ABSTRACT
Novel engineered IL-2 agonists strive to increase the therapeutic window of aldesleukin (human IL-2) by increasing selectivity toward effector over regulatory T cells and reducing dose-limiting toxicities. Here we describe ANV419, an IL-2/anti-IL2 antibody fusion protein designed for selective IL-2 receptor βγ (IL-2 Rβγ) activation by sterically hindering IL-2 from binding to IL-2 Rα. The fusion protein has an IL-2 connected to the light chain complementarity-determining region (CDR) domain of a humanized antibody that binds to IL-2 at the same epitope as IL-2 Rα. Optimization of the selectivity and pharmacological properties led to the selection of ANV419. ANV419 preferentially expands CD8+ T cells and natural killer (NK) cells over Tregs and can be safely administered at doses that elicit strong pharmacodynamic effects and efficacy in mouse tumor models. Its anti-tumor efficacy was enhanced when combined with programmed cell death protein 1 (PD-1) or cytotoxic T-lymphocyte-associated protein 4 (CTLA-4) checkpoint inhibitors. ANV419 also enhances the NK cell killing capacity and increases tumor growth inhibition when used alongside trastuzumab in a Her-2+ xenograft mouse model. In cynomolgus monkeys, the estimated half-life of ANV419 is 24 h, and doses that induced sustained expansion of effector cells were well tolerated without the severe toxicities typically observed with high-dose IL-2. These data support the clinical development of ANV419 in solid tumors and hematological malignancies as monotherapy and in combination with checkpoint inhibitors or agents that induce antibody-dependent cellular cytotoxicity. ANV419 is currently in Phase 1/2 clinical development and may provide cancer patients with a wider therapeutic window than aldesleukin.
Introduction
Much progress has been made in our understanding of how interleukin-2 (IL-2) regulates lymphocyte signaling, expansion, and activity, particularly in tumor immunology. This has rekindled interest in the application of this pleiotropic cytokine toward enhancing anti-tumor responses for the treatment of cancer. High-dose recombinant IL-2 (Proleukin®/aldesleukin) was the first approved cytokine-based immunotherapy for metastatic melanoma (MM) and renal cell carcinoma (RCC)Citation1,Citation2. Mechanistically, IL-2 expands the population of antigen-experienced effector T cells and natural killer (NK) cells, both of which can recognize and kill tumor cells. Treatment with IL-2 has yielded long-lasting responses in MM and RCC, with ca. 15% of MM patients achieving survival of greater than 10 years upon treatment.Citation3,Citation4 However, the burdensome administration schedule of high-dose IL-2, driven by its short half-life of approximately 1.4 h,Citation5–7 as well as the severe dose-limiting toxicities that include capillary leak syndrome, cardiopulmonary, liver, and renal toxicities, have hampered its widespread application in cancer immunotherapy. Furthermore, high-dose IL-2 not only expands anti-tumor effector cells, but it is also a potent growth factor for immunosuppressive regulatory T cells (Tregs), which generally correlate with poor outcomes for cancer patients.Citation8
IL-2 exerts its effects through signaling via two distinct receptor configurations: the dimeric medium-affinity IL-2 Rβγ composed of the β (CD122) and the common γ chain (CD132) and the trimeric high-affinity IL-2 Rαβγ composed of the α chain (CD25) in addition to the β and γ chains.Citation9 IL-2 and the related cytokine IL-15 have similar functions and share the IL-2 Rβ and γ subunits, but differ in the IL-2 Rα and IL-15 Rα chains.Citation10,Citation11 Upon IL-2 binding to the dimeric as well as to the trimeric receptor, signal transduction is induced through IL-2 Rβ and γ. IL-2 Rα increases binding affinity for IL-2 by 10–100-fold, but does not participate directly in signaling.Citation12
At steady state, IL-2 Rβγ is mainly expressed on naïve and memory CD8+ T cells and NK cells that mediate the anti-tumor responseCitation9 and IL-2 Rαβγ is constitutively expressed on Tregs and pulmonary endothelial cells.Citation13,Citation14 After cellular activation, the expression of the IL-2 Rα chain is also induced on CD4+ and CD8+ T cells, thereby increasing their sensitivity to IL-2. The cellular distribution of IL-2 Rαβγ is thought to reduce anti-tumor efficacy of IL-2 by expanding Tregs and contributes to the dose-limiting toxicity observed upon aldesleukin treatment.Citation14 In the context of cancer, activation of Tregs is associated with inhibition of anti-tumor immune responses, and high infiltration of Tregs within the tumor microenvironment is associated with poor survival in various cancers.Citation8 Due to the constitutive expression of IL-2 Rαβγ, Tregs successfully compete with CD8+ T cells and NK cells for IL-2,Citation9 leading to a dampened immune response against the tumor.Citation15,Citation16
A strategy to overcome the challenges posed by therapeutic use of aldesleukin is the generation of agonists with prolonged half-life that are selective for the IL-2 Rβγ. This strategy has the potential to accentuate the efficacy of IL-2 whilst mitigating the known adverse events, due to lack of IL-2 Rα-mediated binding to vascular endothelial cells.Citation14
In this study, we engineered an IL-2 agonist with selectivity for IL-2 Rβγ, prolonged half-life, moderate toxicity, and the ability to effectively inhibit tumor growth in syngeneic mouse models. Following the concept reported by Sahin et al.,Citation17 a humanized anti-IL-2 antibody permitting the binding of IL-2 to IL-2 Rβγ, but blocking its binding to IL-2 Rα, was generated. The N- and C-termini of the IL-2 molecule were joined, the loop between the IL-2 B and C helices was opened and circularly permutated to the complementarity-determining region (CDR) portion of the antibody. The resulting antibody-cytokine fusion molecule, ANV419, exhibited optimal selectivity and affinity toward the IL-2 Rβγ.
Here, we show that ANV419 exhibits excellent selectivity for effector cells, a good therapeutic window, and an extended half-life. ANV419 also demonstrates efficacy in murine syngeneic cancer models, alone and in combination with checkpoint inhibitors (CPI) or antibody-dependent cellular cytotoxicity (ADCC)-inducing antibody therapeutics.
Materials and methods
Design and optimization of the circularly permutated IL-2 fusion proteins
The mouse-anti human IL-2 antibody NARA1Citation18 was humanized by grafting the mouse CDRs onto a human IgG1 consensus sequence. Nine humanized versions were generated by combining VH1, VH3, VH5 and VK1, VK2, VK3 and tested for binding to IL-2 by ELISA. The three best binders were further optimized for stability and higher affinity to IL-2 by using both random mutagenesis and crystal structureCitation18 guided mutagenesis. Stability was assessed by melting temperature and ELISA binding after storage at 56°C for 2 weeks. The N297A mutation was introduced in the Fc portion of the antibody to reduce effector functions. Two of the optimized, and Fc-silent antibodies were selected and called Antibody A and Antibody B. To generate the candidate antibody-IL-2 fusion proteins, the IL-2 was circularly permuted (IL-2CP) by joining its previous N- and C-termini (P2 and T133) and by opening the peptide chain between K76 and N77. The IL-2CPs’ new N- and C-termini were joined to the antibodies’ LCDR1 at residues Y27d and D30, respectively, with Glycine-Serine linkers. Linkers of lengths varying from 0 to 19 (IL-2CP N-terminus to Y27d) or 1–20 (IL-2CP C-terminus to D30) were tested. Detailed methods describing protein production, affinity measurements, and crystal structure are presented in the Supplemental Material.
pSTAT5 assay on mouse splenocytes
STAT5 phosphorylation was analyzed in murine splenocytes from C57BL/6 mice. The cells were incubated with aldesleukin (Akron Biotech) or IL-2-antibody fusion protein, for 15 min, fixed (Cytofix Buffer, BD Biosciences 554,655) and surface stained for the markers CD25, CD3, and NK1.1. After permeabilization (Perm III buffer, BD Biosciences) staining was performed for FoxP3, pSTAT5 pY694, CD4, and CD8b with the antibodies detailed in the Supplemental Material (Supplementary Table 3). Data acquisition was performed with a Cytek® Aurora spectral flow cytometer and the EC50 values were calculated with GraphPad Prism.
Peripheral blood mononuclear cells isolation
Buffy coats or whole blood from healthy volunteers were received from the blood donation centers Blutspendezentrum SRK beider
Basel or Aarau, Switzerland. All blood donations were collected with informed consent from the donors. Peripheral blood mononuclear cells (PBMCs) were isolated by density gradient centrifugation using Ficoll Paque Plus (GE Healthcare).
pSTAT5 assay on human PBMCs
PBMCs were rested overnight in RPMI + 10% fetal bovine serum (FBS) before stimulation with 10 nM aldesleukin (Akron Biotech), IL-15 (R&D Systems) or ANV419 and fixed with Cytofix buffer for 10 min at 37°C and permeabilized with Perm buffer III (BD Biosciences). The following markers were stained with the antibodies detailed in the Supplemental Materials (Supplementary Tables 4–5): CD3, CD4, CD8, CD25, CD45, CD56, CD127, FoxP3, pSTAT5 pY694. Cells were acquired using a Cytek® Aurora spectral flow cytometer and analyzed with FlowJo™ version 10.7.1.
NK cell receptor expression
NK cells were isolated from freshly isolated PBMCs using the Dynabeads Untouched Human NK cells kit (Invitrogen, 11349D), according to the manufacturer’s protocol. Isolated NK cells were resuspended in AIM-V medium (Gibco) and stimulated with 8 nM ANV419, IL-2, IL-15, or phosphate-buffered saline (PBS) for 18 h at 37°C. After stimulation, the cells were washed and stained for viability (Zombie Aqua™ Fixable Viability Kit, Biolegend) and with surface antibody cocktails for detection of CD2, CD3, CD16 (FcγR3a), CD25, CD45, CD56, CD57, CD69, CD158b, NKG2A/CD159a, CD279/PD-1, CD172a/SIRPα, CD314/NKG2D, CD337/NKp30, CD335/NKp46. Details for the antibodies used can be found in the Supplemental Material (Supplementary Table 6). Before acquisition, the stained NK cells were fixed in 0.5% paraformaldehyde solution (BD Cytofix Fixation Buffer, BD Biosciences). NK cells were acquired with a Cytek® Aurora spectral flow cytometer and analyzed with FlowJo™ version 10.7.1.
NK cell killing and ADCC assays
NK cells were isolated from PBMCs using the Dynabeads Untouched Human NK cells Kit (Invitrogen, 11349D). Isolated cells were resuspended in Ham’s F-12K medium (Gibco) containing 10% FBS (Hyclone) and 50 U/mL penicillin/50 µg/mL streptomycin (Gibco) and stimulated with 10 nM ANV419 or medium alone for 16 h at 37°C. HCC1954 or NCI-H929 cells (ATCC) were labeled with Cytolight Rapid Red dye (Sartorius) and plated at a concentration of 0.5 × 104 (HCC1954) or 1 × 104 (NCI-H929) cells per well. For NCI-H929, the plates were coated with a 0.01% poly-L-ornithine solution (Sigma) before cell seeding. trastuzumab (67 pM, Herceptin, Roche) or daratumumab (7 pM, Darzalex, Janssen Biotech) were added to tumor cells and incubated for 0.5–1 h. NK cells were then co-cultured with the tumor cells at a target:effector ratio of 1:5 for up to 24 h. Dead cells were detected using Annexin V Green (Sartorius) at a final dilution of 1:200. As a positive control, staurosporine (Sigma) was used at a final concentration of 1 µM. Data acquisition was performed on an IncuCyte S×1(Sartorius), using repeat scanning every 2 h over 24 h. Percentages of Annexin V-positive tumor cells were calculated relative to the positive control staurosporine. Raw data were generated as total area per image and total Annexin V-positive tumor cells were normalized to total tumor cell area signal. For statistical analysis, individual treatment groups were compared using a paired one-way ANOVA test.
FcγR SNP typing
Total DNA from 1 × 106 PBMCs was isolated and FcγRIIIa variants 158 V/F SNPs were analyzed as in reference.Citation19 DNA isolation and sequencing were conducted at Microsynth, Balgach, Switzerland.
Mouse pharmacodynamic experiments
Ten-week-old female C57BL/6J mice were injected intravenously (i.v.) with compounds or vehicle (PBS) on Day 0. Whole blood samples were collected on study Days 3, 6 and 9 or 0, 3 and 6. Erythrocytes were lysed with BD Pharmlyse buffer (BD Biosciences), leukocytes were stained for viability with Zombie Aqua™ Fixable Viability Kit (Biolegend) and with a surface antibody cocktail for CD3e, CD4, CD8b, CD25, NK-1.1. After fixation and permeabilization with FoxP3/Transcription Factor Staining Buffer (eBioscience), the samples were stained for Ki67 and FoxP3 (further details are given in the Supplemental Material (Supplementary Table 7). The cells were acquired on a Cytek® Aurora spectral flow cytometer and analyzed with FlowJo™ version 10.7.1.
Syngeneic mouse tumor model efficacy studies
6–8 week-old female BALB/c or C57BL/6 mice were inoculated subcutaneously (s.c.) with 1 × 106 or 2 × 106 H22, 1 × 106 Renca cells, or 2 × 105 B16F10 cells, respectively. When the tumors reached a mean size of approximately 80–100 mm3 the mice were randomized and i.v. treated with vehicle or 0.2 mg/kg ANV419 twice (B16F10) or three times per week (H22, Renca). In the CPI combination studies, anti-PD-1 (clone RMP1–14) or anti-CTLA-4 (clone 9D9) were administered twice per week intraperitoneally (i.p.) at 10 or 1 mg/kg, respectively. The studies were performed at CrownBio, Beijing, China.
Analysis of tumor infiltrating lymphocyte
Renca tumors were harvested on Day 5 after randomization and tumor infiltrating lymphocyte (TILs) were isolated by gentle MACS using the mouse tumor dissociation kit (130-096-730, Miltenyi). TILs were stained for the following markers with the antibodies detailed in the Supplementary Material (Supplementary Table 8): CD3, CD4, CD8, CD19, CD11b, CD25, CD45, CD335 (NKp46), FoxP3, F4/80, PD-1, Ki67, and a fixable viability dye (eFluorTM780, eBiosciences). The cells were acquired on a Cytek® Aurora spectral flow cytometer and analyzed with FlowJo™ version 10.7.1.
NCI-N87 xenograft mouse tumor model
6–7 week-old female BALB/c Nude mice were injected s.c. with 5 × 106 NCI-N87 cells in 0.1 mL PBS with 1:1 Matrigel®. When the tumors reached a mean tumor size of approximately 125 mm3, the mice were randomized and either i.v. treated with 0.2 mg/kg ANV419 QW, or i.p. treated with 7.5 mg/kg trastuzumab or vehicle 2QW (n = 8). The study was performed at WuXi Biologics, China.
Cynomolgus monkey toxicology study
Mauritian cynomolgus macaques were dosed with ANV419 by i.v. injection over 1 min at doses of 0.03, 0.1, 0.3 mg/kg, or vehicle control on Days 1 and 15 of the 29-day study. Comprehensive toxicology, pharmacodynamic, and pharmacokinetic parameters were assessed, including clinical and behavioral observation, body weight, food consumption, blood pressure, electrocardiology, respiration, hematology, clinical pathology, histopathology, serum cytokines, immunophenotyping by flow cytometry, and ANV419 serum pharmacokinetics.
Results
Development of a humanized anti-IL-2 antibody/IL-2 fusion protein with specificity for IL-2 Rβγ
With the aim of designing an optimized antibody-IL-2 fusion protein that specifically stimulates IL-2 Rαβγ expressing cells, the crystal structure of the previously characterized mouse anti-IL-2 clone NARA1 complexed to IL-2Citation18 was used to guide the embedding of IL-2 into the light chain of humanized variants of the NARA1 antibody. To preserve the IL-2 Rα blocking orientation of the antibody relative to IL-2, the IL-2 chain was opened between the alpha-helices αB and αC creating anchoring points to the L-CDR1 of the antibody. The N-terminus and C-terminus of IL-2 before helix αA and after helix αD, respectively, were joined thereby generating a circularly permuted IL-2 ( ).
Figure 1. Design of anti-IL-2 antibody-IL-2 fusion proteins with IL-2 circular permutation, to target IL-2 Rβγ. (a) schematic representation of the strategy used to fuse antibodies A, B or C (shown with respective affinities to IL-2 measured by BLI) to IL-2. The antibody-cytokine fusion protein is represented as a drawing or linear construct from N-terminus to C-terminus. (b) IL-2 (aldesleukin) and IL-2 fusion proteins to antibody A, B and C with different linker lengths (G: glycine, S: serine) characterized for their binding affinity to IL-2 Rα, measured by SPR and EC50 values for STAT5 phosphorylation on NK cells, CD8+ T cells and Treg cells. (c) left: crystal structure of IL-2 (blue) in complex with IL-2 Rα (green). Residues of IL-2 in contact with the IL-2 Rα receptor are highlighted in red (PDB code 2B5I). Right: crystal structure of ANV419 (PDB code 8RY2) with parts of ANV419 VH (dark gray), ANV419 VL (light gray), linkers (black) and permuted IL-2 (blue). IL-2 residues in contact with the CDRs of antibody a are highlighted in red. (d) binding kinetics data of ANV419-fab, recombinant IL-2 or recombinant IL-15 fragment to IL-2 Rα and IL-2 Rβγ complex; association (ka) and dissociation (kd) rate constants; affinity constant (KD); half-life of complex T1/2 (= ln2/kd). BLI, Bio-layer interferometry; CDR, complementarity-determining region; EC50, half-maximal effective concentration; IL-2CP, circularly permuted IL-2; VL, variable domain of the antibody light chain; VH, variable domain of the antibody heavy chain.
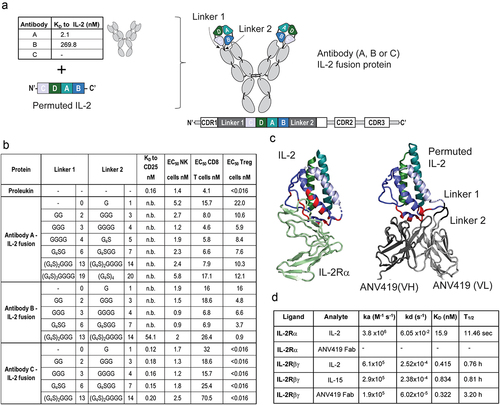
Three antibodies and a set of specific Glycine-Serine linkers were used to generate the candidate fusion proteins: Antibody A binding IL-2 with high affinity (KD 2.1 nM), Antibody B with intermediate affinity (KD 269.8 nM) and Antibody C with no affinity to IL-2. Antibodies A and B were derived from humanization and affinity maturation of the murine NARA1 antibody clone. The antibody-IL-2 fusion proteins were evaluated for competitive binding to the IL-2 Rα recombinant protein by surface plasmon resonance (SPR) analysis (). Fusion proteins to high- or intermediate-affinity IL-2 binding antibodies A and B blocked binding to IL-2 Rα, even with long linkers, and only the linkers of 13/14 amino acids with Antibody B allowed binding of IL-2 Rα. Antibody C did not block the binding of IL-2 Rα to IL-2 with any of the linkers tested. An ELISA competition assay detecting binding to the NARA1 antibodyCitation18 confirmed that increasing linker length allows increased accessibility of the IL-2 Rα-binding epitope on IL-2 (Suplementary ), with the intermediate affinity Antibody B and also with Antibody A when long linkers were used. Accessibility of this epitope poses a risk not only for undesired IL-2Rα binding, but also for compound aggregation in a high concentration formulation through daisy chain formation.
The selectivity of the various fusion proteins for IL-2 Rβγ-expressing cells was compared by measuring the percentage of pSTAT5-positive cells after incubation with mouse splenocytes (). The potency of the Antibody C fusion proteins on Tregs was similar to that of IL-2 (aldesleukin, EC50 of <0.016 nM). Fusion proteins to Antibodies A and B showed markedly decreased potency on Tregs compared to IL-2, indicating preferential stimulation of cells that did not express the IL-2 Rα. EC50 values on CD8+ T cells and NK cells were comparable between the fusion proteins and IL-2.
Fusion proteins where IL-2 was embedded in the variable domain of the heavy chain of Antibody A also showed reduced potency in STAT5 phosphorylation of Tregs, while maintaining effects on CD8+ T cells and NK cells at levels comparable to those of IL-2 (Supplementary Figure 2).
The IL-2 fusion protein to Antibody A with GGG and GGGG linkers, named ANV419, was selected for further study as it showed high selectivity for IL-2 Rβγ expressing cells and low aggregation risk through daisy chain formation. Furthermore, this compound variant avoids G4S-based linkers, where the serines can be sites for difficult to control heterogenous O-glycosylation and O-xylosylation, which can elevate the immunogenicity risk of the therapeutic.Citation20,Citation21,Citation22
The crystal structure of ANV419 was solved and compared to the IL-2 Rα/IL-2 complex (, Supplementary Table 1). Structure alignment illustrated that the binding site of Antibody A to IL-2 (red residues) overlaps with most of the IL-2 residues on the helix αB covered by IL-2 Rα. The structural overlay of ANV419 with the crystal structure of the NARA1 antibody/IL-2 complex (PDB code 5LQB) or the IL-2 R ternary structure (PDB code 2B5I) showed no substantial conformational changes in the circularly permuted IL-2 fused to Antibody A and preserved the binding orientation and interface to the anti-IL-2 antibody (Supplementary Table 2 and Supplementary Figure 3D). This is also reflected in equivalent potency of ANV419 compared to aldesleukin on CD8 and NK cells ().
Figure 2. ANV419 preferentially stimulates CD8+ T cells and NK cells with reduced potency for Treg cells. (a) percentage of phosphorylated STAT5 (pSTAT5)-positive cells out of the parent cell population (CD8+ T cells, NK cells and Treg cells) of mouse splenocytes after 15 min stimulation with a dilution series of IL-2 fusion protein to Antibody C (linker lengths 3, 4), IL-2 (aldesleukin) or ANV419. n = splenocytes from 3 mice, mean ± SEM is shown. (b) percentage of Ki67-positive cells out of the parent population measured in blood from C57BL/6 mice administered with 0.2 mg/kg compound or vehicle control on day 0. n = 3 mice, mean ± SEM (c) percentage of Ki67-positive cells out of the parent population measured in blood from C57BL/6 mice administered with increasing concentrations of ANV419. n = 3 mice, mean ± SEM is shown. NK, natural killer; Treg, regulatory T cells; SEM, standard error of the mean.
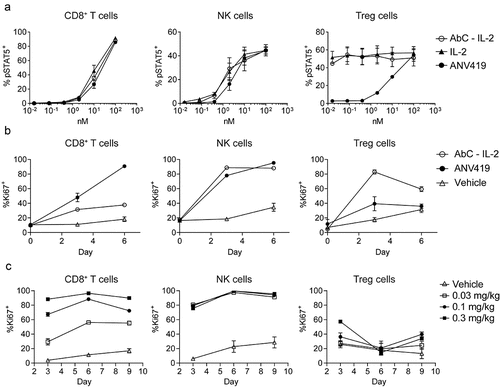
An overlay of the ANV419 structure on the IL-2 ternary complex revealed that the engineered linkage between helices αD and αA of IL-2 stabilizes the former, disordered N- and C-termini of IL-2 in the IL-2 Rα binding pocket (Supplementary Figures 3 and 4). In line with this structural stabilization, ANV419 exhibited a markedly reduced dissociation constant (koff) and longer complex stability (T1/2) with the recombinant IL-2 Rβγ than observed with IL-2 or IL-15 as measured by SPR. However, ANV419 displayed a similar equilibrium dissociation constant (KD) ().
ANV419 selectivity for IL-2 Rβγ expressing cells in vitro and in vivo
In mouse splenocytes stimulated ex vivo, ANV419 had reduced potency in pSTAT5 phosphorylation of Tregs, compared to IL-2 and to the Antibody C fusion protein, but was equipotent to the two control compounds on NK cells and T cells (). In vivo, a single i.v. administration of ANV419 to C57BL/6 mice selectively induced the proliferation (measured by Ki67 staining) of circulating CD8+ T cells and NK cells in a dose-dependent manner compared to vehicle, while having no effect on Tregs up to 0.1 mg/kg and a minimal effect at 0.3 mg/kg (). Administration of the IL-2 fusion protein to Antibody C induced a strong proliferative effect on Tregs and a reduced effect on CD8+ T cells compared to ANV419 ().
ANV419 tumor growth inhibition as monotherapy or in combination with CPI by enhancing CD8+ T cell and NK cell infiltration and expansion
ANV419 was tested in the syngeneic mouse tumor models H22 (hepatic carcinoma), Renca (renal cortical adenocarcinoma), and B16F10 (melanoma), which differ in their TIL immunophenotype and sensitivity to immunotherapy with CPI. H22 hepatic carcinoma tumors responded strongly to monotherapy with ANV419 () and additive tumor growth inhibition (TGI) was observed when ANV419 was combined with PD-1 or CTLA-4 CPIs (). Significant TGI was also achieved in the partially CPI-responsive Renca renal cell carcinoma model with ANV419 monotherapy (), and only limited growth retardation was observed in the poorly immunogenic B16F10 melanoma model (), which was not statistically significant.
Figure 3. ANV419 treatment inhibits tumor growth alone or when combined with CPIs by increasing CD8+ T cells and NK cells in the tumor. Groups of 10–12 mice were s.c. injected with H22 (a – c), Renca (d), or B16F10 (e) tumor cells and randomly assigned to treatment groups on day 0 when the mean tumor size reached ~ 80–100 mm3. Starting on day 1 the mice were i.v. treated with vehicle, 0.2 mg/kg ANV419, 10 mg/kg anti-PD-1, 1 mg/kg anti-CTLA-4, or a combination thereof, twice or three times per week. Tumor volumes were measured three times per week. Tumor volumes mean ± SEM are shown. f-g) groups of 12 mice were s.c. injected with Renca tumor cells, randomized when the tumors reached a mean size of ~ 80–100 mm3, and treated with vehicle or 0.2 mg/kg ANV419 on days 1 and 3. TILs were isolated from the tumors on day 5 and analyzed by flow cytometry. Cell numbers per gram tumor tissue (f) and proliferation as % of Ki67+ from parental cells (g) for CD8+, NK cells and Treg cells. CPIs, checkpoint inhibitors; CTLA-4, cytotoxic T-lymphocyte-associated protein 4; i.v., intravenous; PD-1, programmed death 1; s.c., subcutaneous; SEM, standard error of the mean; TILs, tumor infiltrating lymphocytes. ns: p > 0.05; *: p ≤ 0.05; **: p ≤ 0.01; ***: p ≤ 0.001; ****: p ≤ 0.0001. For A–E statistical analysis was performed for the terminal timepoint; unpaired T-test with Welch’s correction for A, D–G. One-way ANOVA multiple comparison against vehicle control for B and C.
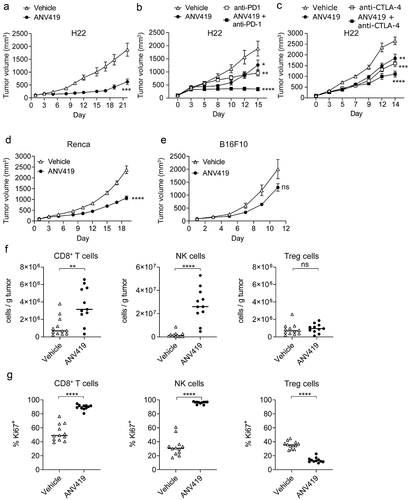
To gain an understanding of the mechanism through which ANV419 achieves tumor growth retardation, we analyzed TILs in Renca tumors by flow cytometry. Treatment with ANV419 significantly enhanced the infiltration of tumors with CD8+ T cells and NK cells (), which correlated with their strong intra-tumoral proliferation as measured by Ki67 staining (). In contrast, as intended by its IL-2 Rβγ selective engineering, the number and proliferation of Tregs was not increased by ANV419 (). Furthermore, an increase was also observed in NKT cells, a cell type that expresses the IL-2 Rβγ, but not in CD4+ T cells which, at steady state, express lower levels of IL-2 Rβ and are less responsive to IL-2,Citation23 and in B cells and macrophages which lack IL-2 R expression entirely (Supplementary Figure 5).
These data provide evidence that ANV419 maintains its selectivity for effector cells at dose levels required for tumor growth inhibition in mice and supports the combination of ANV419 with CPI in a clinical setting.
ANV419 preferentially stimulates human effector cells with IL-2 R signaling potency and kinetics similar to IL-2
To translate the selectivity and potency for signaling through the IL-2 Rβγ to primary human immune cells, ANV419 signaling was assessed on PBMCs. PBMCs were stimulated with ANV419 or IL-2 and after 15 min STAT5 phosphorylation was measured by flow cytometry. CD8+ T cells and NK cells showed similar EC50 values upon stimulation with ANV419 and IL-2 (). In CD4+ T cells and especially in Tregs, reduced IL-2 R-mediated signaling potency was observed upon treatment with ANV419 compared to IL-2 (). In Tregs the EC50 value was reduced by 1500-fold, thus demonstrating ANV419 selectivity for CD8+ T cells and NK cells over Tregs also in human cells.
Figure 4. ANV419 shows selectivity for human CD8+ T cells and NK cells and induces STAT5 phosphorylation at a comparable magnitude and kinetics as recombinant IL-2 and IL-15. (a) PBMCs were stimulated with ANV419 or IL-2 and after 15 min STAT5 phosphorylation was assessed by flow cytometry in CD8+ T cells, NK cells, CD4+ T cells and Tregs. n = 4 donors; mean ± SEM is shown. EC50 values calculated with non-linear regression (curve fit, sigmoidal, 4PL). (b–c) PBMCs from 3 donors were stimulated with 10 nM ANV419, IL-2, IL-15 or left untreated, and STAT5 phosphorylation was measured at the indicated time points in CD8+ T cells and NK cells. B depicts the time course up to 16 min; C depicts the time course from 15 min up to 24 h. NK, natural killer; PBMCs, peripheral blood mononuclear cells. Mean ± SEM is shown.
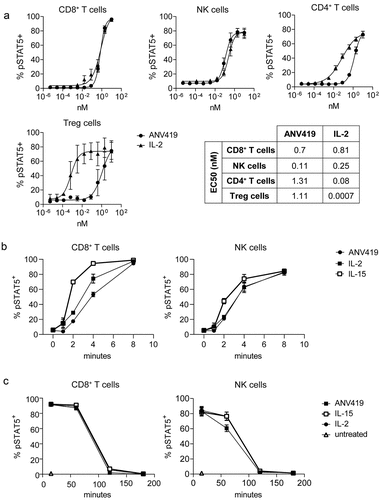
ANV419 is a bivalent IL-2 R agonist as it harbors two IL-2 moieties per molecule and could simultaneously bind and trigger two IL-2 R units. Furthermore, it resembles IL-15 rather than IL-2 in its IL-2 Rβγ-selective binding profile. To test whether these design features affected its IL-2 R signaling kinetics on human CD8+ T cells and NK cells, we measured STAT5 phosphorylation by ANV419 in comparison to IL-2 and IL-15 over time. CD8+ T cells and NK cells stimulated with ANV419 showed comparable pSTAT5 kinetics as with IL-2, while STAT5 phosphorylation tended to occur slightly faster with IL-15 than with ANV419 or IL-2 (). Hence, in CD8+ T cells and NK cells, the IL-2 R signaling potency and kinetics of ANV419 are comparable to IL-2.
ANV419 enhances the activation state of NK cells, but does not alter NK cell receptor surface expression levels
To assess the immunostimulatory effect of ANV419 on NK cells in more detail, human NK cells were isolated from healthy donor PBMCs and incubated with equimolar concentrations of ANV419, IL-2, IL-15, or vehicle control (PBS) for 18 h and analyzed by flow cytometry for changes in the expression levels of activating and inhibitory cell surface receptors, as well as cellular activation markers (Supplementary Figure 6A). Neither the activating NK cell receptors FcγR3a (CD16), NKG2D, NKp46, NKp30 nor the inhibitory NK cell receptors PD1, NKG2A, SIRPα and CD158b showed a striking difference in expression level upon treatment with ANV419. In contrast, the expression of the activation marker CD25 (IL-2 Rα) was increased upon incubation with ANV419 and IL-15, but not upon incubation with IL-2. The lack of surface upregulation of IL-2 Rα in IL-2-treated samples in this assay is likely explained by continued co-internalization of IL-2 Rα with IL-2 Rβγ upon binding of IL-2 to the trimeric receptor,Citation24 but not with ANV419 or IL-15, as they only bind to the dimeric IL-2 Rβγ. The activation marker CD69 was upregulated to a comparable extent upon treatment with all three compounds. CD57, CD45, and CD2 served as controls for which no change in expression was expected. Thus, treatment of NK cells with ANV419, IL-2, and IL-15 did not lead to a substantial change in the expression level of the assessed activating and inhibitory receptors but led to a higher activation state of the NK cells.
ANV419 increases NK cell killing capacity and ADCC
As NK cells showed an activated phenotype upon stimulation with ANV419, we assessed whether this translated into improved effector functions. Interestingly, NK cells treated with ANV419 showed elevated killing of NCI-H929 myeloma () and HCC1954 epithelial breast cancer () target cells. Next, ANV419 was combined with the CD38-specific antibody daratumumab or with the Her2-specific antibody trastuzumab, both of which can act through ADCC. The incubation of NK cells with ANV419 in combination with daratumumab or trastuzumab significantly improved the activity of daratumumab or trastuzumab ().
Figure 5. ANV419 increases NK cell killing capacity in vitro and enhances antibody-mediated tumor growth retardation in vivo. (a) NK cells purified from healthy donor PBMCs were incubated with or without ANV419 and co-cultured with NCI-H929 target cells in the presence or absence of daratumumab. n = 14 donors. (b) NK cells purified from healthy donor PBMCs were incubated with or without ANV419 and co-cultured with HER2+ HCC1954 target cells in the presence or absence of trastuzumab. n = 10 donors. A–B) annexin V positive cells as a percentage of the signal measured with the positive control (staurosporine). Mean ± SEM is shown. Statistical significance was determined by paired one-way ANOVA test. ns: p > 0.05; *: p ≤ 0.05; **: p ≤ 0.01; ***: p ≤ 0.001; ****: p ≤ 0.0001. (c) BALB/c nude mice with s.c. NCI-N87 tumors were i.v. treated with vehicle, 7.5 mg/kg trastuzumab twice per week (BIW), 0.22 mg/kg ANV419 once a week or a combination of trastuzumab and ANV419. n = 8 mice; mean ± SEM is shown; *p ≤ 0.05, **p ≤ 0.01 on Day 40. One-way ANOVA multiple comparison.
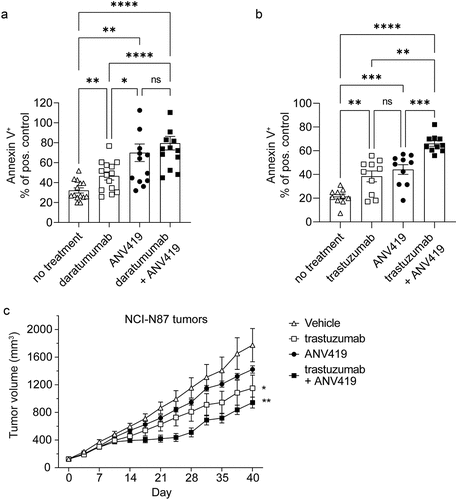
It has been described that patients with the FcγR3a genotype 158 V/V derive greater benefit from trastuzumab than patients who express the low-affinity allele 158F/F.Citation25,Citation26 In contrast to trastuzumab treatment alone, which showed reduced killing by NK cells expressing FcγR3a 158F/F, ANV419 in combination with trastuzumab led to increased NK killing independent of the FcγR3a genotype (Supplementary Figure 6B).
We also assessed the combination of ANV419 with trastuzumab in the NCI-N87 xenograft nude mouse model (which lacks T cells). NCI-N87 is a human gastric adenocarcinoma cell line expressing HER2. ANV419 treatment as well as trastuzumab treatment had single-agent activity and showed additive effects in inhibiting tumor growth in this model (). The data above provide important preclinical evidence that the combination of ANV419 with ADCC-inducing antibody therapeutics may have potential clinical merit and could be beneficial for all patients independent of their FcγR3a genotype.
Favorable toxicology profile at pharmacologically relevant ANV419 doses in cynomolgus monkeys
The ANV419 pharmacology and toxicology was investigated in cynomolgus monkeys in a 4-week GLP study at doses of 0.03, 0.1, and 0.3 mg/kg (Days 1 and 15). The pharmacokinetic profile of ANV419 after i.v. injection was multi-phasic and was characterized by target-mediated drug disposition (TMDD) at doses up to 0.1 mg/kg (). The non-linear kinetics were approached by a single-compartmental model using the Michaelis–Menten approximation. Using this approximation, the central volume of distribution was estimated at 0.124 L, and the clearance at 0.00366 L/h. This allowed the estimation of the half-life of ANV419, in the absence of TMDD or at target saturation, of approximately 24 h.
Figure 6. ANV419 has an extended half-life and promotes the proliferation of CD8+ T cells and NK cells in cynomolgus monkeys. ANV419 was administered i.v. At 0.03 mg/kg, 0.1 mg/kg, or 0.3 mg/kg on days 1 and 15. a) the pharmacokinetic profile of ANV419 was measured in serum after the first (day 1) and second dose (day 15). b) the time course of CD8+ T cell, NK and Treg expansion (cell count/μL) and proliferation (Ki67) was determined by immunophenotyping of whole blood samples pre-dose (days 1 and 15) and after ANV419 i.v. administration. Top: CD8+ T cell, NK cell and Treg cell count/μL blood. Bottom: % Ki67+ cells from parental population for CD8+ T cells, NK cells and Treg cells. n = 6 animals (3 males, 3 females) per group, mean ± SEM is shown.
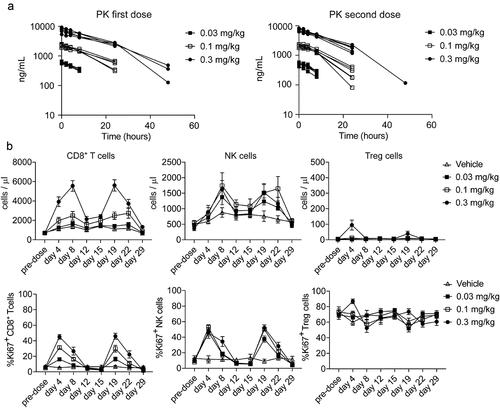
Administration of ANV419 every 2 weeks resulted in a dose-dependent increase in circulating CD8+ T cells up to 10-fold, and NK cells up to 6-fold, with peak cell counts observed on Day 8 after the first injection and at similar levels on Day 19 after the second injection. The increased CD8+ T cell and NK cell counts reverted close to baseline levels before the second injection. Tregs showed a more transient increase only at Day 4 at the highest dose tested, which was less pronounced on Day 19 after the second injection (). Proliferation of CD8+ T cells, as measured by Ki67 positivity, increased with increasing dose levels, while NK cells were more sensitive to ANV419 treatment with near maximal proliferation already observed at the starting dose. For Tregs no dose-response in proliferation levels could be observed due to the high Ki67 positivity already seen at baseline (). This sustained preferential expansion of anti-tumor effector cells over Tregs is in line with the intended IL-2 Rβγ-directed design of ANV419.
Consistent with its pharmacological mechanism of action, ANV419 induced a mild dose-dependent systemic inflammatory response. An induction of anti-inflammatory feedback loops was observed, mainly at the highest dose level, through upregulation of IL-1Ra at 4 h and IL10 at 24 h. The inflammatory serum marker C-reactive protein (CRP) was induced at 72 h post-injection with an attenuated increase after the second injection of ANV419 (). No increase in serum levels of the pro-inflammatory cytokines IFNγ, TNFα, IL-1β, IL-5, IL-6, IL-8, or granulocyte macrophage colony-stimulating factor (GM-CSF) was detectable at 4 h, 24 h, or 72 h (Supplementary Figure 7). Paralleling the inflammatory signal, a transient non-adverse reduction in platelet counts was observed after each injection (), with a milder reduction after the second injection. Contrary to the toxicities usually seen with high-dose IL-2, no evidence of capillary leak syndrome was observed as blood pressure remained stable, and no fluid accumulation in tissues was observed. Furthermore, the eosinophil counts remained within the normal range for cynomolgus monkeys and there was no evidence of eosinophilia (). Overall, the findings of the GLP study suggest that injection of ANV419 at a schedule of every 2 weeks induces the intended levels of expansion of CD8+ T cells and NK cells, without triggering the significant adverse effects of high-dose IL-2.
Figure 7. ANV419 induces a transient dose-dependent inflammatory response. cynomolgus monkeys were injected with ANV419 i.v. On days 1 and 15. a) CRP, IL-1Ra and IL-10 serum levels. CRP samples were collected from pre-dose to Day 29. IL-1Ra and IL-10 data samples were taken at first dose pre-dose, 4 h, 24 h and 72 h. b) platelet and eosinophil counts in whole blood. Normal range for eosinophils observed at Charles River Laboratories in cynomolgus monkeys c) body weight (in kg) and blood pressure (diastolic and systolic) over the study period. n = 6 (3 males and 3 females) per group, mean ± SEM is shown. CRP, C-reactive protein.
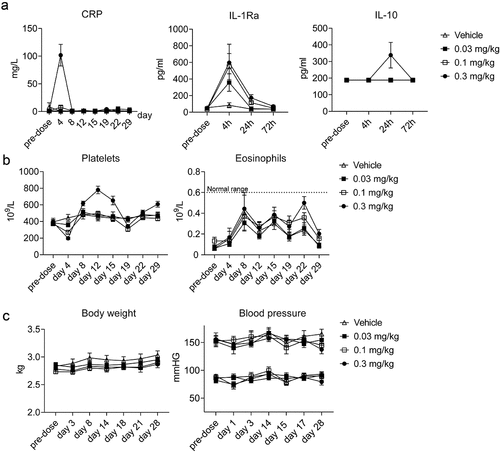
Discussion
When first approved by the US Food and Drug Administration in 1992, recombinant IL-2 (Proleukin®) showed great promise for the treatment of cancer. It is, however, no longer in widespread clinical use largely due to its comparatively moderate efficacy, which must be balanced against its high toxicity and burdensome administration schedule. Various approachesCitation27,Citation28 have been taken to enhance the anti-tumor activity of IL-2 and increase its therapeutic window. Strategies include mutation of IL-2, pegylation, fusion to decoy IL-2 Rα or half-life extension domains, and generation of multi-specific antibodies or fully synthetic peptide IL-2 receptor agonists, with the intent of reducing IL-2 binding to the IL-2 Rα receptor subunit and thereby improving the selectivity for cytotoxic effector T cells and NK cells and extend its half-life.Citation27,Citation28 Approaches to reduce the toxicity of recombinant IL-2 include attenuating mutations in the IL-2 Rβ binding domain of IL-2,Citation29 and tumor-specific protease-activated molecules that have been designed to release active IL-2 only in the tumor microenvironment,Citation30–32 thereby reducing systemic exposure. However, these approaches have been limited by insufficient potency,Citation33,Citation34 incomplete selectivity for IL-2 Rβγ,Citation35 or significant adverse effects.Citation36,Citation37
In this study, we endeavored to circumvent the drawbacks of recombinant IL-2 through a protein engineering approach. Our approach is based on the fusion of IL-2 to the high affinity anti-IL-2 Antibody A, via a circular permutation strategy, which retains the antigen/antibody interactions and still allows binding and activation of IL-2 Rβγ. A related approach has been reported for covalently linked human IL2/NARA1 complexes.Citation17
By binding to IL-2 at the precise site where IL-2 Rα also binds, Antibody A sterically hinders binding of the circularly permutated IL-2 to IL-2 Rα. As a result, ANV419 exhibits no detectable binding to IL-2 Rα but retains binding activity similar to IL-2 (aldesleukin) for IL-2 Rβγ. We examined the role of linker length and antibody affinity for their effect on selective signaling by the fusion protein. High-affinity antibody fusions maintain selectivity over a wide range of linker lengths, whereas no-affinity antibody fusions are unselective even with very short linkers (). Taken together with the crystal structure results, the data indicate that high-affinity antibody/antigen interactions are key in maintaining the signaling selectivity of the fusion protein.
In PBMC, the signaling kinetics in ANV419-stimulated CD8+ T cells or NK cells are comparable to recombinant IL-2 and IL-15. Thus, through its receptor binding and signaling properties, ANV419 achieves highly selective IL-2 signaling on CD8+ T cells and NK cells with 1500-fold reduced potency on Tregs. This selective signaling profile translates into the preferential proliferation of CD8+ T cells and NK cells in mice and cynomolgus monkeys treated with ANV419. High selectivity for anti-tumor effector cells may be critical for enhancing the clinical efficacy of IL-2 therapeutics. Clinical findings with pegylated IL-2 (bempegaldesleukin) suggest that significant expansion of Tregs may have contributed to its failure in the Phase 3 study PIVOT IO-001.Citation38 Consistent with its intended design, ANV419 expands CD8+ T cells and NK cells in mouse syngeneic tumor models and reduces tumor growth, particularly in CPI-sensitive models. With its ability to expand anti-tumor effector cells, ANV419 is well-suited for use in combination with complementary cancer immunotherapy regimens. To this end, we tested ANV419 in combination with the CPI PD-1 and CTLA-4 and observed that ANV419 further enhanced the anti-tumor responses compared to treatment with either CPI alone.
Given the observation that ANV419 significantly stimulated the proliferation of NK cells and enhanced their infiltration into tumors in the mouse experiments, we investigated in greater detail the effects of ANV419 treatment on these cells. Whilst in vitro stimulation with ANV419 only induces minimal changes in the activating NK cell receptor profiles, it significantly increased their killing capacity. When combined with ADCC-inducing antibodies, the cytotoxic capacity of ANV419-stimulated NK cells is strongly enhanced, particularly in donors that expressed the FcγR3a of the low-affinity 158F/F genotype. This in vitro finding was corroborated in the Her2-expressing NCI-N87 xenograft nude mouse model, which lacks T cells. In this model, ANV419 enhanced tumor growth inhibition by trastuzumab, suggesting that ANV419 and ADCC-competent therapeutic antibodies may be a combination worth exploring in the clinical setting.
Data from experiments in the cynomolgus monkey indicate that the half-life of ANV419, estimated at approximately 24 h, is in the range observed for a GD2-targeted IL-2 antibody fusion protein (25 h in cynomolgus monkeys),Citation39 and is significantly longer than the 1.4 h reported for high-dose IL-2 in human patientsCitation7, but shorter than the half-life typically observed for IgG antibodies. It is unclear why IL-2 fusion to an antibody or other half-life extension domains such as albumin does not extend the compound half-life beyond 1–2 days. It is conceivable that the IL-2 domain alters compound recycling and catabolism beyond target-mediated disposition, as was observed for the GD2 targeted IL-239, thereby limiting the half-life achievable through such approaches.
In line with its extended half-life, a single injection of ANV419 induced an extended proliferative burst, leading to an increase in circulating CD8+ T cells and NK cells to therapeutically relevant levels as observed with high-dose IL-2 treatmentCitation15 and which remained elevated for approximately 2 weeks. This favorable pharmacokinetic/pharmacodynamic profile supports a dosing schedule of every 2 weeks in patients, which would significantly alleviate the patient burden compared to treatment regimens with high-dose IL-2Citation7 and other IL-2 receptor agonists with short half-lives requiring daily administration to achieve similar pharmacodynamic responses.Citation15 The sustained expansion of effector cells observed in the cynomolgus monkey experiments was well tolerated and was not accompanied by the severe vascular leak syndrome and excessive eosinophil expansion typically observed with high-dose IL-2.
Taken together, the data presented here provide the rationale for the clinical development of ANV419 in solid tumors and hematological malignancies as a monotherapy as well as in combination with checkpoint inhibitors or ADCC-inducing treatments. A multi-part Phase 1 dose-escalation study of ANV419 is ongoing in solid cancer patients (NCT04855929) and the early clinical Phase 1 data suggest that the intended preferential stimulation of CD8+ T cells and NK cells, as well as the tolerability profile translates to cancer patients.Citation40 As expected from the in vitro selectivity of ANV419 for CD8+ T cell signaling (), the clinical data show strong increases in proliferating CD8+ T cells and NK cells with markedly reduced Treg proliferation in peripheral blood relative to proleukinCitation15 or bempegaldesleukin.Citation41 A Phase 1/2 study in metastatic melanoma (NCT05578872) has been initiated.
Abbreviation
ADCC | = | Antibody-dependent cellular cytotoxicity |
CDR | = | Complementarity-determining region |
CPI | = | Checkpoint inhibitor |
CRP | = | C-reactive protein |
CTLA-4 | = | Cytotoxic T-lymphocyte-associated protein 4 |
EC50 | = | Half maximal effective concentration |
FcγR | = | Fragment crystallizable gamma receptor |
FBS | = | Fetal bovine serum |
IL-2 | = | Interleukin 2 |
IL-2CP | = | Circularly permuted IL-2 |
IL-2 R | = | IL-2 receptor |
i.v. | = | intravenous |
MM | = | Metastatic melanoma |
NK cells | = | Natural killer cells |
PBMCs | = | Peripheral blood mononuclear cells |
PD-1 | = | Programmed cell death protein 1 |
pSTAT5 | = | Phosphorylated STAT5 |
RCC | = | Renal cell carcinoma |
s.c. | = | subcutaneous |
SPR | = | Surface plasmon resonance |
TGI | = | Tumor growth inhibition |
TIL | = | Tumor infiltrating lymphocyte |
VL | = | Immunoglobulin light chain variable region |
VH | = | Immunoglobulin heavy chain variable region |
Authors’ contributions
Barbara Brannetti developed the circularly permuted fusion protein concept and designed ANV419, Patrizia Murer, Kirsten Richter, Andreas Katopodis, Catherine Regnier and Christoph Huber designed the study, Jean-Michel Rondeau generated the crystal structure of ANV419, Simone Popp and Kirsten Richter developed methodology. Research was performed by Patrizia Murer, Laetitia Petersen and Nicole Egli. Patrizia Murer, Kirsten Richter, Christoph Huber and Andreas Katopodis analyzed the data and wrote the manuscript with input from all authors.
Authors’ disclosures
This study was funded by Anaveon AG, Basel, Switzerland and the Novartis Institutes of Biomedical Research, Basel, Switzerland.
PM, LP, NE, KR, AK, and CH were employees of Anaveon AG during the conduct of this study. BB, J-MR, SP and CR were employees of Novartis during the conduct of this study. AK is a co-founder and shareholder of Anaveon AG. PM, LP, NE, KR and CH have received employee options in Anaveon AG.
Ethics approval
All animal experiments carried out at Anaveon were approved by the Cantonal Veterinary Office and performed in accordance with the Swiss laws for animal welfare and protection and the “Ethical Principles and Guidelines for Experiments on Animals by the Swiss Academies of Arts and Sciences”.
The animal studies performed by Wuxi Biologics or CrownBio were approved by the respective Institutional Animal Care and Use Committees (IACUC).
The non-human primate (NHP) study was performed at Charles River Laboratories, France, and the study protocol was approved by the institution’s registered ethics committee. The study was performed in accordance with the OECD Principles of Good Laboratory Practice at an AAALAC-accredited laboratory.
For studies with buffy coats or whole blood from healthy human donors, sample life-cycle management and record keeping, including planning, acquisition, collection, storage, release for use, anonymization and destruction was performed in compliance with the Swiss Human Research Act (HRA; May 2021) and other applicable ethics regulations by the Swiss ethics committee. All blood donations were collected with informed consent from the donors.
Synopsis
ANV419 is a novel IL-2 cytokine-antibody fusion protein with selective affinity for IL-2 Rβγ. For the immunotherapy of cancer ANV419 preferentially stimulates CD8+ T and NK cells over Tregs, thereby promoting anti-tumor actions whilst avoiding Treg-mediated immunosuppression.
KMAB-2024-0047R2_Supp M_M and tables.docx
Download MS Word (47.6 KB)KMAB-2024-0047R2_Supp Figures.docx
Download MS Word (3.3 MB)Acknowledgments
We would like to thank Iwan Beuvink for affinity maturation of the humanized antibody, Emmanuelle Wirth for crystalizing ANV419 and Daniel Kaiser for technical support in assays. We also thank Christoph Bucher, Onur Boyman, and Jennifer Sims for useful discussions.
Supplementary material
Supplemental data for this article can be accessed online at https://doi.org/10.1080/19420862.2024.2381891
Disclosure statement
The study was funded by Anaveon AG. PM, LP, NE, KR, AK, and CH were employees of Anaveon AG during the conduct of this study. BB, J-MR, SP and CR were employees of Novartis during the conduct of this study. AK is a co-founder and shareholder of Anaveon AG. PM, LP, NE, KR and CH have received employee options in Anaveon AG.
Data availability statement
All data relevant to the study are included in the article or uploaded as supplementary information. Diffraction data and crystallographic coordinates for ANV419 have been deposited with the PDB code
Additional information
Funding
References
- Häffner AC, Garbe C, Burg G, Büttner P, Orfanos CE, Rassner G, The prognosis of primary and metastasising melanoma. An evaluation of the TNM classification in 2,495 patients. Br J Cancer. 1992;66(5):856–15. doi:https://doi.org/10.1038/bjc.1992.373.
- Smith FO, Downey SG, Klapper JA, Yang JC, Sherry RM, Royal RE, Kammula US, Hughes MS, Restifo NP, Levy CL, et al. Treatment of metastatic melanoma using interleukin-2 alone or in conjunction with vaccines. Clin Cancer Res. 2008;14(17):5610–5618. doi:https://doi.org/10.1158/1078-0432.Ccr-08-0116.
- Atkins MB, Lotze MT, Dutcher JP, Fisher RI, Weiss G, Margolin K, Abrams J, Sznol M, Parkinson D, Hawkins M, et al. High-dose recombinant interleukin 2 therapy for patients with metastatic melanoma: analysis of 270 patients treated between 1985 and 1993. J Clin Oncol. 1999;17(7):2105–2116. doi:https://doi.org/10.1200/jco.1999.17.7.2105.
- Rosenberg SA, Yang JC, Topalian SL, Schwartzentruber DJ, Weber JS, Parkinson DR, Seipp CA, Einhorn JH, White DE, Treatment of 283 consecutive patients with metastatic melanoma or renal cell cancer using high-dose bolus interleukin 2. Jama. 1994;271(12):907–913. doi:10.1001/jama.1994.03510360033032.
- Jeal W, Goa KL. Aldesleukin (recombinant interleukin-2): a review of its pharmacological properties, clinical efficacy and tolerability in patients with renal cell carcinoma. BioDrugs. 1997;7(4):285–317. doi:https://doi.org/10.2165/00063030-199707040-00005.
- Kirchner GI, Franzke A, Buer J, Beil W, Probst‐Kepper M, Wittke F, Övermann K, Lassmann S, Hoffmann R, Kirchner H, et al. Pharmacokinetics of recombinant human interleukin-2 in advanced renal cell carcinoma patients following subcutaneous application. Br J Clin Pharmacol. 1998;46(1):5–10. doi:https://doi.org/10.1046/j.1365-2125.1998.00036.x.
- Proleukin injection label. [Accessed 2023 May]. https://www.accessdata.fda.gov/drugsatfda_docs/label/2012/103293s5130lbl.pdf.
- Ohue Y, Nishikawa H. Regulatory T (treg) cells in cancer: can treg cells be a new therapeutic target? Cancer Sci. 2019;110(7):2080–2089. doi:https://doi.org/10.1111/cas.14069.
- Malek TR, Castro I. Interleukin-2 receptor signaling: at the interface between tolerance and immunity. Immunity. 2010;33(2):153–65. doi:https://doi.org/10.1016/j.immuni.2010.08.004.
- Ring AM, Lin J-X, Feng D, Mitra S, Rickert M, Bowman GR, Pande VS, Li P, Moraga I, Spolski R, et al. Mechanistic and structural insight into the functional dichotomy between IL-2 and IL-15. Nat Immunol. 2012;13(12):1187–1195. doi:https://doi.org/10.1038/ni.2449.
- Waldmann TA. The shared and contrasting roles of IL2 and IL15 in the life and death of normal and neoplastic lymphocytes: implications for cancer therapy. Cancer Immunol Res. 2015;3(3):219–227. doi:https://doi.org/10.1158/2326-6066.Cir-15-0009.
- Spolski R, Li P, Leonard WJ. Biology and regulation of IL-2: from molecular mechanisms to human therapy. Nat Rev Immunol. 2018;18(10):648–659. doi:https://doi.org/10.1038/s41577-018-0046-y.
- Arumugam P, Carroll KL, Berceli SA, Barnhill S, Wrenshall LE, Expression of a functional IL-2 receptor in vascular smooth muscle cells. J Immunol. 2019;202(3):694–703. doi:https://doi.org/10.4049/jimmunol.1701151.
- Krieg C, Létourneau S, Pantaleo G, Boyman O, Improved IL-2 immunotherapy by selective stimulation of IL-2 receptors on lymphocytes and endothelial cells. Proc Natl Acad Sci USA. 2010;107(26):11906–11. doi:https://doi.org/10.1073/pnas.1002569107.
- Ahmadzadeh M, Rosenberg SA, IL-2 administration increases CD4+ CD25(hi) Foxp3+ regulatory T cells in cancer patients. Blood. 2006;107(6):2409–2414. doi:https://doi.org/10.1182/blood-2005-06-2399.
- Sim GC, Martin-Orozco N, Jin L, Yang Y, Wu S, Washington E, Sanders D, Lacey C, Wang Y, Vence L, et al. IL-2 therapy promotes suppressive ICOS+ treg expansion in melanoma patients. J Clin Invest. 2014;124(1):99–110. doi:https://doi.org/10.1172/jci46266.
- Sahin D, Arenas-Ramirez N, Rath M, Karakus U, Hümbelin M, van Gogh M, Borsig L, Boyman O. An IL-2-grafted antibody immunotherapy with potent efficacy against metastatic cancer. Nat Commun. 2020;11(1):6440. doi:https://doi.org/10.1038/s41467-020-20220-1.
- Arenas-Ramirez N, Zou C, Popp S, Zingg D, Brannetti B, Wirth E, Calzascia T, Kovarik J, Sommer L, Zenke G, et al. Improved cancer immunotherapy by a CD25-mimobody conferring selectivity to human interleukin-2. Sci Transl Med. 2016;8(367):367ra166. doi:https://doi.org/10.1126/scitranslmed.aag3187.
- Keller CW, Ruck T, McHugh D, Pfeuffer S, Gross CC, Korsukewitz C, Melzer N, Klotz L, Meuth SG, Münz C, et al. Impact of FcγR variants on the response to alemtuzumab in multiple sclerosis. Ann Clin Transl Neurol. 2019;6(12):2586–2594. doi:https://doi.org/10.1002/acn3.50935.
- Spahr C, Shi SD, Lu HS. O-glycosylation of glycine-serine linkers in recombinant fc-fusion proteins: attachment of glycosaminoglycans and other intermediates with phosphorylation at the xylose sugar subunit. MAbs. 2014;6(4):904–914. doi:https://doi.org/10.4161/mabs.28763.
- Wen D, Foley SF, Hronowski XL, Gu S, Meier W. Discovery and investigation of O-xylosylation in engineered proteins containing a (Ggggs)n linker. Anal Chem. 2013;85(9):4805–4812. doi:https://doi.org/10.1021/ac400596g.
- Wolf B, Piksa M, Beley I, Patoux A, Besson T, Cordier V, Voedisch B, Schindler P, Stöllner D, Perrot L, et al. Therapeutic antibody glycosylation impacts antigen recognition and immunogenicity. Immunology. 2022;166(3):380–407. doi:https://doi.org/10.1111/imm.13481.
- Au-Yeung BB, Smith GA, Mueller JL, Heyn CS, Jaszczak RG, Weiss A, Zikherman J. IL-2 modulates the TCR signaling threshold for CD8 but not CD4 T cell proliferation on a single-cell level. J Immunol. 2017;198(6):2445–2456. doi:https://doi.org/10.4049/jimmunol.1601453.
- Robb RJ, Greene WC. Internalization of interleukin 2 is mediated by the beta chain of the high-affinity interleukin 2 receptor. J Exp Med. 1987;165(4):1201–1206. doi:https://doi.org/10.1084/jem.165.4.1201.
- Gavin PG, Song N, Kim SR, Lipchik C, Johnson NL, Bandos H, Finnigan M, Rastogi P, Fehrenbacher L, Mamounas EP, et al. Association of polymorphisms in FCGR2A and FCGR3A with degree of trastuzumab benefit in the adjuvant treatment of ERBB2/HER2–positive breast cancer. JAMA Oncol. 2017;3(3):335–341. doi:https://doi.org/10.1001/jamaoncol.2016.4884.
- Jacobs SA, Robidoux A, Abraham J, Pérez-Garcia JM, La Verde N, Orcutt JM, Cazzaniga ME, Piette F, Antolín S, Aguirre E, et al. NSABP FB-7: a phase II randomized neoadjuvant trial with paclitaxel + trastuzumab and/or neratinib followed by chemotherapy and postoperative trastuzumab in HER2(+) breast cancer. Breast Cancer Res. 2019;21(1):133. doi:https://doi.org/10.1186/s13058-019-1196-y.
- Hernandez R, Põder J, LaPorte KM, Malek TR. Engineering IL-2 for immunotherapy of autoimmunity and cancer. Nat Rev Immunol. 2022;22(10):614–628. doi:https://doi.org/10.1038/s41577-022-00680-w.
- Overwijk WW, Tagliaferri MA, Zalevsky J. Engineering IL-2 to give new life to T cell immunotherapy. Annu Rev Med. 2021;72(1):281–311. doi:https://doi.org/10.1146/annurev-med-073118-011031.
- Moynihan K, Pappas D, Sultan H, Park T, Kumar M, Lan R, Ni I, Chen J, Chin M, Schumacher T, et al. 1092 the CD8+ T cell selectivity of AB248 is essential for optimal anti-tumor activity and safety in nonclinical models. J Immunother Cancer. 2022;10:A1135–1135. doi:https://doi.org/10.1136/jitc-2022-SITC2022.1092.
- Hsu EJ, Cao X, Moon B, Bae J, Sun Z, Liu Z, Fu Y-X. A cytokine receptor-masked IL2 prodrug selectively activates tumor-infiltrating lymphocytes for potent antitumor therapy. Nat Commun. 2021;12(1):2768. doi:https://doi.org/10.1038/s41467-021-22980-w.
- Nirschl CJ, Brodkin HR, Hicklin DJ, Ismail N, Morris K, Seidel-Dugan C, Steiner P, Steuert Z, Sullivan JM, Tyagi E, et al. Discovery of a conditionally activated IL-2 that promotes antitumor immunity and induces tumor regression. Cancer Immunol Res. 2022;10(5):581–596. doi:https://doi.org/10.1158/2326-6066.Cir-21-0831.
- Norville K, Skrombolas D, Ferry SL, Kearns N, Frelinger JG. A protease activatable interleukin-2 fusion protein engenders antitumor immune responses by interferon gamma-dependent and interferon gamma-Independent mechanisms. J Interferon Cytokine Res. 2022;42(7):316–328. doi:https://doi.org/10.1089/jir.2022.0043.
- Ptacin JL, Caffaro CE, Ma L, San Jose Gall KM, Aerni HR, Acuff NV, Herman RW, Pavlova Y, Pena MJ, Chen DB, et al. An engineered IL-2 reprogrammed for anti-tumor therapy using a semi-synthetic organism. Nat Commun. 2021;12(1):4785. doi:https://doi.org/10.1038/s41467-021-24987-9.
- Sharma P, Goswami S, Raychaudhuri D, Siddiqui BA, Singh P, Nagarajan A, Liu J, Subudhi SK, Poon C, Gant KL, et al. Immune checkpoint therapy—current perspectives and future directions. Cell. 2023;186(8):1652–1669. doi:https://doi.org/10.1016/j.cell.2023.03.006.
- Charych D, Khalili S, Dixit V, Kirk P, Chang T, Langowski J, Rubas W, Doberstein SK, Eldon M, Hoch U, et al. Modeling the receptor pharmacology, pharmacokinetics, and pharmacodynamics of NKTR-214, a kinetically-controlled interleukin-2 (IL2) receptor agonist for cancer immunotherapy. PLOS ONE. 2017;12(7):e0179431. doi:https://doi.org/10.1371/journal.pone.0179431.
- Klein C, Waldhauer I, Nicolini VG, Freimoser-Grundschober A, Nayak T, Vugts DJ, Dunn C, Bolijn M, Benz J, Stihle M, et al. Cergutuzumab amunaleukin (CEA-IL2v), a CEA-targeted IL-2 variant-based immunocytokine for combination cancer immunotherapy: overcoming limitations of aldesleukin and conventional IL-2-based immunocytokines. Oncoimmunology. 2017;6(3):e1277306. doi:https://doi.org/10.1080/2162402x.2016.1277306.
- Ribba B, Boetsch C, Nayak T, Grimm HP, Charo J, Evers S, Klein C, Tessier J, Charoin JE, Phipps A, et al. Prediction of the optimal dosing regimen using a mathematical Model of tumor uptake for immunocytokine-based cancer immunotherapy. Clin Cancer Res. 2018;24(14):3325–3333. doi:https://doi.org/10.1158/1078-0432.Ccr-17-2953.
- Lebbe C, Ravimohan S, Datta A, Chhibber A, Couselo EM, Pereira C, Sandhu S, Zhou M, Curti B, Khushalani N, et al. 1473 Mechanism of action of bempegaldesleukin (BEMPEG) plus nivolumab (NIVO) in patients with unresectable or metastatic melanoma from the phase 3 randomized open-label PIVOT IO-001 clinical trial. J Immunother Cancer. 2022;10:A1532. doi:https://doi.org/10.1136/jitc-2022-SITC2022.1473.
- Gillies SD, Lo K-M, Burger C, Lan Y, Dahl T, Wong W-K. Improved circulating half-life and efficacy of an antibody-interleukin 2 immunocytokine based on reduced intracellular proteolysis. Clin Cancer Res. 2002;8(1):210–216.
- Joerger M, Calvo E, Laubli H, Lopez J, Alonso G, Corral de la Fuente E, Hess D, König D, Sanchez Perez V, Bucher C, et al. Phase 1 first-in-human dose-escalation study of ANV419 in patients with relapsed/refractory advanced solid tumors. J Immunother Cancer. 2023;11(11):e007784. doi:https://doi.org/10.1136/jitc-2023-007784.
- Diab A, Tannir NM, Bentebibel S-E, Hwu P, Papadimitrakopoulou V, Haymaker C, Kluger HM, Gettinger SN, Sznol M, Tykodi SS, et al. Bempegaldesleukin (NKTR-214) plus nivolumab in patients with advanced solid tumors: phase I dose-escalation study of safety, efficacy, and immune activation (PIVOT-02). Cancer Discov. 2020;10(8):1158–1173. doi:https://doi.org/10.1158/2159-8290.Cd-19-1510.