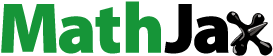
ABSTRACT
In the classical “mind-body” wording, “body” is usually associated with the “mass aspect” of living entities and “mind” with the “immaterial” one. Thoughts, consciousness and soul are classified as immaterial. A most challenging question emerges: Can something that is truly immaterial, thus that in the wording of physics has no mass, exist at all? Many will answer: “No, impossible.” My answer is that it is very well possible, that no esoteric mechanisms need to be invoked, but that this possibility is inherent to 2 well established but undervalued physiological mechanisms. The first one is electrical in nature. In analogy with “genome,” “proteome” etc. “electrome” (a novel term) stands for the totality of all ionic currents of any living entity, from the cellular to the organismal level. Cellular electricity is truly vital. Death of any cell ensues at the very moment that it irreversibly (excluding regeneration) loses its ability to realize its electrical dimension. The second mechanism involves communication activity that is invariably executed by sender-receiver entities that incessantly handle information. Information itself is immaterial (= no mass). Both mechanisms are instrumental to the functioning of all cells, in particular to their still enigmatic cognitive memory system. Ionic/electrical currents associated with the cytoskeleton likely play a key role but have been largely overlooked. This paper aims at initiating a discussion platform from which students with different backgrounds but all interested in the immaterial dimension of life could engage in elaborating an integrating vocabulary and in initiating experimental approaches.
Introduction
In addition to their 3 dimensions in space (x, y, z axes) and their time dimension, living systems also have a self-generated “electrical dimension” and an “immaterial” one that is inherent to their ability to handle (immaterial) information. When dealing with this immaterial dimension of “Life” the term “soul” is widely used, in particular with respect to the transition from “still alive” to “just dead” in humans, and when facing the intriguing question whether or not “the soul” persists one way or another after death. With respect to “consciousness,” a most intriguing key aspect of Life's immaterial dimension, the consensus seems to be growing that it is a ubiquitous property of all living systems, from bacteria to animals, plants and fungi. But consensus is missing on whether the immaterial dimension of Life ultimately has a biophysical basis or not.
Today, the “material sciences” and the “non-material ones” as they are sometimes referred to, hold very different opinions on these challenging questions.Citation1 Some protagonists of the latter even claim that “Biology is beyond physical sciences.”Citation2 Such a statement meets with great skepticism and even blunt rejection among experimentalists. Caetano-AnollésCitation3 states that such opinions should not be published in scientific journals. But rejection of confrontation does not advance insight and rapprochement.
For both the exact sciences and the humanities the key problem is: “Can something that is commonly thought to be truly immaterial (incorporeal and immaterial in the definition of “Soul” in the Encyclopedia Britannica), thus that has no mass in the meaning of classical physics, exist?” In a first reaction students of the exact sciences may be inclined to say: “No, impossible.” This is reflected in the scientific literature: one will not encounter “soul” with the cited meaning in textbooks of western biology or medicine unless when figuratively speaking. In psychology which, in origin, was the study of the “psyche” or “soul,” not “soul” but attitude and behavior represent its essence
If one searches the vast literature on e.g. consciousness, soul, thoughts etc. it soon becomes apparent that many authors give the impression to be either unaware of, or at least do not attribute any importance to the well documented fact that all cells have an immaterial self-generated electrical dimension, and that it is exactly this electrical activity that disappears at death along with thoughts and consciousness. Concurrently, one also gets confronted with the fact that the exact sciences seem to be reluctant to forward possible ideas for a biophysical underpinning of Life's immaterial dimension. Is there no common ground between the exact sciences and the humanities, or could it be that we have all overlooked the possibility that what we call “soul” is simply an aspect of this largely undervalued self-generated cellular electrical activity?
The analysis of what exactly changes at the very moment a living system passes from “still alive” to “just not alive anymore”Citation4,5,6 may help to (partially) define the immaterial dimension of Life with a vocabulary from the exact sciences. It may also help to answer the question whether or not only humans but all living systems/communicating compartments, bacteria inclusive have “a similar immaterial dimension, electrical in nature.”Citation7,8,9
In this paper I will try to demystify the idea that understanding the immaterial dimension of Life is beyond the capacity of the human brain, and that therefore one should not even spend efforts in lifting the veil. Some of its aspects are normal properties of information handling in sender-receiver systems that need self-generated electric/ionic currents for their functioning. Yet, I fully agree that the full picture, namely the full understanding of what thoughts and consciousness etc. are will not be possible earlier than the biophysical and biochemical principles governing the cognitive memory system will have been uncovered. In all honesty, I do not expect this to happen in the near future.
My approach represents a fundamental change in paradigm: from information handling (in particular in our brain) as a supposed almost complete metaphysics process by some students in this domain, to a normal down to earth intelligible aspect of the activity of any sender-receiver compartment.
An unambiguous definition of “Life” as the conceptual framework for “body and mind”
Living versus non-living or inanimate matter: Widely used but scientifically wrong terminology
This dichotomous wording dates from long before the chemical nature of “matter” became known. It is so deeply rooted in all languages, and it sounds so logical that everybody assumes that nothing is wrong with it. But at the present time we know that classical matter = atoms, and that the atoms present in living matter are not different from their counterparts in non-living matter. In other words, the atoms in living matter do not have a special feature as to make them “animated.” The difference living – non-living is not situated in the atoms themselves but in the way the entities in which they end up “behave.” When combined into entities organized as sender-receiver compartments, the combinations of atoms can engage, under proper conditions, in communication and problem-solving activities.Citation5 Combinations of atoms that do not form sender-receiver entities cannot by themselves engage in communication activities (see later). In short: what is classically called “living matter” can communicate, while “non-living matter” never can on its own. Atoms alone do not make the difference, but the activities to which they are instrumental do. For pragmatic reasons, I accept that the living - non-living wording continues to be used for some time.
Definition of a communicating compartment
Communication is an important topic in this paper. Therefore it may not be superfluous to recall the following definition.Citation5,6 A biological communicating compartment, or simply “compartment,” is a unit based on carbon chemistry and on electricity carried by inorganic ions. This unit
- | is limited by a moderately “leaky” boundary with appropriate “holes;” | ||||
- | can stockpile the right form(s) and amounts of energy; | ||||
- | can generate gradients that can be used for communication for the purpose of enabling the | ||||
- | compartment to function from its lowest to its highest level of compartmental organization (see later). |
Life
Over 100 different definitions of “Life” have been published in the past.Citation5 To my knowledge, none takes all presently known dimensions and types of organization of living systems into account in an unambiguous and holistic way as in the definition that I logically deduced.Citation5,6 It meets all criteria a good definition of Life should meet according the philosophers of science Schetjer and Agassi.Citation10 In this deduction opposing the situations “still alive” vs. “just not alive any longer,” instead of by following the classical procedure of comparing the properties of living- versus non-living matter, yielded the view that communication activity is what “Life” is all about.
It reads: Life (as an activity) reads: “Life” sounds like a noun in English, but it denotes an activity, thus it is in fact a verb. In some languages, German e.g., the noun Leben - Life and the verb Leben – to live are the same word. What we call “Life” is nothing other than the total sum of all acts of communication exerted by a given sender-receiver compartment at moment t, at all levels of its compartmental organization (cell organelle, cell, tissue,…, whole organism,…, population, community, Gaia level). The simplest symbolic notation reads: L = ∑C.
Because Life is an activity of a given sender-receiver compartment of which there exist many different forms, one can specify the definition further as:
L = Life; S = type of compartment; t = moment at which the communication acts are executed; 1 = lowest level of compartmental organization (1 = prokaryotic cell or cell organelle in a eukaryotic cell); j = highest level of compartmental organization (cell, tissue, organ, organism, …, aggregate, …, population, community, the Gaia-level). For a symbolic notation that highlights how to compare ‘biological’ and ‘mechanical’ life (e.g. computer-life), as well as for the answer to whether or not a virus or a computer is alive, see refs. Citation4, 6. This definition is compatible with both Neo- and Meta-Darwinian evolutionary theory.Citation11,12
Thus ‘Life’ has both a qualitative (nature of the communication acts) and a quantitative (number of communication acts) aspect.
What changes at “death”? Definition of death
During my trial to define “life,” I was confronted with the problem that hardly any literature existed on what exactly changes at the moment of death. I only succeeded in defining “death” unambiguously in a non-circular way after I first had found a way to classify living systems in a system that is not based on genetic relatedness but on the principles of communication. In my system, there are at least 16 different levels of communicational organization that can be grouped into 3 categories as outlined in detail before:Citation4,5,11
- | Mono-organismal compartments: compartments restricted to one and the same organism (8 levels) | ||||
- | Polyorganismal-monospecies compartments: compartments consisting of more than one individual of the same species (6 levels) | ||||
- | Polyorganismal-heterospecies compartments: compartments consisting of individuals belonging to different species (2 levels) |
Any of these levels, e.g., the cell, the multicellular individual organism, the aggregate, the population, the community etc. can die. By trying to answer the question what exactly changes when any of these compartmental levels passed from “still alive” to “no longer alive,” an unexpected common denominator for “death” emerged. It reads:
Any biological compartment, whatever its degree of complexity dies at the very moment that it irreversibly (to exclude regeneration) loses its ability to communicate at its highest level of compartmental organization.
The duality of death: Multicellular organisms can die while their constituting cells remain alive for a while
For the 2 lowest levels of compartmental organization in my classification system, namely the prokaryotic- and the eukaryotic cell, it can be shown by electrophysiological methods that a cell is dead from the moment that its voltage gradient over its plasma membrane is irreversibly lost. Here the plasma membrane is the highest possible level of communication, and electric events are crucial in communication at this level.
The situation is more complex in multicellular compartments. Medical doctors, in particular when confronted with situations of deep coma will certainly agree. The following thought-experiment that has already been described before,Citation5 will illustrate the duality of the death problem. Sensitive souls should assume that the virtual experimental animal had been anaesthetized before the sequence of events. Is a chicken dead when one leg is amputated? No. Two legs? No. Two legs and 2 wings? No. Thus, “death” is not primarily a matter of loss of classical mass. Is a chicken dead at the very moment of decapitation? Some will say yes, other will say no because the headless body can still move around for a while, be it in an uncoordinated way. Who is right? Is just cutting through the central nervous system in the neck region without removing any tissue sufficient to instantly kill the animal? Yes. One additional experiment: Imagine that a chicken is decapitated in a laboratory and that immediately upon decapitation, all organs are dissected and brought into tissue culture, where they continue to exhibit a number of so called “typical properties” of living matter as outlined in the PICERAS approach of KoshlandCitation13 (). Some cells will even multiply. The chicken does not exist anymore, but its parts are still alive: this I call “the duality of death.” Is it allowed to say that the chicken is fully dead? Yes, even if all its constituent cells are still alive for a while. Thus, “death” refers to a particular level of compartmental organization, namely the highest one. In the case of e.g. a vertebrate, the brain is the highest (coordinating) level of communication. A brain-dead person is no longer a person, but a corpse which is an aggregate of cells and tissues.
Figure 1. Two graphical representations of the essence of “Life.” (A) In the classical approach of Koshland,Citation13 “Life” is thought to be far too multifaceted to condense its essence in a single sentence. In this view, the most viable option is to list the key properties of Life. Koshland thought that Reproduction is what Life is all about. (B) In digital-era wording, Communication/problem-solving activity emerges as the very essence of being alive.Citation5,6
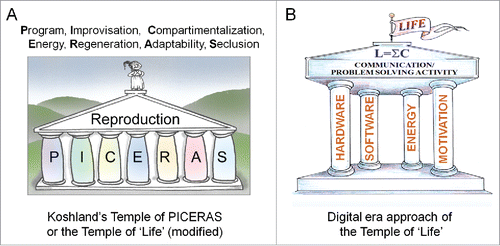
This type of reasoning can be repeated for any of the multicellular types of compartments. For example, at the level of the population, the highest level is the communication activity among the members of the population. If the constituting members of a small population (e.g., of deer) are in whatever way dispersed so far from each other that they cannot hear, smell and see each other, and that they will never meet again, the population can be declared dead, even if all constituting individuals continue to live and even when pregnant females will give birth. The highest level of communication here is the inter-individual use of any sort of language. For the constituting cells of each organism, their highest level remains the plasma membrane, with electrical activity at its core.
Thus in conclusion: the transition from “still alive” to “no longer alive” involves a drastic change, namely a total and irreversible collapse of the communication activity (= handling of information) at the highest level of compartmental/communicational organization of the dying entity under consideration.
Do these 2 activities, namely the electrical- and the communicational ones represent the very essence of what in daily language is often referred to as “the soul”? Or are they only side-aspects, because, perhaps, other as yet unknown immaterial aspects/activities might still be missing?
The body-mind duality with respect to “death”
The “body of organisms,” their hardware in digital era () wording, uses fossil stardust as building blocks, the most abundant ones being the atoms C, H, O, N, P, Cl, Na, K, Ca etc. Atoms with mass higher than 1 (= H) up to that of iron (Fe) which has mass 56 are all “multiples” of H that came into existence by fusion reactions in stars. Elements with mass higher than 56 are formed during supernova explosions that take place when some stars die. Thus the saying that the hardware of our body literally consists of fossil stardust and that therefore it is as old as the universe (approximately 13.8 billion years) is scientifically and literally correct.Citation5 The constituents of the hardware, namely the atoms, are recycled after death. Thus absolute “death” does not apply to the hardware aspect of living systems: it is in a continuous flow of transformation.
The “mind part,” usually referred to as the immaterial part or the software part in digital era wording,Citation5,6 is often thought to arise de novo at the moment of multiplication of cells and organisms (see later). It is subject to change lifelong. The question whether there is “life after death” only applies to mind part. In this paper the focus will be on what happens with “the electrical dimension” at death.
Biological/cellular electricity: Not esoteric at all, but often highly undervalued
Although all prokaryotic and eukaryotic cell types use self-generated electricity, the variety of functions to which it is instrumental is seldom adequately covered in textbooks of introductory biology, at least not in such a way that students become aware of the over-all importance of “the electrical dimension” of cells ().Citation14 Even more, the “electrical dimension of cells” is not a major teaching issue in the humanities in general, philosophy inclusive: unknown is unloved.
Figure 2. Left: Two key features are seldom represented in classical pictures of a model cell. The first one concerns its “electrical dimension,” represented here by the voltage gradient over the plasma membrane and the ability of cells to drive, at least during part of the cell cycle, a self-generated electric current through themselves. In unstimulated (= resting) cells the cytoplasmic side of the plasma membrane is at negative potential. The second feature is that cells have 2 memory systems, the genetic/epigenetic one which is well understood, and the cognitive one which remains highly enigmatic. Right: The voltage gradient over the plasma membrane is based upon a gradient of inorganic ions. In animal cells, the cytoplasm contains more K+ than e.g. blood, while the opposite situation prevails for Na+. Ion pumps are enzymes that can transport an ion against its concentration gradient. This process requires energy delivered by the mitochondria (M). Ion channels allow the passage of a particular ion(s) down its gradient. This is a passive process; it does not require energy. The membrane potential results mainly from the interplay between ion pumps and channels.Citation5,14
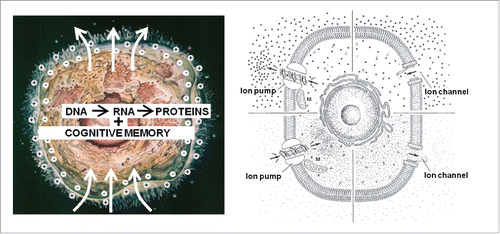
Figure 3. Schematic representation of the electric field around a variety of systems, as measured by the vibrating probe technique developed by ref. Citation16. The electrogenic ion fluxes are produced by the systems themselves. Adapted from refs. Citation5,10,12,14,18.
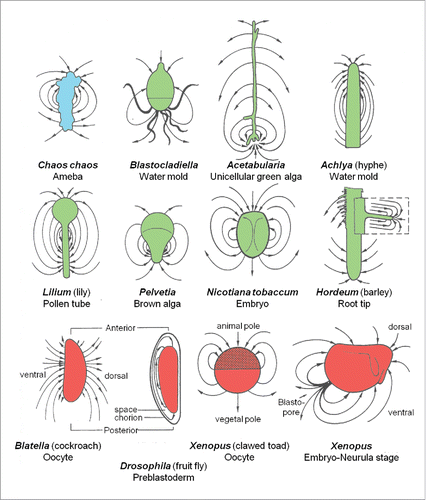
Electric fish were at the origin of the analysis of the phenomenon “electric current”
To many people, electrical phenomena in living systems still have an aura of being somewhat esoteric. That must certainly have been the case when “victims” experienced strange, unpleasant but transient shocks upon contact with (disturbed) electric fish. An electric fish is any fish that can generate an electric field from a specialized structure called an electric organ.Citation15 Such organs are made up of modified muscle or nerve cells. The electric organ discharge can be so strong that (electroreceptive) animals feel it as a strong electric shock, with paralysis and occasionally even death as a result. Yet, the great majority of electric fish species do not produce such strong shocks. It is known that the head of sharks is surrounded by an electric field that helps to localize preys.
It was only after the cause of the shock-induction could be studied in non-living systems, e.g. in the primordial bimetal batteries that the biophysical foundations of biological and non-biological electricity (e.g., Ohm's law) etc. could be established. It was still later that it became clear that “fish electric current” and “electric current from the socket,” although they obey the same rules, differ in a major aspect, namely in the type of carrier of charge, inorganic ions vs. electrons respectively. Finally, it turned out that the electrical activity in neurons (= the best studied cell type) and in all other prokaryotic and eukaryotic cell types is based upon the same principles as that in electric organs.
A concise introduction into the nature of self-generated biological electricity
Biological electric current is not carried by electrons but by simple inorganic ions
An electric current is the transport of charge through a conductor. Today every educated person knows that the electricity from the socket that we use in daily life is due to transport of electrons. The speed of electron-based electricity nears the speed of light, namely 300,000 km/sec when the resistance of the medium is very low, otherwise it is somewhat lower. It is less well known that biological electricity is not carried by electrons but by simple inorganic ions such as H+, K+, Na+, Ca2+, Cl− and HCO−. The velocity of action potentials in neurons ranges between 1 and 100 m.sec−1, depending upon the characteristic properties of the electrical circuit model. These values overlap with the propagation of ionic currents along actin fibers (see later).Citation16 Thus ionic currents are a “slow type” of electricity.
The lipid nature of the plasma- and other membranes is essential for ion-based electricity. Why ionic gradients?
The reason why cells do not use electron-based electricity for establishing a transmembrane voltage gradient is that the plasma membrane which mainly consists of a lipid bilayer in which proteins with a hydrophobic moiety are embedded, is permeable to electrons, prohibiting that electrons can be concentrated and confined in the cytoplasm. However, the cited inorganic ions are much larger than electrons. They cannot freely pass a lipid bilayer meaning that if there is a mechanism for transporting these ions in a selective way through the membrane(s), establishing a concentration gradient over both sides of a membrane becomes possible under proper conditions.
In eukaryotic animal cells, the concentration of K+ is much higher in the cytoplasm than in the extracellular fluid, in particular in the blood. The opposite is true for Na+ and Ca2+. The concentration of Na+ in the cytoplasm of resting (= unstimulated) cells is low while it is high in blood. An influx of Na+ into the cytoplasm, e.g. when an action potential is generated in a neuron, causes depolarization of the plasma membrane. This means that the voltage gradient over the plasma membrane decreases, e.g., from −60 mV to −30 mV. An efflux repolarizes the membrane. For Ca2+ the situation is more complex. Ca2+ is a very toxic ion. In resting cells the concentration of free Ca2+ in the cytoplasm is very low. In extracellular fluid it is orders of magnitude higher. There are also storage sites for Ca2+ inside eukaryotic cells where high Ca2+ concentrations are possible, in particular the endoplasmic reticulum and the mitochondria.
There must be a reason why the cytoplasmic concentration of K+ in animal- and other eukaryotic cell types is high. I think that such high concentration is, among other functions, needed for protecting the storage of cognitive information that is stored, by I guess, in an electrical system associated with the cytoskeleton (see later). If it were for osmoregulation purposes, other possibilities would also be possible and would have become established in some species of animals and plants. In animal cells at least, this is not the case. There must also be a reason why the influx of Na+ in e.g. neurons for generating an action potential is limited to the membrane region and why it only lasts very short. Maybe, longer periods of elevated cytoplasmic Na+-rise damage the cognitive storage system (see later).
Ion channels and ion pumps
For the selective transmembrane transport of inorganic ions the cell uses a system that involves specialized proteins that reside in the plane of the membrane(s) (). Ion channels are proteins that can be “gated” by specific triggers thereby allowing (more or less selective) passive transport; this means down the concentration gradient (= from a higher to a lower concentration) of the ion (e.g., Na+, K+, Ca2+..) involved. This type of transport does not require energy. In contrast, ion pumps are proteins that also reside in the plane of the membrane but they can transport specific ions from a place where the concentration of the ion which is considered is lower (e.g. in the extracellular compartment) to the other side of the membrane where the concentration of this ion is already higher. Ion pumps are enzymes. Their pumping activity requires energy in the form of ATP (adenosine triphosphate) and is named “active transport by ion pumps.” The interplay between the various types of ion channels, ion pumps and some other types of transporters generates, under proper conditions, a voltage gradient over the plasma membrane.
A voltage gradient of e.g., “only” 40 mV over a membrane corresponds to about 50,000 Volts per cm
In eukaryotic cells, the cited voltage gradient over the plasma membrane is, on the average, of the order of 40–80 millivolts, depending on the cell type and the physiological conditions. In unstimulated (= resting) cells, the cytoplasmic side of the plasma membrane is always at negative potential as compared to the extracellular side (). Because the plasma membrane is only about 7–8 nanometers thick, a 40 mV transmembrane potential corresponds to a gradient of about 50,000 Volts per cm, which is huge. In bacteria it is even higher. Thus the electrical dimension of cells is not at all a negligible epiphenomenon; to the contrary, it is of truly vital importance.
Transcellular electric currents
It took until the development of a very sensitive piece of equipment called “the vibrating probe” by Jaffe and NuccitelliCitation17 before it became technically possible to detect weak self-generated electric fields around very small entities, e.g. a single ameba, a root tip, a slime mold, a very young fruit fly embryo etc ().Citation14 Before, one was not even aware that such an invisible extracellular electric field even existed. If e.g., an ameba is surrounded by a self-generated electric field, it means that an ionic current leaves the cell at a particular location of the cell and enters at one or more other sites, this to close the current loops. This apparent polarity is due to the fact that the ion transporting proteins (ion pumps and ion channels) which reside in the plasma membrane are not spherically symmetrically distributed over the whole surface of the cell. They do not float freely in the plane of the (liquid) membrane because they are held in place by various mechanisms, e.g. by being anchored to specific proteins of the cytoskeleton, in particular actin (see later). This way (part of the) pumps and channels can be kept segregated from each other. It is this segregation that enables the transcellular transport of inorganic ions.
The transcellular ionic current system enables that charged macromolecules which are in solution in the cytoplasmic fluid/gel can be forced to undergo “electrophoretic transport.” This system of “self-electrophoresis” was originally discovered in ovarioles (egg tubes) of a silk moth.Citation18 Several years later, it yielded the concept of “the cell as a miniature electrophoresis chamber.”Citation14
Transferability of the cellular electrical system to the next generation(s)
Not a fully inherited, but a shared cell-physiological feature
We usually don't realize that the molecular tools/machinery to generate cellular electricity do not arise de novo at each cell division. When a cell divides (fission in bacteria or mitosis in eukaryotic cells e.g.,) () the existing electrical machinery which is present in the plasma membrane-cytoskeletal complex of the parental cell is divided over the 2 daughter cells. This means that the electricity-generating tool system of cells is transferred to the next generation by sharing. Genetic inheritance of the electrical system only starts playing a role when additional protein molecules with ion transporting activity are needed for keeping the electrical functioning going during the further development and functioning of the daughter cell. The ultimate consequences of this sharing are: 1. The principles of (molecular) genetics do not suffice for explaining the electrical dimension/activity in the 2 daughter cells that arise from cell division; 2. The electrical dimension of all contemporary prokaryotic and eukaryotic cells comes from pinching off/sharing part of part of the parental membrane system, cell generation after generation, up to the ancient Progenote and LUCA (Last Universal Common Ancestor). Thus, any progeny not only inherits its genome from the parent(s), it also receives electrical information by sharing part of the parental “electrome” (see later).
Figure 4. Sharing the ability to generate their own inorganic ion-based “electrical dimension” by cells. (A) For the topic “Cell Division,” textbooks usually focus only on the transfer of genetic information, e.g., during mitosis in a eukaryotic cell. In this approach, the electrical dimension is assumed to arise de novo in the daughter cells and to fully depend on DNA → RNA→ Proteins. (B) At cell division, not only the genome gets divided over the daughter cells, but the existing electrical properties of the parental cell as well and this in both eukaryotic (B) and prokaryotic (C) cells. (D). As the outcome of “the double asymmetry principle,”Citation5,19 all cells of a differentiated multicellular system acquire different membrane properties and can therefore generate an individualized “electrome.” A: from ref. Citation20, B: from ref. Citation21, and C: from refs. Citation20, 21 but modified.
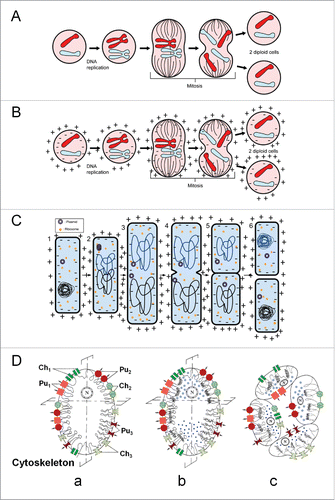
Figure 5. Visualization of the idea of “the electrome of the human brain.” (A) How a wallpaper manufacturerCitation23 depicts activity in the brain. (B) By adding color to some major brain parts, the electrical heterogeneity of the brain is emphasized. In reality each of the approximately 100 billion neurons of the human brain has its own individualized electrome, but such unimaginably high complex system cannot be graphically represented. One should also take into account that the brain does not operate independently from the other cells of the body. The authors of “The embodied Mind”Citation24 pointed to the fact that conceptually brain and body are one, and that one cannot fully understand the functioning of the brain if it is isolated from the other parts of the body. Evidently, the brain is more vital than some other organs because of its coordinating functions. With respect to physics: the ampere is the SI unit for both inorganic ion- and electron-based electric current.
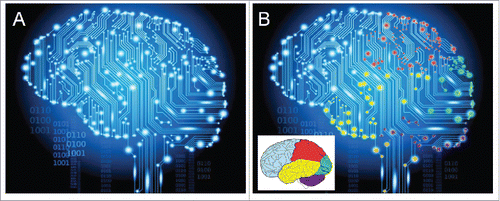
The electrical system of Prokaryotes probably reflects more the “original” system at the onset of Life on earth. It probably relied predominantly on the transmembrane flux/gradient of H+. The more modern ion transporting ATPases for Na+, K+, Na+-K+, Ca2+ and Cl− of eukaryotes are thought to be mutated H+-ATPases.
Maternal versus paternal: The role of the cytoskeleton and symmetry in cell division
In mitosis, the division is more or less symmetrical. Hence the daughter cells are rather alike or identical. During meiosis in animals, cell division is asymmetrical in females but not in males. When the egg cell gets fertilized, the contribution of the plasma membrane-cytoskeletal complex from the spermatozoon, due to its tiny size, is much smaller than that of the oocyte. This means that during early development, thus after the Ca2+ explosion at fertilization (see later), it is the plasma membrane-cytoskeletal complex of the oocyte that almost exclusively accounts for the establishment of the zygote's electrome. Later in development, when de novo protein synthesis starts, both maternal and paternal genomes contribute to the synthesis of ion transporters. Thus, the electrome of the progeny can become a mix of the electromes of both parents.
The cell's “electrome”: A versatile concept
Electrome: A novel term and definition
To facilitate the wording concerning “the electrical dimension of biological systems,” I here introduce a new collective term, namely “electrome.” In analogy with “genome,” “proteome,” “peptidome, “metabolome,” ”connectome” etc., “electrome” stands for the totality of all inorganic-ion (H+, K+, Na+, Ca2+, Cl−, HCO−)-based electrical acts (= ionic currents) of any living entity, from the level of the cell to that of the whole organism. The SI unit for ionic current, thus for the amount of charge carried by inorganic ions transiting per unit time, is the ampere, in the same way as ampere is the SI unit for electron-based electric current.
The variables contributing to the versatility of the electrome
In multicellular organisms, there cannot exist 2 cells with a fully identical electrome. That is due to the fact that many factors influence the electrome's composition. This has been outlined before in the formulation of “The cell as a miniature electrophoresis chamber concept.”Citation14
The major levels which contribute to the establishment of a given ionic environment (ionic concentrations, potential and ionic gradients, ionic currents, secondary chemical gradients) in the cytoplasm and/or in cell organelles e.g. the nucleus, the mitochondria and the endoplasmic reticulum are multiple. The major categories are:Citation14
- | Factors operating at the plasma lemma: here the different types of proteinaceous ion transporters (ion channels, ion pumps, cotransporters etc.) and the ways their activity is regulated are particularly important. | ||||
- | The way channels and pumps are distributed, either spherically symmetrically or asymmetrically, determines whether cells can drive an ionic current through themselves. | ||||
- | Multicellular organisms: not only the total number of cells matters, but the way cells are connected also matters: single cells, electrically coupled cells in multicellular systems etc. | ||||
- | Factors operating in the cytoplasm, e.g., is a particular ion free or is it bound to macromolecules, or is it stored in membrane sacs like a large part of Ca2+? Where in the cell do the cell organelles, e.g. the nucleus, reside? | ||||
- | The membrane properties of membrane-bound cell organelles, e.g., do they harbor ion pumps and channels? | ||||
- | For effects on gene expression, the ionic environment around the chromosomes is important.Citation19 | ||||
- | The lipid composition and fluidity of membranes. Electrophoresis in the plane of the membrane. | ||||
- | The connection between ion transporters and elements of the cytoskeleton, in particular actin.Citation16 | ||||
- | This list is not exhaustive. |
A tentative symbolic notation
In analogy with the symbolic notation for LifeCitation4,5,6 which was cited before (section 2.3), a symbolic notation for electrome at different levels of communicational organization could read:
At the cell- and brain level:
At any level of communicational organization:
S = system; 1 = lowest level of compartmental organization (prokaryotic cell, some cell organelles) in which ionic currents are generated; j: highest level of compartmental organization. These levels are numerous and they are similar to the ≥ 16 levels of communicational organization that can be categorized in living systems;Citation4,5,11 t = moment at which the electrome is measured.
The more cells a tissue, organ, or organism contains, the larger the electrome. The human brain e.g. consists of about 100 billion neurons, all different from each other. In the symbolic notation only the quantitative aspects are taken into account because they are, in principle at least, measurable by electrophysiological and other methods. The qualitative aspects are not quantifiable. Hence this notation can only grab a fraction of the complexity of the electrome.
Given the multitude of influencing candidate actors of the cell's electrical system and their non-spherical distribution over the membranes that are dynamic units by themselves, it follows that there are as many different electromes as there are cells. It also follows that the electrome of a brain is not a static but a dynamic concept: there are no 2 brains with an identical electrome (). Finally, the electrome is subject to developmental changes, both quantitative and qualitative. The electrome of a zygote is smaller than that of an adult. The electrome of the brain of an aged person that has been subject to deleterious aging toxins and other mechanisms may be qualitatively less performant than that of a juvenile.Citation22
Self-generated cellular electricity is truly vital
Neural activity as an example
Like electron-based electricity in our daily lives can be used for many applications, like in lamps, in heating equipment, radios, computers, refrigerators, motors and many more, ion-based biological electricity has also many possible functions (ref. Citation13 and textbooks of physiology). For readers who are not familiar with the biophysical mechanisms of bioelectricity, one example, namely that of an action potential in a neuron is given. The key is that a gradient of both Na+ and K+ over the plasma membrane of an excitable cell type (neuron, muscle cell) has to be generated.
In unstimulated (= resting) neurons, the concentration of Na+ in blood is much higher than in the cytoplasm. The opposite is true for the second major ion involved, namely [K+]. This gradient is generated and maintained by Na++K+-ATPases that reside in the plasma membrane of the neurons. During an action potential the permeability of the plasma membrane for Na+ and K+ changes under the influence of different types of neurotransmitters, neuromodulators etc. In excitatory neurons-neurotransmitters this results in a depolarization of the membrane (= the voltage gradient over the membrane decreases). This change in gradient has to be quickly restored by the activity of Na+-K+-ATPases. Depending upon the system, an action potential usually travels along the axon at a speed of about 80–100 m per second. In inhibitory neurons, the plasma membrane hyperpolarizes, thereby prohibiting that an action potential is launched.
As already cited, our brain contains up to about 100 billion neurons. Most of them make contact, through synapses, with many other neurons. This means that the axon/cable network of the brain is very long, and that the “electrome” of our brain is unimaginably variable and complex. There cannot be 2 brains with the same electrome.
If one thinks about it, the situation is that the seemingly very complex processes in our brain boil down to the regulated and coordinated flux, sometimes low and sometimes high, of mainly Na+ and K+ across plasma membranes of the neurons. Thus cytoplasmic- and transmembrane fluxes of Na+, K+ and Ca2+ to name the major players ultimately enable our sensing and thinking. Does that mean that they, or better the charge they carry, also (partially) shape our “soul”? In my opinion they do.
Are thoughts manifestations of ionic currents in the brain?
Thought is a fundamental activity familiar to everyone. It is not an exclusive activity of humans. What it exactly is and how thoughts are generated remains enigmatic. Linking thoughts to electric (= ionic) currents in the brain may sound a bridge too far for some people. Yet exactly this functional connection is nowadays exploited by engineers who use electric signals captured from a relatively large number of electrodes implanted in the brain. An appealing application and illustration of the possibilities of this technique is that of a monkey that can steer a wheelchair toward a plate with raisins to reward him when he steers in the right direction: see website of the Prof. Miguel Nicolelis laboratory at Duke University in the USA, as well as for information on his Relativistic Brain Theory.Citation25
The electrome is “mortal.” Death of a cell: Like a deflating balloon
Death ensues when the gradient Na+/K+ over the plasma membrane in which [Na+] is higher in blood than in the cytoplasm while the opposite situation prevails for [K+], levels off (= depolarization of the membrane) to equal concentrations on both sides of the membrane, and collapses irreversibly. This happens when the membrane has become excessively leaky and/or when the ion pumps responsible for this gradient became irreversibly inactive. It should not be overlooked that there can also be ionic gradients in the intracellular membrane systems. This is particularly true for Ca2+. Life not only begins with a “Ca2+-explosion” at the moment of fertilization of an egg,Citation26 it also ends with a Ca2+-explosion. Indeed, at death the rough- and smooth endoplasmic reticulum (RER and SER) release the Ca2+ that is stored in their lumen.
Death is then characterized by the absence of the ionic/voltage gradient, not by the disappearance of some inorganic ions or molecules. Thus the total mass of the brain, apart from differences in ionic mass, of dying cells or of the whole organism does not change at death. Therefore in this respect the electrical activity is part of the immaterial dimension of Life. This situation could be compared to pricking an inflated balloon. Inside the balloon, the gas molecules are, due to the higher pressure, closer to each other than outside. Upon pricking the pressure gradient runs down and the distance between the constituting gas molecules normalizes to that of the outside world. Thus, not the gas molecules change, but their gradient. In similar fashion, it is not the total mass or the totality of atoms that changes at death, it is only their interactions that have changed.
The immaterial “Mind- or Spirit part” of living systems: Immortal or not?
The mystery of the storage site and mechanism of cognitive information
Less than half a century ago, the principles governing both the genetic- and cognitive memory systems were unknown. It was believed that the human brain was too underdeveloped to ever understand them. Invoking divine intervention to provide each newborn entity with an individual novel information handling system (genetic and cognitive) was up to then an acceptable approach. Since the discovery of the double helix structure of DNA and the principle of the central dogma of molecular biology DNA → RNA → Proteins,Citation27 heredity has been demystified. Today, apart from some details, genetics and epigenetics are very well understood. This not yet the case for the second memory system, the cognitive one, of which the principles of coding and retrieving stored information remain largely unexplained.Citation27
The first problem in uncovering the biophysical foundations of the cognitive memory is that many biologists continue thinking that they are coded for in full in the genome and the cited central dogma, and that hence, in principle, it should be possible to understand it in full if one applies methods of molecular biology. Of course, proteins are needed for the construction of the compartment in which the cognitive memory operates, namely the cell as a sender-receiver compartment that incessantly handles information. But not all activity in the cognitive memory depends upon (de novo) protein synthesis. The cognitive memory operates at the speed of action potentials, which in animals, travel in axons/neurons at about 80–100 m per second.Citation16 De novo protein synthesis takes at least several to many minutes. Furthermore, if storing additional cognitive information would require de novo synthesis of carrier proteins, the brain of animals as an example would grow continuously unless old storage proteins would be eliminated at the same pace. There is no indication that this happens.
Apart from proteins, at least 3 more players are involved, namely: 1. cellular electricity carried by inorganic ions; 2. the lipid plasma membrane with all its embedded proteinaceous receptors, in particular those that respond to messages delivered by the sense organs; and 3. a storage vehicle for the incoming information. This list is evidently over-simplistic.
Storing cognitive information: Necessary requirements for a good storage system. Cellular networks in brains
Short- and Long term memory: How can cognitive information be stored in cells?
Until recently Long-Term Memory (LTM) was believed to be stored in the brain of animals as changes in synaptic connections. However Chen et al.Citation28 succeeded in dissociating LTM storage and synaptic change in the mollusk Aplysia, a model in neurophysiological research, thereby challenging the idea that stable synapses store long-term memories. This resuscitates the long-standing question how and where short- and long-term memories are stored in cells. Bacteria can learn,Citation29 and neural aspects of biological systems are obvious already in bacteria and other unicellular (eukaryotic) biological units.Citation30 This raises another question, namely whether the memory system(s) in “higher” organisms is still based upon the primordial memory system that came into being along with the Progenote? Given the fact that The First Principles of Physiology are remarkably well conserved in evolution,Citation31 discovering this ur-principle would help to uncover the central dogma of the cognitive memory system.
Theoretically, the ideal storage system would act instantly and storage of useful information should preferably last as long as the cell lives. Only the cell's electric system acts at relatively high speed, at least as compared to the speed of protein synthesis. A photon-based system might be even faster, but apart from some theoretical considerations there is no solid experimental evidence that cellular photonics (which can be measured) play a role in the cognitive memory system. Furthermore, the cognitive system should operate in both prokaryotes and eukaryotes, single celled and multicellular systems. Because prokaryotes can learn, they must have a cognitive memory system. It is unlikely that when eukaryotes came into being, that they started de novo with a different system than the prokaryotes of which they are descendants. The more complex eukaryotes became, the more complex and specialized their memory system became. The brain of animals is a good example. Here networks and numerous synapses are prominent.
It can also be excluded that soluble inorganic ions, thus ions that are not (firmly) attached to a large molecular carrier could by themselves act as a memory-storage system because of lack of specificity and because of the problem of diffusion and transmembrane transport. Lipids and sugars and most proteins do not persist lifelong; their lifespan is limited. Thus they are not good candidates for serving as a material carrier for storing cognitive information. Which molecules remain intact lifelong? Without any doubt, DNA does. But the information that comes from outside the cell has to be transported into the nucleus to the DNA. Thus a carrier is needed that extends from the plasma membrane into the nucleus. In such scenario, the storage system must be a complex, namely the “plasma membrane-cytoskeleton - DNA complex.” But DNA as storage system for cognitive information would only be an option on condition that the cognitive memory acts in a different way than the central dogma of molecular biology.
The cytoskeleton of eukaryotes: Microtubules, actin, tubulin
Another possibility is that very long-lived proteins might act as storage sites. Some proteins last long, e.g., those which serve as anchor for DNA should preferably last as long as DNA itself. Actin not only forms part of the cytoplasmic skeleton but of the nuclear skeleton of eukaryotes as well.Citation32,33 It is highly conserved in evolution. I am not the only one who thinks that, actin, perhaps in the context of the complex cytoskeletal network might, perhaps, be (part of) the seat of the cognitive memory system. In mammals, the second major cytoskeletal protein tubulin is also thought to play a role in learning and memory.Citation34,35
A number of years ago, I became particularly intrigued by the work of Lin and Cantiello.Citation36 They investigated whether actin, one of the most abundant intracellular proteins that forms long linear polyelectric polymers in solution, can conduct electricity. They isolated actin fibers and applied a current at one end and measured whether a signal arrived at the other end. They did experiments under both high (100 mM KCl) and low (1 mM KCl) ionic strength conditions. Electrical currents were observed about the polymer's surface under both conditions. It was stated that these data were consistent with a dynamic behavior of the counterionic cloud surrounding the actin filaments that support ionic movements along the longitudinal axis upon electrical stimulation (). The recordings showed that the counterionic waves were highly nonlinear in nature and that they remained long after the electrical stimulation of the actin filaments had ceased. The authors claimed that they demonstrated that actin filaments can function as biological “electrical wires” that can be conceptualized as nonlinear inhomogeneous transmission lines that may play an important role in coupling intracellular signals.
Figure 6. Possible roles of the cytoskeleton in conducting ionic currents. (A) Prokaryotes have proteins belonging to the same families as the major cytoskeletal proteins of eukaryotes. This strenghtens the idea that cytosketal proteins came into existence long before eukaryotes appeared.Citation38 (B) The cytoskeleton of eukaryotes is a very complex system.Citation39 (C) Actin occurs in 2 forms, G-actin and F-actin.Citation40 The latter is a double helix. (D) Actin filaments support a traveling ionic cloud which is affected by the dipole moments of the monomers (after ref. Citation37). (E) A propagating nonlinear wavetrain of charges following electrical stimulation at one end of an actin filament (After ref. Citation36). (F) Cytoskeletal elements in a neuron according to ref. Citation37 (modified (with permission) by adding actin in the nuclear skeleton (A-NS). Each neuron contains microtubules (MTs) interconnected by MAP2 (in the dendrite) and MAP-tau (in the axon). Connections between MT and actin filaments are shown as well as actin linkage to the membrane.
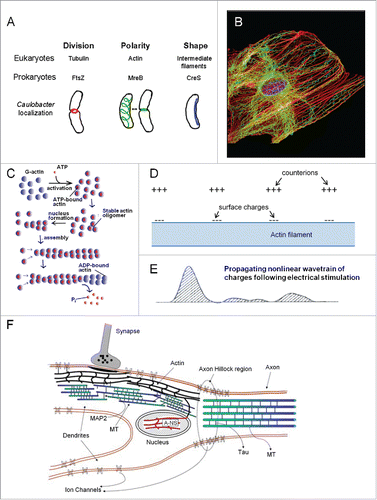
The biophysical principles supporting this hypothesis were further outlined in more detail by Priel et al.Citation37 Actin proteins and microtubules have highly charged surfaces that enable them to conduct electric signals in the form of ionic currents as well as to participate in processing of information. That includes a condensation of counterions on the filament's surface. These authors also advanced a challenging concept, namely that cytoskeletal structures may behave as a liquid state machine. This concept provides a means for real-time computation without the need for stable attractors. It is thought to be compatible with the possibility of a memory effect of such system. It also seems compatible with the view that the cytoskeleton might affect synaptic ion channel function such that the desired state of the channel appears in a higher open probability.Citation37 Cytoskeletal filaments are often directly connected with both ionotropic and metabotropic types of membrane embedded receptors, thereby linking (in neurons) synaptic inputs to intracellular functions.Citation37 The authorsCitation37 propose an information processing model based on cytoskeletal networks that may underlie certain types of learning and memory.
The idea that the cognitive memory might (partially) be based upon “moving forth and back” electrical charges and fix an imprint at a specific location in the cytoskeleton is appealing. Such system is present in all prokaryotic and eukaryotic cells, it is a most versatile system, and it can, in principle, be used for short or for long term purposes. It is very fast and it can be modulated during physiological changes and during development. In my opinion, short- and long-term memory probably use the same electric principles, but that does not at all exclude that they may differ in the “memory proteomes” involved. The key difference between both memory types may be that the stability, meaning the sensitivity to polymerization and depolymerization of cytoskeletal proteins (microtubules (MTs) and actin) varies: some parts may be very unstable in a specific location in the cell, and may be suited for a role in the short term memory only. Other parts of the cytoskeleton may be very stable and may be better suited for storing the stable electric charge imprints that may, perhaps, enable storing cognitive information for longer terms, up to lifelong.
Actin is also a component of the nuclear skeleton
As already cited, Saumann and BerryCitation32 observed that under routine immunocytochemical conditions, no actin could be detected in insect follicle cell nuclei, but that immune- and phalloidin detection of actin became possible after pretreatment with endonucleases to remove a substantial part of chromosomal DNA. In polytene chromosomes of Drosophila, nuclear actin (Actin-related protein Arp-4Citation41) is directly associated with the chromosomes. Now one of its roles is known: it contributes to chromosome movements.Citation33 A considerable amount of actin is present in the filamentous form (F-actin), a double helix, rather than monomeric actin (G-actin). Nuclear actin has also been localized in Xenopus embryo chromosomes, suggesting a role in diploid vertebrate cells as well.Citation32 Recently, improved methods have been developed to visualize F-actin dynamics in axons, resolving unexpected actin behaviors.Citation42
The following challenging idea occurred to me: Could it be that chromosomal actin, or better DNA-associated actin, reflects a primordial system in which the 2 carriers of information, namely DNA as the carrier of genetic/epigenetic information on one hand, and cytoskeletal proteins (in particular actin) as carrier of cognitive information on the other, were initially tightly associated so that they would be concurrently divided over the daughter cells upon cell fission (cell division)? Was perhaps, the original role of actin-like molecules primarily in storing cognitive information in electrical form and was a role in motility secondary? In any case, 2 carriers of information that are both double helices (DNA and F-actin) and that, in eukaryotes at least, are partially intertwined appeals to my imagination. This picture can only hold true if the cytoskeletal proteins that we best known from their presence in eukaryotic cells have counterparts in prokaryotes, the progenitors of the eukaryotes.
The cytoskeleton of bacteria
If the hypothesis that the eukaryotic cytoskeleton plays a key role in storing cognitive information is correct, it is evident that one should ask the question whether or not the eukaryotic cytoskeleton stands on its own, or whether it has been inherited from their prokaryotic ancestors. Presently, whether any of the cytoskeletal proteins plays a role in bacterial memory remains unknown.
A possible solution for the problem of the ancient origin of the cytoskeletal part of the eukaryotic cognitive memory system was found when it was discovered that prokaryotes have actin-like molecules with similar properties to actin of eukaryotes ().Citation43 Wang et al.Citation44 found that the bacterial MreB protein assembles into filaments with a subunit repeat similar to that of the F-actin, the physiological polymer of eukaryotic actin. MreB forms large fibrous (helicalCitation45) spirals under the cell membrane of rod-shaped cells. ParM (= plasmid-encoded actin homolog) and MamK protein are other actin homologs.Citation45-48 They are involved in cell-shape determination, positioning and proteins and nucleoprotein complexes and macromolecular trafficking, etc. Today, the prokaryotic origin of other elements of the eukaryotic cytoskeleton is also well documented. FtsZ is the bacterial homolog of tubulin. It forms a filamentous ring around the middle of the cell.Citation45 It is a key player in cell division of bacteria and some eukaryotic organelles. CreS is a counterpart of intermediate filaments. In short: the cited cytoskeletal proteins are indispensable for cellular tasks that require the cell to accurately position molecules, similar to the function of the eukaryotic cytoskeleton.Citation45 Eun et al.Citation48 emphasize that one should not automatically assume that because cytoskeletal proteins of prokaryotes and eukaryotes belong to the same protein families, that their functions should also be similar. This is clearly not the case for the role of cytoskeletal proteins, in particular actin, for generating a skeleton in bacteria. Their role in prokaryotes is thought to be more important for elaborating new cell walls.
The second source of the “incorporeal nature of the Soul”: Handling of information
Handling information by a sender-receiver
In addition to their electrical dimension, living systems also have another immaterial dimension, thus a dimension (or better activity) without classical mass. It is inherent to their universal architecture as sender-receivers ().
Figure 7. The sender-receiver is the basic unit of architecture and functioning of any living system. From ref. Citation5.
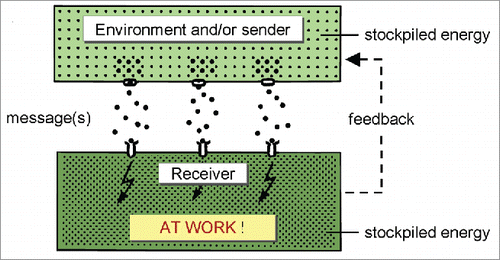
Indeed, the basic activity of all living systems is communication.Citation4,5,6 A simple yet adequate definition for communication is “Communication is transfer of information (in a sender-receiver system).”Citation5
The immaterial nature of information
Like for communication, numerous definitions for “information” have been advanced over the years.Citation5 In essence, information is any propagation of cause and effect in a system. The many definitions that exist are often discipline-specific. My definition of information,Citation5 intended for use in biology, reads: A message contains “information” when, upon being decoded and amplified by a competent (= with matching receptor(s)) receiver, part of the stored energy in that receiver is mobilized sooner or later for doing some sort of ‘work’ (as defined in physics). This can be contraction of muscles, engaging in feedback, activation of the cognitive memory system, activation or inactivation of specific genes etc. This is the meaning of AT WORK in .
Information is itself immaterial, but it usually needs a material carrier (e.g., blood, air, water etc.) for being transported. Under proper conditions, absence of something can act as information and initiate a response, evidently on condition that a decoding program had been installed beforehand in the receiver. Thus this type of information is undeniably immaterial. In case of e.g. a transfer of hormone-carried information, it is the carrier molecule that has mass, not the information it contains. Imagine a bottle containing testosterone molecules standing on a shelf. The testosterone molecules do not carry “androgenic information” as long as they are in the bottle. If the testosterone molecules are administered in proper concentrations to a vertebrate, they will be transported through the blood to the different tissues. They only bring information to those tissues/cells that have (membrane) receptors for testosterone, not to cells devoid of such receptors. Upon binding to a receptor, the testosterone molecule will be internalized. At that moment the testosterone molecule remains intact, meaning that it is not instantly degraded thereby losing mass. The binding to the receptor sets off the cascade for mobilizing energy to do work. Thus it is not the testosterone molecule itself that brings energy, neither does it lose mass. Its presence initiates the mobilization of energy. Thus the information contained in the testosterone molecule is itself immaterial, but it needs a carrier for its transport. The responsive cell can be compared to a mouse trap in which the spring is stretched (= uploaded with energy), waiting for the trigger to be touched.
Another example for the immaterial nature of information; after a text processing program is installed on a computer, the program can be used again and again without losing mass. The memory (e.g., Compact Disk ) in which the program is stored is material and it acts as carrier. The program is a “pathway” that originated in the brain of programmers.
Consciousness
Is consciousness a ubiquitous property of all living systems?
When dealing with the immaterial aspects of Life, one inevitably gets confronted with the most mysterious aspect of all, namely consciousness. In the absence of any known “materialistic” explanation as to its nature, the situation is that there is hardly any possibility to get grip on its nature. The same holds true for “thoughts.” While I think that consciousness must be based upon some universal biophysical principle, there is a vast body of literature that places consciousness outside the “material world.”Citation49-54 Because I am not a specialist in this domain, I present my ideas on consciousness as those of “an unbiased outsider-observer with a background in the exact sciences.”
I like the definition of consciousness formulated by Trewavas and BaluskaCitation8: “In the simplest sense, consciousness is an awareness of the outside world,” as well as their view that consciousness is not at all restricted to the Homo sapiens, neither to animals but that it is a universal property of all living systems. This view is compatible with evolutionary theory.Citation11,12
Stating that an organism is aware of the outside world means that it gets input on the conditions of the environment. All sensor systems in the body of an animal, for light, sound waves, odors, smells, touch etc. use the ionic current system of the nervous system to convey the information to specific parts of the brain. The brain has also receptors for many different types of internal signaling molecules, e.g. hormones. Many receptors reside in the plasma membrane and use secondary messengers for signal transduction. A key second messenger is Ca2+. Calcium currents are physiologically very important.Citation55-60 Thus, the awareness of the outside and the inner world involves a very complex, incessant change in the electrical activity, thus in the electrome of the brain which is an electrical representation/image of the perceived conditions in the external and internal environments. The electrome of the whole brain totals the sub-electromes of the various parts of the brain that represent in their totality the individual cellular electromes of all interconnected brain cells ().
As stated before, the biophysical principle enabling consciousness must have been present in the Progenote in the form of a First Physiological Principle.Citation31 Such principles have been conserved ever since. It means that non-animal organisms too must have and use the same principles. The difference is then only that consciousness is distributed over the entire organism, e.g., over the whole plant. Plants not only have all 5 of our senses, but 15 more.Citation61 In an anthropocentric world view that usually considers plants as unthinking and inert, such view may meet with unbelief. It has drastic consequences for thinking on what is “ethical behavior” in a multi-organismal environment, in particular with respect to “what type of food is ethical to eat.”
Does consciousness have an electrical component? Is “electroconsciome” a useful term?
The key issue in the discussion on consciousness is, in my opinion: “Does what we call “(ubiquitous) consciousness” equal the totality of electrical phenomena in any given communicating compartment (a cell, our brain, the totality of a plant etc.), or is the electrical activity only the prerequisite for enabling consciousness that then would be of a different nature than electrical?
I favor the idea that “electrome” and “consciousness” are largely overlapping concepts but given that experimental evidence is missing, I am also open to the view the electrical aspect is only part of consciousness. I think that as long we have not uncovered the biophysics of the cognitive memory system, nobody is able to answer this question.
A catchy one-liner for defining a living system
Taking into account all what has been said before on matter as fossil stardust and on the ability of living systems to generate their own electricity and to communicate, one could say that: “Living systems are self-electrifying and talking aggregates of fossil stardust.”
Final conclusions
Living systems have 2 memory systems, each with their own rules. The principles of the genetic and epigenetic memory are already well understood. Those of the immaterial aspects of Life which are linked to the cognitive memory-system continue to be more enigmatic, but progress is made.
Models for future research
The elucidation of the principles of genetics in the 20th century involved, as a start, the analysis of the genome of a simple cellular model, namely Escherichia coli. That paved the way to the later mapping the genome of eukaryotes, some with a small, others with a large genome. In my opinion, understanding the biophysics of the cognitive memory will also have to start from a simple but relatively large model organism, e.g. a unicellular eukaryote like an ameba, even if some amebae have a large genome. E.g. the genome of Amoeba dubia contains about 670 billion base pairs, over 200 times larger than in humans, and the one of Amoeba proteus has 290 billion.Citation62 The problem is that for the analysis of the genome, extracts can be used. This is less the case for the cognitive memory because homogenization kills the electrical dimension. Yet, for studying the possible role of some cellular constituents, e.g., elements of the cytoskeleton, in vitro experiments are possible as already shown by Lin and Cantiello.Citation36 A next generation of ultra-nanotechnologies will likely be needed. Also, the role of intracellular ionic currents will have to be functionally analyzed. The late Professor Lionel Jaffe was a pioneer in documenting slow and fast Ca2+-currents.Citation55-60 The role of such currents remains enigmatic. Ca2+ is so important for a variety of cellular functions, that an important role in the cognitive memory can be anticipated. With respect to the cognitive memory, a working hypothesis could be that the electricity inherent to action potentials plays a role in adducing cognitive information coded in moving waves of counterions. Whether the Ca2+ currents that arise inside the cell are part of that mechanism, or/and that they may, perhaps, also be needed for retrieving stored information remains to be further elucidated.
Discussion
This paper aims at showing that some aspects of “the immaterial aspect-dimension of Life” can be understood as normal, intelligible properties of living systems/compartments. It is inherent to their construction as sender-receivers that incessantly handle immaterial information, and that need self-generated electricity, also something invisible but nonetheless measurable, to do so. Thus no need to invoke an “Absolute Consciousness” or “Cosmic consciousness” and statements like “Why Biology is beyond physical sciences”Citation2 to deal with (some of?) the immaterial aspects of Life. I agree with Caetano-AnollésCitation3 that biological systems do obey the laws of (bio)chemistry, physics and above all, of communication.Citation3,5
In medical- and neurobiological sciences, the role of inorganic ions in physiology is well documented, in particular in the functioning of neurons, muscles and kidneys. For them, the fact that death ensues when the self-generated electrical dimension of a cell(complex) irreversibly collapses is nothing new. In physiology, this electric dimension is neither “incorporeal,” neither immortal. After collapse of the electrome, the participating ions are still around. They only thing that changed is that the gradient in which they (in particular Na+, K+, Cl− and Ca2+) occurred on both sides of the plasma membrane is gone: the system returned from its situation “far from thermodynamic equilibrium” to “equilibrium.” At death, consciousness at the cellular level as well as the cognitive memory system are irreversibly lost along with the dissipation of the electrical gradients.
The major challenge for Biology for the near- and hopefully not too distant future is the deciphering of the biophysical principles underlying the cognitive memory. Self-generated cellular electricity is undoubtedly a major player. It can be expected that further advancements in nanotechnology will be required to enable substantial progress in better mapping of electromes.
If the “electrome equals part of the classical “incorporeal soul” hypothesis would gain credibility, despite the (massive?) rejection that it may encounter in some circles upon first contact, it may have far-reaching consequences for the exact biological-medical sciences, and even more for psychology, philosophy and religion. The idea that plants, fungi, bacteria have an “electrome-soul” that is based upon the same principles as that of the Homo sapiens is indeed challenging in many aspects.
Disclosure of potential conflicts of interest
No potential conflicts of interest were disclosed.
Acknowledgments
My sincere thanks to the anonymous authors of (parts of) from Wikipedia and other sources,Citation20,37-39 and of from Wallpaper HD,Citation23 and in particular to Marijke Christiaens and Julie Puttemans for their invaluable help in the preparation of all figures. My thanks as well to Michael Gaffney for text correction and discussion.
References
- Shanta BN. Life and consciousness – The Vedāntic view. Communicative Integrative Biol 2015; 8(5):e1085138; http://dx.doi.org/10.1080/19420889.2015.1085138
- Shanta BN, Muni BV. Why biology is beyond physical sciences? Adv Life Sci 2016; 6:13-30; http://dx.doi.org/10.5923/j.als.20160601.03
- Caetano-Anollés G. Creationism and intelligent design are incompatible with scientific progress: A response to Shanta and Vêdanta. Communicative Integrative Biol 2016; 9(1):e1123356; http://dx.doi.org/10.1080/19420889.2015.1123356
- De Loof A, Vanden Broeck J. Communication: the key to defining “life”, “death” and the force driving evolution. “Organic chemistry-based-” versus “artificial” life”. Belg J Zool 1996; 125:5-28.
- De Loof A. Communication, Life, Mega-Evolution. Decrypting Life's Nature. Leuven, Belgium: Leuven University Press; 2002.
- De Loof A. How to deduce and teach the logical and unambiguous answer, namely L = ∑C, to “What is Life?” using the principles of communication? Communicative Integrative Biol 2015; 8:e1059977; http://dx.doi.org/10.1080/19420889.2015.1059977
- Edelman DB, Baars BJ, Seth AK. Identifying hall marks of consciousness in non-mammalian species. Conscious Cogn 2005; 14:169-187; PMID:15766896; http://dx.doi.org/10.1016/j.concog.2004.09.001
- Trewavas AJ, Baluška F. The ubiquity of consciousness. EMBO Rep 2011; 12:1221-1225; PMID:22094270; http://dx.doi.org/10.1038/embor.2011.218
- Buhner SH. Plant intelligence and the imaginal realm: Beyond the door of perception into the dreaming of earth. Rochester, VT: Bear & Company (1 edition) 2014.
- Schejter A, Agassi J. On the definition of life. J Gen Phil Sci 1994; 25:97-106; http://dx.doi.org/10.1007/BF00769279
- De Loof A. Organic and cultural evolution can be seamlessly integrated using the principles of communication and problem-solving: The foundations for an extended Evolutionary Synthesis (EES) as outlined in the Mega-Evolution concept. LEB 2014; 2(4):247-269; http://dx.doi.org/10.9784/LEB2(4)DeLoof.01
- De Loof A. From Darwin's On the Origin of Species by Means of Natural Selection… to The evolution of Life with Communication Activity as its very essence and driving force (= Mega-Evolution). LEB 2015; 3(3):153-187; http://dx.doi.org/10.9784/LEB3(3)DeLoof.01
- Koshland DE. The seven pillars of life. Science 2002; 292:2215-2216; http://dx.doi.org/10.1126/science.1068489
- De Loof A. The electrical dimension of cells: The cell as a miniature electrophoresis chamber. Int Rev Cytol 1986; 104:251-352; PMID:3531065; http://dx.doi.org/10.1016/S0074-7696(08)61927-0
- Wikipedia: Electric fish. [accessed 2016 Apr 4]. http://en.wikipedia.org/wiki/Electricfish
- Tuszyński JA, Portet S, Dixon JM, Luxford C, Cantiello HF. Ionic wave propagation along actin filaments. Biophys J 2004; 86:1890-1903; PMID:15041636; http://dx.doi.org/10.1016/S0006-3495(04)74255-1
- Jaffe LF, Nuccitelli R. An ultrasensitive probe for measuring steady electric extracellular electric currents. J Cell Biol 1974; 63:614-628; PMID:4421919; http://dx.doi.org/10.1083/jcb.63.2.614
- Woodruff RI, Telfer WH. Electrophoresis of proteins in intercellular bridges. Nature 1980; 286:84-86; PMID:7393329; http://dx.doi.org/10.1038/286084a0
- De Loof A. Differentiation: “Keep the genome constant but change over and over again its ionic and/or macromolecular environment”. A conceptual synthesis. Belg J Zool 1993; 123:77-91.
- Wikipedia: Mitosis. [accessed 2016 Apr 4]. http://en.wikipedia.org/wiki/Mitosis
- Wikipedia: Fission (biology). [accessed 2016 Apr 4]. http://en.wikipedia.org/wiki/Fission(biology)
- De Loof A, De Haes W, Boerjan B, Schoofs L. The fading electricity theory of ageing: the missing biophysical principle? Ageing Res Rev 2013; 12:58-63; PMID:22940501; http://dx.doi.org/10.1016/j.arr.2012.08.001
- WallpaperHD. http://www.wallpaperhd.pk/machine-learning-smart-brain-wallpaper
- Varela F, Thompson E, Rosch E. The embodied mind: cognitive science and human experience. Cambridge, MA: MIT Press; 1992.
- Nicolelis G, Cicurel R. The relativistic brain: How it works and why it cannot be simulated by a Turing machine. Natal, Montreux, Durham, Sao Paulo: Kios Press; 2015.
- Ridgway EB, Gilkey JC, Jaffe LF. Free calcium increases explosively in activating medaka eggs. Proc Natl Acad Sci U.S.A. 1977; 74:623-627; http://dx.doi.org/10.1073/pnas.74.2.623
- Crick F. Central dogma of molecular biology. Nature 1970; 227:561-563; PMID:4913914; http://dx.doi.org/10.1038/227561a0
- Chen S, Cai D, Pearce K, Sun PY-W, Roberts AC, Glanzman DL. Reinstatement of long-term memory following erasure of its behavioral and synaptic expression in Aplysia. eLife 2014; 3:e03896; PMID:25402831
- Shapiro JA. Bacteria are small but not stupid: cognition, natural genetic engineering and socio-bacteriology. Studies Hist Philos Sci 2007; 38:807-819.
- Baluška F, Mancuso S. Deep evolutionary origins of neurobiology. Turning the essence of ‘neural’ upside-down. Communicative Integrative Biol 2009; 2(1):60-65; http://dx.doi.org/10.4161/cib.2.1.7297
- Torday JS. The emergence of Physiology and Form: Natural selection revisited. Biology 2016; 5(2):15; http://dx.doi.org/10.3390/biology5020015
- Sauman I, Berry SJ. An actin infrastructure is associated with eukaryotic chromosomes: structural and functional significance. Eur J Cell Biol 1994; 64:348-356; PMID:7529180
- Spichal M, Brion A, Herbert S, Coumac A, Marbouty M, Zimmer C, Koszul R, Fabre E. Evidence for action dual role in regulating chromosome organization and dynamics in yeast. J Cell Sci 2016; 129(4):681-92; Jan 13. ppi: jcs.175745; PMID:26763908
- Li L, Yang XJ. Tubulin acetylation: responsible enzymes, biological functions and human diseases. Cell Mol Life Sci 2015; 72:4237-4255; PMID:26227334; http://dx.doi.org/10.1007/s00018-015-2000-5
- Craddock TJ, Hameroff SR, Ayoub AT, Klobukowski M, Tuszynski JA. Anesthetics act in quantum channels in brain microtubules to prevent consciousness. Curr Top Med Chem 2015; 15:523-533; PMID:25714379; http://dx.doi.org/10.2174/1568026615666150225104543
- Lin EC, Cantiello HF. A novel method to study the electrodynamic behavior of actin filaments. Evidence for cable-like properties of actin. Biophys J 1993; 65:1371-1378; PMID:8274631; http://dx.doi.org/10.1016/S0006-3495(93)81188-3
- Priel A, Tuszyński JA, Woolf NJ. Neural cytoskeleton capabilities for learning and memory. J Biol Phys 2010; 36:3-21; PMID:19669423; http://dx.doi.org/10.1007/s10867-009-9153-0
- Wikipedia: Prokaryotic cytoskeleton. [accessed 2016 Apr 4]. http://en.wikipedia.org/wiki/Prokaryotic cytoskeleton
- Wikipedia: Cytoskeleton. [accessed 2016 Apr 4]. http://en.wikipedia.org/wiki/Cytoskeleton
- Wikipedia: Actin. [accessed 2016 Apr 4]. http://en.wikipedia.org/wiki/Actin
- Frankel S, Sigel EA, Craig C, Elgin SC, Mooseker MS, Artavanis-Tsakonas S. An actin-related protein in Drosophila colocalizes with heterochromatin protein 1 in pericentric heterochromatin. J Cell Sci 1997; 110:1999-2012; PMID:9378752
- Ladt K, Ganguly A, Roy S. Axonal actin in action: Imaging actin dynamics in neurons. Methods Cell Biol 2016; 131:91-106; PMID:26794509; http://dx.doi.org/10.1016/bs.mcb.2015.07.003
- Van den Ent F, Amos LA, Löwe J. Prokaryotic origin of the actin cytoskeleton. Nature 2001; 413:39-44; PMID:11544518; http://dx.doi.org/10.1038/35092500
- Wang H, Xie L, Luo H, Xie J. Bacterial cytoskeleton and implications for new antibiotic targets. J Drug Target 2016; 24:392-398; PMID:26548775; http://dx.doi.org/10.3109/1061186X.2015.1095195
- Löwe J, van den Ent F, Amos LA. Molecules of the bacterial cytoskeleton. Annu Rev Biophys Biomol Struct 2004; 33:177-198; PMID:15139810; http://dx.doi.org/10.1146/annurev.biophys.33.110502.132647
- Carballido-López R. The bacterial actin-like cytoskeleton. Microbiol Mol Biol Rev 2006; 70:888-909; http://dx.doi.org/10.1128/MMBR.00014-06
- Shaevitz JW, Gitai Z. The structure and function of bacterial actin homologs. Cold Spring Harb Perspect Biol 2010; 2(9):a000364; PMID:20630996; http://dx.doi.org/10.1101/cshperspect.a000364
- Eun Y-J, Kapoor M, Hussain S, Garner EC. Bacterial filament systems: toward understanding their emergent behavior and cellular functions. J Biol Chem 2015; 290:17181-17189; PMID:25957405; http://dx.doi.org/10.1074/jbc.R115.637876
- Wikipedia: Consciousness. [accessed 2016 Apr 4]. http://en.wikipedia.org/wiki/Consciousness
- Ryle G. The concept of mind. Chicago, IL: University of Chicago Press; 1949.
- Perreira Jr. A, Ricke H. What is consciousness? Towards a preliminary definition. J Consciousness Stud 2009; 16:28-45.
- Velmans M. Understanding Consciousness. Edition 2. Adingdon, UK: Routledge/Psychology Press (Taylor and Francis Group); 2009.
- Vimal R. Meanings attributed to the term ‘Consciousness’: an overview. J Consciousness Stud 2009; 16:9-27.
- Dehaene S. Consciousness and the brain: Deciphering how the brain codes our thoughts. London, UK: Penguin Books; 2014.
- Jaffe LF. Control of development by steady ionic currents. Fed Proc 1981; 40:125-127; PMID:7193143
- Jaffe LF. The path of calcium in cytosolic calcium oscillations: a unifying hypothesis. Proc Natl Acad Sci U.S.A. 1991; 88:9883-9887; http://dx.doi.org/10.1073/pnas.88.21.9883
- Jaffe LF. Classes and mechanisms of calcium waves. Cell Calcium 1993; 14:736-745; PMID:8131190; http://dx.doi.org/10.1016/0143-4160(93)90099-R
- Jaffe LF. Fast calcium waves. Cell Calcium 2010; 48:102-113; PMID:20883893; http://dx.doi.org/10.1016/j.ceca.2010.08.007
- Jaffe LF, Nuccitelli R. Electrical controls of development. Annu Rev Biophys Bioeng 1977; 6:445-476; PMID:326151; http://dx.doi.org/10.1146/annurev.bb.06.060177.002305
- Jaffe LF, Woodruff RI. Large electrical currents traverse developing Cecropia follicles. Proc Natl Acad Sci U.S.A. 1979; 76:1328-1332; http://dx.doi.org/10.1073/pnas.76.3.1328
- Marcuso S, Viola A. Brilliant Green: The surprising history and science of plant intelligence. Washington DC: Island Press; 2015. ISBN-13: 978-1610916035.
- Winstead ER. Sizing up genomes: amoeba is king. GNN (Genomes News Network) 2001 Feb; 12:2001.