chronic Airway Diseases. J Korean Med Sci. 2016;31(10):1560–65. doi:10.3346/jkms.2016.31.10.1560.
- Barton JC, Bertoli LF, Barton JC, Acton RT. Selective subnormal IgG3 in 121 adult index patients with frequent or severe bacterial respiratory tract infections. Cell Immunol. 2016;299:50–57. doi:10.1016/j.cellimm.2015.09.004.
- Abrahamian F, Agrawal S, Gupta S. Immunological and clinical profile of adult patients with selective immunoglobulin subclass deficiency: response to intravenous immunoglobulin therapy. Clin Exp Immunol. 2010;159(3):344–50. doi:10.1111/j.1365-2249.2009.04062.x.
- Veskitkul J, Vichyanond P, Pacharn P, Visitsunthorn N, Jirapongsananuruk O. Clinical characteristics of recurrent acute rhinosinusitis in children. Asian Pacific Journal of Allergy and Immunology. 2015;33(4):276–80. doi:10.12932/AP0591.33.4.2015.
- Herrod HG. Follow-up of pediatric patients with recurrent infection and mild serologic immune abnormalities. Annals of Allergy, Asthma & Immunology: Official Publication of the American College of Allergy, Asthma, & Immunology. 1997;79(5):460–64. doi:10.1016/S1081-1206(10)63044-X.
- Bernatowska-Matuszkiewicz E, Pac M, Skopcynska H, Pum M, Eibl MM. Clinical efficacy of intravenous immunoglobulin in patients with severe inflammatory chest disease and IgG3 subclass deficiency. Clin Exp Immunol. 1991;85(2):193–97. doi:10.1111/j.1365-2249.1991.tb05703.x.
- Olinder-Nielsen AM, Granert C, Forsberg P, Friman V, Vietorisz A, Bjorkander J. Immunoglobulin prophylaxis in 350 adults with IgG subclass deficiency and recurrent respiratory tract infections: a long-term follow-up. Scand J Infect Dis. 2007;39(1):44–50. doi:10.1080/00365540600951192.
- Barlan IB, Geha RS, Schneider LC. Therapy for patients with recurrent infections and low serum IgG3 levels. J Allergy Clin Immunol. 1993;92(2):353–55. doi:10.1016/0091-6749(93)90179-J.
- Neidich SD, Fong Y, Li SS, Geraghty DE, Williamson BD, Young WC, et al. Antibody Fc effector functions and IgG3 associate with decreased HIV-1 risk. J Clin Invest. 2019;129(11):4838–49. doi:10.1172/JCI126391.
- Kam YW, Simarmata D, Chow A, Her Z, Teng TS, Ong EK, et al. Early appearance of neutralizing immunoglobulin G3 antibodies is associated with chikungunya virus clearance and long-term clinical protection. J Infect Dis. 2012;205(7):1147–54. doi:10.1093/infdis/jis033.
- Walker MR, Eltahla AA, Mina MM, Li H, Lloyd AR, Bull RA. Envelope-Specific IgG3 and IgG1 Responses Are Associated with Clearance of Acute Hepatitis C Virus Infection. Viruses. 2020;12(1). doi:10.3390/v12010075.
- Taylor RR, Allen SJ, Greenwood BM, Riley EM. IgG3 antibodies to Plasmodium falciparum merozoite surface protein 2 (MSP2): increasing prevalence with age and association with clinical immunity to malaria. Am J Trop Med Hyg. 1998;58(4):406–13. doi:10.4269/ajtmh.1998.58.406.
- Metzger WG, Okenu DMN, Cavanagh DR, Robinson JV, Bojang KA, Weiss HA, McBride JS, Greenwood BM, Conway DJ. Serum IgG3 to the Plasmodium falciparum merozoite surface protein 2 is strongly associated with a reduced prospective risk of malaria. Parasite Immunol. 2003;25(6):307–12. doi:10.1046/j.1365-3024.2003.00636.x.
- Roussilhon C, Oeuvray C, Müller-Graf C, Tall A, Rogier C, Trape J-F, et al. Long-Term Clinical Protection from Falciparum Malaria Is Strongly Associated with IgG3 Antibodies to Merozoite Surface Protein 3. PLoS Med. 2007;4(11):e320. doi:10.1371/journal.pmed.0040320.
- Stanisic DI, Richards JS, McCallum FJ, Michon P, King CL, Schoepflin S, et al. Immunoglobulin G subclass-specific responses against Plasmodium falciparum merozoite antigens are associated with control of parasitemia and protection from symptomatic illness. Infect Immun. 2009;77(3):1165–74. doi:10.1128/IAI.01129-08.
- Lang PO, Mendes A, Socquet J, Assir N, Govind S, Aspinall R. Effectiveness of influenza vaccine in aging and older adults: comprehensive analysis of the evidence. Clin Interv Aging. 2012;7:55–64. doi:10.2147/CIA.S25215.
- Stepanova L, Naykhin A, Kolmskog C, Jonson G, Barantceva I, Bichurina M, et al. The humoral response to live and inactivated influenza vaccines administered alone and in combination to young adults and elderly. Journal of Clinical Virology: The Official Publication of the Pan American Society for Clinical Virology. 2002;24(3):193–201. doi:10.1016/S1386-6532(01)00246-3.
- Frasca D, Diaz A, Romero M, Mendez NV, Landin AM, Blomberg BB. Effects of age on H1N1-specific serum IgG1 and IgG3 levels evaluated during the 2011-2012 influenza vaccine season. Immunity & Ageing: I & A. 2013;10(1):14. doi:10.1186/1742-4933-10-14.
- El-Madhun AS, Cox RJ, Haaheim LR. The effect of age and natural priming on the IgG and IgA subclass responses after parenteral influenza vaccination. J Infect Dis. 1999;180(4):1356–60. doi:10.1086/315003.
- Cao RY, Dong DY, Liu RJ, Han JF, Wang GC, Zhao H, et al. Human IgG subclasses against enterovirus Type 71: neutralization versus antibody dependent enhancement of infection. PLoS One. 2013;8(5):e64024. doi:10.1371/journal.pone.0064024.
- Kuzmina NA, Younan P, Gilchuk P, Santos RI, Flyak AI, Ilinykh PA, et al. Antibody-Dependent Enhancement of Ebola Virus Infection by Human Antibodies Isolated from Survivors. Cell Rep. 2018;24(7):1802–1815 e1805. doi:10.1016/j.celrep.2018.07.035.
- Zimmerman MG, Quicke KM, O'Neal JT, Arora N, Machiah D, Priyamvada L, et al. Cross-Reactive Dengue Virus Antibodies Augment Zika Virus Infection of Human Placental Macrophages. Cell Host Microbe. 2018;24(5):731–742 e736. doi:10.1016/j.chom.2018.10.008.
- Katzelnick LC, Gresh L, Halloran ME, et al.Katzelnick LC, Gresh L, Halloran ME, Mercado JC, Kuan G, Gordon A, et al. Antibody-dependent enhancement of severe dengue disease in humans. Science. 2017;358(6365):929–32. doi:10.1126/science.aan6836.
- Thulin NK, Brewer RC, Sherwood R, Bournazos S, Edwards KG, Ramadoss NS, et al. Maternal Anti-Dengue IgG Fucosylation Predicts Susceptibility to Dengue Disease in Infants. Cell Rep. 2020;31(6):107642. doi:10.1016/j.celrep.2020.107642.
- Wang TT, Sewatanon J, Memoli MJ, Wrammert J, Bournazos S, Bhaumik SK, et al. IgG antibodies to dengue enhanced for FcgammaRIIIA binding determine disease severity. Science. 2017;355(6323):395–98. doi:10.1126/science.aai8128.
- Liu F, Bergami PL, Duval M, Kuhrt D, Posner M, Cavacini L. Expression and functional activity of isotype and subclass switched human monoclonal antibody reactive with the base of the V3 loop of HIV-1 gp120. AIDS Res Hum Retroviruses. 2003;19(7):597–607. doi:10.1089/088922203322230969.
- Carter PJ. Potent antibody therapeutics by design. Nat Rev Immunol. 2006;6(5):343–57. doi:10.1038/nri1837.
- Jefferis R. Antibody therapeutics: isotype and glycoform selection. Expert Opin Biol Ther. 2007;7(9):1401–13. doi:10.1517/14712598.7.9.1401.
- Salfeld JG. Isotype selection in antibody engineering. Nat Biotechnol. 2007;25(12):1369–72. doi:10.1038/nbt1207-1369.
- Jefferis R. Glycosylation as a strategy to improve antibody-based therapeutics. Nat Rev Drug Discov. 2009;8(3):226–34. doi:10.1038/nrd2804.
- Morell A, Terry WD, Waldmann TA. Metabolic properties of IgG subclasses in man. J Clin Invest. 1970;49(4):673–80. doi:10.1172/JCI106279.
- Kronvall G, Williams RC Jr. Differences in anti-protein A activity among IgG subgroups. Journal of Immunology. 1969;103:828–33.
- Gaudinski MR, Coates EE, Houser KV, Chen GL, Yamshchikov G, Saunders JG, et al. Safety and pharmacokinetics of the Fc-modified HIV-1 human monoclonal antibody VRC01LS: A Phase 1 open-label clinical trial in healthy adults. PLoS Med. 2018;15(1):e1002493. doi:10.1371/journal.pmed.1002493.
- Robbie GJ, Criste R, Dall'acqua WF, Jensen K, Patel NK, Losonsky GA, et al. A novel investigational Fc-modified humanized monoclonal antibody, motavizumab-YTE, has an extended half-life in healthy adults. Antimicrob Agents Chemother. 2013;57(12):6147–53. doi:10.1128/AAC.01285-13.
- Zhu Q, McLellan JS, Kallewaard NL, Ulbrandt ND, Palaszynski S, Zhang J, et al. A highly potent extended half-life antibody as a potential RSV vaccine surrogate for all infants. Sci Transl Med. 2017;9(388). doi:10.1126/scitranslmed.aaj1928.
- Webster CI, Bryson CJ, Cloake EA, Jones TD, Austin MJ, Karle AC, et al. A comparison of the ability of the human IgG1 allotypes G1m3 and G1m1,17 to stimulate T-cell responses from allotype matched and mismatched donors. MAbs. 2016;8(2):253–63. doi:10.1080/19420862.2015.1128605.
- Bartelds GM, de Groot E, Nurmohamed MT, et al. Surprising negative association between IgG1 allotype disparity and anti-adalimumab formation: a cohort study. Arthritis Res Ther. 2010;12(6):R221. doi:10.1186/ar3208.
- Bartelds GM, de Groot E, Nurmohamed MT, Hart MH, van Eede PH, Wijbrandts CA, et al. IgG1 heavy chain-coding gene polymorphism (G1m allotypes) and development of antibodies-to-infliximab. Pharmacogenet Genomics. 2009;19(5):383–87. doi:10.1097/FPC.0b013e32832a06bf.
- Shearer MH, Dark RD, Chodosh J, Kennedy RC. Comparison and Characterization of Immunoglobulin G Subclasses among Primate Species. Clinical Diagnostic Laboratory Immunology. 1999;6(6):953–58. doi:10.1128/CDLI.6.6.953-958.1999.
- Van Loghem E, Frangione B, Recht B, Franklin EC. Staphylococcal protein A and human IgG subclasses and allotypes. Scand J Immunol. 1982;15(3):275–78. doi:10.1111/j.1365-3083.1982.tb00649.x.
- Lopez E, Scott NE, Wines BD, Hogarth PM, Wheatley AK, Kent SJ, et al. Low pH Exposure During Immunoglobulin G Purification Methods Results in Aggregates That Avidly Bind Fcγ Receptors: implications for Measuring Fc Dependent Antibody Functions. Front Immunol. 2019;10. doi:10.3389/fimmu.2019.02415
- Saito S, Namisaki H, Hiraishi K, Takahashi N, Iida S. A stable engineered human IgG3 antibody with decreased aggregation during antibody expression and low pH stress. Protein Science: A Publication of the Protein Society. 2019;28(5):900–09. doi:10.1002/pro.3598.
- Ito T, Tsumoto K. Effects of subclass change on the structural stability of chimeric, humanized, and human antibodies under thermal stress. Protein Science: A Publication of the Protein Society. 2013;22(11):1542–51. doi:10.1002/pro.2340.
- Baici A, Knopfel M, Fehr K, Skvaril F, Boni A. Kinetics of the different susceptibilities of the four human immunoglobulin G subclasses to proteolysis by human lysosomal elastase. Scand J Immunol. 1980;12(1):41–50. doi:10.1111/j.1365-3083.1980.tb00039.x.
- Turner MW, Bennich HH, Natvig JB. Simple method of subtyping human G-myeloma proteins based on sensitivity to pepsin digestion. Nature. 1970;225(5235):853–55. doi:10.1038/225853b0.
- Virella G, Parkhouse RM. Papain sensitivity of heavy chain sub-classes in normal human IgG and localizaton of antigenic determinants for the sub-classes. Immunochemistry. 1971;8(3):243–50. doi:10.1016/0019-2791(71)90478-2.
- Fang J, Qian JJ, Yi S, Harding TC, Tu GH, VanRoey M, et al. Stable antibody expression at therapeutic levels using the 2A peptide. Nat Biotechnol. 2005;23(5):584–90. doi:10.1038/nbt1087.
- Ye Z, Hellstrom I, Hayden-Ledbetter M, Dahlin A, Ledbetter JA, Hellstrom KE. Gene therapy for cancer using single-chain Fv fragments specific for 4-1BB. Nat Med. 2002;8(4):343–48. doi:10.1038/nm0402-343.
- Hessell AJ, Shapiro MB, Powell R, Malherbe DC, McBurney SP, Pandey S, et al. Reduced Cell-Associated DNA and Improved Viral Control in Macaques following Passive Transfer of a Single Anti-V2 Monoclonal Antibody and Repeated Simian/Human Immunodeficiency Virus Challenges. J Virol. 2018;92(11). doi:10.1128/JVI.02198-17.
- Braster R, Grewal S, Visser R, Einarsdottir HK, van Egmond M, Vidarsson G, et al. Human IgG3 with extended half-life does not improve Fc-gamma receptor-mediated cancer antibody therapies in mice. PLoS One. 2017;12(5):e0177736. doi:10.1371/journal.pone.0177736.
- Giuntini S, Reason DC, Granoff DM, Weiser JN. Combined roles of human IgG subclass, alternative complement pathway activation, and epitope density in the bactericidal activity of antibodies to meningococcal factor h binding protein. Infect Immun. 2012;80(1):187–94. doi:10.1128/IAI.05956-11.
- Lucisano Valim YM, Lachmann PJ. The effect of antibody isotype and antigenic epitope density on the complement-fixing activity of immune complexes: a systematic study using chimaeric anti-NIP antibodies with human Fc regions. Clin Exp Immunol. 1991;84(1):1–8. doi:10.1111/j.1365-2249.1991.tb08115.x.
- Varshney AK, Kuzmicheva GA, Lin J, Sunley KM, Bowling RA, Jr., Kwan TY, et al. A natural human monoclonal antibody targeting Staphylococcus Protein A protects against Staphylococcus aureus bacteremia. PLoS One. 2018;13(1):e0190537. doi:10.1371/journal.pone.0190537.
- Huynh T, Stecher M, Mckinnon J, Jung N, Rupp ME. Safety and Tolerability of 514G3, a True Human Anti-Protein A Monoclonal Antibody for the Treatment of S. aureus Bacteremia. Open Forum Infectious Diseases. 2016;3(suppl_1). doi:10.1093/ofid/ofw172.1057.
- Campa MJ, Gottlin EB, Bushey RT, Patz EF Jr. Complement Factor H Antibodies from Lung Cancer Patients Induce Complement-Dependent Lysis of Tumor Cells, Suggesting a Novel Immunotherapeutic Strategy. Cancer Immunology Research. 2015;3(12):1325–32. doi:10.1158/2326-6066.CIR-15-0122.
- Natsume A, Shimizu-Yokoyama Y, Satoh M, Shitara K, Niwa R. Engineered anti-CD20 antibodies with enhanced complement-activating capacity mediate potent anti-lymphoma activity. Cancer Sci. 2009;100(12):2411–18. doi:10.1111/j.1349-7006.2009.01327.x.
- Alsaid H, Skedzielewski T, Rambo MV, Hunsinger K, Hoang B, Fieles W, et al. Non invasive imaging assessment of the biodistribution of GSK2849330, an ADCC and CDC optimized anti HER3 mAb, and its role in tumor macrophage recruitment in human tumor-bearing mice. PLoS One. 2017;12(4):e0176075. doi:10.1371/journal.pone.0176075.
- Menke-van der Houven van Oordt CW, McGeoch A, Bergstrom M, McSherry I, Smith DA, Cleveland M, et al. Immuno-PET Imaging to Assess Target Engagement: experience from8 Zr-Anti-HER3 mAb (GSK2849330) in Patients with Solid Tumors. J Nucl Med. 2019;60(7):902–09. doi:10.2967/jnumed.118.214726.
- Drilon A, Somwar R, Mangatt BP, Edgren H, Desmeules P, Ruusulehto A, et al. Response to ERBB3-Directed Targeted Therapy in NRGRearranged Cancers. Cancer Discov. 2018;8(6):686–95. doi:10.1158/2159-8290.CD-17-1004.
- Idusogie EE, Wong PY, Presta LG, Gazzano-Santoro H, Totpal K, Ultsch M, et al. Engineered antibodies with increased activity to recruit complement. Journal of Immunology. 2001;166(4):2571–75. doi:10.4049/jimmunol.166.4.2571.
- Kellner C, Derer S, Valerius T, Boosting PM. ADCC and CDC activity by Fc engineering and evaluation of antibody effector functions. Methods. 2014;65(1):105–13. doi:10.1016/j.ymeth.2013.06.036.
- Moore GL, Chen H, Karki S, Lazar GA. Engineered Fc variant antibodies with enhanced ability to recruit complement and mediate effector functions. MAbs. 2010;2(2):181–89. doi:10.4161/mabs.2.2.11158.
- Klein JS, Jiang S, Galimidi RP, Keeffe JR, Bjorkman PJ. Design and characterization of structured protein linkers with differing flexibilities. Protein Engineering, Design & Selection: PEDS. 2014;27(10):325–30. doi:10.1093/protein/gzu043.