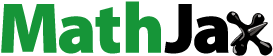
ABSTRACT
Group living animals can use the behavior of others as cues for the presence of threat in the environment and adjust their behavior accordingly. Therefore, different social phenomena that modulate the response to threat, such as social buffering, social transmission (contagion), and facilitation of alarm responses can be seen as different manifestations of social information use in threat detection. Thus, social phenomena that are functionally antagonistic, such as social buffering and social transmission of fear, may rely on shared neurobehavioral mechanisms related to the use of social information in decision-making about the presence of threat in the environment. Here, we propose a unifying conceptual framework for the study of social information use in threat perception based on signal detection theory.
Social support is a major factor in stress coping and resilience in humans. The mere availability of conspecifics has been shown to reduce the behavioral and physiological responses to aversive events (e.g. ref. Citation1). This phenomenon, known as social buffering, has also been documented in several animal species. In a seminal study, Davitz and MasonCitation2 showed that rats (Rattus norvegicus) exhibited lower fear behavior responses when exposed to an aversive stimulus if in the presence of conspecifics. Social buffering of the physiological stress response has also been demonstrated. In a mother-infant separation paradigm in squirrel monkeys (Saimiri sciureus), the cortisol increase in infants separated from their mothers was lower when they were placed in a familiar social environment containing colony members.Citation3 Similarly, mothers separated from their infants did not increase their cortisol levels if placed in their social groups after infant separation.Citation4 Many experiments have corroborated these findings and added knowledge regarding the behavioral and physiological effects of social buffering across different species, including non-human primates,Citation5 rodents,Citation6 birds,Citation7 fishCitation8 and invertebrates,Citation9 suggesting that social buffering is an evolutionary conserved process among social animals (for a review see ref. Citation10).
Despite the growing evidence on the ubiquity of social buffering, relatively little is known about the mechanisms through which social cues modulate the behavioral and physiological responses to aversive events. To date, different sensory modalities conveying relevant information for social buffering have been identified in different species [e.g., vocal cues in marmosets (Callithrix jacchus);Citation11 olfactory cues in rats;Citation12 visual cues in sheep (Ovis aries)Citation13], and even within the same species different cues may be used to communicate social information to conspecifics (e.g., tactile and olfactory cues in ratsCitation14). In rodents, where most studies on the neurobiological mechanisms of social buffering have been conducted, it has been shown that information from multiple social cues is integrated and converges to the amygdala where it increases the release of oxytocin, which in turn increases dopamine transmission in the nucleus accumbens, hence promoting social bonding.Citation15,16 In parallel, an increase in oxytocin in the hypothalamus is responsible for the dampening of the neuroendocrine responses.Citation15,17 Understanding the contribution of different sensory modalities to a given buffering event and how they are integrated in the brain to affect behavioral and physiological responses is of particular importance to assess if the ubiquity of social buffering across taxa reflects homology of its underlying neural mechanisms, or if it rather reflects convergent evolution. Thus, detailed neuroethological studies in non-mammalian species are urgently needed.
In our study we investigated the mechanisms of social buffering of fear in zebrafish (Danio rerio).Citation8 First, we assessed the influence of different sensory modalities to the same buffering episode and respective effectiveness in a long-lasting exposure to threat. After showing that zebrafish decreased their fear response to alarm substance (AS, a known fear-inducing stimulus in this species) when in the joint presence of olfactory (shoal water) and visual (sight of shoal) cues of conspecifics, we exposed zebrafish to AS in the presence of olfactory or visual social cues separately. Our results showed that although both sensory modalities were equally effective in the short term, the visual cue was more effective in decreasing fear responses toward AS in a long-lasting exposure.Citation8 These differences may reflect the fact that olfactory and visual cues are refreshed at different rates in the environment, with olfactory information remaining invariable in the water for longer periods of time, hence limiting the rate at which it can be updated.Citation8 Secondly, we characterized the changes in brain activity associated with social buffering in zebrafish, using the transient expression of an immediate early gene (c-fos) as a marker of neuronal activity. We have shown that a set of brain nuclei that are putative homologues to those involved in social buffering in mammals were also active during social buffering in zebrafish, namely the medial part of the dorsal telencephalon (Dm, homolog of the mammalian pallial amygdala), the ventral nucleus of the ventral telencephalon (Vv, homolog of the mammalian nucleus accumbens and septum), and the preoptic area (POA, homolog of the mammalian preoptic area/paraventricular nucleus).Citation8 In sum, our work indicates that multimodal social cues mediate social buffering in zebrafish and that these are integrated and processed in homologous brains areas to those previously identified in mammals to ameliorate the response to threat.
Social information use in threat detection
Interestingly, the presence of a conspecific may act either as a threat ameliorating stimulus or as a stressor itself, depending on several factors, namely the familiarity with the conspecific (e.g., ref. Citation18), the social context (e.g., dyadic encounter in rats in a novel environment or in a territory),Citation19,20 and the state/ behavior of the conspecific (e.g., stress status in rats).Citation21 Moreover, social transmission of fear/anxiety between a fearful/anxious individual and previously fearless/non-anxious conspecifics has also been documented both in humans and other animals.Citation22-24 Therefore, social buffering and social transmission of fear/anxiety can be viewed as complementary social phenomena. Indeed, if viewed from an information processing perspective, these 2 phenomena can be viewed as different aspects of social information use in the context of threat perception. In group living species individuals can use social information to assess potential threats in the environment, promoting better adaptation to dynamic contexts. In this perspective, social buffering of fear can be seen as animals using social information indicating the absence of a threat to diminish their fear response to a threat that they have detected themselves.Citation7,17,25 On the other hand, in the case of social transmission of fear, animals can use social signals (e.g., alarm calls in mammals and birds; see ref. Citation26) or fear cues (e.g., cessation of movement in rats; see ref.Citation 24) to infer the presence of a threat in the environment, even when they have not detected it directly. Hence, the use of social information predicts the evolution of both social buffering and social transmission of fear in social animals.
Here we propose a unifying conceptual framework for social phenomena that modulate threat perception based on the use of social information by animals when making a decision to activate (or to end, in the case of recovery studies) a behavioral/physiological response to the presence of threat in the environment. This framework is based on the application of signal detection theory to animal communication,Citation27,28 and has the following premises:
(1) | Although the detection of threat in the environment can elicit different types of alarm responses, such as fear, anxiety and stress, there is a decision-making process in which the individual decides whether a threat is present, and therefore to trigger the response. | ||||||||||||||||||||||
(2) | Individuals have to sample information available in the environment that signals the presence of threat. Given that sensory data always have some degree of ambiguity (e.g., due to ambient noise), threat cues are not 100% unambiguous (e.g., an individual may have to decide if a rustle in the grass indicates the presence of a predator or if it is just the wind), and typically there is some overlap between the distribution of ambient noise and the distribution of the threat cue (i.e. on average predators make more rustling than the wind, but there are occasions where they make less; ). Hence, individuals need to establish a criterion (aka signal detection threshold) for that threat presence. If individuals set a high threshold for triggering a threat response, they will fail to detect a real threat frequently (misses), but they will activate few false alarms; conversely, if they set a low threshold, they will miss fewer real threats, but at the cost of more frequent false alarms (). Therefore, there is a trade-off between misses and false alarms. Figure 1. Signal detection theory applied to threat detection in animals. (A) The distributions of the threat cue and of background noise within the decision-maker overlap, and the difference in position between the 2 distributions reflects the sensitivity (d’) of the decision-maker to the threat cue; the decision-maker uses a criterion (i.e., threat detection threshold, β), to decide when a threat is present (i.e., when the internal signal is stronger than β); given this decision there are 4 possible outcomes: (i) the individual reports the threat present when it is (hit); (ii) the individual reports the threat present when it is absent (false alarm); (iii) the individual reports the threat absent when it is present (miss); and (iv) the individual reports the signal absent when it is absent (correct rejection); the probabilities for each of these outcomes are represented by the area under the curves. (B) Illustration of a hypothetical individual with higher threat sensitivity (d’; i.e., the difference between the proportion of hits and false alarms is higher); note that threat sensitivity (d’) and threat detection threshold (β) are independent measures of decision-making; in this example the sensitivity increased in comparison to (A), whereas the threshold remained the same. (C) Illustration of a hypothetical individual with a high threat threshold (β); it will reduce the number of false alarms at the cost of increasing the number of misses. (D) Illustration of a hypothetical individual with a low threat threshold; it will reduce the number of misses at the cost of increasing the number of false alarms. ![]() | ||||||||||||||||||||||
(3) | According to error management theoryCitation29 individuals should adjust the signal detection threshold according to: (1) the relative costs of misses (Cm) vs. false alarms (Cfa); and (2) the probability of threat (Pt) being present in the environment (e.g., how frequent is the presence of predator) or not (Pnt), with the optimal threshold (OT) value given by the following equation: | ||||||||||||||||||||||
(4) | In group living species individuals can gather information from the behavior of others.Citation30 Thus, threat cues can be detected either directly or from observing the behavior of others (i.e., Pt has a direct and an indirect/social component). It has been recently demonstrated that the trade-off between misses and false alarms (i.e., increase in hits can only be achieved with a concomitant increase in false alarms) described above for individual decision-making, can be overcome in a group of decision makers using a quorum decision rule, when the threshold is set above the false alarm rate and below the true-positive rate of solitary decision-makers.Citation31 Therefore, the use of social information in threat perception allows this basic trade-off in individual signal detection theory to be avoided. | ||||||||||||||||||||||
(5) | If one considers the relative costs of misses vs. false alarms to be fixed, then the optimal threshold will depend exclusively on the probability of a threat being present. In this situation, one can consider 3 scenarios:
|
Therefore, social facilitation, social buffering and social contagion of fear/anxiety should be seen as complementary phenomena and resulting from the use of social information in threat detection. Since these 3 phenomena are potentially using the same sensory and cognitive mechanisms to collect and process social information for social-decision making, it is expected that they share neural mechanisms related to social information use. As a consequence, the perspective proposed here predicts that functionally antagonistic social phenomena (i.e., induce fear vs. reduce fear) may in fact share the same neurobehavioral mechanisms. Finally, it should also be highlighted that the application of signal detection theory to threat perception provides measures of sensitivity to the threat cue (d’) and of subject bias (β), independent of each other, that can be compared across widely different situations.
Table 1. Social modulation of threat responses (stress, fear, anxiety). Individuals can combine direct and indirect/social information to assess the presence of threat in the environment and respond accordingly, resulting in different social phenomena.
Disclosure of potential conflicts of interest
No potential conflicts of interest were disclosed.
Acknowledgments
The authors are thankful to Peter McGregor for revising a previous version of the manuscript, which contributed to improve the final paper.
Funding
The preparation of this manuscript was supported by a FCT research grant (PTDC/BIA-ANM/0810/2014) given to R.F.O.
References
- Heinrichs M, Baumgartner T, Kirschbaum C, Ehlert U. Social support and oxytocin interact to suppress cortisol and subjective responses to psychosocial stress. Biol Psychiatry 2003; 54:1389-98; PMID:14675803; https://doi.org/10.1016/S0006-3223(03)00465-7
- Davitz JR, Mason DJ. Socially Facilitated Reduction of a Fear Response in Rats. J Comp Physiol Psychol 1955; 48:149-51; PMID:13242680; https://doi.org/10.1037/h004293610.1037/h0046411
- Coe CL, Mendoza SP, Smotherman WP, Levine S. Mother-infant attachment in the squirrel monkey: Adrenal response to separation. Behav Biol 1978; 22:256-63; PMID:415729; https://doi.org/10.1016/S0091-6773(78)92305-2
- Mendoza SP, Coe CL, Lowe EL, Levine S. The physiological response to group formation in adult male squirrel monkeys. Psychoneuroendocrinology 1978; 3:221-9; PMID:107541; https://doi.org/10.1016/0306-4530(78)90012-4
- Wittig RM, Crockford C, Weltring A, Langergraber KE, Deschner T, Zuberbühler K. Social support reduces stress hormone levels in wild chimpanzees across stressful events and everyday affiliations. Nat Commun 2016; 7:13361; PMID:27802260; https://doi.org/10.1038/ncomms13361
- Fuzzo F, Matsumoto J, Kiyokawa Y, Takeuchi Y, Ono T, Nishijo H. Social buffering suppresses fear-associated activation of the lateral amygdala in male rats: behavioral and neurophysiological evidence. Front Neurosci 2015; 9:1-8; PMID:25653585; https://doi.org/10.3389/fnins.2015.00099
- Edgar J, Held S, Paul E, Pettersson I, I'Anson Price R, Nicol C. Social buffering in a bird. Anim Behav 2015; 105:11-9; https://doi.org/10.1016/j.anbehav.2015.04.007
- Faustino AI, Tacão-Monteiro A, Oliveira RF. Mechanisms of social buffering of fear in zebrafish. Sci Rep 2017; 7:44329; PMID:28361887; https://doi.org/10.1038/srep44329
- Tian L, Preisser EL, Haynes KF, Zhou X. Social buffering in a eusocial invertebrate: termite soldiers reduce the lethal impact of competitor cues on workers. Ecology 2017; 98:952-60; PMID:28122113; https://doi.org/10.1002/ecy.1746
- Kikusui T, Winslow JT, Mori Y. Social buffering: relief from stress and anxiety. Philos Trans R Soc Lond B Biol Sci 2006; 361:2215-28; PMID:17118934; https://doi.org/10.1098/rstb.2006.1941
- Rukstalis M, French JA. Vocal buffering of the stress response: Exposure to conspecific vocalizations moderates urinary cortisol excretion in isolated marmosets. Horm Behav 2005; 47:1-7; PMID:15579259; https://doi.org/10.1016/j.yhbeh.2004.09.004
- Takahashi Y, Kiyokawa Y, Kodama Y, Arata S, Takeuchi Y, Mori Y. Olfactory signals mediate social buffering of conditioned fear responses in male rats. Behav Brain Res 2013; 240:46-51; PMID:23183219; https://doi.org/10.1016/j.bbr.2012.11.017
- da Costa AP, Leigh AE, Man M-S, Kendrick KM. Face pictures reduce behavioural, autonomic, endocrine and neural indices of stress and fear in sheep. Proc R Soc B Biol Sci 2004; 271:2077-84; https://doi.org/10.1098/rspb.2004.2831
- Latané B. Gregariousness and fear in laboratory rats. J Exp Soc Psychol 1969; 5:61-9; https://doi.org/10.1016/0022-1031(69)90046-810.1016/0022-1031(69)90006-7
- Lieberwirth C, Wang Z. The neurobiology of pair bond formation, bond disruption, and social buffering. Curr Opin Neurobiol 2016; 40:8-13; PMID:27290660; https://doi.org/10.1016/j.conb.2016.05.00610.1016/j.ceb.2016.01.009
- Young LJ, Wang Z. The neurobiology of pair bonding. Nat Neurosci 2004; 7:1048-54; PMID:15452576; https://doi.org/10.1038/nn1327
- Smith AS, Wang Z. Hypothalamic oxytocin mediates social buffering of the stress response. Biol Psychiatry 2014; 76:281-8; PMID:24183103; https://doi.org/10.1016/j.biopsych.2013.09.017
- Graves FC, Hennessy MB. Comparison of the effects of the mother and an unfamiliar adult female on cortisol and behavioral responses of pre-and postweaning guinea pigs. Dev Psychobiol 2000; 36:91-100; PMID:10689280; https://doi.org/10.1002/(SICI)1098-2302(200003)36:2<91::AID-DEV1>3.0.CO;2-1
- Miczek KA. Intraspecies aggression in rats: Effects of d-amphetamine and chlordiazepoxide. Psychopharmacologia 1974; 39:275-301; PMID:4476082; https://doi.org/10.1007/BF00422968
- Miczek KA. A new test for aggression in rats without aversive stimulation: differential effects of d-amphetamine and cocaine. Psychopharmacology 1979; 60:253-9; PMID:108702; https://doi.org/10.1007/BF0043228410.1007/BF00426664
- Kiyokawa Y, Kikusui T, Takeuchi Y, Mori Y. Partner's stress status influences social buffering effects in rats. Behav Neurosci 2004; 118:798-804; PMID:15301605; https://doi.org/10.1037/0735-7044.118.4.798
- Olsson A, Phelps EA. Learned Fear of “‘ Unseen ’” Faces After Pavlovian, Observational, and Instructed Fear. Psychol Sci 2004; 15:822-8; PMID:15563327; https://doi.org/10.1111/j.0956-7976.2004.00762.x
- Mineka S, Davidson M, Cook M, Keir R. Observational conditioning of snake fear in rhesus monkeys. J Abnorm Psychol 1984; 93:355-72; PMID:6542574; https://doi.org/10.1037/0021-843X.93.4.355
- Pereira AG, Cruz A, Lima SQ, Moita MA. Silence resulting from the cessation of movement signals danger. Curr Biol 2012; 22:R627-8; PMID:22917506; https://doi.org/10.1016/j.sbi.2012.07.00610.1016/j.cub.2012.06.01510.1016/j.cub.2012.02.022
- Kiyokawa Y, Honda A, Takeuchi Y, Mori Y. A familiar conspecific is more effective than an unfamiliar conspecific for social buffering of conditioned fear responses in male rats. Behav Brain Res 2014; 267:189-93; PMID:24698797; https://doi.org/10.1016/j.bbr.2014.03.043
- Hollén LI, Radford AN. The development of alarm call behaviour in mammals and birds. Anim Behav 2009; 78:791-800; https://doi.org/10.1016/j.anbehav.2009.07.021
- Wiley RH. Signal detection and animal communication. Adv Study Behav 2006; 36:217-47.
- Wiley RH. Signal Detection, Noise, and the Evolution of Communication. In: Brumm H, editor. Animal Communication and Noise. Berlin, Heidelberg( Germany): Springer-Verlag; 2013. p. 7-30.
- Haselton M, Nettle D. The paranoind optimist: an integrative evolutionary model of cognitive biases. Personal Soc Psychol Rev 2006; 10:47-66; https://doi.org/10.1207/s15327957pspr1001_3
- Hoppitt W, Laland KN. Social learning: an introduction to mechanisms, methods and models. Princeton( NJ): Princeton University Press; 2013.
- Wolf M, Kurvers RHJM, Ward AJW, Krause S, Krause J. Accurate decisions in an uncertain world: collective cognition increases true positives while decreasing false positives. Proc Biol Sci 2013; 280:20122777; PMID:23407830; https://doi.org/10.1098/rspb.2013.239110.1098/rspb.2012.2777