Abstract
The development of potential metrics for comparing black carbon (BC) to carbon dioxide has been requested within legislation in the United States and has been discussed at the United Nations Framework Convention on Climate Change Conference of the Parties in Poznan: therefore, it is important to further investigate the advantages and drawbacks to using such a metric. For context, we summarize the various proposed CO2 equivalent metrics and the rationales for developing them. We use BC marginal abatement curves to examine the implications of using 100-year global warming potentials to include BC in greenhouse gas (GHG) trading regimes. This idealized study demonstrates the impacts on emissions of CO2, and radiative forcing over time. Finally, we address the drawbacks of trading poorly quantified short-lived emissions with GHGs despite different physical interactions with the climate system. While the case for reducing BC for both health and climate benefits is compelling, there are reasons for limiting the use of BC metrics to illustrative analyses, such as identifying which BC mitigation actions would provide the greatest climate benefits, rather than using these metrics for trading with GHGs. Indeed, market-based mechanisms in general may not be appropriate for BC regulation at this time.
1. Introduction
H.R. 2996, the US Department of the Interior, Environment, and Related Agencies Appropriations Act of 2010, which was signed into law on 30 October 2009, calls for “… an assessment of potential metrics and approaches for quantifying the climatic effects of black carbon emissions (including its radiative forcing and warming effects) and comparing those effects to the effects of carbon dioxide and other greenhouse gases …” Therefore, it is worth another examination of what applications of these metrics are appropriate, where their use may be counterproductive, and what good alternatives might exist.
Black carbon (BC)'s potential importance as a factor in anthropogenic climate change has been recognized for more than a decade (Haywood and Shine Citation1995). On a global scale, changes in BC loading from preindustrial have been estimated to have a forcing effect of 0.34 W/m2 ± 0.25 W/m2 by the Intergovernmental Panel on Climate Change (IPCC) (Forster et al. Citation2007). The broad uncertainty range does not even include the central estimate of 0.9 W/m2 of a more recent review (Ramanathan and Carmichael Citation2008), which in turn has an uncertainty of 45%. Additional snow albedo feedback may be on the order of 0.1 ± 0.1 W/m2 (Forster et al. Citation2007) but studies indicate that the efficacy of that forcing may be three times the efficacy of CO2 forcing on a global scale (Flanner et al. Citation2007), with even larger relative impacts when considering Arctic systems or the Hindu Kush-Himalayan-Tibetan (HKHT or third pole) region. This range of estimates demonstrates that the radiative forcing uncertainty due to BC loading is large compared to the uncertainty in forcing due to changes in well-mixed GHG concentrations of only 10% of 2.63 W/m2 (Forster et al. Citation2007).
Radiative forcing can be used as a measure of how the atmospheric loading of BC compares to the loading of other greenhouse gases (GHGs). However, there is also significant interest in comparing the emissions of BC to those of the other gases, and this article explores the implications of developing a metric that can be useful for that task.
2 Rationales for developing BC metrics
Metrics facilitate quantitative comparisons between two different actions. Such a comparison enables ranking of different options by, for example, cost-effectiveness for achieving a given goal. This allows decision makers to ensure that resources are being distributed in an efficient fashion. Where possible, policymakers would prefer a single number for comparison. Metrics entered the climate change arena in 1990 when the IPCC chose to use a formulation for global warming potentials (GWPs) parallel to the metric used by the Montreal Protocol, namely the ozone depleting potential.
Perhaps the largest driver for developing a metric is that a metric is necessary in order to enable market-based trading systems, where an additional reduction of one substance can be traded for an increased emission of another substance. These markets can then efficiently equalize marginal abatement costs between substances, a trait that is known as “what” based flexibility (Böhringer et al. Citation2006). Other such flexibility mechanisms include “where” based flexibility for trading between regions and “when” based flexibility for inter-temporal trading (banking and borrowing). For example, using global “where” flexibility for CO2 reductions is justified on the grounds that as a well-mixed gas, reductions in one region of the world will have approximately the same climate impacts as reductions in another region. “When” flexibility is justified because the benefits of CO2 reductions are usually not considered to differ greatly depending on the year in which they take place. A number of papers have demonstrated advantages of including “what” based flexibility in climate policies in order to lower costs of achieving temperature reductions goals, though many of these papers use a counterfactual that involves only reducing carbon dioxide and therefore are not really addressing the benefits of trading but rather the benefits of a multi-gas policy (Böhringer et al. Citation2006).
A related goal is to give BC reduction projects access to the funds provided by carbon-offset markets. If BC reductions are being underprovided relative to the desire of policymakers, and policy tools such as command and control regulations are undesirable or infeasible, then enabling access to an existing source of funds can achieve reduction goals. The existence of offset systems also gives private companies incentives to seek out reportable, verifiable, measurable, enforceable, and additional sources of reductions, and the inventiveness of the market may discover new options for reductions that governmental regulation would not have found. Policies such as the European Trading System and some of the proposed US cap and trade laws only explicitly cover CO2 and a subset of sectors, but allow offsets from non-covered sectors and gases to enter by the use of verifiable offsets. This is in addition to international offsets through the clean development mechanism that enables reductions of GHGs in developing nations that are not otherwise covered by Kyoto to enter the Kyoto trading regime. Given that these offset mechanisms exist, it may be desirable to include climatically relevant pollutants such as BC within climate reduction trading programs.
Metrics for comparing BC to GHGs are also useful for evaluating individual reduction measures. For example, Jacobson studied the impacts of converting from gasoline to diesel vehicles (Jacobson Citation2002, Citation2005) and showed that under certain emissions and CO2 lifetime assumptions, the net temperature impact of the conversion could be warming for up to a century or more despite the reductions of CO2. Metrics are useful so that rather than running simulations for every combination of reductions, one can just add up the CO2-equivalent values in order to evaluate, in a rough fashion, the existence of net positive or negative reductions from a given action. Indeed, one of the first applications of GWPs was to calculate the net effects of switching from oil to natural gas (Rodhe Citation1990).
Even if BC/GHG metrics are not appropriate for use in trading programs, they can be useful for giving a broad view of options. Metrics can be used to compare the CO2-equivalent value of available BC reductions to other available GHG reductions, thereby giving a qualitative estimate of the appropriate level of effort spent addressing these reductions, even if these metrics are not used directly to ensure equal CO2-equivalent prices between different options. Existing legislation also provides implicit relative valuation, and metrics can be used to determine potential areas of undervaluation. Similarly, for industries and individuals that examine their “carbon footprints”, use of a metric allows them to quickly determine which parts of their operations have the largest climate impacts and are worth the most effort to examine for emissions reductions opportunities.
3 Limitations of metrics for comparing BC to CO2
Despite the existence of a number of rationales for developing BC metrics, there are a number of reasons for caution in the use of these metrics, mainly based on several key differences between BC and CO2. We review three of these differences here: drastically different atmospheric lifetimes, more uncertainty in terms of the radiative forcing of BC, and different mechanisms of interaction with the climate system.
The lifetime of BC is on the order of 1 or 2 weeks, compared to a lifetime of more than a year for the shortest lived hydrofluorocarbon in the Kyoto basket, and a lifetime of decades to centuries or more for CO2. This first raises a temporal valuation issue of how to weigh benefits several decades from now against benefits today. However, in addition to a valuation issue, a short temporal lifetime means that BC will not be well-mixed globally (1–2 months is required for mixing within a hemisphere, and on the order of 1 year for mixing between hemispheres), unlike the GHGs to which it is being compared. This means that unlike most GHGs, the climatic effect of BC can depend significantly on when and where it is emitted, with strong dependence on the albedo of the surfaces below the plume, local interactions with clouds, and the number of daylight hours available. Importantly, only certain BC emissions will have direct impacts on certain vulnerable systems such as the Arctic or HKHT plateau. Therefore, “where” based flexibility mechanisms are less appropriate for BC.
As noted in the Introduction section, the uncertainty in determining the forcing for BC is large compared to the equivalent uncertainty for GHGs. This uncertainty is due in part to the interactions between BC and other substances in the atmosphere – most of the higher estimates of forcing, such as from Ramanathan and Carmichael (Citation2008), come from studies that include more realistic mixing of BC with other aerosols. Geographic heterogeneity of BC loading and the indirect interactions with clouds add further complexity on top of the difficulties of accurately modeling the aging process. Trading a well-understood gas for BC would therefore introduce unnecessary uncertainty.
The third difference involves the mechanism of interaction with the climate system – BC absorbs incoming and reflecting solar radiation, whereas GHGs absorb only outgoing longwave radiation. BC therefore has a dimming effect that GHGs do not have, BC creates a different vertical profile of warming, and BC has unique hydrological and meteorological interactions. For example, atmospheric heating and dimming from BC and other aerosols has been linked to perturbations in the South Asian monsoon and other tropical precipitation trends (Ramanathan and Carmichael Citation2008). It would be difficult to capture these interactions within one single global metric.
Therefore, while metrics based on global average properties such as radiative forcing or temperature change have been generally accepted for substances which are similar, such as the long-lived GHGs, there are a number of key climatic issues that need to be kept in mind when extending these metrics to short-lived aerosols such as BC.
4 Existing metrics for comparing BC to CO2
Several standard metrics have been used for comparing the climatic effects of GHGs on the global scale. The GWPs used in Kyoto are a measure of the integrated radiative forcing over 100 years resulting from emitting a pulse of a GHG. While a recent IPCC Expert Meeting determined that there are still shortcomings to the GWP approach even for the well-mixed gases, such as the somewhat arbitrary choice of 100 years for the integration and a need to better characterize uncertainties such as the sensitivity of the GWP to the choice of carbon cycle model, the judgment was that the GWPs are still a useful metric for use in climate policy (IPCC 2009). Some alternate metrics have been assessed in the literature. Manne and Richels (Citation2001) used a forward-looking model to calculate the optimal relative values of different gases over time in order to meet a given target such as temperature at a certain date or a maximum rate of change of temperature. More recently, global temperature potentials (GTPs) have been developed that use the change in temperature after 100 years as a metric rather than integrated radiative forcing (Shine et al. Citation2005a). Part of the rationale for a temperature metric is that it is a better proxy than radiative forcing for those impacts that are of interest to humans and ecosystems (e.g. sea level rise, ice retreat, heat waves). There are two forms of this GTP metric: the first involves a pulse of emissions, and the second involves sustained reductions of emissions throughout the 100-year time period. The analytic solution for a GTP with sustained reductions is very similar to a GWP, though computational solutions may differ depending on details of the model.
Recently, there have been several extensions of these metrics to comparing BC and CO2 emissions. Metrics based on integrating either reductions or forcings over 100-year timescales show per unit mass BC emissions reductions having an impact ranging from 330 (Jacobson Citation2005) to 2240 (Jacobson Citation2007) that of per unit mass CO2 emissions reductions. However, this fairly wide span of values is perhaps misleading: the lowest estimates are metrics for “fossil soot” (a mixture of BC and OC), some estimates include the snow albedo effect, some include dependence on the location of the emissions (such as in Rypdal et al. Citation2009), some are temperature based where others are radiation based, and some use a drastically different carbon cycle model. Some of these estimates were presented in of Bond (Citation2007), but we can now include several additional estimates published in the intervening years. Here we also present extrapolations that adjust for some of the major structural differences between the estimates. After adjustments for carbon cycle lifetime and removing organic carbon (OC) and indirect effects, the 100-year integrated metrics show a range of global mean values from 480 to about 1130 for the direct effect of BC, and a range of 700 to about 1340 if snow albedo effects are included ().
Table 1. BC warming potentials.
Rather than sustaining reductions for 100 years for the GTP, it is possible to calculate a similar metric using shorter term reductions. Berntsen et al. (Citation2006) reduce BC emissions for a 20-year time span, and find that for reducing global temperatures in 100 years, these BC reductions are only 120–230 more effective than an equal reductions by mass of CO2 emissions. Boucher and Reddy (Citation2008) find that when emissions are reduced for a short pulse (1 year), the resulting GTP values are usually a factor 7 lower than their GWPs. Shine et al. also extended their GTP concept in the spirit of Manne and Richels (Citation2001), finding that as the temperature target is approached, the value of short-lived substances skyrockets. In this new analysis (Shine et al. Citation2007), BC has a GTP of about 100 in 2010 (similar to the Boucher and Reddy result) but the GTP exceeds 22,000 as the climate approaches the target temperature. By not valuing the short-term benefits of emission reductions, reliance on non-integrative measures would mean that for the extreme case where reductions of emissions today had zero effect on temperature in 100 years, there would be no incentive to reduce those emissions despite possibly significant short-term benefits.
Using different time periods other than 100 years has also been explored for metrics. Those who are concerned with near-term Arctic impacts commonly suggest 20-year GWPs as appropriate. Jacobson estimates a 20-year GWP for BC of 4470 (Jacobson Citation2007). However, for those concerned about the long-term problem, even 100-year GWPs can be considered to be too short (Solomon et al. Citation2009).
5 Inclusion of black carbon in US Climate Policy
If a single metric can be agreed upon for BC, there will likely be interest in including BC as a potential offset mechanism in climate policies such as the recent American Clean Energy and Security Act of 2009 (ACES) bill which was passed in the US House of Representatives, or S.1733 (the Kerry–Boxer bill) which is being debated in the US Senate – S.1733 already includes a Clean Vehicle Technology investment fund that may stimulate further BC reductions. Acknowledging the limitations of the metrics discussed above, it can be instructive to examine the potential implications of their use in such climate policies. The ACES bill allows up to a billion metric Mgs each of domestic and international offsets. Using an arbitrary $40 price, and marginal abatement curves for BC mitigation (Sarofim et al. Citation2010), it is possible to calculate the quantity of BC that would be abated given different GWPs. The least expensive diesel retrofit abatement option was found to be $35,600 per Mg of BC for retrofitting 2006 class 8b trucks, which would yield 21,500 Mgs of lifetime abatement. This would be cost effective at a GWP of 890, given the above price. At a higher GWP of 1250, abatement would be worthwhile at $50,000 per Mg of BC. At this price, we found that 66,000 Mgs of BC abatement was available, mainly for class 8 trucks and older, more polluting busses. At this upper end, that would be 82.5 million Mgs CO2eq – less than 10% of the available domestic retrofit potential in 2010, and the reductions themselves would be spread over the lifetime of the retrofitted vehicles. As the GWP estimate for BC increases, not only do more retrofits become cost effective, but each Mg of BC reduced displaces a larger quantity of CO2 reductions. Inclusion of a credit or subsidy for air quality benefits, or an increased carbon price, would also make more BC retrofits cost-effective. Note that estimates of the air quality benefits in the US of mobile source carbon reductions are on the order of 165,000 to 1.9 million dollars/Mg (Fann et al. Citation2009), significantly larger than the likely combinations of GWPs and near-term carbon prices.
shows BC emissions over time in the business as usual case, where emissions drop due to vehicle turnover after 2007 when all new on-road vehicles were required to install diesel particulate filters, and a retrofit scenario case where all retrofits less expensive than $50,000/Mg BC are installed. shows the radiative forcing of the BC reduced over time, and compares this to the radiative forcing from the CO2 emissions that would be displaced by the traded BC permits, assuming the Bern carbon cycle approximation for CO2 concentrations and a BC GWP of 1000. Note that there are additional 47,000 Mgs of BC abatement available annually at this price from reductions in the biomass/open burning sector – however, these reductions are accompanied by a significant fraction of organic carbon, and one of the major challenges of including BC offsets in any policy will be appropriate accounting for OC. About 400,000 Mgs of BC are emitted total in the US, which at a GWP of 1000 is about 6% as large as the total GHG emissions of the nation. These emissions are projected to decline by more than a third due to existing air quality policies such as the Clean Air Highway and non-road Diesel Rules, demonstrating the importance of health benefits as a driver for mitigation of BC.
Figure 1. (a) Word 3 Future US BC emissions from on-road sources under business as usual and also with a policy assuming that all diesel retrofits available for less than $50K/lifetime Mg of BC abated are implemented in 2010. (b) The tall, blue curve shows the radiative forcing change from the BC reductions in Figure 1a. The long, green curve shows the radiative forcing change from a reduction of CO2 in each year of the program that would result from trading the BC reductions for CO2 reductions at a GWP of 1000. This highlights the difference in the temporal nature of radiative forcing changes resulting from BC and CO2 emission reductions.
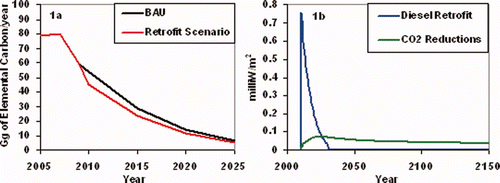
By using the range of GWPs presented in (480 to 1340 for BC, and 0 to −250 for OC) and an EPA emissions inventory for the US, it is also possible to show the net climate effect of various emissions sources () as an example of one illustrative use of metrics.
6 Extension to global scale
Globally, Rypdal et al. (Citation2009) found that there are 416 Ggs of BC reduction available annually at an average cost of $7200/Mg BC (probably less than $20,000 marginal cost). This is sufficient to supply almost half of the international offset program for the American Clean Energy and Security Act, but as the international offset program of the Act is likely to be saturated, and there will be competition for international offsets with other nation's climate programs, the implications for US climate policy of making this offset pool available are unclear. According to Bond et al. (Citation2004), a total of 8 Tg of BC are emitted annually around the world. At a GWP of 1000, this would be 8 GtCO2eq, or about 20% as large as total global GHG emissions excluding land-use change and forestry. Inclusion of BC in global climate treaties could therefore have a fairly significant impact on emissions prices and quantities. However, it should be noted that as developing nation economies grow, it is likely that the GHG emissions of these nations will grow quickly while the BC emissions may be controlled through clean air policies, in which case the relative contribution of BC may decrease to levels similar to those in the US and Europe.
7 Discussion
This article explores several issues involved in developing and using metrics for comparing BC and GHGs. This includes an illustrative assessment of the magnitude of the effect of including BC in GHG trading regimes at the national and international level. In the case where BC emissions are small in comparison to total GHG reductions, which may be true in the case of US domestic policies, the various distortions introduced by the differences in effect of BC and the long-lived GHGs may be smaller than the advantages of an additional source of funding for BC reductions. However, on the global scale there are currently significantly more BC emissions and abatement available, making the distortions more important. The choice of time scale and metric is also important, but the 100-year integrated forcing metric may be an acceptable compromise.
However, better solutions may exist that enable multi-attribute optimization, where both short-term rate of change and long-term commitment issues can be addressed. One way to do this is to create a set of policies that address short-term forcers and reduce them based on how much short-term change is acceptable, and a separate policy for long-term forcers that addresses a willingness to accept long-term impacts and temperature changes. The relative stringencies of the policies may end up being different in terms of $/mass-CO2 equivalent measured by 100-year GWPs, but because they are solving different problems the prices should not be expected to be equal.
There are also some significant implementation issues. The uncertainties in forcing are paralleled by uncertainties in inventories. These uncertainties may make it difficult to meet standards for measureable, reportable, and verifiable reductions, as does the small, mobile, variable, or area nature of many BC sources. Co-emitted substances such as organic carbon and sulfates also need to be taken into account. For CO2 policies, the possible ancillary reductions of sulfates due to diminished use of coal have been of academic interest but policymakers have ignored this effect for purposes of CO2 trading. This may be because of the perceived priority of health interests, or the indirect nature of the ancillary reductions, or perhaps because of the very different lifetimes of the two substances. However, it is unlikely that BC climate policies can take the same approach. As shows, reduction of BC from some sectors may well be net cooling rather than net warming.
There is clearly a role for BC emissions reductions in mitigation of future climate change and future climate strategies and treaties. Various metrics such as GWPs and GTPs can serve an important role in selection of abatement options and illustrating effects of reduction programs. However, like others before us (Shine et al. Citation2005b; Forster et al. Citation2006) we caution that the use of these metrics for trading may be unwise, or at the least premature, due to the differences in climate characteristics such as lifetime and hydrological cycle impacts, the uncertainties in inventory and global forcing impacts, and the difficulty in creating viable measurement programs for verifiable reductions. The advantages of “what” flexibility do not necessarily outweigh the disadvantages of reducing a complex problem to a single attribute for market optimization. We encourage further exploration of methods to reduce emissions of BC and other short-lived species without necessarily using “what” or “where” based flexibility. Indeed, there might even be a benefit to including CH4 and the short-lived HFCs in a single policy aimed at reducing the rate of near-term climate change, while another policy continues to focus on CO2 and the other truly long-lived GHGs.
Notes
Disclaimer: This publication was developed under Cooperative Agreement No. X3 83232801 awarded by the U.S. Environmental Protection Agency to the American Association for the Advancement of Science. It has not been formally reviewed by the EPA. The views expressed in this document are solely those of the author and do not necessarily reflect those of the Agency. The EPA does not endorse any products or commercial services mentioned in this publication.
References
- Berntsen , T , Fuglestvedt , J , Myhre , G , Stordal , F and Berglen , T F . 2006 . Abatement of greenhouse gases: does location matter? . Climatic Change. , 74 ( 4 ) : 377 – 411 .
- Böhringer , C , Löschel , A and Ruthetford , T F . 2006 . Efficiency gains from “what”-flexibility in climate policy: an integrated CGE assessment . Energy J. , : 405 – 424 .
- Bond , T C . 2007 . Can warming particles enter global climate discussions? . Environ Res Lett. , 2 ( 4 ) : 045030 (9p)
- Bond , T C , Streets , D G , Yarber , K F , Nelson , S M , Woo , J H and Klimont , Z . 2004 . A technology-based global inventory of black and organic carbon emissions from combustion . J Geophys Res-Atmos. , 109 ( D14 ) : D14203 (43p)
- Bond , T C and Sun , H L . 2005 . Can reducing black carbon emissions counteract global warming? . Environ Sci Technol , 39 ( 16 ) : 5921 – 5926 .
- Boucher , O and Reddy , M S . 2008 . Climate trade-off between black carbon and carbon dioxide emissions . Energy Policy. , 36 : 193 – 200 .
- Fann , N , Fulcher , C M and Hubbell , B J . 2009 . The influence of location, source, and emission type in estimates of the human health benefits of reducing a ton of air pollution . Air Qual Atmos Health. , 2 : 169 – 176 .
- Flanner , M G , Zender , C S , Randerson , J T and Rasch , P J . 2007 . Present-day climate forcing and response from black carbon in snow . J Geophys Res-Atmos. , 112 ( D11 ) : D11202 (17p)
- Forster , P , Ramaswamy , V , Artaxo , P , Berntsen , T , Betts , R , Fahey , D W , Haywood , J , Lean , J , Lowe , D C Myhre , G . 2007 . “ Changes in atmospheric constituents and in radiative forcing ” . In Climate change 2007: the physical science basis. Contribution of Working Group I to the Fourth Assessment Report of the Intergovernmental Panel on Climate Change , Edited by: Solomon , S , Qin , D , Manning , M , Chen , Z , Marquis , M , Avery , K B , Tignor , M and Miller , H L . 129 – 234 . Cambridge, United Kingdom and New York, NY, USA : Cambridge University Press .
- Forster , P , Shine , K and Stuber , N . 2006 . It is premature to include non-CO2 effects of aviation in climate trading schemes . Atmos Environ. , 40 ( 6 ) : 1117 – 1121 .
- Hansen , J , Sato , M , Kharecha , P , Russell , G , Lea , D W and Siddall , M . 2007 . Climate change and trace gases . Philos Trans R Soc A. , 365 : 1925 – 1954 .
- Haywood , J M and Shine , K P . 1995 . The effect of anthropogenic sulfate and soot on the clear sky planetary radiation budget . Geophys Res Lett. , 22 : 603 – 606 .
- IPCC . 2009 . “ Meeting report of the expert meeting on the science of alternative metrics ” . In IPCC Working Group I Technical Support Unit , Edited by: Plattner , G K , Stocker , T F , Midgley , P and Tignor , M . 75 Bern, , Switzerland : University of Bern .
- Jacobson , M Z . 2002 . Control of fossil–fuel particulate black carbon and organic matter, possibly the most effective method of slowing global warming . J Geophys Res-Atmos. , 107 ( D19 ) : 4410 – 4432 .
- Jacobson , M Z . 2005 . Correction to control of fossil–fuel particulate black carbon and organic matter, possibly the most effective method of slowing global warming . J Geophys Res-Atmos. , 110 : D14105
- Jacobson , M Z . 2007 . Testimony for the hearing on black carbon and climate change, U.S House Committee on Oversight and Government Reform [Cited October 18]. Available from: http://oversight.house.gov/images/stories/documents/20071018110606.pdf.
- Manne , A S and Richels , R G . 2001 . An alternative approach to establishing trade-offs among greenhouse gases . Nature. , 410 ( 6829 ) : 675 – 677 .
- Ramanathan , V and Carmichael , G . 2008 . Global and regional climate changes due to black carbon . Nature Geosci. , 1 ( 4 ) : 221 – 227 .
- Reddy , M S and Boucher , O . 2007 . Climate impact of black carbon emitted from energy consumption in the world's regions . Geophys Res Lett. , 34 ( 11 ) : 5
- Rodhe , H . 1990 . A comparison of the contribution of various gases to the greenhouse effect . Science. , 248 ( 4960 ) : 1217 – 1219 .
- Rypdal , K , Rive , N , Berntsen , T , Klimont , Z , Mideksa , T K , Myhre , G and Skeie , R B . 2009 . Costs and global impacts of black carbon abatement strategies . Tellus B. , 61 ( 4 ) : 625 – 641 .
- Sarofim , M C , DeAngelo , B J , Beach , R H , Weitz , K A , Bahner , M A and Zapata Figueroa , AM. 2010 . Marginal abatement cost curves for U.S. black carbon emissions . J Integr Environ Sci. , 7 ( S1 ) : 279 – 288 .
- Shine , K P , Berntsen , T K , Fuglestvedt , J S and Sausen , R . 2005b . Scientific issues in the design of metrics for inclusion of oxides of nitrogen in global climate agreements . PNAS. , 102 ( 44 ) : 15768 – 15773 .
- Shine , K P , Berntsen , T K , Fuglestvedt , J S , Skeie , R B and Stuber , N . 2007 . Comparing the climate effect of emissions of short- and long-lived climate agents . Philos Trans R Soc A – Math Phys Eng Sci. , 365 ( 1856 ) : 1903 – 1914 .
- Shine , K P , Fuglestvedt , J S , Hailemariam , K and Stuber , N . 2005a . Alternatives to the global warming potential for comparing climate impacts of emissions of greenhouse gases . Climatic Change. , 68 ( 3 ) : 281 – 302 .
- Solomon , S , Plattner , G K , Knutti , R and Friedlingstein , P . 2009 . Irreversible climate change due to carbon dioxide emissions . Proc Natl Acad Sci USA. , 106 ( 6 ) : 1704 – 1709 .