ABSTRACT
Leaf litter chemical traits were measured for Cycas micronesica plants in Guam following leaf herbivory by the scale Aulacaspis yasumatsui, the butterfly Chilades pandava caterpillar, or the leaf miner Erechthias sp. to determine the influence of the non-native pests on litter quality. Scale herbivory increased litter phenols above those of undamaged leaves but did not influence lignin or cellulose concentrations. Butterfly caterpillar herbivory increased litter phenols above and decreased litter lignin below those of undamaged leaves, but did not influence cellulose concentrations. Leaf miner herbivory increased litter lignin concentrations above those of undamaged leaves, but did not influence phenols or cellulose concentrations. Herbivory influenced 8 of 12 essential elements that were quantified. Herbivory by all 3 insects increased nitrogen and potassium litter concentrations and decreased calcium and iron litter concentrations when compared with undamaged litter. The responses were idiosyncratic among herbivores for the remaining essential elements. Stoichiometry among the chemical constituents indicated that herbivory increased litter quality and predicted more rapid biogeochemical cycling in Guam's ecosystems as a result of these 3 non-native insect invasions.
Introduction
Cycas micronesica was the most abundant tree species in Guam's forests as recently as 2002.Citation1 The invasions of the armored scale Aulacaspis yasumatsui and the microlepidopteran leaf miner Erechthias sp were documented in 2003.Citation2,3 The subsequent invasion of the butterfly Chilades pandava was documented in 2005.Citation4 The interactions of these 3 non-native pests were dissimilar as they enacted a concerted attack on Guam's insular C. micronesica population.Citation5 Epidemic mortality of the C. micronesica population was well-studied in the years following the invasions.Citation6
Direct consequences of insect herbivory such as plant mortality or the physical removal of tissue from plant organs are readily observed. But the indirect consequences of insect herbivory are also of critical importance at organismal and system levels, and include modifications of leaf and litter biochemistry and speed of litter decomposition.Citation7-9 Various ex situ and in situ conservation programs have been enacted to conserve Guam's remaining C. micronesica populations.Citation10 An understanding of how these non-native pests are affecting system-level dynamics will improve management decisions in these conservation programs.
Our objectives were to determine chemistry of C. micronesica leaf litter following herbivory by these 3 non-native insects. We focused on chemical traits that are known predictors of litter qualityCitation11 in order to forecast how altered leaf chemistry may change biogeochemical processes.
Results
Insect herbivory influenced the total phenols in C. micronesica leaf litter (P = 0.001). Armored scale infestations generated a 65% increase and butterfly caterpillar herbivory generated a 78% increase above that of undamaged leaves (). Contrarily, total phenols in litter following leaf miner herbivory were not different from those of undamaged leaf litter. Herbivory also influenced lignin in leaf litter (P < 0.001). Butterfly caterpillar herbivory caused a decline in lignin to 78% of that of control litter and leaf miner herbivory caused an increase in lignin to 117% of that of control litter (). The lignin concentration in litter damaged by armored scale infestations was not different from that of undamaged leaf litter. Cellulose concentration was not influenced by herbivory (P = 0.1348), and the overall mean was 242 mg·g−1.
Figure 1. The influence of Aulacaspis yasumatsui, Chilades pandava, and Erechthias sp. leaf herbivory on Cycas micronesica leaf litter chemistry. Means ± SE, n = 10. (A) Total phenols (GAE = gallic acid equivalent). (B) Lignin.
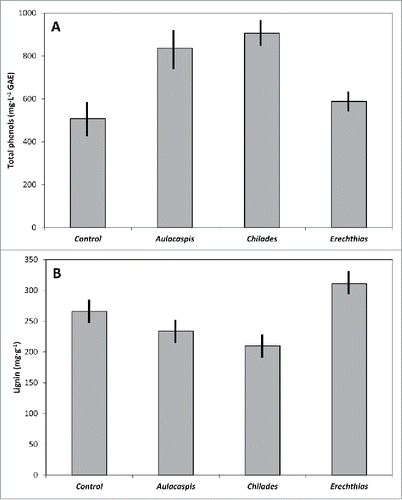
Leaf herbivory also influenced elemental concentrations of C. micronesica leaf litter (). Of the numerous elements that we measured, only carbon, phosphorus, copper, and zinc were unaffected by herbivory. Herbivory by all 3 insects increased nitrogen and potassium concentrations above those of undamaged leaf litter. All three insects decreased calcium and iron concentrations below those of undamaged leaf litter. Magnesium, sulfur, manganese, and boron concentrations were idiosyncratic, with no general trend emerging from the data. Armored scale and leaf miner leaf herbivory decreased magnesium and sulfur concentrations below those of undamaged leaves, but butterfly damage did not influence magnesium or sulfur concentrations. Armored scale and butterfly caterpillar damage decreased manganese concentrations below those of undamaged leaves, but leaf miner damage did not influence manganese concentrations. Leaf miner damage decreased boron concentration below that of undamaged leaves, but armored scale and butterfly damage did not influence boron concentrations.
Table 1. The influence of Aulacaspis yasumatsui, Chilades pandava, or Erechthias sp herbivory of Cycas micronesica leaflets on leaf litter macro and micronutrients. Means ± SE, n = 10.
Potassium and iron litter concentrations exhibited the greatest response to herbivory by these 3 non-native insects (). Leaves damaged by armored scale had 6.45-fold greater potassium concentration in litter than undamaged leaves. Undamaged leaves had 4.25-fold greater iron concentration in litter than leaves damaged by armored scale.
Quotients that were calculated with carbon or lignin concentration as the numerator exhibited similar trends among the nutrients used as denominator, with litter from undamaged leaves exhibiting the greatest values (). Quotients that were calculated with phenolics as the numerator exhibited more idiosyncratic responses among the nutrients that were used as denominator. Phenolics:nitrogen of litter from undamaged leaves was less than that of litter from insect-damaged leaves, and increased in the order scale<butterfly<leaf miner. Phenolics:phosphorus of litter from scale and butterfly caterpillar damaged leaves was similar, and was greatly decreased below that of undamaged leaves. Phenolics:phosphorus of litter from undamaged leaves did not differ from that of leaf miner damaged leaves. Both scale and butterfly caterpillar damage decreased phenolics:potassium below that of undamaged leaves, with scale damage decreasing the quotient to a greater degree. Phenolics:potassium of litter from undamaged leaves did not differ from that of leaf miner damaged leaves.
Table 2. The influence of Aulacaspis yasumatsui, Chilades pandava, or Erechthias sp. herbivory of Cycas micronesica leaflets on derived stoichiometric traits of leaf litter. Means ± SE, n=10.
The quotients with potassium as the denominator exhibited the greatest variability among the leaf damage types. Carbon:potassium of undamaged leaf litter was 8.4-fold greater than, lignin:potassium of undamaged leaf litter was 9.2-fold greater than, and phenolics:potassium of undamaged leaf litter was 6.6-fold greater than that of scale-damaged leaf litter.
Discussion
Infestations of A. yasumatsui influenced chemical constituents that persisted in soils following epidemic C. micronesica plant death,Citation12 providing indirect evidence that the infestations altered biochemistry of litterfall.Citation13 Herein we have provided direct evidence of this leaf response. To our knowledge, this represents the first study for any extant Cycadales species that reports chemical changes of plant tissue following herbivory. Leaf tissue herbivory by these non-native insects caused substantial alterations in the concentration and stoichiometry of essential elements and secondary compounds in C. micronesica leaf litter. Some of these changes in chemistry are known predictors of litter decomposition speed. As a result, these findings indicate substantial changes in nutrient cycling and cascading changes in ecosystem function as a result of the damage imposed by these invasive pests.
The stoichiometric relations of litter constituents () collectively predicted that C. micronesica litter decomposition will be more rapid following leaf herbivory by these insects, as decreased values of the reported quotients are associated with increased decomposition speed. The relationships with phenolics as the numerator were more variable than with carbon or lignin. The relationships with potassium as the denominator were more variable than with nitrogen or phosphorus. Therefore, litter tissue phenolics and potassium may be highly influential in controlling herbivore-damaged C. micronesica leaf litter decomposition. The mean of all 9 quotients in may provide a generalized estimate of litter decomposition speed, and this mean predicted that decomposition would be most rapid for leaf litter damaged by butterfly caterpillars. Based on this predictor, litter decomposition speed will proceed in the order butterfly>scale>leaf miner>undamaged leaves. However, the trends among the leaf damage types were not homogeneous for the response variables, so litter decay incubation studies will be required to unambiguously determine the influence of insect herbivory on nutrient turnover traits.
Changes in stoichiometry of host leaf compounds and nutrient elements may be generated by insect herbivores through various mechanisms. The most studied of those mechanisms have been disruption of natural resorption efficiency through accelerated leaf abscission, which may increase litter quality, and induction of defensive secondary compounds, which may decrease litter quality. Citation7,14, 15
Our study illuminated 2 additional mechanisms that, to our knowledge, have not been explicitly discussed in relation to post-herbivory leaf stoichiometry. First, post-herbivory leaf chemistry is unquestionably influenced by selective consumption of leaf constituents by the herbivore. This culling of some leaf constituents while other constituents remain intact may profoundly change the stoichiometry of leaf biochemicals that persist through to litterfall and that move up the food chain. A behavior that benefits some cycad herbivores involves removal and sequestering of specific plant toxins after feeding on cycad tissue.Citation16,17 Moreover, the phenomenon of elemental stoichiometry among trophic components of various systems has been used to explain numerous components of ecosystem function.Citation18-21 Second, leaf age at the time of herbivory may also directly mediate post-herbivory leaf chemistry through differences in the extent and duration of leaf maturation after herbivory. To our knowledge, these 2 influential factors have not been explicitly discussed within the context of changes in litter chemistry following insect herbivory.
The disparate feeding guilds of the 3 insects we studied may profoundly influence the preferential consumption of varied leaf constituents. For example, the homopteran feeding style of A. yasumatsui removes biochemicals but does not remove entire sections of mesophyll or dermal tissue. Therefore, many chemicals within the structural tissues of the laminae persist throughout the piercing-sucking feeding by this armored scale. In contrast, the lepidopteran chewing style of Chilades pandava caterpillars removes all leaf constituents in a homogeneous manner from the portions of the leaflets that are damaged because the entire lamina cross section is consumed during feeding. The influence of the microlepidopteran Erechthias larvae may be intermediate, because all mesophyll tissue is consumed within the tunnels created by the leaf miner larvae while epidermal tissue is left intact. Therefore, biochemicals that are preferentially loaded into epidermal cells may increase in relative concentration after leaf miner feeding, while biochemicals that are preferentially loaded into mesophyll cells may be entirely removed or decrease in relative concentration. The influence of herbivore feeding guild on induced plant defensive compounds has been discussed,Citation22 but to our knowledge the influence on plant elemental stoichiometry following selective removal of elements has not.
The timing of herbivory by the 3 insects we have studied is highly contrasting. For example, oviposition of eggs by Chilades pandava is restricted to palatable, expanding leaves. Therefore, continued expansion and maturation of the leaves after partial leaf consumption may provision those leaves with a greater potential for induced chemical responses and sustained nutrient import. In contrast, oviposition of eggs by the leaf miner Erechthias is restricted to old leaves.Citation2 Therefore, the potential for induced biosynthesis of metabolites and sustained nutrient import following herbivory may be minimal even if the host plant species is equipped with the machinery for induced defenses, merely because leaf maturation preceded the time of herbivory. The armored scale A. yasumatsui can infest leaves of any age, but because of the speed of leaf expansionCitation23 the scale usually colonizes leaf surfaces after full leaf expansion. Clearly, timing of herbivory per se may directly affect C. micronesica leaf chemistry following herbivory.
Cycad plants associate with nitrogen-fixing cyanobacteria within specialized roots.Citation24 The uninterrupted access to newly fixed nitrogen may enable cycad plants to maintain a homeostasis of leaf nitrogen concentration throughout heterogeneous soil conditions and herbivory pressures. Therefore, nitrogenous leaf constituents that are removed during herbivory may be more easily replaced than constituents that rely on other soil-derived elements.
Our focus was on C. micronesica leaf litter quality as affected by non-native insect herbivory. This leaf response represents one of many ways that insect herbivory may affect soil nutrient relations. Other mechanisms that generate afterlife effects of herbivory on ecosystem nutrient cycling include additions of excrement produced by insects, additions of fresh leaf pieces dislodged by herbivory, additions of dead insect bodies, changes in throughfall chemistry, changes in plant community richness or density through preferential host plant choice and selective plant mortality, and changes in litter decomposition through changes in canopy light gap dynamics.Citation25,26
Our case study provides a stark example of how invasive insects can change community structure through host plant choice and selective mortality. These invasions preferentially culled C. micronesica plants from Guam's habitats, reducing plant density in some habitats from 3,850 to 290 plants per hectare in 6 y.Citation6 We have argued that the changes in ecosystem function following selective removal of foundation species that have no redundant functional species are far greater than changes following removal of a rare species or a species with functionally redundant species.Citation27 Indeed, the once-dominant C. micronesica was listed under the IUCN Red ListCitation28 and the United States Endangered Species ActCitation29 as a result of the insect invasions, emphasizing the drastic changes in forest structure. Moreover, C. micronesica is the only native gymnosperm in the Mariana Islands, so it is phylogenetically unique. From the 10 most abundant native tree species on Guam,Citation1 C. micronesica is the only species with nitrogen-fixing root symbionts. Guam's C. micronesica trees are hosts for Dihammus marianarum, a native Cerambycidae beetle that feeds on stem tissue, and the native pollinator Anatrachyntis sp. that feeds on dispensable male cone tissue,Citation2,30 illuminating the co-extinction threats that result from loss of the tree species. Undoubtedly, inclusion of direct measurement of every means by which non-native insect herbivory influences plant communities is needed in future studies and can improve understanding of plant population ecology, evolution, chemical ecology, and invasion biology.Citation31-36
Plants that are endemic to oceanic islands are naïve in many respects, and C. micronesica evolved in the absence of any known native leaf herbivore. Therefore, the evolutionary pressures that may have selected for constitutive and induced chemical defenses to leaf herbivory were previously absent. An interesting question that remains to be answered is how the post-herbivory chemical responses of C. micronesica and other naïve Cycas species that did not co-evolve with a leaf-feeding herbivore differ from those of the Cycas species that did co-evolve with a leaf-feeding insect. We have reported highly contrasting levels of damage among Cycas species caused by Chilades pandava herbivory in a common garden setting.Citation37,38 The results lend evidence that constitutive chemical deterrents are highly heterogeneous among the Cycas species, and perhaps the species that co-evolved with Chilades pandava also possess greater inducible chemical responses to leaf infestations of insect herbivores.
The paucity of studies assessing the changes in litter chemical traits following damage by invasive insects constitutes a clear research need that can greatly improve our understanding of the manifold cascading responses to alien invasions. Considering the growing appreciation for the importance of ionomicsCitation39 and the communication role for many ions in plant physiology, these non-native pests are likely altering C. micronesica plant biology in numerous ways that are not presently understood.
We selectively harvested from plants that exhibited herbivory by only one of the 3 insects for this first look at host plant responses. Most published studies on plant-insect interactions have also focused on single herbivores. More in-depth studies on how these 3 herbivores interact during sequential or simultaneous infestations of C. micronesica plants may yield results that cannot be predicted from single herbivore studies.Citation40-42
In summation, invasive specialist herbivores have the power to devastate native plant populations. Our study from an oceanic island revealed how non-native insects can also alter chemical traits of litterfall from a native host species. Chemical signatures in C. micronesica leaves before and after damage by the 3 studied insects predicted profound changes to ecosystem processes. The various management plots that have been constructed for ex situ and in situ conservation of C. micronesica are ideally positioned to quantify the divarication of biogeochemical processes within the managed plots from those processes in adjacent unmanaged plant populations that are being threatened by these specialist pests.
Materials and methods
Ten locations within natural C. micronesica populations were identified in Dec. 2011 and defined as 10 replications from which leaflets were harvested. Every location had experienced chronic infestations of Erechthias since 2004, and various levels of A. yasumatsui and Chilades pandava infestations since 2005. The soils of all 10 locations were formed in slope alluvium, loess, and residuum overlying coralline limestone (Clayey-skeletal, gibbsitic, nonacid, isohyperthermic Lithic Ustorthents).Citation43 The most prevalent sympatric tree species were Aglaia mariannensis, Eugenia spp., Ficus spp., Morinda citrifolia, Ochrosia mariannensis, Pandanus tectorius, and Triphasia trifolia.
The center and perimeter of circular plots with 20-m radius were marked. Canopy openness above each study plot was assessed by means of fish-eye digital photograph (Nikon Coolpix 995 with a Nikon FC-E8 Fisheye Converter) analyzed with WinsCANOPY software (Regent Instruments Inc., Quebec City). Percent open canopy was 20.2 ± 1.7. A 0-15 cm deep soil sample was collected from the center of each plot, and pH was 7.3 ± 0.1 and organic matter was 2.9 ± 0.1 mg g−1. The elemental content included carbon of 13.5 ± 1.3 mg g−1, nitrogen of 0.9 ± 0.1 mg g−1, phosphorus of 30.4 ± 5.8 mg kg−1, potassium of 50.5 ± 4.4 mg kg−1, magnesium of 176.2 ± 19.1 mg kg−1, and calcium of 2987 ± 331 mg kg−1.
All of the leaflets for each insect damage category were harvested within each circular plot. Our goal was to predict the legacy effect of insect-damaged leaf litter, so all leaflets were harvested from senescent leaves. Because plant ontogeny can influence plant chemistry, trees sourced for the leaflets were restricted to 1.5-2.5 m in height to limit age differences. In order to approximate a homogeneous level of herbivory, leaflets with 50% infestation or damage were selected for all 3 herbivores. A common phenotype of A. yasumatsui infestations is for abaxial leaflet surfaces to exhibit heavy infestations and adaxial surfaces to exhibit minimal infestations. The leaflets that were collected to represent A. yasumatsui damage conformed to this form of infestation. Leaflets representing the C. pandava and Erechthias sp damage were restricted to leaflets that exhibited ca. 50% herbivory. For all 3 insects, the leaflets were harvested from leaves that exhibited ca. 50% herbivory of the entire compound leaf.
Leaflets were stored in ambient conditions until they were prepared for analysis. Leaflets were dried at 70°C, then milled to pass through a 1-mm screen. Total polyphenols were determined by the Folin-Ciocalteu method and expressed as gallic acid equivalents.Citation44 Lignin was quantified with the acetyl-bromide method.Citation45 Cellulose was determined according to AOAC International.Citation46 Carbon and nitrogen were determined by dry combustion (FLASH EA1112 CHN analyzer; Thermo Fisher, Waltham, MA). Other macro and micronutrients were determined by inductively coupled plasma optical emission spectrometry (Spectro Genesis; SPECTRO Analytical Instruments, Kleve, Germany).Citation47
Data for control leaflets and the damaged leaflets for the 3 insect herbivores were subjected to one-way ANOVA. Means separation was by least significant difference for variables that exhibited significant differences.
Disclosure of potential conflicts of interest
No potential conflicts of interest were disclosed.
Acknowledgments
We thank Gil Cruz for help with field work and Paris Marler for help with data management.
Funding
Support for field work provided by US. Forest Service Project No. 10-DG-11059702-095. Support for analytical work provided by the USDA National Institute of Food and Agriculture, McIntire Stennis project 1005274 and the United States Department of the Navy through N40192-12-2-8000 and N40192-13-2-8003, administered by Naval Facilities Engineering Command Marianas, Guam.
References
- Donnegan JA, Butler SL, Grabowiecki W, Hiserote BA, Limtiaco D. Guam's forest resources, 2002. Resource Bulletin PNW-RB-243. Portland: U.S. Department of Agriculture, Forest Service, Pacific Northwest Research Station; 2004.
- Marler TE, Muniappan R. Pests of Cycas micronesica leaf, stem, and male reproductive tissues with notes on current threat status. Micronesica 2006; 39:1-9.
- Marler TE. Cycad aulacaspis scale invades the Mariana Islands. Memoirs New York Bot Gard 2012; 106:20-35.
- Moore A, Marler TE, Miller R, Muniappan R. Biological control of cycad aulacaspis scale on Guam. The Cycad Newsletter 2005; 28:6-8.
- Marler TE. Temporal variations in leaf miner, butterfly, and stem borer infestations of Cycas micronesica in relation to Aulacaspis yasumatsui incidence. HortScience 2013; 48:1334-8.
- Marler TE, Lawrence JH. Demography of Cycas micronesica on Guam following introduction of the armoured scale Aulacaspis yasumatsui. J Trop Ecol 2012; 28:233-42; http://dx.doi.org/10.1017/S0266467412000119
- Chapman SK, Hart SC, Cobb NS, Whitham TG, Koch GW. Insect herbivory increases litter quality and decomposition: an extension of the acceleration hypothesis. Ecology 2003; 84:2867-76; http://dx.doi.org/10.1890/02-0046
- Fang X-M, Christenson LM, Wang F-C, Zeng J-P, Chen F-S. Pine caterpillar outbreak and stand density impacts on nitrogen and phosphorus dynamics and their stoichiometry in Masson pine ( Pinus massoniana) plantations in subtropical China. Can J For Res 2016; 46:601-9; http://dx.doi.org/10.1139/cjfr-2015-0357
- Schweitzer JA, Bailey JK, Hart SC, Wimp GM, Chapman SK, Whitham TG. The interaction of plant genotype and herbivory decelerate leaf litter decomposition and alter nutrient dynamics. Oikos 2005; 110:133-45; http://dx.doi.org/10.1111/j.0030-1299.2005.13650.x
- Anonymous. Conserving our nation's only native cycad species. Currents Fall 2014; 28-31. Available at: http://greenfleet.dodlive.mil/files/2014/10/Fall14_Conserving_Cycad_Species.pdf
- García-Palacios P, McKie BG, Handa IT, Frainer A, Hättenschwiler S. The importance of litter traits and decomposers for litter decomposition: a comparison of aquatic and terrestrial ecosystems within and across biomes. Funct Ecol 2016; 30:819-29; http://dx.doi.org/10.1111/1365-2435.12589
- Marler TE, Dongol N. Do phytotoxic compounds in soils after scale-infested Cycas micronesica litter deposits explain reduced plant growth? HortScience 2013; 48:1571-3.
- Watson GW, Marler TE. Does cycad aulacaspis scale ( Aulacaspis yasumatsui, Hemiptera: Diaspididae) play a direct role in causing soil phytotoxicity? Commun Integr Biol 2014; 7:e278811-3; PMID:25083170; http://dx.doi.org/10.4161/cib.27881
- Herms D, Mattson WJ. The dilemma of plants: to grow or defend. Quarterly Rev Biol 1992; 67:283-335; http://dx.doi.org/10.1086/417659
- May JD, Killingbeck KT. Effects of herbivore-induced nutrient stress on correlates of fitness and on nutrient resorption in scrub oak ( Quercus ilicifolia). Can J Forest Res 1995; 25:1858-64; http://dx.doi.org/10.1139/x95-200
- Prado A. The cycad herbivores. Antennae 2011; 18:3-6.
- Schneider D, Wink M, Sporer F, Lounibos P. Cycads: their evolution, toxins, herbivores and insect pollinators. Naturwissenschaften 2002; 89:281-94; http://dx.doi.org/10.1007/s00114-002-0330-2
- Carrillo Y, Ball BA, Molina M. Stoichiometric linkages between plant litter, trophic interactions and nitrogen mineralization across the litter-soil interface. Soil Biol Bioch 2016; 92:102-10; http://dx.doi.org/10.1016/j.soilbio.2015.10.001
- Cherif M, Loreau M. Plant – herbivore – decomposer stoichiometric mismatches and nutrient cycling in ecosystems. Proc R Soc B 2013; 280:20122453PMID:23303537; http://dx.doi.org/10.1098/rspb.2012.2453
- Martinson HM, Schneider K, Gilbert J, Hines JE, Hambäck PA, Fagan WF. Detritivory: stoichiometry of a neglected trophic level. Ecol Res 2008; 23:487-91; http://dx.doi.org/10.1007/s11284-008-0471-7
- Persson J, Fink P, Goto A, Hood JM, Jonas J, Kato S. To be or not to be what you eat: regulation of stoichiometric homeostasis among autotrophs and heterotrophs. Oikos 2010; 119:741-51; http://dx.doi.org/10.1111/j.1600-0706.2009.18545.x
- Leitner M, Boland W, Mithöfer A. Direct and indirect defences induced by piercing-sucking and chewing herbivores in Medicago truncatula. New Phytol 2005; 167:597-606; PMID:15998409; http://dx.doi.org/10.1111/j.1469-8137.2005.01426.x
- Marler TE, Dongol N. Models to describe Cycas micronesica leaf and strobili development. HortScience 2011; 46:1333-7.
- Norstog KJ, Nicholls TJ. The biology of the cycads. New York: Cornell University Press; 1997.
- Hunter MD. Insect population dynamics meets ecosystem ecology: effects of herbivory on soil nutrient dynamics. Agric For Entom 2001; 3:77-84; http://dx.doi.org/10.1046/j.1461-9563.2001.00100.x
- Lovett GM, Christenson LM, Groffman PM, Jones CG, Hart JE, Mitchell MJ. 2002. Insect defoliation and nitrogen cycling in forests. BioScience 2002; 52:335-41; http://dx.doi.org/10.1641/0006-3568(2002)052%5b0335:IDANCI%5d2.0.CO;2
- Marler TE, Lawrence JH. Canopy and knowledge gaps when invasive alien insects remove foundation species. Commun Integr Biol 2013; 6(1):e22331; PMID:23847712
- Marler T, Haynes J, Lindström A. Cycas micronesica. The IUCN Red List of Threatened Species 2010: e.T61316A12462113. Available at: http://dx.doi.org/10.2305/IUCN.UK.2010-3.RLTS.T61316A12462113.en
- USFWS [U.S. Fish and Wildlife Service]. Endangered and threatened wildlife and plants; endangered status for 16 species and threatened status for 7 species in Micronesia. Federal Register 2015; 80(190):59424-97.
- Marler TE. Cycad mutualist offers more than pollen transport. Amer J Bot 2010; 97:841-5; PMID:21622449; http://dx.doi.org/10.3732/ajb.0900251
- Gandhi KJK, Herms DA. Direct and indirect effect of alien insect herbivores on ecological processes and interactions in forests of eastern North America. Biol Invasions 2010; 12:389-405; http://dx.doi.org/10.1007/s10530-009-9627-9
- Kenis M, Auger-Rozenberg MA, Roques A, Timms L, Péré C, Cock MJW, Settele J, Augustin S, Lopez-Vaamonde C. Ecological effects of invasive alien insects. Biol Invasions 2009; 11:21-45; http://dx.doi.org/10.1007/s10530-008-9318-y
- Kizlinski ML, Orwig DA, Cobb RC, Foster DR. Direct and indirect ecosystem consequences of an invasive pest on forests dominated by eastern hemlock. J. Biogeography 2002; 29:1489-503; http://dx.doi.org/10.1046/j.1365-2699.2002.00766.x
- le Mellec A, Michalzik B. Impact of pine lappet ( Dendrolimus pini) mass outbreak on C and N fluxes to the forest floor and soil microbial properties in a Scots pine forest in Germany. Can J For Res 2008; 38:1829-41; http://dx.doi.org/10.1139/X08-045
- Lovett GM, Canham CD, Arthur MA, Weathers KC, Fitzhugh RD. Forest ecosystem responses to exotic pests and pathogens in Eastern North America. BioScience 2006; 56:395-405; http://dx.doi.org/10.1641/0006-3568(2006)056%5b0395:FERTEP%5d2.0.CO;2
- Orwig DA, Cobb RC, D'Amato AW, Kizlinski ML, Foster DR. 2008. Multi-year ecosystem response to hemlock woolly adelgid infestation in southern New England forests. Can J For Res 2008; 38:834-43; http://dx.doi.org/10.1139/X07-196
- Marler TE, Lindström AJ, Terry LI. Chilades pandava damage among 85 Cycas species in a common garden setting. HortScience 2012; 47:1832-6.
- Marler TE, Lindström A, Terry LI. Information-based or resource-based systems may mediate Cycas herbivore interactions. Plant Signal Behav 2012; 7:760-2; PMID:22751309; http://dx.doi.org/10.4161/psb.20470
- Salt DE, Baxter I, Lahner B. Ionomics and the study of the plant ionome. Annu Rev Plant Biol 2008; 59:709-33; PMID:18251712; http://dx.doi.org/10.1146/annurev.arplant.59.032607.092942
- Gómez S, Orians CM, Preisser EL. Exotic herbivores on a shared native host: tissue quality after individual, simultaneous, and sequential attack. Oecologia 2012; 169:1015-24; PMID:22311255; http://dx.doi.org/10.1007/s00442-012-2267-2
- Marquis RJ. Genotypic variation in leaf damage in Piper arieianum (Piperaceae) by a multispecies assemblage of herbivores. Evolution 1990; 44:104-20; http://dx.doi.org/10.2307/2409527
- Rodriguez-Saona CR, Musser RO, Vogel H, Hum-Musser SM, Thaler JS. Molecular, biochemical and organismal analyses of tomato plants simultaneously attacked by herbivores from two feeding guilds. J Chem Ecol 2010; 36:1043-57; PMID:20820890 ; http://dx.doi.org/10.1007/s10886-010-9854-7
- Young FJ. Soil Survey of Territory of Guam. United States Department of Agriculture Soil Conservation Service. 1988.
- Waterman PG, Mole S. Extraction and chemical quantification. In Waterman PG, Mole S, eds. Analysis of phenolic plant metabolites. Oxford, UK: Blackwell Science; 1994:66-103.
- Iiyama K, Wallis AFA. 1990. Determination of lignin in herbaceous plants by an improved acetyl bromide procedure. J Sci Food Agric 1990; 51:145-61; http://dx.doi.org/10.1002/jsfa.2740510202
- AOAC. Official Method 973. 18 fibre (acid detergent) and lignin in animal feed. In: Official methods of analysis of AOAC International, 16th ed. AOAC International, Arlington, VA. 1997:28-9.
- Hou X, Jones BT. Inductively Coupled Plasma/Optical Emission Spectrometry. In Meyers RA, ed. Encyclopedia of Analytical Chemistry. Chichester: John Wiley & Sons; 2000:9468-85.