ABSTRACT
During cytokinesis, actomyosin ring constriction drives furrow formation. In animal cells, Rho GTPases drive this process through the positioning and assembly of the actomyosin ring, and through extracellular matrix remodeling within the furrow. In the fission yeast S. pombe, actomyosin ring constriction and septum formation are concurrent processes. While S. pombe is the primary source from which the mechanics of ring assembly and constriction stem, much less is known about the regulation of Rho GTPases that control these processes. Of the six Rho GTPases encoded in S. pombe, only Rho1, the RhoA homologue, has been shown to be essential for cytokinesis. While Rho3, Rho4, and Cdc42 have defined roles in cytokinesis, Rho2 and Rho5 play minor to no roles in this process. Here we review the roles of the Rho GTPases during cytokinesis, with a focus on their regulation, and discuss whether crosstalk between GTPases, as has been reported in other organisms, exists during cytokinesis in S. pombe.
Overview
Cytokinesis is necessary for growth, development, cell differentiation, and wound repair; defects in this process can cause diseases such as cancer [Citation1–Citation3]. In metazoans and fungi, cytokinesis occurs via the formation and subsequent constriction of an actomyosin contractile ring. As the ring constricts, it also pulls in the membrane to enable furrow formation, thus physically separating the cell into two daughter cells. In most cells, ring constriction is precisely timed to occur only after the chromosomes segregate, thus ensuring the genomic integrity of the daughter cells. Fungal cells have a cell wall, and in addition to ring constriction and membrane furrow formation, they also form a septum (cell-wall) barrier. Much of what is known about the fundamentals of cytokinesis stem from decades of research with the fission yeast model, Schizosaccharomyces pombe. In fission yeast, cytokinesis involves the assembly of the actomyosin ring, which then undergoes constriction concurrent with the formation of the septum and the membrane furrow [Citation4,Citation5]. First, cortical nodes containing the anillin protein Mid1 assemble around the nucleus which then recruit proteins that are involved in ring formation [Citation6–Citation11]. These include the type II myosin Myo2, its regulatory light chain Rlc1, its light chain Cdc4, the F-BAR protein Cdc15 and the formin Cdc12 [Citation11–Citation18]. The formin Cdc12 nucleates actin and this together with the type II myosin organizes the actin filaments to form a ring like structure [Citation19–Citation22]. After the ring assembles, it only starts to constrict once the septum building apparatus is recruited to the division site and the chromosomes start segregation [Citation23–Citation25].
While it is unclear how the cellular events – ring constriction, septum formation, and membrane ingression – occur simultaneously, we now understand some of the fundamental steps that promote each of these events during cytokinesis. First, the primary septum starts forming as the ring constricts. This requires the delivery of septum synthesizing enzyme Bgs1 to the division site [Citation23,Citation26,Citation27]. Simultaneously, new membrane material is also delivered to the division site for furrow formation [Citation28]. Next, the glucan synthases Bgs4 and Mok1/Ags1 are delivered to the division site to enable secondary septum formation [Citation25]. While Bgs1 is a linear 1,3-β-glucan synthase that mainly builds the primary septum, it is not sufficient to provide rigidity and strength to the septum [Citation26,Citation29]. Bgs4, the 1,6 branched 1,3- β-glucan synthase and Mok1/Ags1 the α-1,3-glucan synthase provides the necessary strength to ensure proper septum morphology and cell integrity [Citation29–Citation32]. After the septum is fully synthesized, glucanases are delivered to the division site to digest the primary septum and separate the two daughter cells [Citation33–Citation35]. Inability to perform any of these events in a precise spatiotemporal manner can lead to cytokinesis failure and cell death. However, our knowledge of how cells properly organize these sequential events and their spatiotemporal regulation is limited.
The conserved small Rho GTPases, Cdc42, Rho1, Rho3 and Rho4 have been reported to promote different steps in cytokinesis () [Citation24,Citation36–Citation39]. Cdc42 is activated at the division site in early anaphase and this promotes delivery of the glucan synthase Bgs1 to the division site [Citation24]. Rho1 in turn activates the glucan synthases and promotes septum formation [Citation40]. Rho3 and Rho4 are required for delivery of the septum digesting glucanases that lead to cell separation [Citation38,Citation41]. These conserved small Rho GTPases are active when GTP-bound and inactive when the GTP is hydrolyzed to GDP [Citation42]. GTPases are activated by guanine nucleotide exchange factors (GEFs) and inactivated by GTPase activating proteins (GAPs) [Citation42]. Most eukaryotes have multiple GTPases, and each has its corresponding GEFs and GAPs. Modulation of the GEFs and GAPs can tune GTPase activity and thus regulate cellular processes that require precision [Citation43]. GTPases crosstalk to self-organize and regulate complex processes such as migration, wound healing, and polarization [Citation44–Citation48]. GTPases also undergo multiple feedback pathways to enable self-organization of its activity, leading to distinct cellular outcomes [Citation47,Citation49]. It is possible that these Rho GTPases fine tune signaling pathways to spatiotemporally regulate distinct cytokinetic events. Here we review and discuss recent advances in our understanding of how Rho GTPases regulate the different, sequential steps in cytokinesis.
Figure 1. Rho family GTPases play distinct roles during cytokinesis. Cdc42 (green) is activated at the assembled actomyosin ring (red) and this promotes onset of ring constriction and septum formation. Rho1 (blue) is activated at the division site to promote septum synthesis. The precise timing of initiation of Rho1 activation is not known. After the septum forms, Rho3 (yellow) and Rho4 (orange) localize to the division site at the outer rim of the septum barrier to promote delivery of the septum digesting glucanases. Digestion of the primary septum leads to cell separation.
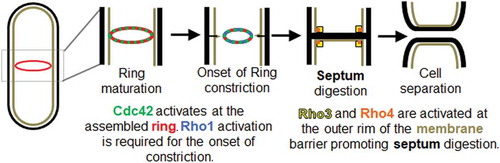
Cdc42
Cdc42 is the major regulator of cell polarity in most eukaryotes [Citation50]. In fission yeast, Cdc42 activates the formin For3 to promote actin organization [Citation51] and the Pak1 kinase to regulate downstream processes [Citation52]. It also plays an important role in polarized trafficking by regulating exocyst-mediated delivery [Citation53]. Cdc42 is activated at the division site during cytokinesis by its two GEFs, Gef1 and Scd1 [Citation24,Citation54]. Activation of Cdc42 at the division site initiates during ring assembly in a Gef1-dependent manner [Citation24,Citation55]. This leads to the recruitment of Bgs1 and initiation of septum ingression and ring constriction. Both Bgs1 localization to the ring and the onset of ring constriction is delayed in mutants lacking gef1 [Citation24]. Scd1 localizes to the division site next in a Gef1-dependent manner to activate Cdc42 along the ingressing membrane [Citation24,Citation49]. Thus, two waves of Cdc42 activation facilitate the recruitment of Bgs1 to specific sites of the ring/membrane barrier: Gef1 facilitates Bgs1 delivery to the ring while Scd1 promotes Bgs1 localization along the ingressing membrane [Citation24]. Although the mechanism through which Cdc42 promotes Bgs1 delivery is not known, it is feasible that this occurs through regulation of actin-dependent trafficking, as gef1 mutants show defects in the localization of the type V myosin as well as fewer non-medial actin cables [Citation24]. Although Cdc42 is required for Bgs1 recruitment, it does not contribute to the localization of Bgs4 [Citation24,Citation56]. Whether Cdc42 regulates the delivery of the other glucan synthases is unknown. Thus, Cdc42 may promote delivery of specific cargoes in addition to the regulation of actin cable formation during cytokinesis.
It has been reported that Bgs1 localization to the division site is promoted by the F-BAR protein Cdc15 [Citation57,Citation58]. However, Bgs1 localization during cytokinesis is regulated by many factors, including Cdc42 [Citation24,Citation59]. This suggests that although Cdc15 is present at the ring prior to the onset of ring constriction, Bgs1 localization to the actomyosin ring is dependent on Cdc42 activation, which is at first solely mediated through Gef1 [Citation24]. Upon blocking endocytosis with the arp2/3 inhibitor CK-666, the ring is able to form, but does not constrict due to the failure to recruit Bgs1 [Citation60]. Furthermore, Cdc15 levels do not increase at the ring after ring assembly in these cells [Citation60]. The endocytosis defective arp3-c1 mutant also exhibits similar phenotypes [Citation60]. A recent report indicates that Cdc42 activation, mediated through Gef1, promotes endocytosis [Citation60]. Specifically, gef1 mutants exhibit endocytic patches with higher levels of Cdc15, as well as extended patch lifetimes [Citation60]. Moreover, these defects can be alleviated upon expression of constitutively active Cdc42, suggesting that Cdc42 activation promotes endocytosis [Citation60].
The septum ingresses centripetally indicating that cell wall must be deposited in a uniform manner along the membrane adjacent to the actomyosin ring. This suggests that Cdc15, and thus the septum building apparatus with which it interacts, must localize uniformly along the actomyosin ring to facilitate centripetal septum deposition [Citation27,Citation29–Citation31,Citation60-Citation62]. In gain of function mutants of the formin cdc12, cdc12Δ503, the cells display excessive actin cables and delayed endocytic patch lifetimes [Citation63]. gef1Δ mutants when combined with cdc12Δ503, show septum ingression defects and do not constrict centripetally [Citation60]. In these gef1Δcdc12Δ503 mutants, the distribution of Cdc15 along the ring is not uniform, resulting in faster constriction rates in areas of the ring that have elevated levels of Cdc15. It is likely that, post ring assembly, additional Cdc15 is recruited to the ring from endocytic patches [Citation60]. In agreement, uneven distribution of Cdc15 along the ring results when endocytosis is disrupted [Citation60]. Together, these findings indicate that membrane trafficking events, including endocytosis, are required to uniformly organize Cdc15 along the ring to facilitate centripetal septum ingression and ring constriction, and that Cdc42 regulates this process.
While Cdc42 is required for the early stages of septum formation, it needs to be inactivated in order for cytokinesis to complete [Citation24]. Cells overexpressing constitutively active Cdc42 form a septum but fail to undergo cell separation [Citation24,Citation64]. Similar observations have been made in budding yeast [Citation36,Citation65]. The GAPs that inactivate Cdc42, Rga3, 4 and 6, localize to the division site suggesting that they lead to Cdc42 inactivation at that site [Citation66–Citation69]. It is not known why Cdc42 needs to be inactivated for completion of cytokinesis.
Recent reports have provided some insights into how the regulators of Cdc42 are themselves regulated. Gef1 has been shown to localize to the division site in a Cdc15 dependent manner [Citation70]. This relationship is also maintained at the cell poles. Cdc15 has been shown to promote Cdc42 activation at the division site and the cell poles in a Gef1-dependent manner [Citation70]. The molecular details of how Cdc15 promotes Gef1 localization is unclear. Another report indicates that Scd1 localization to the division site depends on Gef1 [Citation49]. Gef1 activates Cdc42 at the division site and this acts as a seed for the recruitment of the scaffold protein Scd2. Scd2 then recruits Scd1 to this site. Thus, this compromises a feed-forward pathway where Gef1 promotes Scd1 recruitment. This behavior between the GEFs has also been shown at the cell poles [Citation49]. While Gef1 promotes Scd1 recruitment, Scd1 itself has been shown to promote restriction of Gef1 from the division site [Citation49]. Gef1 localizes to the ring membrane interface and constricts with the ring. Once the ring completes constriction, Gef1 is no longer localized to that site. In scd1Δ cells however, Gef1 lingers at the division site even after the cell has completed constriction. It is unclear how Scd1 promotes the removal of Gef1 from the division site.
Rho1
Like cdc42, rho1 mutants are highly pleiotropic. Rho1 serves as the regulatory subunit of the Bgs1, Bgs2, Bgs3, and Bgs4 glucan synthases, is essential for primary septum formation, and must be inactivated to permit cell separation [Citation40,Citation71]. While Rho1 is activated by Rgf1, Rgf2, and Rgf3, only Rgf3 is essential [Citation37,Citation72,Citation73]. Overexpression of rgf1 or rgf2 results in multi-septated cells and morphology defects [Citation37]. Rgf3 activity, and hence Rho1 activity, is essential for ring formation, as rgf3 switch-off cells do not form competent actomyosin rings [Citation37]. While Rgf1 and Rgf2 contribute to Rho1 activation during septum formation, they primarily regulate Rho1-mediated cell wall deposition during polarized growth [Citation37]. Rgf3 is transcribed by the Ace2-network, along with other proteins necessary for septum formation and cell separation [Citation73]. While Rgf3 localizes to the ring/membrane interphase, Rgf1 and Rgf2 localize to the ingressing membrane [Citation37,Citation73]. This localization pattern is identical to that of the Cdc42 GEFs Gef1 and Scd1, respectively [Citation24], and it is possible that the Rho1 GEFs specifically activate Rho1 at different locations to ensure proper septum synthesis.
The Septation Initiation Network (SIN) pathway is required for proper timing of cytokinesis with respect to completion of mitosis [Citation74]. The SIN pathway promotes timely assembly of the actomyosin ring, recruitment of the septum synthesizing enzyme Bgs1, and promotes septum formation once the chromosomes are segregated in mitosis [Citation61,Citation75–Citation77]. In SIN mutants, the contractile ring assembles but does not undergo constriction [Citation75]. Rho1-mediated activation of the glucan synthase is required for septum formation and septum synthesis [Citation40,Citation71]. Therefore, it is likely that the SIN regulates Rho1 activation to ensure that constriction initiates only upon chromosome segregation to maintain genome integrity. The final kinase in the SIN pathway, Sid2, is required for septum formation and ring constriction [Citation78]. Overexpression of rho1 rescues the sid2-250 ring constriction defect, suggesting that Rho1 activation may be mediated by the SIN during cytokinesis [Citation75]. The SIN pathway is activated by the protein Etd1 in a timely manner with respect to chromosome segregation [Citation76]. Etd1 has also been proposed to link SIN with septum formation [Citation76,Citation79]. Biochemical assays indicate that GTP-Rho1 is reduced in cells lacking etd1, as is 1,3-β-glucan synthase activity, suggesting that Edt1 positively regulates Rho1 activation [Citation76]. Since Etd1 appears to lack both GEF domain and GEF activity, how it promotes Rho1 activation is unclear. An early protein in the SIN pathway cascade is the GTPase Spg1, required for activation of the downstream kinases Sid1 and Sid2 [Citation80,Citation81]. Rho1 signaling initiates a positive feedback with Spg1, further activating SIN signaling during cytokinesis [Citation76].
The paxillin homologue in S. pombe, Pxl1, was identified as a suppressor of the cdc42-1625 temperature sensitive phenotype [Citation82]. Paxillins are LIM-domain containing proteins that facilitate protein-protein interaction and association with the cell wall/extracellular matrix [Citation82]. However, Pxl1 binds Rho1, not Cdc42, and reduces the amount of GTP-Rho1 [Citation82]. Loss of pxl1 results in cells with more GTP-Rho1 and an increased septation index, similar to cells lacking the Rho1 GAP Rga5 [Citation82,Citation83]. This suggests that Pxl1 negatively regulates Rho1, and this is further supported by the observation that pxl1 exhibits strong genetic interactions with rgf3 [Citation82]. An additional function of Pxl1 is to stabilize the actomyosin ring and promote proper septum synthesis through interaction with Bgs1 [Citation58]. How Bgs1 function cooperates with Pxl1 during cytokinesis is unclear, although it has been hypothesized that Pxl1 may act as a mechanosensor that transmits ring tension to the glucan synthases to initiate septum synthesis [Citation58]. Pxl1 binds Cdc15 and Rlc1 and aids actomyosin ring formation [Citation58]. Whether this is dependent on the inhibition of Rho1 is not known. In budding yeast, Rho1 must be temporarily inactivated to enable septum ingression [Citation36]. However, this does not seem to be the case in fission yeast [Citation58]. Paxillin also recruits the calcineurin phosphatase Ppb1 to the division site, which dephosphorylates Cdc15 to enable it to scaffold Bgs1 and initiate septum synthesis [Citation84].
Rho2 and Rho5
Cells overexpressing rho2 exhibit morphological and cytokinetic defects [Citation85]. Past studies primarily focused on the role of Rho2 in the regulation of cell integrity and cell polarity. However, rho2 overexpressing cells have a high septation index and often contain multiple septa, indicating a defect in cell separation [Citation85]. In keeping with this phenotype, Rho2 localizes to the septum during cytokinesis [Citation85]. However, the role of Rho2 in this process has not been directly demonstrated. Rho1 and Rho2 likely regulate the synthesis of distinct glucans, as cell wall defects resulting from one impaired Rho GTPase cannot be complemented by overexpression of the other [Citation85]. The most compelling role for Rho2 during cytokinesis is the activation of the α-glucan synthase Mok1/Ags1, via the protein kinase c homologue Pck2 [Citation86,Citation87]. During cytokinesis, Mok1/Ags1 contributes to the formation of both the primary and secondary septa and is required to prevent lysis during cell separation [Citation31].
With the help of a genetic screen, Rho2 was identified to act upstream of the Pck2-Pmk1 Mitogen activated protein kinase pathway [Citation88]. Rho2 promotes activation of Pmk1 likely by facilitating its phosphorylation [Citation88]. Loss of pmk1 leads to a defect in cell separation and septum morphology [Citation89,Citation90]. Interestingly, loss of a negative regulator of Pmk1, the phosphatase pmp1, also leads to cell separation defects [Citation91]. Thus, it is likely that Rho2 may regulate cytokinesis through the MAP kinase Pmk1. However, it is unclear how this is brought about or why both loss of and hyperactivation of Pmk1 leads to cell separation defects. While pmk1Δ leads to cell separation defects, rho2Δ does not show similar defects. It is possible that in addition to Rho2, other GTPases may also be involved in Pmk1 activation.
Rho5 is not an essential gene, as its activity is redundant with Rho1. Indeed, overexpression of rho5 can complement cells with impaired Rho1 activity [Citation39]. The view that Rho5 is not particularly effective, and that it functions primarily during meiosis and sporulation, explains why rho5Δ cells do not exhibit the polarity defects of cells lacking rho1 [Citation39]. Similar to the overexpression of rho1 or rho2, cells overexpressing rho5 also exhibit morphological defects and have an elevated septation index [Citation39]. Rho5 localizes to the poles during interphase and to the division site during M-phase [Citation39]. While it has not been tested, it is possible that Rho5 contributes to septum formation or ring stability in a manner similar to Rho1. A recent report identifies the GEF Gef2 as an in vitro binding partner of Rho1, Rho4, and Rho5 [Citation92]. Gef2, and its adaptor protein, Nod1, contribute to the positioning of the division site, and confer stability to the actomyosin ring [Citation92]. It is tempting to speculate that Rho1 or Rho5-mediated glucan synthase activity is required to position or stabilize the actomyosin ring. While Rho1 and Rho5 have a positive role in cytokinesis, it is unclear why overexpression of these proteins lead to an increased septation index. It is possible that excessive Rho1/5 activation leads to uncontrolled septum synthesis that may interfere with the septum digestion process. Alternately, it is possible that increasing Rho1 and Rho5 impairs proper activation of other small GTPases involved in cytokinesis, such as Rho3 and Rho4. This may lead to defects in cell separation that would result in a high septation index.
Rho3 and Rho4
Aside from Rho1, the roles of Rho3 and Rho4 are the best characterized among this family of GTPases. Together, Rho3 and Rho4 enable cell separation through the delivery and secretion of the cell wall glucanases, Agn1 and Eng1 [Citation38,Citation41,Citation93,Citation94]. rho3Δ rho4Δ double mutants fail to separate and grow in a multiseptated state [Citation94]. The observation that rho3Δ mutants phenocopy the cytokinetic defects of exocyst mutants, and that rho3 overexpression complements cell separation and thermosensitivity defects of sec8-1 and exo70Δ mutants, respectively, suggests that Rho3 most likely functions by promoting exocyst activity [Citation41]. To date, no Rho3 GEFs have been identified. Although Gef3 binds Rho3, it does not function as a Rho3 GEF, but likely stabilizes or recruits Rho3 at the ring/membrane interphase [Citation95]. While gef3Δ cells exhibit delayed cell separation, gef3Δ combined with for3Δ or exocyst mutants exhibit the severe phenotypes of rho3Δ mutants, suggesting that Gef3 plays an important role in the Rho3-mediated vesicle trafficking and tethering events that are required for cell separation [Citation95]. While Rho3 likely enables fusion of vesicles containing cell wall hydrolases to the rim of the septum/membrane barrier in an exocyst-dependent manner, this hypothesis has not been fully tested.
Unlike rho3 overexpression, rho4 is not sufficient to suppress the cell separation defects of exocyst mutants [Citation94]. However, agn1 or eng1 overexpression suppresses the multi-septation phenotype exhibited by rho4Δ mutants [Citation94]. Moreover, Agn1 and Eng1 fail to localize to the septum at elevated temperatures in rho4Δ mutants, and their secretion is impaired [Citation93,Citation94]. While Rho4 is dependent on the exocyst for its localization, the exocyst and the septins require Rho4 to localize to the division site [Citation94]. Together, this suggests that Rho4 positions the septin ring and exocyst complex to enable the Rho3-mediated secretion of Agn1 and Eng1 that facilitates cell separation. Rho4 may also regulate septum formation, as rho4Δ cells exhibit excessive secondary septum [Citation38]. Rho4 has but one bonafide GEF, Gef3, which activates Rho4 at the septin ring [Citation96]. Deletion of gef3 results in the mislocalization of Agn1 and Eng1, and results in delayed cell abscission [Citation92]. An additional GEF, Gef2, also interacts with Rho4. Gef2, binds Rho1, Rho4, and Rho5, although its GEF activity toward these GTPases have yet to be demonstrated [Citation92]. Gef2 promotes Rho4 localization to the division site, although the mechanism has not been elucidated [Citation92]. Gef2 localizes to cortical nodes and aids in the positioning of the contractile ring, for which its GEF activity is required [Citation92]. It is tempting to speculate that Rho1 or Rho5 may play a role in this process, since the Rho1 orthologue RhoA mediates the placement and assembly of the actomyosin ring in higher eukaryotes [Citation97].
Concluding remarks
Just as there are multiple steps in cytokinesis that must occur in a precise order, there are multiple Rho GTPases to regulate each step of this process. Cdc42 controls several events during cytokinesis, beginning with the timing of constriction onset through the localization of Bgs1 [Citation24]. Through the regulation of endocytic patch dynamics, Cdc42 regulates Cdc15 distribution along the actomyosin to ensure centripetal septum formation [Citation60]. Cdc42, along with Rho1 and Rho4, also regulates septum morphology to ensure that the cell is competent for cell separation [Citation96]. Cdc42 and Rho4 regulate the membrane trafficking and remodeling events necessary for cell separation [Citation96]. Rho1 is the primary regulator of septum formation, although Rho2 and Rho5 may contribute to this process [Citation39,Citation40,Citation86]. Rho1 also reinforces SIN activity during cytokinesis [Citation75,Citation76]. Finally, Rho3 and Rho4 regulate the delivery and secretion of the glucanases that promote cell separation [Citation38,Citation41,Citation93,Citation94]. Given the overlap of Rho GTPase activity in many of these processes, it is possible that crosstalk between these GTPases coordinates their activity, or prevents the premature activity of another.
Although Rho GTPase crosstalk has been well documented in other organisms, little is known about this process in fission yeast [Citation98]. The clearest example of crosstalk between Rho GTPases involves RhoA and Cdc42, which are mutually antagonistic in Xenopus oocytes during wound healing [Citation46]. However, this type of relationship has not yet been described in S. pombe. Cdc42 and Rho4 activity may be coordinated during the process of septum formation. rho4Δ mutants exhibit septum defects consisting of excessive secondary septum, similar to those of scd1Δ cells [Citation24,Citation38]. Furthermore, rho4Δ exhibits a strong genetic interaction with the Cdc42 GEF, Gef1, in which gef1Δ rho4Δ and gef1Δ gef3Δ double mutant populations exhibit an elevated septation index and a high fraction of multiseptated cells [Citation96]. Gef3/Rho3 and Cdc42 may also play complementary roles in the regulation of the membrane trafficking and remodeling steps during late cytokinesis [Citation95]. These two GTPase signaling pathways likely converge at the For3/Pob1 complex, which is essential for cell separation [Citation99–Citation101]. Pob1 is a downstream effector of Cdc42 and is required for actin organization and membrane trafficking [Citation99–Citation101]. pob1-664 mutants show secretory vesicle accumulation and exhibit actin cable defects, but can be complemented by overexpression of rho3 [Citation101]. Rho3 also appears to antagonize Rho1, as deletion of gef3 suppresses cell integrity defects of rgf3 switch off mutants [Citation95]. Crosstalk may also exist between Rho3 and Rho4 at multiple levels. First, Rho4 positions the septin ring and the exocyst at the division site, which is required for the subsequent localization of Rho3 [Citation41,Citation94]. Second, Rho4 is activated at the division site by the Rho3 GEF Gef3 [Citation96]. It is likely that this then facilitates Rho3 localization through Gef3.
In budding yeast, the polo kinase Cdc5 recruits the Rho1 GEFs Tus1 and Rom2 to the bud neck, which in turn enables Rho1 activation to initiate ring assembly [Citation102]. The fission yeast orthologue of polo kinase, Plo1, initiates SIN signaling to control the timing of cytokinesis [Citation7,Citation81]. To what extent the SIN leads to the activation of the Rho GTPases during cytokinesis in S. pombe is unclear. An interesting feedback loop exists between the SIN and Rho1. The SIN protein Etd1 activates Rho1, which in turn enhances SIN activity [Citation76]. SIN signaling likely coordinates with cell separation as well as with septum formation. Cells mutated in the terminal SIN kinase, Sid2, lyse after multiple rounds of mitosis uninterrupted by cytokinesis. Lysis of sid2-250 cells is suppressed by the deletion of either rho4 or gef2, suggesting that SIN signaling and cell separation may be integrated by the GEF Gef2 [Citation92].
By requiring a sequential step of events involving multiple GTPases, the cell provides a mechanism to ensure that multiple waves/sequences of proteins are delivered to a precise location at a specific time. Since cytokinetic events are precisely timed, such a manner of regulation would function as an internal clock or checkpoint to ensure the fidelity of cell separation.
Disclosure statement
The authors do not have any financial or non-financial competing interests.
Additional information
Funding
References
- Li R. Cytokinesis in development and disease: variations on a common theme. Cell Mol Life Sci. 2007 Dec;64(23):3044–3058. PubMed PMID: 17882379.
- Guertin DA, Trautmann S, McCollum D. Cytokinesis in eukaryotes. Microbiol Mol Biol Rev. 2002 Jun;66(2):155–178. PubMed PMID: 12040122; PubMed Central PMCID: PMC120788.
- Sagona AP, Stenmark H. Cytokinesis and cancer. FEBS Lett. 2010 Jun 18;584(12):2652–2661. PubMed PMID: 20371245.
- Pollard TD. The value of mechanistic biophysical information for systems-level understanding of complex biological processes such as cytokinesis. Biophys J. 2014 Dec 2;107(11):2499–2507. PubMed PMID: 25468329; PubMed Central PMCID: PMCPMC4255220.
- Pollard TD, Wu JQ. Understanding cytokinesis: lessons from fission yeast. Nat Rev Mol Cell Biol. 2010 Feb;11(2):149–155. PubMed PMID: 20094054; PubMed Central PMCID: PMCPMC2819279.
- Paoletti A, Chang F. Analysis of mid1p, a protein required for placement of the cell division site, reveals a link between the nucleus and the cell surface in fission yeast. Mol Biol Cell. 2000 Aug;11(8):2757–2773. PubMed PMID: 10930468; PubMed Central PMCID: PMCPMC14954.
- Bahler J, Steever AB, Wheatley S, et al. Role of polo kinase and Mid1p in determining the site of cell division in fission yeast. J Cell Biol. 1998 Dec 14;143(6):1603–1616. PubMed PMID: 9852154; PubMed Central PMCID: PMCPMC2132972.
- Hachet O, Simanis V. Mid1p/anillin and the septation initiation network orchestrate contractile ring assembly for cytokinesis. Genes Dev. 2008 Nov 15;22(22):3205–3216. PubMed PMID: 19056897; PubMed Central PMCID: PMC2593605.
- Celton-Morizur S, Bordes N, Fraisier V, et al. C-terminal anchoring of mid1p to membranes stabilizes cytokinetic ring position in early mitosis in fission yeast. Mol Cell Biol. 2004 Dec;24(24):10621–10635. PubMed PMID: 15572668; PubMed Central PMCID: PMC533969.
- Coffman VC, Nile AH, Lee IJ, et al. Roles of formin nodes and myosin motor activity in Mid1p-dependent contractile-ring assembly during fission yeast cytokinesis. Mol Biol Cell. 2009 Dec;20(24):5195–5210. PubMed PMID: 19864459; PubMed Central PMCID: PMC2793295.
- Laporte D, Coffman VC, Lee IJ, et al. Assembly and architecture of precursor nodes during fission yeast cytokinesis. J Cell Biol. 2011 Mar 21;192(6):1005–1021. PubMed PMID: 21422229; PubMed Central PMCID: PMC3063137.
- Wu JQ, Kuhn JR, Kovar DR, et al. Spatial and temporal pathway for assembly and constriction of the contractile ring in fission yeast cytokinesis. Dev Cell. 2003 Nov;5(5):723–734. PubMed PMID: 14602073.
- Mulvihill DP, Hyams JS. Role of the two type II myosins, Myo2 and Myp2, in cytokinetic actomyosin ring formation and function in fission yeast. Cell Motility Cytoskeleton. 2003 Mar;54(3):208–216. PubMed PMID: 12589679.
- Kitayama C, Sugimoto A, Yamamoto M. Type II myosin heavy chain encoded by the myo2 gene composes the contractile ring during cytokinesis in Schizosaccharomyces pombe. J Cell Biol. 1997 Jun 16;137(6):1309–1319. PubMed PMID: 9182664; PubMed Central PMCID: PMC2132538.
- McCollum D, Balasubramanian MK, Pelcher LE, et al. Schizosaccharomyces pombe cdc4+ gene encodes a novel EF-hand protein essential for cytokinesis. J Cell Biol. 1995 Aug;130(3):651–660. PubMed PMID: 7622565; PubMed Central PMCID: PMCPMC2120525.
- Le Goff X, Motegi F, Salimova E, et al. The S. pombe rlc1 gene encodes a putative myosin regulatory light chain that binds the type II myosins myo3p and myo2p. J Cell Sci. 2000 Dec;113 Pt 23:4157–4163. PubMed PMID: 11069761.
- Naqvi NI, Wong KC, Tang X, et al. Type II myosin regulatory light chain relieves auto-inhibition of myosin-heavy-chain function. Nat Cell Biol. 2000 Nov;2(11):855–858. PubMed PMID: 11056543.
- Chang F, Drubin D, Nurse P. cdc12p, a protein required for cytokinesis in fission yeast, is a component of the cell division ring and interacts with profilin. J Cell Biol. 1997 Apr 7;137(1):169–182. PubMed PMID: 9105045; PubMed Central PMCID: PMCPMC2139860.
- Pelham RJ, Chang F. Actin dynamics in the contractile ring during cytokinesis in fission yeast. Nature. 2002 Sep 5;419(6902):82–86. PubMed PMID: 12214236.
- Vavylonis D, Wu JQ, Hao S, et al. Assembly mechanism of the contractile ring for cytokinesis by fission yeast. Science. 2008 Jan 4;319(5859):97–100. PubMed PMID: 18079366.
- Kamasaki T, Osumi M, Mabuchi I. Three-dimensional arrangement of F-actin in the contractile ring of fission yeast. J Cell Biol. 2007 Aug 27;178(5):765–771. PubMed PMID: 17724118; PubMed Central PMCID: PMCPMC2064542.
- Huang J, Huang Y, Yu H, et al. Nonmedially assembled F-actin cables incorporate into the actomyosin ring in fission yeast. J Cell Biol. 2012 Nov 26;199(5):831–847. PubMed PMID: 23185032; PubMed Central PMCID: PMC3514790.
- Le Goff X, Woollard A, Simanis V. Analysis of the cps1 gene provides evidence for a septation checkpoint in Schizosaccharomyces pombe. MGG. 1999 Aug;262(1):163–172. PubMed PMID: 10503548.
- Wei B, Hercyk BS, Mattson N, et al. Unique Spatiotemporal Activation Pattern of Cdc42 by Gef1 and Scd1 Promotes Different Events during Cytokinesis. Mol Biol Cell. 2016 Mar 3;27(8):1235–1245. PubMed PMID: 26941334.
- JC GC, Ramos M, Konomi M, et al. Specific detection of fission yeast primary septum reveals septum and cleavage furrow ingression during early anaphase independent of mitosis completion. PLoS Genet. 2018 May;14(5):e1007388. PubMed PMID: 29813053; PubMed Central PMCID: PMCPMC5993333.
- Cortes JC, Konomi M, Martins IM, et al. The (1,3)beta-D-glucan synthase subunit Bgs1p is responsible for the fission yeast primary septum formation. Mol Microbiol. 2007 Jul;65(1):201–217. PubMed PMID: 17581129.
- Cortes JC, Ishiguro J, Duran A, et al. Localization of the (1,3)beta-D-glucan synthase catalytic subunit homologue Bgs1p/Cps1p from fission yeast suggests that it is involved in septation, polarized growth, mating, spore wall formation and spore germination. J Cell Sci. 2002 Nov 1;115(Pt 21):4081–4096. PubMed PMID: 12356913.
- Wang N, Lee IJ, Rask G, et al. Roles of the TRAPP-II Complex and the Exocyst in Membrane Deposition during Fission Yeast Cytokinesis. PLoS Biol. 2016 Apr;14(4):e1002437. PubMed PMID: 27082518; PubMed Central PMCID: PMC4833314.
- Munoz J, Cortes JC, Sipiczki M, et al. Extracellular cell wall beta(1,3)glucan is required to couple septation to actomyosin ring contraction. J Cell Biol. 2013 Oct 28;203(2):265–282. PubMed PMID: 24165938; PubMed Central PMCID: PMC3812973.
- Cortes JC, Carnero E, Ishiguro J, et al. The novel fission yeast (1,3)beta-D-glucan synthase catalytic subunit Bgs4p is essential during both cytokinesis and polarized growth. J Cell Sci. 2005 Jan 1;118(Pt 1):157–174. PubMed PMID: 15615781.
- Cortes JC, Sato M, Munoz J, et al. Fission yeast Ags1 confers the essential septum strength needed for safe gradual cell abscission. J Cell Biol. 2012 Aug 20;198(4):637–656. PubMed PMID: 22891259; PubMed Central PMCID: PMCPMC3514033.
- Hochstenbach F, Klis FM, van Den Ende H, et al. Identification of a putative alpha-glucan synthase essential for cell wall construction and morphogenesis in fission yeast. Proc Natl Acad Sci U S A. 1998 Aug 4;95(16):9161–9166. PubMed PMID: 9689051; PubMed Central PMCID: PMCPMC21309.
- Lee IJ, Coffman VC, Wu JQ. Contractile-ring assembly in fission yeast cytokinesis: recent advances and new perspectives. Cytoskeleton. 2012 Oct;69(10):751–763. PubMed PMID: 22887981.
- Pollard TD. Mechanics of cytokinesis in eukaryotes. Curr Opin Cell Biol. 2010 Feb;22(1):50–56. PubMed PMID: 20031383; PubMed Central PMCID: PMC2871152.
- Goyal A, Takaine M, Simanis V, et al. Dividing the spoils of growth and the cell cycle: the fission yeast as a model for the study of cytokinesis. Cytoskeleton. 2011 Feb;68(2):69–88. PubMed PMID: 21246752; PubMed Central PMCID: PMC3044818.
- Onishi M, Ko N, Nishihama R, et al. Distinct roles of Rho1, Cdc42, and Cyk3 in septum formation and abscission during yeast cytokinesis. J Cell Biol. 2013 Jul 22;202(2):311–329. PubMed PMID: 23878277; PubMed Central PMCID: PMC3718969.
- Mutoh T, Nakano K, Mabuchi I. Rho1-GEFs Rgf1 and Rgf2 are involved in formation of cell wall and septum, while Rgf3 is involved in cytokinesis in fission yeast. Genes Cells. 2005 Dec;10(12):1189–1202. PubMed PMID: 16324155.
- Nakano K, Mutoh T, Arai R, et al. The small GTPase Rho4 is involved in controlling cell morphology and septation in fission yeast. Genes Cells. 2003 Apr;8(4):357–370. PubMed PMID: 12653963.
- Nakano K, Arai R, Mabuchi I. Small GTPase Rho5 is a functional homologue of Rho1, which controls cell shape and septation in fission yeast. FEBS Lett. 2005 Sep 26;579(23):5181–5186. PubMed PMID: 16146630.
- Arellano M, Duran A, Perez P. Rho 1 GTPase activates the (1-3)beta-D-glucan synthase and is involved in Schizosaccharomyces pombe morphogenesis. Embo J. 1996;15(17):4584–4591. PubMed PMID: 8887550; eng.
- Wang H, Tang X, Balasubramanian MK. Rho3p regulates cell separation by modulating exocyst function in Schizosaccharomyces pombe. Genetics. 2003 Aug;164(4):1323–1331. PubMed PMID: 12930742; PubMed Central PMCID: PMCPMC1462644.
- Bos JL, Rehmann H, Wittinghofer A. GEFs and GAPs: critical elements in the control of small G proteins. Cell. 2007 Jun 1;129(5):865–877. PubMed PMID: 17540168.
- Das M, Nunez I, Rodriguez M, et al. Phosphorylation-dependent inhibition of Cdc42 GEF Gef1 by 14-3-3 protein Rad24 spatially regulates Cdc42 GTPase activity and oscillatory dynamics during cell morphogenesis. Mol Biol Cell. 2015 Aug 5. PubMed PMID: 26246599. DOI:10.1091/mbc.E15-02-0095.
- Yang L, Wang L, Zheng Y. Gene targeting of Cdc42 and Cdc42GAP affirms the critical involvement of Cdc42 in filopodia induction, directed migration, and proliferation in primary mouse embryonic fibroblasts. Mol Biol Cell. 2006;17(11):4675–4685. PubMed PMID: 16914516; eng.
- Machacek M, Hodgson L, Welch C, et al. Coordination of Rho GTPase activities during cell protrusion. Nature. 2009;461(7260):99–103. PubMed PMID: 19693013; eng.
- Benink HA, Bement WM. Concentric zones of active RhoA and Cdc42 around single cell wounds. J Cell Biol. 2005 Jan 31;168(3):429–439. PubMed PMID: 15684032; PubMed Central PMCID: PMC2171735.
- Das M, Drake T, Wiley DJ, et al. Oscillatory Dynamics of Cdc42 GTPase in the Control of Polarized Growth. Science. 2012 May 17;337(6091):239–243. PubMed PMID: 22604726; Eng.
- Howell AS, Jin M, Wu CF, et al. Negative feedback enhances robustness in the yeast polarity establishment circuit. Cell. 2012 Apr 13;149(2):322–333. PubMed PMID: 22500799; PubMed Central PMCID: PMC3680131.
- Hercyk B, Rich J, Das ME. A novel interplay between GEFs orchestrates Cdc42 activation in cell polarity and cytokinesis. bioRxiv. 2018. DOI:10.1101/364786
- Etienne-Manneville S. Cdc42–the centre of polarity. J Cell Sci. 2004;117(Pt 8):1291–1300. PubMed PMID: 15020669; eng.
- Martin SG, Rincon SA, Basu R, et al. Regulation of the formin for3p by cdc42p and bud6p. Mol Biol Cell. 2007;18(10):4155–4167. PubMed PMID: 17699595; eng.
- Tu H, Wigler M. Genetic evidence for Pak1 autoinhibition and its release by Cdc42. Mol Cell Biol. 1999 Jan;19(1):602–611. PubMed PMID: 9858584; PubMed Central PMCID: PMC83918.
- Bendezu FO, Vincenzetti V, Martin SG. Fission yeast Sec3 and Exo70 are transported on actin cables and localize the exocyst complex to cell poles. PloS One. 2012;7(6):e40248. PubMed PMID: 22768263; PubMed Central PMCID: PMC3386988.
- Rincon S, Coll PM, Perez P. Spatial regulation of Cdc42 during cytokinesis. Cell Cycle (georgetown, Tex). 2007;6(14):1687–1691. PubMed PMID: 17637568; eng.
- Hirota K, Tanaka K, Ohta K, et al. Gef1p and Scd1p, the Two GDP-GTP exchange factors for Cdc42p, form a ring structure that shrinks during cytokinesis in Schizosaccharomyces pombe. Mol Biol Cell. 2003 Sep;14(9):3617–3627. PubMed PMID: 12972551; PubMed Central PMCID: PMC196554.
- Estravis M, Rincon S, Perez P. Cdc42 regulation of polarized traffic in fission yeast. Commun Integr Biol. 2012 Jul 1;5(4):370–373. PubMed PMID: 23060961; PubMed Central PMCID: PMC3460842.
- Arasada R, Pollard TD. Contractile ring stability in S. pombe depends on F-BAR protein Cdc15p and Bgs1p transport from the Golgi complex. Cell Rep. 2014 Sep 11;8(5):1533–1544. PubMed PMID: 25159149; PubMed Central PMCID: PMC4163078.
- Cortes JC, Pujol N, Sato M, et al. Cooperation between Paxillin-like Protein Pxl1 and Glucan Synthase Bgs1 Is Essential for Actomyosin Ring Stability and Septum Formation in Fission Yeast. PLoS Genet. 2015 Jul;11(7):e1005358. PubMed PMID: 26132084; PubMed Central PMCID: PMCPMC4489101.
- Estravis M, Rincon SA, Santos B, et al. Cdc42 regulates multiple membrane traffic events in fission yeast. Traffic. 2011 Dec;12(12):1744–1758. PubMed PMID: 21899677.
- Onwubiko UN, Mlynarczyk PJ, Wei B, et al. A Cdc42 GEF, Gef1, through endocytosis organizes F-BAR Cdc15 along the actomyosin ring and promotes concentric furrowing. J Cell Sci. 2019 Feb 1. PubMed PMID: 30709916. DOI:10.1242/jcs.223776.
- Liu J, Tang X, Wang H, et al. The localization of the integral membrane protein Cps1p to the cell division site is dependent on the actomyosin ring and the septation-inducing network in Schizosaccharomyces pombe. Mol Biol Cell. 2002 Mar;13(3):989–1000. PubMed PMID: 11907277; PubMed Central PMCID: PMC99614.
- Martin V, Garcia B, Carnero E, et al. Bgs3p, a putative 1,3-beta-glucan synthase subunit, is required for cell wall assembly in Schizosaccharomyces pombe. Eukaryot Cell. 2003 Feb;2(1):159–169. PubMed PMID: 12582133; PubMed Central PMCID: PMC141170.
- Coffman VC, Sees JA, Kovar DR, et al. The formins Cdc12 and For3 cooperate during contractile ring assembly in cytokinesis. J Cell Biol. 2013 Oct 14;203(1):101–114. PubMed PMID: 24127216; PubMed Central PMCID: PMC3798249.
- Miller PJ, Johnson DI. Cdc42p GTPase is involved in controlling polarized cell growth in Schizosaccharomyces pombe. Mol Cell Biol. 1994 Feb;14(2):1075–1083. PubMed PMID: 8289788; PubMed Central PMCID: PMC358463.
- Atkins BD, Yoshida S, Saito K, et al. Inhibition of Cdc42 during mitotic exit is required for cytokinesis. J Cell Biol. 2013 Jul 22;202(2):231–240. PubMed PMID: 23878274; PubMed Central PMCID: PMC3718968.
- Tatebe H, Nakano K, Maximo R, et al. Pom1 DYRK regulates localization of the Rga4 GAP to ensure bipolar activation of Cdc42 in fission yeast. Curr Biol. 2008;18(5):322–330. PubMed PMID: 18328707; eng.
- Das M, Wiley DJ, Medina S, et al. Regulation of cell diameter, For3p localization, and cell symmetry by fission yeast Rho-GAP Rga4p. Mol Biol Cell. 2007;18(6):2090–2101. PubMed PMID: 17377067; eng.
- Revilla-Guarinos MT, Martin-Garcia R, Villar-Tajadura MA, et al. Rga6 is a Fission Yeast Rho GAP Involved in Cdc42 Regulation of Polarized Growth. Mol Biol Cell. 2016 Mar 09. PubMed PMID: 26960792; PubMed Central PMCID: PMCPMC4850039. DOI:10.1091/mbc.E15-12-0818.
- Gallo Castro D, Martin SG. Differential GAP requirement for Cdc42-GTP polarization during proliferation and sexual reproduction. J Cell Biol. 2018 Dec 3;217(12):4215–4229. PubMed PMID: 30279276; PubMed Central PMCID: PMCPMC6279383.
- Hercyk BS, Das ME. F-BAR Cdc15 Promotes Gef1-mediated Cdc42 Activation During Cytokinesis and Cell Polarization in S. pombe. bioRxiv. 2019;552927. DOI:10.1101/552927
- Nakano K, Arai R, Mabuchi I. The small GTP-binding protein Rho1 is a multifunctional protein that regulates actin localization, cell polarity, and septum formation in the fission yeast Schizosaccharomyces pombe. Genes Cells. 1997 Nov;2(11):679–694. PubMed PMID: 9491802.
- Tajadura V, Garcia B, Garcia I, et al. Schizosaccharomyces pombe Rgf3p is a specific Rho1 GEF that regulates cell wall beta-glucan biosynthesis through the GTPase Rho1p. J Cell Sci. 2004 Dec 1;117(Pt 25):6163–6174. PubMed PMID: 15546915.
- Morrell-Falvey JL, Ren L, Feoktistova A, et al. Cell wall remodeling at the fission yeast cell division site requires the Rho-GEF Rgf3p. J Cell Sci. 2005 Dec 1;118(Pt 23):5563–5573. PubMed PMID: 16291723.
- Simanis V. Pombe’s thirteen - control of fission yeast cell division by the septation initiation network. J Cell Sci. 2015 Apr 15;128(8):1465–1474. PubMed PMID: 25690009.
- Jin QW, Zhou M, Bimbo A, et al. A role for the septation initiation network in septum assembly revealed by genetic analysis of sid2-250 suppressors. Genetics. 2006 Apr;172(4):2101–2112. PubMed PMID: 16415366; PubMed Central PMCID: PMCPMC1456390.
- Alcaide-Gavilan M, Lahoz A, Daga RR, et al. Feedback regulation of SIN by Etd1 and Rho1 in fission yeast. Genetics. 2014 Feb;196(2):455–470. PubMed PMID: 24336750; PubMed Central PMCID: PMCPMC3914619.
- Roberts-Galbraith RH, Gould KL. Stepping into the ring: the SIN takes on contractile ring assembly. Genes Dev. 2008 Nov 15;22(22):3082–3088. PubMed PMID: 19056889.
- Sparks CA, Morphew M, McCollum D. Sid2p, a spindle pole body kinase that regulates the onset of cytokinesis. J Cell Biol. 1999 Aug 23;146(4):777–790. PubMed PMID: 10459013; eng.
- Garcia-Cortes JC, McCollum D. Proper timing of cytokinesis is regulated by Schizosaccharomyces pombe Etd1. J Cell Biol. 2009 Sep 7;186(5):739–753. PubMed PMID: 19736319; PubMed Central PMCID: PMCPMC2742193.
- Schmidt S, Sohrmann M, Hofmann K, et al. The Spg1p GTPase is an essential, dosage-dependent inducer of septum formation in Schizosaccharomyces pombe. Genes Dev. 1997 Jun 15;11(12):1519–1534. PubMed PMID: 9203579.
- Cullen CF, May KM, Hagan IM, et al. A new genetic method for isolating functionally interacting genes: high plo1(+)-dependent mutants and their suppressors define genes in mitotic and septation pathways in fission yeast. Genetics. 2000 Aug;155(4):1521–1534. PubMed PMID: 10924454; PubMed Central PMCID: PMCPMC1461180.
- Pinar M, Coll PM, Rincon SA, et al. Schizosaccharomyces pombe Pxl1 is a paxillin homologue that modulates Rho1 activity and participates in cytokinesis. Mol Biol Cell. 2008 Apr;19(4):1727–1738. PubMed PMID: 18256290; PubMed Central PMCID: PMC2291433.
- Calonge TM, Arellano M, Coll PM, et al. Rga5p is a specific Rho1p GTPase-activating protein that regulates cell integrity in Schizosaccharomyces pombe. Mol Microbiol. 2003 Jan;47(2):507–518. 3312 [pii]. PubMed PMID: 12519200; eng.
- Martin-Garcia R, Arribas V, Coll PM, et al. Paxillin-Mediated Recruitment of Calcineurin to the Contractile Ring Is Required for the Correct Progression of Cytokinesis in Fission Yeast. Cell Rep. 2018 Oct 16;25(3):772–783 e4. PubMed PMID: 30332655.
- Hirata D, Nakano K, Fukui M, et al. Genes that cause aberrant cell morphology by overexpression in fission yeast: a role of a small GTP-binding protein Rho2 in cell morphogenesis. J Cell Sci. 1998 Jan;111(Pt 2):149–159. PubMed PMID: 9405296.
- Arellano M, Valdivieso MH, Calonge TM, et al. Schizosaccharomyces pombe protein kinase C homologues, pck1p and pck2p, are targets of rho1p and rho2p and differentially regulate cell integrity. J Cell Sci. 1999 Oct;112(Pt 20):3569–3578. PubMed PMID: 10504305; eng.
- Katayama S, Hirata D, Arellano M, et al. Fission yeast alpha-glucan synthase Mok1 requires the actin cytoskeleton to localize the sites of growth and plays an essential role in cell morphogenesis downstream of protein kinase C function. J Cell Biol. 1999 Mar 22;144(6):1173–1186. PubMed PMID: 10087262; PubMed Central PMCID: PMCPMC2150588.
- Ma Y, Kuno T, Kita A, et al. Rho2 is a target of the farnesyltransferase Cpp1 and acts upstream of Pmk1 mitogen-activated protein kinase signaling in fission yeast. Mol Biol Cell. 2006 Dec;17(12):5028–5037. PubMed PMID: 17005909; PubMed Central PMCID: PMCPMC1679671.
- Zaitsevskaya-Carter T, Cooper JA. Spm1, a stress-activated MAP kinase that regulates morphogenesis in S.pombe. Embo J. 1997 Mar 17;16(6):1318–1331. PubMed PMID: 9135147; PubMed Central PMCID: PMCPMC1169729.
- Toda T, Dhut S, Superti-Furga G, et al. The fission yeast pmk1+ gene encodes a novel mitogen-activated protein kinase homolog which regulates cell integrity and functions coordinately with the protein kinase C pathway. Mol Cell Biol. 1996 Dec;16(12):6752–6764. PubMed PMID: 8943330; PubMed Central PMCID: PMCPMC231678.
- Sugiura R, Toda T, Shuntoh H, et al. pmp1+, a suppressor of calcineurin deficiency, encodes a novel MAP kinase phosphatase in fission yeast. Embo J. 1998 Jan 2;17(1):140–148. PubMed PMID: 9427748; PubMed Central PMCID: PMCPMC1170365.
- Zhu YH, Ye Y, Wu Z, et al. Cooperation between Rho-GEF Gef2 and its binding partner Nod1 in the regulation of fission yeast cytokinesis. Mol Biol Cell. 2013 Oct;24(20):3187–3204. PubMed PMID: 23966468; PubMed Central PMCID: PMC3806657.
- Santos B, Martin-Cuadrado AB, Vazquez de Aldana CR, et al. Rho4 GTPase is involved in secretion of glucanases during fission yeast cytokinesis. Eukaryot Cell. 2005 Oct;4(10):1639–1645. PubMed PMID: 16215171; PubMed Central PMCID: PMCPMC1265894.
- Perez P, Portales E, Santos B. Rho4 interaction with exocyst and septins regulates cell separation in fission yeast. Microbiology. 2015 May;161(Pt 5):948–959. PubMed PMID: 25724972.
- Munoz S, Manjon E, Sanchez Y. The putative exchange factor Gef3p interacts with Rho3p GTPase and the septin ring during cytokinesis in fission yeast. J Biol Chem. 2014 Aug 8;289(32):21995–22007. PubMed PMID: 24947517; PubMed Central PMCID: PMC4139216.
- Wang N, Wang M, Zhu YH, et al. The Rho-GEF Gef3 interacts with the septin complex and activates the GTPase Rho4 during fission yeast cytokinesis. Mol Biol Cell. 2015 Jan 15;26(2):238–255. PubMed PMID: 25411334; PubMed Central PMCID: PMC4294672.
- Chircop M. Rho GTPases as regulators of mitosis and cytokinesis in mammalian cells. Small GTPases. 2014;5. PubMed PMID: 24988197; PubMed Central PMCID: PMC4160341. DOI:10.4161/sgtp.29770.
- Guilluy C, Garcia-Mata R, Burridge K. Rho protein crosstalk: another social network?. Trends Cell Biol. 2011 Dec;21(12):718–726. PubMed PMID: 21924908; PubMed Central PMCID: PMCPMC3221770.
- Toya M, Iino Y, Yamamoto M. Fission yeast Pob1p, which is homologous to budding yeast Boi proteins and exhibits subcellular localization close to actin patches, is essential for cell elongation and separation. Mol Biol Cell. 1999 Aug;10(8):2745–2757. PubMed PMID: 10436025; PubMed Central PMCID: PMCPMC25510.
- Rincon SA, Ye Y, Villar-Tajadura MA, et al. Pob1 participates in the Cdc42 regulation of fission yeast actin cytoskeleton. Mol Biol Cell. 2009 Oct;20(20):4390–4399. PubMed PMID: 19710424; PubMed Central PMCID: PMC2762138.
- Nakano K, Toya M, Yoneda A, et al. Pob1 ensures cylindrical cell shape by coupling two distinct rho signaling events during secretory vesicle targeting. Traffic. 2011 Jun;12(6):726–739. PubMed PMID: 21401840.
- Yoshida S, Kono K, Lowery DM, et al. Polo-like kinase Cdc5 controls the local activation of Rho1 to promote cytokinesis. Science. 2006 Jul 7;313(5783):108–111. PubMed PMID: 16763112.