Abstract
Impact of redox active transition metals on activation of cell death signaling in plant cells have been documented to date. We have recently reported that GC-rich DNA oligomers with high affinity for binding of copper and catalytic activity for removal of ROS as novel plant cell-protecting agents. Here, we show that similar DNA oligomers protect the mouse macrophage-like RAW264.7 cells from copper-induced cell death, suggesting that the phenomenon firstly observed in plant model can be expanded to a wider range of cells and/or organisms including mammalian cells.
It has been well documented that the toxicity of copper ions can be largely attributed to their redox activity. The Cu+ ion readily reacts with hydrogen peroxide (H2O2) to generate hydroxyl radicals (HO•) via Fenton chemistry, which damages cellular components such as proteins, membrane lipids and nucleic acids,Citation1 while Cu2+ reportedly allows the oxidation of cellular thiols to form disulfides,Citation2 and the generation of nitric oxide (NO) through interaction with S-nitrosothiols.Citation3 Based on the redox actions of copper, the antimicrobial activity of copper has long been recognized, and therefore, copper has been used in a medical context from the ancient time to the present day.Citation4
Due to toxic actions, undesirable effects of transition metals including copper have been reported in various organisms including agriculturally important plants. Among such metals, ions of copper (Cu+/2+) are known to be phytotoxic, thus, induce a series of biological and chemical reactions in plant cells including the burst of production of reactive oxygen species (ROS) and stimulation of calcium channel opening allowing a transient increase in cytosolic calcium concentrations.Citation5-8 For an instance, impact of copper ions on oxidative and calcium signal transductions leading to cell death in plant cells have been demonstrated in suspension cultured cell-line of transgenic tobacco (Nicotiana tabacum L., cell line BY-2) expressing aequorin, a calcium-responsive luminescent protein, in cytosolic space.Citation6
Furthermore, ions of copper (chiefly Cu2+) taken up by living plant cells was shown to bind and damage the DNA inside nuclei,Citation9,10 through binding to the motifs rich in guanine (G) and cytosine (C) bases at physiological pH.Citation11 By making use of the Cu-binding nature of GC-rich DNA, we have recently reported that DNA fragments with GC-rich Cu-binding motifs can be used as novel plant cell-protecting agents preventing the Cu-induced cell death.Citation12 Addition of double-stranded GC-rich DNA fragments (especially DNA hexamer, CGCGCG sequence), prior to the addition of Cu2+, effectively blocked both the Cu2+-induced calcium influx (an early event in plant programmed cell death) and the resultant cell death in the aequorin-expressing tobacco cell suspension. Interestingly, DNA-mediated protection of the tobacco cells from Cu toxicity was shown to be largely due to mitigation of oxidative stress since the DNA-Cu complex formed in vitro was shown to catalyze the removal of superoxide anion radicals in a manner similar to the activity of superoxide dismutase (SOD).Citation12
Apart from the models in the plant kingdom, we hypothesized that cell protecting actions of GC-rich DNA fragments can be universally observed even in animal cells. In the present study, murine leukemic monocyte macrophage-like RAW264.7 cells were used as a model mammalian cell-line. Monocytic macrophages are highly important components in mammalian immune system which are attracted by chemokines,Citation13,14 and in turn they attract and induce the accumulation of neutrophils at the site of inflammation through secretion of different sets of chemokines.Citation15
RAW264.7 cells were cultured as adherent cells in RPMI-1640 medium supplemented with 10% heat-inactivated FBS under a humidified 5% CO2 atmosphere at 37°C. When the cells were grown to confluency, they were collected by scraping with a rubber policeman and seeded at a lower cell density. Newly harvested RAW264.7 cells were further conditioned in CO2 incubator for 24 hours after 450 μl of inoculating cell suspension was added onto each well of 48-well microplates. Then cells were washed and medium was replaced with 450 μl of fresh medium (RPMI-1640). Copper (II) sulfate was first dissolved in RPMI-1640 medium and added to the cells (50 μl). After further incubation of the Cu2+-treated cells in the presence and absence of GC-rich DNA oligomers for 12 hours, the rate of cell death was evaluated. Counting of dead cells were performed under a microscope after staining with Trypan blue ().
Figure 1. Copper-induced cell death. Typical morphological change in dead cells partially observed in the presence of low dose Cu2+ (100 μM) was visualized after staining with Trypan blue.
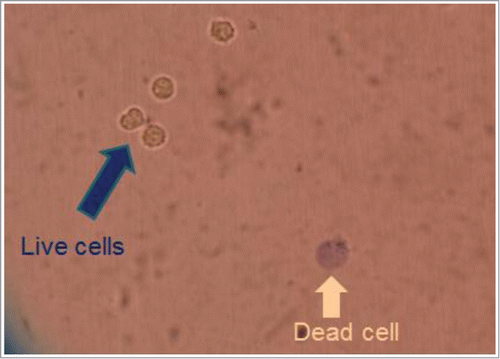
In the preliminary experiments, we examined the toxic range of copper (II) sulfate in RAW264.7 cells and observed that LC50 value for CuSO4 was around 500 μM and 95% of cells were killed at 1 mM CuSO4 (data not shown). With 100 μM CuSO4, 15–20% of cell death was shown to be induced. In the following experiments, effect of 100 μM CuSO4 was tested as moderate level of cell death-inducing stimulus.
For mitigation of Cu-induced cellular toxicity, effects of 3 different CG-repeated DNA sequences, namely, DNA tetramer designated as (CG)2, hexamer designated as (CG)3, and dodecamer designated as (CG)6, were examined. In our previous model, plant cells were mixed up with DNA hexamer, (CG)2 or other model DNA sequences, at 23 ± 1°C. In this range of temperature, (CG)2 sequence presents at fully double-stranded form. In contrast, in the present study, RAW264.7 cells were maintained at 37°C, therefore, only (CG)6, the longest sequence examined, was shown to be at fully double-stranded state as judged from the calculated melting temperatures (Tm; ).
Table 1. Melting temperatures (Tm) for DNA oligomers examined with 3 different methods
Here, we observed that GC-rich DNA oligomers protected the murine macrophage-like RAW264.7 cells from cell death induced by 100 μM CuSO4 (). As expected from Tm, (CG)6, the longest and double stranded chain of DNA, showed the most strong action preventing the toxicity of copper in a dose-dependent manner (). The data obtained here suggest that the phenomenon of interest firstly observed in our plant cell model (with tobacco cells) should not be limited only within the plant kingdom but it could be observed in wider range of organisms covering mammalian cells.
Figure 2. Effects of oligo-DNA sequences on mitigation of copper toxicity. Effects of 3 different DNA sequences with CG-repeats differed in length were examined. Cells treated with 100 μM CuSO4 were incubated for 12 hours with and without DNA oligomers. Bars, SD (n = 8 for control and n = 4 for rest of data points).
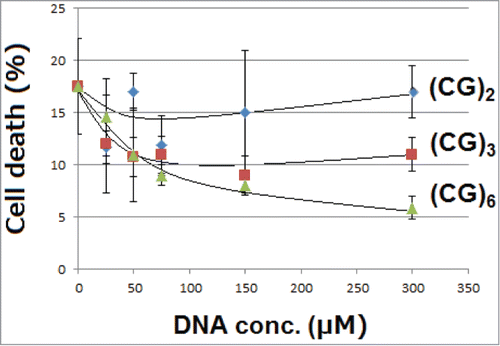
In this addendum report, only the data on toxicity mitigation by DNA are presented without examining the details of the mechanism hidden behind the phenomenon. As discussed in the previous report,Citation12 there could be 2 distinct modes of action of GC-rich DNA sequences acting against the toxic behaviors of Cu ions, namely, (1) removal of free Cu ions through chelating action of GC-rich DNA, and (2) catalytic activity of DNA/Cu complex effectively removing superoxide anion radicals.Citation12 Similarly to the former mode (trapping of free Cu ions), an approach for protection of living (plant) cells by a human prion protein-derived Cu-binding peptide (amino acid sequence, KTNMKHMA) have been reported.Citation6 The latter mode by DNA-Cu complex (catalytic removal of superoxide) is rather unique, which is distinct from the actions of conventional ROS scavengers and antioxidants.Citation12 Contrary, formation of complex between Cu ions and known Cu-binding peptides including mammalian prions’ Cu-binding helical peptide,Citation16 mammalian prions’ octarepeat sequence,Citation17 hexa repeat sequence in chicken prion,Citation18 ozone-induced Cu-binding peptides from saltbush plants,Citation19 and synthetic Cu-binding peptides,Citation20,21 reportedly resulted in no ROS scavenging activity, but instead, robust generation of superoxide anion radical was manifested. Taken together, Cu-bound biomolecules can be grouped into (i) redox inert complexes,Citation6 (ii) pro-oxidants,Citation16-21 or (iii) anti-oxidants.Citation12
In this study, we focused solely on the toxicity of Cu in the macrophage-like cells. In turn, it is also known that Cu causes bacterial destruction by targeting the iron–sulfur cluster enzymes that are essential for survival.Citation22 As above, Cu is toxic toward eukaryotic cells as well as toward bacteria, and the complex system of transporters and chaperones involved in homeostasis and trafficking of this ion is now well understood.Citation1,23 Interestingly, in some intracellular pathogenic bacteria such as Salmonella enterica sv. Typhimurium and Mycobacterium tuberculosis, systems conferring tolerance toward copper ions have evolved.Citation24. Therefore, movement of key transition metal ions (chiefly of Cu) is recognized to be of critical importance in the interaction between macrophages and intracellular pathogens.Citation1 Recent study by Achard et al.,Citation1 revealed the role of Cu ions in mouse macrophages’ responses to infection by Salmonella enterica sv. Typhimurium. Accordingly, both lipopolysaccharide (LPS) and infection with Salmonella effectively triggered Cu accumulation within punctate intracellular vesicles (through copper uptake into cells) in bone-marrow-derived macrophages and this event peaked at ca. 18 h post-stimulation.Citation1 Interestingly, localization of the Cu hot spots are distinct from Salmonella containing vacuoles and lysosomes. Although the Cu hot spot formation may contribute to antimicrobial responses against intracellular bacterial pathogens in macrophages, these condition may also increase the risk of Cu-induced cell death in the hosting macrophages. Therefore, in the future experiments, toxic impact of Cu and its mitigation by DNA oligomers must be assessed in these extreme conditions possibly using LPS, under which uptake of Cu by macrophages are largely enhanced.
Disclosure of Potential Conflicts of Interest
No potential conflicts of interest were disclosed.
Funding
TK was supported by a grant of Regional Innovation Strategy Support Program implemented by Ministry of Education, Culture, Sports, Science and Technology (MEXT), Japan.
References
- Achard ME, Stafford SL, Bokil NJ, Chartres J, Bernhardt PV, Schembri MA, Sweet MJ, McEwan AG. Copper redistribution in murine macrophages in response to Salmonella infection. Biochem J 2012; 444:51-7; PMID:22369063; http://dx.doi.org/10.1042/BJ20112180
- Hiniker A, Collet JF, Bardwell JCA. Copper stress causes an in vivo requirement for the Escherichia coli disulfide isomerase DsbC. J Biol Chem 2005; 280:33785-91; PMID:16087673; http://dx.doi.org/10.1074/jbc.M505742200
- Williams DLH. The chemistry of S -nitrosothiols. Acc Chem Res 1999; 32:869-76; http://dx.doi.org/10.1021/ar9800439
- Borkow G, Gabbay J. Copper as a biocidal tool. Curr Med Chem 2005; 12:2163-75; PMID:16101497; http://dx.doi.org/10.2174/0929867054637617
- Inoue H, Kudo T, Kamada H, Kimura M, Yamaguchi I, Hamamoto H. Copper elicits an increase in cytosolic free calcium in cultured tobacco cells. Plant Physiol Biochem 2005; 43:1089-94; PMID:16330216; http://dx.doi.org/10.1016/j.plaphy.2005.09.003
- Kagenishi T, Yokawa K, Kuse M, Isobe M, Bouteau F, Kawano T. Prevention of copper-induced calcium influx and cell death by prion-derived peptide in suspension-cultured tobacco cells. Z Naturforsch 2009; 64c:411-7
- Gonzalez A, Vera J, Castro J, Dennett G, Mellado M, Morales B, Correa JA, Moenne A. Co-occurring increases of calcium and organellar reactive oxygen species determine differential activation of antioxidant and defense enzymes in Ulva compressa (Chlorophyta) exposed to copper excess. Plant Cell Environ 2010; 33:1627-40
- Min HL, Cai SJ, Rui Z, Sha S, Xie KB, Xu QS. Calcium-mediated enhancement of copper tolerance in Elodea canadensis. Biol Plantar 2013; 57:365-9; http://dx.doi.org/10.1007/s10535-012-0275-z
- Chiou SH. DNA- and Protein-scission activities of ascorbate in the presence of copper ion and a copper-peptide complex. J Biochem 1983; 94:1259-67; PMID:6654857
- Yokawa K, Kagenishi T, Kawano T. Prevention of oxidative DNA degradation by copper-binding peptides. Biosci Biotechnol Biochem 2011; 75:1377-9; PMID:21737913; http://dx.doi.org/10.1271/bbb.100900
- Tajmir-Riahi HA, Langlais M, Savoie R. A laser Raman spectroscopic study of the interaction of calf-thymus DNA with Cu(II) and Pb(II) ions: metal ion binding and DNA conformational changes. Nucleic Acids Res 1988; 16:751-62; PMID:3340554; http://dx.doi.org/10.1093/nar/16.2.751
- Iwase J, Furukawa H, Hiramatsu T, Bouteau F, Mancuso S, Tanaka K, Okazaki T, Kawano T. Protection of tobacco cells from oxidative copper toxicity by catalytically active metal-binding DNA oligomers. J Exper Bot 2014; 65:1391-402; http://dx.doi.org/10.1093/jxb/eru028
- Preobrazhensky AA, Dragan S, Kawano T, Gavrilin MA, Gulina IV, Chakravarty L, Kolattukudy PE. Monocyte chemotactic protein-1 receptor CCR2B is a glycoprotein that has tyrosine sulfation in a conserved extracellular N-terminal region. J Immunol 2000; 165:5295-303; PMID:11046064; http://dx.doi.org/10.4049/jimmunol.165.9.5295
- Gavrilin MA, Gulina IV, Kawano T, Dragan S, Chakravarti L, Kolattukudy PE. Site-directed mutagenesis of CCR2 identified amino acid residues in transmembrane helices 1, 2, and 7 important for MCP-1 binding and biological functions. Biochem Biophys Res Commun 2005; 327:533-40; PMID:15629146; http://dx.doi.org/10.1016/j.bbrc.2004.12.037
- Sakai S, Kawamata H, Kogure T, Mantani N, Terasawa K, Umatake M, Ochiai H. Inhibitory effect of ferulic acid and isoferulic acid on the production of macrophage inflammatory protein-2 in response to respiratory syncytial virus infection in RAW264.7 cells. Mediat Inflam 1999; 8:173-5; http://dx.doi.org/10.1080/09629359990513
- Yokawa K, Kagenishi T, Goto K, Kawano T. Tyrosine-dependent superoxide generation catalyzed by a peptide fragment corresponding to the helical copper-binding sequence in prion protein. Int J Biol Sci 2009; 5:53-63; PMID:19158988; http://dx.doi.org/10.7150/ijbs.5.53
- Kawano T. Prion-derived copper-binding peptide fragments catalyze the generation of superoxide anion in the presence of aromatic monoamines. Int J Biol Sci 2007; 3:57-63; http://dx.doi.org/10.7150/ijbs.3.57
- Yokawa K, Kagenishi T, Kawano T. Cypridina luciferin analog, CLA as a probe for the enzymatic reaction of superoxide generation catalyzed by copper-binding hexapeptide found in chicken prion protein. Luminescence 2010; 25:139
- Yokawa K, Kagenishi T, Kawano T. Superoxide generation catalyzed by the ozone-inducible plant peptides analogous to prion octarepeat motif. Plant Signal Behav 2011; 6:477-82; PMID:21350332; http://dx.doi.org/10.4161/psb.6.4.14744
- Kagenishi T, Yokawa K, Kadono T, Uezu K, Kawano T. Copper-binding peptides from human prion protein and newly designed peroxidative biocatalysts. Z Naturforsch 2011; 66 c:182-90; http://dx.doi.org/10.5560/ZNC.2011.66c0182
- Yokawa K, Kagenishi T, Kawano T. Thermo-stable and freeze-tolerant nature of aromatic monoamine-dependent superoxide-generating activity of human prion-derived Cu-binding peptides. Biosci Biotechnol Biochem 2009; 73:1218-20; PMID:19420682; http://dx.doi.org/10.1271/bbb.90012
- Macomber L, Imlay JA. The iron-sulfur clusters of dehydratases are primary intracellular targets of copper toxicity. Proc Natl Acad Sci USA 2009; 106:8344-9; http://dx.doi.org/10.1073/pnas.0812808106
- Balamurugan K, Schaffner W. Copper homeostasis in eukaryotes: teetering on a tightrope. Biochim Biophys Acta 2006; 1763:737-46; PMID:16784785; http://dx.doi.org/10.1016/j.bbamcr.2006.05.001
- Dupont CL, Grass G, Rensing C. Copper toxicity and the origin of bacterial resistance – new insights and applications. Metallomics 2006; 3:1109-18; http://dx.doi.org/10.1039/c1mt00107h