ABSTRACT
Prior to initiate energy-consuming processes, such as DNA replication or cell division, cells need to evaluate their metabolic status. We have recently identified and characterized a new connection between metabolism and cell division in the α-proteobacterium Caulobacter crescentus. We showed that an NAD-dependent glutamate dehydrogenase (GdhZ) coordinates growth with cell division according to its enzymatic activity. Here we report the conserved role of GdhZ in controlling cell division in another α-proteobacterium, the facultative intracellular pathogen Brucella abortus. We also discuss the importance of amino acids as a main carbon source for α-proteobacteria.
Undoubtedly, a cell needs to coordinate growth and cell division with nutrient availability. This metabolic control of cell division is particularly important to ensure cell size homeostasis of a growing population.Citation1–4 Although this concept is widely accepted, little is known about how the nutritional status is communicated to the cell cycle. Our recent discovery that the glutamate dehydrogenase GdhZ coordinates metabolism with cell division in C. crescentus () highlights the central role of metabolic enzymes in signaling nutrient fluctuations to the cell cycle.Citation5 Here we discuss the conserved role of GdhZ in controlling cell division in α-proteobacteria, and debate the importance of amino acids catabolism among those bacteria.
Figure 1. Metabolic control of cell division by GdhZ in Caulobacter crescentus C. crescentus has developed a complex asymmetric cell cycle to optimize its survival in oligotrophic environments. (a) Each cell division produces a small swarmer cell and a large stalked cell. The swarmer cell is proposed to be a settler looking for new environments whereas the stalked cell is responsible for colonizing these environments by giving birth to progeny cells. When a swarmer cell finds favorable conditions, it differentiates into a stalk cell to enter into a replicative cycle. (b-c) C. crescentus has evolved a system in which the NAD-dependent glutamate dehydrogenase GdhZ acts as a proxy, signaling nutrient availability to the division apparatus, thereby coordinating growth with cell division. FtsZ and GdhZ are respectively represented in green and red.Citation5
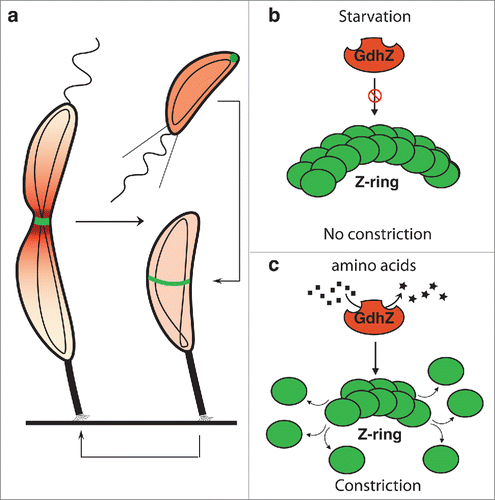
GdhZ coordinates growth with cell division in the intracellular pathogen brucella abortus
The α-subdivision of proteobacteria includes gram-negative bacteria with diverse and contrasting lifestyles, ranging from free-living, symbiotic to pathogenic bacteria.Citation6 Despite this apparent heterogeneity, α-proteobacteria share some unexpected common features such as an asymmetric cell division followed by a differentiation process.Citation7–9 As the large NAD-dependent glutamate dehydrogenases, to which GdhZ belongs, are particularly well conserved among α-proteobacteria,Citation10 we postulated that the cell division control found in C. crescentus might be conserved as well. To address this question we created an in-frame deletion of gdhZ homolog in the facultative intracellular pathogen Brucella abortus 2308 (gdhZBa). Similarly to gdhZ loss-of-function mutants in C. crescentus,Citation5 the ΔgdhZBa strain had a strong growth defect when grown in complex medium (). Interestingly, ΔgdhZBa cells were slightly elongated and branched (), which typically indicates a cell division defect in bacteria growing from one pole.Citation11,12 Furthermore growth and cell division defects were suppressed when glucose or xylose was added to the complex media or when xylose was used as the sole carbon source in a synthetic medium ( and data not shown). Altogether these observations suggest that the role of GdhZ in controlling cell division might be conserved in B. abortus, and likely in other α-proteobacteria.
Figure 2. GdhZ coordinates growth with cell division in Brucella abortus. (a) Growth of B. abortus wild-type (black) and ΔgdhZBa (gray) cells in complex medium (2YT) or in synthetic medium with xylose as the only carbon source (Plommet Xylose), showing GdhZ is required for optimal growth in complex mediium. (b) Phase contrast imaging of B. abortus wild-type and ΔgdhZBa cells grown in complex medium (2YT) illustrating the morphological defects with elongated and branched cells (arrows) developed by ΔgdhZBa cells. Cells were imaged in mid-exponential phase of growth. Scale bar, 2 μm.
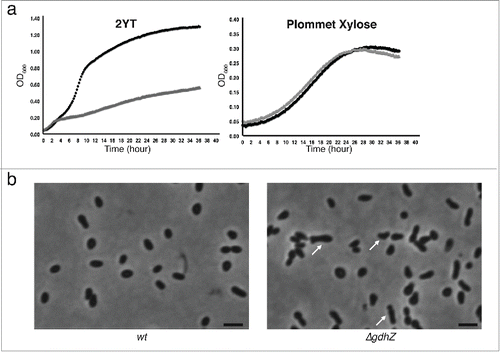
GdhZ is required for efficient intracellular replication of Brucella abortus
As an intracellular pathogen, Brucellae reside preferentially within trophoblasts and macrophages,Citation13,14 in which it can survive and proliferate to produce chronic infections.Citation15,16 Intracellular Brucellae hijack the intracellular vesicular trafficking to finally reach and replicate into the endoplasmic reticulum.Citation17 Althougth widely accepted to be a nutrient poor environment, the exact composition of the Brucella containing vacuole (BCV) remains unclear.Citation16 To test whether GdhZ is important for intracellular replication, murine raw macrophages were infected with either wild-type or ΔgdhZBa strain, and the number of intracellular bacteria was determined at 2 hrs, 24 hrs and 48 hrs post-infection (PI). As GdhZ constitutes a key entry point into the tricarboxylic acid (TCA) cycle for several amino acids, the ΔgdhZBa mutant would be unable to efficiently replicate inside host cells if amino acids were a main carbon source during infection. As illustrated in , the ΔgdhZBa mutant was already impaired 2 hrs PI and intracellular Brucellae failed to replicate efficiently at 24 hrs and 48 hrs PI. During the first 6–8 hrs following entry into the cell, intracellular Brucellae are in a G1 state and do not proliferate.Citation18 Therefore, the difference in the number of intracellular bacteria between the strains at 2 hrs post-infection might be due to a defect in the internalization process. Since bacteria were cultivated in complex media prior to infect, the proper entry of ΔgdhZBa cells inside macrophages might be affected by their morphological defect (). To circumvent this problem, B. abortus wild-type and ΔgdhZBa strains were grown in complex medium supplemented with glucose prior to infect murine macrophages. Interestingly, when glucose was added to the preculture medium, both wild-type and ΔgdhZBa intracellular bacteria were present at similar levels at 2 hrs PI, indicating that the entry impairment observed with ΔgdhZBa cells was very likely a secondary effect (). Nevertheless, at 24 hrs and 48 hours PI, ΔgdhZBa cells failed to efficiently replicate compared to wild-type cells, whatever the medium used for cultivating Brucella before infecting macrophages. Altogether these results strongly suggest that GdhZ is required for optimal intracellular replication of Brucella, and emphasize the importance of amino acids as a main carbon source for Brucellae inside host cells.
Figure 3. GdhZ is required for efficient replication of Brucella abortus inside murine raw macrophages. Internalization and intracellular replication of B. abortus wild-type and ΔgdhZBa cells into murine raw macrophages. RAW 264.7 macrophages were cultured at 37 °C (5 % CO2 atmosphere) in DMEM (Invitrogen) supplemented with 10% fetal bovine serum (Gibco), 4.5 g/L glucose, 1.5 g/L NaHCO3 and 4 mM glutamine. Cultures of Brucella were prepared in DMEM at a multiplicity of infection of 50. Bacteria were centrifuged at 400 x g for 10 min at 4 °C and then incubated for 1 hr at 37 °C (5 % CO2 atmosphere). Cells were washed twice with fresh medium and then incubated in medium supplemented with 50 μg/ml gentamicin to kill extracellular bacteria. Prior to infections, bacteria were grown in (a) 2YT or (b) 2YT + 0.2 % glucose. The significant pairwise comparisons are indicated for p < 0.5 (*), p < 0.01 (**) and p < 0.001 (***) in Student t-tests.
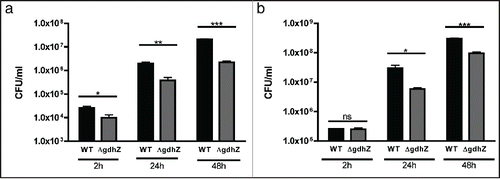
Amino acids as a main carbon source for α-proteobacteria
Other metabolic regulators of cell division have been described in Escherichia coli and Bacillus subtilis.Citation19–21 Interestingly, all these regulators link glycolysis to cell division and both species use glucose and hexoses as major carbon sources. On the other hand, the deletion of gdhA in E. coli, coding for an anabolic NADP-dependent glutamate dehydrogenase, did neither slow down growth in complex (LB) or synthetic (M9G) media, nor interfere with cell division (data not shown). In contrast, C. crescentus and B. abortus use GdhZ, catabolizing amino acids, to coordinate metabolism with cell division. Based on these observations we postulate that bacteria have evolved by connecting the cell division machinery with the catabolic routes whose activity reflects nutrient fluctuations in the environment. In other words, such regulations would underline the main carbon sources used by bacteria, indicating that amino acids would constitute a favorite carbon source for C. crescentus, B. abortus, and likely other α-proteobacteria. This hypothesis is further supported by the fact that amino acids transporters and catabolic enzymes are induced in BCV.Citation22
It is noteworthy that, whatever the main carbon source is, metabolic enzymes constitute perfect candidates to fulfill additional regulatory functions. Indeed, the conformational change induced upon substrate(s) binding or product(s) release, can serve as a proxy for the cell to monitor nutrient availability. This is thereby not surprising that most of the proteins in the glycolytic and the TCA pathways are moonlighting enzymes, i.e. enzymes with secondary (regulatory) functions.Citation23 In this perspective and knowing that a NAD-dependent glutamate dehydrogenase connects the nitrogen cycle to the TCA cycle, GdhZ was very likely selected to coordinate growth with cell division in α-proteobacteria.
Disclosure of potential conflicts of interest
No potential conflicts of interest were disclosed.
Funding
F.B. held a FRIA (Fund for Research Training in Industry and Agriculture) fellowship from the National Fund for Scientific Research (F.R.S.-FNRS). R.H. is a FNRS Research Associate. This work was supported by a Research Project (PDR T.0053.13) to X.D.B and a Research Credit (CDR 1.5067.12) to R.H from the F.R.S.-FNRS.
References
- Fantes P, Nurse P. Control of cell size at division in fission yeast by a growth-modulated size control over nuclear division. Exp Cell Res 1977; 107:377-86; PMID:872891; http://dx.doi.org/10.1016/0014-4827(77)90359-7
- Schaechter M, Maaloe O, Kjeldgaard NO. Dependency on medium and temperature of cell size and chemical composition during balanced grown of Salmonella typhimurium. J Gen Microbiol 1958; 19:592-606; PMID:13611202; http://dx.doi.org/10.1099/00221287-19-3-592
- Donachie WD, Begg KJ. Cell length, nucleoid separation, and cell division of rod-shaped and spherical cells of Escherichia coli. J Bacteriol 1989; 171:4633-9; PMID:2670889
- Sargent MG. Control of cell length in Bacillus subtilis. J Bacteriol 1975; 123:7-19; PMID:806582
- Beaufay F, Coppine J, Mayard A, Laloux G, De Bolle X, Hallez R. A NAD-dependent glutamate dehydrogenase coordinates metabolism with cell division in Caulobacter crescentus. EMBO J 2015; 34:1786-800; PMID:25953831; http://dx.doi.org/10.15252/embj.201490730
- Batut J, Andersson SGE, O'Callaghan D. The evolution of chronic infection strategies in the α-proteobacteria. Nat Publishing Group 2004; 2:933-45.
- Hallez R, Bellefontaine A-F, Letesson J-J, De Bolle X. Morphological and functional asymmetry in α-proteobacteria. Trends Microbiol 2004; 12:361-5; PMID:15276611; http://dx.doi.org/10.1016/j.tim.2004.06.002
- Hallez R, Mignolet J, Van Mullem V, Wery M, Vandenhaute J, Letesson J-J, Jacobs-Wagner C, De Bolle X. The asymmetric distribution of the essential histidine kinase PdhS indicates a differentiation event in Brucella abortus. EMBO J 2007; 26:1444-55; PMID:17304218; http://dx.doi.org/10.1038/sj.emboj.7601577
- Lam H, Matroule J-Y, Jacobs-Wagner C. The asymmetric spatial distribution of bacterial signal transduction proteins coordinates cell cycle events. Dev Cell 2003; 5:149-59; PMID:12852859; http://dx.doi.org/10.1016/S1534-5807(03)00191-6
- Minambres B. A New Class of Glutamate Dehydrogenases (GDH). Biochemical and genetic characterization of the first member, the AMP-requiring NAD-specific GDH of Streptomyces clavuligerus. J Biol Chem 2000; 275:39529-42; PMID:10924516; http://dx.doi.org/10.1074/jbc.M005136200
- Latch JN, Margolin W. Generation of buds, swellings, and branches instead of filaments after blocking the cell cycle of Rhizobium meliloti. J Bacteriol 1997; 179:2373-81; PMID:9079925
- Brown PJB, de Pedro MA, Kysela DT, Van der Henst C, Kim J, De Bolle X, Fuqua C, Brun YV. Polar growth in the Alphaproteobacterial order Rhizobiales. Proce Natl Acad Sci U S A 2012; 109:1697-701; http://dx.doi.org/10.1073/pnas.1114476109
- Enright FM. The pathogenesis and pathobiology of Brucella infections in domestic animals. Animal brucellosis. CRC Press; 1990.
- Moreno E, Gorvel JP. Invasion, intracellular trafficking and replication of Brucella organisms in professional and non-professional phagocytes. Brucella: molecular and cellular biology 2004; 287-312.
- Roop RM II, Bellaire BH, Valderas MW, Cardelli JA. Adaptation of the brucellae to their intracellular niche. Mol Microbiol 2004; 52:621-30; PMID:15101970; http://dx.doi.org/10.1111/j.1365-2958.2004.04017.x
- Köhler S, Michaux-Charachon S, Porte F, Ramuz M, Liautard J-P. What is the nature of the replicative niche of a stealthy bug named Brucella? Trends Microbiol 2003; 11:215-9; PMID:12781524; http://dx.doi.org/10.1016/S0966-842X(03)00078-7
- Starr T, Ng TW, Wehrly TD, Knodler LA, Celli J. Brucella intracellular replication requires trafficking through the late endosomal/lysosomal compartment. Traffic 2008; 9:678-94; PMID:18266913; http://dx.doi.org/10.1111/j.1600-0854.2008.00718.x
- Deghelt M, Mullier C, Sternon J-F, Francis N, Laloux G, Dotreppe D, Van der Henst C, Jacobs-Wagner C, Letesson J-J, De Bolle X. G1-arrested newborn cells are the predominant infectious form of the pathogen Brucella abortus. Nat Commun 2014; 5:4366; PMID:25006695; http://dx.doi.org/10.1038/ncomms5366
- Hill NS, Buske PJ, Shi Y, Levin PA. A Moonlighting Enzyme Links Escherichia coli Cell Size with Central Metabolism. PLoS Genet 2013; 9:e1003663; PMID:23935518; http://dx.doi.org/10.1371/journal.pgen.1003663
- Monahan LG, Hajduk IV, Blaber SP, Charles IG, Harry EJ. Coordinating Bacterial Cell Division with Nutrient Availability: a Role for Glycolysis. mBio 2014; 5:e00935-14-e00935-14; PMID:24825009; http://dx.doi.org/10.1128/mBio.00935-14
- Weart RB, Lee AH, Chien A-C, Haeusser DP, Hill NS, Levin PA. A metabolic sensor governing cell size in bacteria. Cell 2007; 130:335-47; PMID:17662947; http://dx.doi.org/10.1016/j.cell.2007.05.043
- Lamontagne J, Forest A, Marazzo E, Denis F, Butler H, Michaud J-F, Boucher L, Pedro I, Villeneuve A, Sitnikov D, et al. Intracellular Adaptation of Brucella abortus. J Proteome Res 2009; 8:1594-609; PMID:19216536; http://dx.doi.org/10.1021/pr800978p
- Huberts DHEW, van der Klei IJ. Biochimica et Biophysica Acta. BBA-Mol Cell Res 2010; 1803:520-5; PMID:20144902