ABSTRACT
Obesity is an enormous worldwide health concern. Chronic illnesses associated with obesity include type-2 diabetes, hypertension, atherosclerosis and certain cancers. Communication between fat storage organs and the brain is essential for regulating feeding, metabolism and organismal activity—and hence obesity control. Model organism research provides opportunities to decipher conserved molecular mechanisms that regulate fat storage and activity levels, which is fundamental to understanding this disorder. We recently identified a transcription factor (ETS-5) that acts in specific neurons of the nematode Caenorhabditis elegans to control intestinal fat levels. Furthermore, we discovered a feedback mechanism where intestinal fat controls feeding and motor programs, similar to humans, where a sated stomach can inhibit feeding and induce lethargy. The precise molecular signals and neuronal circuitry underpinning brain-intestinal communication in C. elegans are however yet to be discovered. As most animals store surplus energy as fat, communication mechanisms that relay external information regarding food availability and quality, and internal energy reserves are likely conserved. Therefore, our identification of a neuronally-expressed transcriptional regulator that controls intestinal fat levels opens up new avenues of investigation for the control of metabolic disease and obesity.
Maintaining metabolic homeostasis requires a balance between eating, storing and using energy. The nervous system integrates information about internal nutrient stores, external food quality and availability, and previous food experience to determine the correct behavioral and physiological response to the current environment. The brain not only modulates food intake to maintain metabolic homeostasis, it can communicate with the intestine directly to induce changes in metabolic-gene expression. An important component of brain-intestine communication is transcriptional regulation. For example, changes to the external environment or internal metabolic state can alter gene expression of neuronal signaling factors and nuclear hormone receptors in the intestine.Citation1-3 These transcriptional changes are key to the communication networks between neurons, the intestine and activity programs, since they ensure the correct signaling molecules and receptors are present at the right time, and in the right place. Inducing changes in gene expression is a far slower process than acute calcium-based signaling. As such, transcriptional regulation potentiates longer-lasting responses to environmental cues that complement fast-acting synaptic responses.Citation1 We recently identified a member of the E twenty-six transcription factor family in C. elegans, named ETS-5, which acts in a specific set of neurons to modulate fat storage in the intestine.Citation4 Here we further expand and explain our findings, and discuss their significance in relation to the most recent work in this field.
ETS-5 is a transcription factor expressed in multiple sensory neurons in C. elegans.Citation5 We previously showed that ETS-5 is important for specifying CO2 sensing fate of the BAG neurons, and have recently discovered that ETS-5 acts in the ASG and BAG neurons to regulate behavioral state switching in the presence of food.Citation4,5 In the laboratory, C. elegans is fed a uniform Escherichia coli food source, on which they spontaneously transition between 3 behavioral states - roaming (active), dwelling (inactive) and quiescence (sleep-like state).Citation6-8 Switching between these behavioral states occurs in the absence of external stimuli, suggesting that internally-derived, as well as external food cues, can generate these behaviors. Roaming animals move rapidly and turn infrequently to explore their habitat. Dwelling animals are characterized by slow movement and frequent turning to enable exploitation of the local environment. In contrast, quiescent animals are immotile and do not feed. Quiescence is induced by satiety and closely resembles postprandial somnolence, or “food coma” in mammals.Citation9 Quiescent behavior in the laboratory is rarely observed (5% of the time) in wild type strains because worms are typically cultured on low quality food (the OP50 E. coli strain), thus laboratory-raised nematodes rarely reach the level of satiety that triggers a quiescent state.Citation6 C. elegans strains that are unable to synthesize or store fat are defective in quiescent behaviors,Citation3 arguing that fat levels in the intestine are an important determinant in behavioral state switching.
Our recent study found that ets-5 mutant animals exhibit increased quiescence behavior and store more intestinal fat than wild type animals.Citation4 We hypothesized that ets-5 mutants likely exhibit increased quiescence because they are fully sated. Consistent with this, when we decreased fat levels in the intestine of the ets-5 mutant, by malnourishing worms or by reducing the expression of POD-2, an acetyl carboxylase, which is crucial for fatty acid synthesis in the intestine, animals explored more.Citation4 Thus, decreasing intestinal energy stores overrides the ets-5 mutant quiescence phenotype. Interestingly, we found that ets-5 mutant animals revert to wild type exploratory behavior very rapidly when malnourished. This suggests that mechanical stimuli in the pharynx indicating low food intake may predominate over internal satiety cues. Taken together, the ets-5 mutation leads to increased intestinal fat deposition, such that animals are sated and enter a quiescent or sleep-like state.
The question remained how ETS-5 controls intestinal fat stores from the ASG/BAG neurons? One possibility is that the ets-5 mutant consumes food more quickly than wild type animals, thus gaining more energy in a shorter space of time, which could lead to quiescence. However, the ets-5 mutant does not display increased pharyngeal pumping—a key indicator of increased food consumption, making it unlikely that the phenotype is due to increased food intake. ETS-5 is required to specify the BAG neurons,Citation5,10 and these neurons enable the nematode to sense O2 and CO2. Therefore, it is possible that the gas-sensing function of these neurons may be responsible for the increased intestinal fat storage. However, animals in which the GCY-9 CO2 sensing and GCY-31/GCY-33 O2 sensing moleculesCitation5,Citation11 are removed, and are deficient in O2 and CO2 sensing, explore like wild type animals. Therefore, an inability to sense O2 and CO2 does not contribute to the exploration phenotype of ets-5 mutant animals. In addition, the ets-5 mutant proved invariant from wild type animals in multiple other gustatory and odortaxis behavioral assays, and exhibited normal locomotion speed.Citation4 These data support the idea that the ets-5 mutants do not gain fat because of altered food sensing or seeking ability, but because the communication between the nervous system and the intestine is dysregulated.
How does the neuronal function of ETS-5 fit into known pathways that govern the brain-intestine communication axis? One of the most well studied regulators of the brain-intestine axis is serotonin (5HT).Citation12-14 5HT is synthesized and secreted from a discrete number of neurons in C. elegans,Citation15 and promotes the breakdown of body fat by inducing ATGL-1 lipase expression in the intestine.Citation12 5HT signals to the URX and ASI neurons, which results in secretion of the neuropeptide FLP-7 into the pseudocoelomic fluid.Citation16 Intestinal cells bind circulating FLP-7 via the NPR-22 receptor, which results in increased ATGL-1 expression.Citation16 Generally, 5HT is a signal of satiety; promoting dwelling behavior and fat breakdown.Citation7,12 We used a mutant for the 5HT receptor MOD-1, which explores more than wild type animals,Citation7 to study the relationship between 5HT signaling and ETS-5 function. We found that when both 5HT signaling and ETS-5 are absent, these mutants exhibit normal levels of quiescence, yet still have reduced exploration.Citation4 This suggests that ETS-5 acts genetically downstream of 5HT signaling in terms of promoting exploratory behavior, but 5HT is genetically upstream of ETS-5 in terms of promoting quiescence, thus highlighting the exquisite complexity of the neural networks that govern behavioral-state switches. In addition to 5HT, the activity of dopamine-expressing neurons has also been shown to promote dwelling behavior.Citation17 Therefore, monoaminergic seems to be critical for regulating organismal activity.
The pigment-dispersing factor (PDF) neuropeptide-signaling pathway, acts antagonistically to 5HT signaling, to promote exploratory behavior.Citation7 We found that mutants for the PDF-neuropeptide receptor—PDFR-1, do not roam and have a higher incidence of quiescence than the ets-5 mutant. Animals in which PDF signaling and ETS-5 is removed exhibit the pdfr-1 mutant phenotype, therefore ETS-5 acts genetically upstream of PDF signaling. Other signaling mechanisms that control food-dependent behavioral state switching in C. elegans include the cGMP, TGF-β and insulin pathways.Citation6,8,18 How ETS-5 coordinates behavioral state switching through or in conjunction with these signaling pathways will be a major subject for future studies.
The ASG/BAG neurons, whose function in behavioral state switching is specified by ETS-5 activity, play an integral part in the neuronal-intestine axis. As the C. elegans intestine is not innervated, signaling between the nervous system and intestine must ultimately be through the action of neuropeptides. Indeed, we found that neuropeptide secretion from the ASG/BAG neurons is important for them to fulfil this function. We showed that knocking down UNC-31, a protein required for neuropeptide secretion,Citation19 specifically in the ASG/BAG neurons of wild type animals significantly decreased exploratory behavior.Citation4 Which neuropeptides are being regulated by ETS-5 still remains an open question. We showed that deleting 2 known ETS-5-regulated neuropeptides—FLP-13 and FLP-195—caused reduced exploration, but not to the same extent as ets-5 mutant animals. Thus, ETS-5 may act in the ASG and BAG neurons to control neuropeptide release or may directly control the expression of FLP-13 and FLP-19, and likely other neuropeptides, for signaling to other components of the network.
What may those other components be? As mentioned above, neuropeptides secreted from neurons are capable of circulating in the pseudocoelomic fluid and directly interacting with neuropeptide receptors on the surface of intestinal cells. However, these neuropeptidergic pathways are also likely to participate in networks of neuronal interactions to provide enhanced modulatory capacity for survival in ephemeral habitats. The accessibility of the C. elegans wiring diagram will enable a full appreciation of the molecular and cellular requirements for the regulation of food-associated behaviors.
In conclusion, the identification of a transcription factor acting in the nervous system to control fat levels in the intestine provides a platform for the identification and characterization of brain-intestinal communication mechanisms. The C. elegans genetic model provides an excellent opportunity to identify such means of communication. As the molecules and pathways under study are conserved in mammals, future work will potentially be of benefit in our understanding of the causes of obesity.
Figure 1. Neuronal regulation of intestinal fat and motor activity in C. elegans. ETS-5 expression in the ASG and BAG neurons controls intestinal fat levels through an unknown mechanism.4 We hypothesize that intestinal fat levels are interpreted by the nervous system to regulate feeding and activity programs. The question marks denote, as yet, unknown brain-intestinal communication processes.
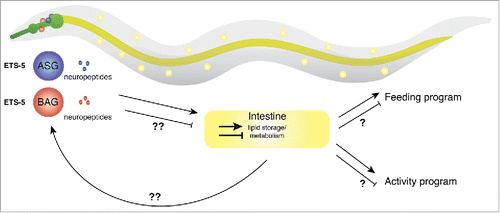
Disclosure of potential conflicts of interest
No potential conflicts of interest were disclosed.
Funding
This work was supported by the European Research Council under Grant number 260807; Victorian Endowment for Science, Knowledge and Innovation Fellowship under Grant number VIF 23.
References
- Greene JS, Dobosiewicz M, Butcher RA, McGrath PT, Bargmann CI. Regulatory changes in two chemoreceptor genes contribute to a Caenorhabditis elegans QTL for foraging behavior. eLife 2016; 5:e21454; https://doi.org/10.7554/eLife.14749 10.7554/eLife.21454
- Greene JS, Brown M, Dobosiewicz M, Ishida IG, Macosko EZ, Zhang X, Butcher RA, Cline DJ, McGrath PT, Bargmann CI. Balancing selection shapes density-dependent foraging behaviour. Nature 2016; 539:254-8; PMID:27799655; https://doi.org/10.1038/nature19848
- Hyun M, Davis K, Lee I, Kim J, Dumur C, You YJ. Fat metabolism regulates satiety behavior in C. elegans. Sci Rep 2016; 6:24841; PMID:27097601; https://doi.org/10.1038/srep24841
- Juozaityte V, Pladevall-Morera D, Podolska A, Norgaard S, Neumann B, Pocock R. The ETS-5 transcription factor regulates activity states in Caenorhabditis elegans by controlling satiety. Proc Natl Acad Sci USA 2017; 114(9):E1651-E1658; PMID:28193866; https://doi.org/10.1073/pnas.1610673114
- Brandt JP, Aziz-Zaman S, Juozaityte V, Martinez-Velazquez LA, Petersen JG, Pocock R, Ringstad N. A single gene target of an ETS-family transcription factor determines neuronal CO2-chemosensitivity. PLoS ONE 2012; 7:e34014; PMID:22479504; https://doi.org/10.1371/journal.pone.0047889 10.1371/journal.pone.0052763 10.1371/journal.pone.0048252 10.1371/journal.pone.0038741 10.1371/journal.pone.0034014 10.1371/journal.pone.0037236
- You YJ, Kim J, Raizen DM, Avery L. Insulin, cGMP, and TGF-beta signals regulate food intake and quiescence in C. elegans: a model for satiety. Cell Metab 2008; 7:249-57; https://doi.org/10.1016/j.cmet.2008.01.005
- Flavell SW, Pokala N, Macosko EZ, Albrecht DR, Larsch J, Bargmann CI. Serotonin and the neuropeptide PDF initiate and extend opposing behavioral states in C. elegans. Cell 2013; 154:1023-35; https://doi.org/10.1016/j.cell.2013.08.001
- Ben Arous J, Laffont S, Chatenay D. Molecular and sensory basis of a food related two-state behavior in C. elegans. PLoS ONE 2009; 4:e7584; PMID:19851507; https://doi.org/10.1371/journal.pone.0007584
- Gallagher T, You YJ. Falling asleep after a big meal: neuronal regulation of satiety. Worm 2014; 3:e27938; PMID:25057453; https://doi.org/10.4161/worm.27938
- Guillermin ML, Castelletto ML, Hallem EA. Differentiation of carbon dioxide-sensing neurons in Caenorhabditis elegans requires the ETS-5 transcription factor. Genetics 2011; 189:1327-39; https://doi.org/10.1534/genetics.111.133835
- Zimmer M, Gray JM, Pokala N, Chang AJ, Karow DS, Marletta MA, Hudson ML, Morton DB, Chronis N, Bargmann CI. Neurons detect increases and decreases in oxygen levels using distinct guanylate cyclases. Neuron 2009; 61:865-79; doi:S0896-6273(09)00158-510.1016/j.neuron.2009.02.013
- Noble T, Stieglitz J, Srinivasan S. An integrated serotonin and octopamine neuronal circuit directs the release of an endocrine signal to control C. elegans body fat. Cell Metabol 2013; 18:672-84; https://doi.org/10.1016/j.cmet.2013.09.007
- Nonogaki K, Abdallah L, Goulding EH, Bonasera SJ, Tecott LH. Hyperactivity and reduced energy cost of physical activity in serotonin 5-HT(2C) receptor mutant mice. Diabetes 2003; 52:315-20; PMID:12540602; https://doi.org/10.2337/diabetes.52.2.315
- Chan EW, He Y, Chui CS, Wong AY, Lau WC, Wong IC. Efficacy and safety of lorcaserin in obese adults: a meta-analysis of 1-year randomized controlled trials (RCTs) and narrative review on short-term RCTs. Obes Rev 2013; 14:383-92; PMID:23331711; https://doi.org/10.1111/obr.12015
- Sze JY, Victor M, Loer C, Shi Y, Ruvkun G. Food and metabolic signalling defects in a Caenorhabditis elegans serotonin-synthesis mutant. Nature 2000; 403:560-4; PMID:10676966; https://doi.org/10.1038/35000609
- Palamiuc L, Noble T, Witham E, Ratanpal H, Vaughan M, Srinivasan S. A tachykinin-like neuroendocrine signalling axis couples central serotonin action and nutrient sensing with peripheral lipid metabolism. Nat Commun 2017; 8:14237; PMID:28128367; https://doi.org/10.1038/ncomms14237
- McCloskey RJ, Fouad AD, Churgin MA, Fang-Yen C. Food Responsiveness regulates episodic behavioral states in Caenorhabditis elegans. J Neurophysiol 2017; jn 00555 02016; PMID:28228583; https://doi.org/10.1152/jn.00555.2016
- Fujiwara M, Sengupta P, McIntire SL. Regulation of body size and behavioral state of C. elegans by sensory perception and the EGL-4 cGMP-dependent protein kinase. Neuron 2002; 36:1091-102; PMID:12495624; https://doi.org/10.1016/S0896-6273(02)01093-0
- Sieburth D, Madison JM, Kaplan JM. PKC-1 regulates secretion of neuropeptides. Nature Neurosci 2007; 10:49-57; PMID:17128266; https://doi.org/10.1038/nn1810