Abstract
A liquid chromatography-tandem mass spectrometry-based isotope dilution method was developed for the analysis of the triazine compounds melamine (MEL), ammeline (AMN), ammelide (AMD) and cyanuric acid (CYA) in infant formula samples purchased in Canada in 2008 for the purpose of a combined exposure and risk assessment. Infant formula samples were extracted with 1:1 acetonitrile–water, cleaned up on disposable ion-exchange solid-phase extraction cartridges, and analysed by ultra-high-performance liquid chromatography-tandem mass spectrometry. MEL and CYA were detected in almost all infant formula products: the highest concentrations observed were 0.32 mg kg−1 MEL and 0.45 mg kg−1 CYA. Samples that were relatively high in MEL in this survey tended to be low in CYA, and vice versa. Concentrations of AMN and AMD were very low in all samples. The total of MEL-related compounds (sum of all four analytes) in all samples was below the interim standard of 0.5 mg kg−1 for infant formula products established by Health Canada.
Introduction
Melamine (MEL) and the related compounds ammeline (AMN), ammelide (AMD) and cyanuric acid (CYA) are 1,3,5-triazine compounds (). MEL and CYA are both produced in high volumes (>1000 tonnes year−1): MEL is used in a variety of materials, including plastics, insulation, cleansers and flame retardants; CYA is used in the production of plastics and disinfectants, and as a chlorine stabilizer in swimming pools. AMN and AMD are not industrially produced but are metabolites of MEL and are often found as impurities in MEL and CYA feedstocks (Ehling et al. Citation2007). In addition, many triazine-based chemicals can degrade to MEL or CYA, such as the pesticide cyromazine (Yokley et al. Citation2000) and a variety of disinfectants used in food processing (World Health Organization (WHO) Citation2009).
Figure 1. Chemical structures of melamine (MEL), ammeline (AMN), ammelide (AMD) and cyanuric acid (CYA).
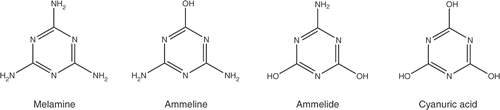
Because MEL and CYA are inexpensive, readily available and nitrogen rich, they were surreptitiously added to food and/or feed ingredients to boost their apparent protein content and increase their market value. Such adulteration exploits a limitation of the most common protein tests, which use total nitrogen as an indicator of protein content. Two separate adulteration incidents have garnered heavy media attention. In 2007, wheat gluten and rice protein concentrate containing significant amounts of MEL and CYA was sold by several Chinese companies. Its use in pet foods resulted in the deaths of a number of pets in Europe and North America. In 2008, thousands of Chinese infants became ill after drinking MEL-adulterated milk, with six confirmed deaths (Gossner et al. Citation2009).
The adulteration incidents in China, combined with the use of milk powder and vegetable protein as ingredients in many processed foods and the global nature of modern food production, prompted many countries to examine the extent of contamination in their own food supply, to establish limits for these chemicals in foods, and to recall violative products (Food Standards Australia New Zealand (FSANZ) Citation2008; Canadian Food Inspection Agency (CFIA) Citation2009; US Food and Drug Administration (USFDA) Citation2009). Canada adopted interim standards of 1 mg kg−1 MEL in infant formula (later revised to 0.5 mg kg−1) and 2.5 mg kg−1 MEL in foods containing dairy-based ingredients, and many countries adopted these or similar limits. The WHO (Citation2009) stated that limits of 1 mg kg−1 MEL in powdered infant formula and 2.5 mg kg−1 MEL in other foods would provide a sufficient margin of safety for dietary exposure.
Although MEL has been the primary research and policy focus, MEL and CYA together exhibit behaviour that may be overlooked in exposure and risk assessments that examine each compound in isolation. MEL and CYA are relatively non-toxic individually, but in combination they form a complex that precipitates in the kidneys and induces renal failure (Puschner et al. Citation2007; Dobson et al. Citation2008). Infant formula analysis and risk assessment of MEL were performed in 2008 by Health Canada's Bureau of Chemical Safety (Health Canada Citation2008b; Tittlemier et al. Citation2009). The current paper details the expansion of the analytical method to include CYA, AMD and AMN, and results of the analysis of the original infant formula samples (purchased in 2008) for these additional compounds.
Materials and methods
Standards and reagents
AMN (≥95% purity) and AMD (≥98% purity) were obtained from TCI America (Portland, OR, USA). Individual ampoules containing 100 µg ml−1 MEL (≥98% purity), CYA (≥98% purity), 13C3-ammeline (13C-AMN; ≥98% purity, 99% isotopic purity), 13C3-ammelide (13C-AMD; ≥98% purity, 99% isotopic purity), 15N3,13C3-melamine (15N13C-MEL; ≥95% purity, ≥98% isotopic purity) and 15N3,13C3-cyanuric acid (15N13C-CYA; ≥90% purity, ≥98% isotopic purity) were purchased from Cambridge Isotope Laboratories (CIL; Andover, MA, USA). Ampoules containing 100 µg ml−1 13C3-melamine (13C-MEL; ≥98% purity, ≥99% isotopic purity), 13C3-cyanuric acid (13C-CYA; ≥98% purity, ≥99% isotopic purity) were obtained from Wellington Laboratories, Inc. (Guelph, ON, Canada). Standards were diluted with 9:1 (v/v) acetonitrile–water.
Acetonitrile (ACN), dichloromethane (DCM), methanol (MeOH) and water used in LC-MS/MS analysis were of OmniSolv grade, obtained from EMD Chemicals (Darmstadt, Germany). Water used in sample and standard preparation was purified using a Milli-Q system (Millipore, Billerica, MA, USA). Hydrochloric acid (HCl; 37.4%, reagent grade), concentrated formic acid (>96%, reagent grade) and ammonium formate (97%, reagent grade) were obtained from Sigma-Aldrich (Madison, WI, USA). Concentrated ammonium hydroxide (NH4OH; 30%) was purchased from Mallinckrodt Baker, Inc. (Phillipsburg, NJ, USA). Glacial acetic acid and sodium hydroxide (NaOH; analytical grade pellets) were obtained from BDH Chemicals Ltd (Poole, UK).
Samples
Ninety-four infant formula products were purchased from major retailers in Ottawa, ON, Canada. Products were purchased in the autumn of 2008, and included liquid (n = 31), powdered (n = 63), milk-based (n = 73), soy-based (n = 19), iron-fortified (n = 71) and calcium-fortified (n = 17) formulas. Powdered formula and unopened liquid formula containers were stored at room temperature. Samples were analysed as purchased and were not prepared as for consumption.
Extraction and clean-up
Approximately 1.0 g of infant formula (liquid or powder) was accurately weighed into a 50 ml polypropylene centrifuge tube. Samples were fortified with 50 ng each of 13C-MEL, 13C-AMN and 13C-AMD, and 500 ng 13C-CYA to monitor analyte recovery. After being allowed to stand at room temperature for 30 min, 20 ml 1:1 ACN–water and 10 ml DCM were added, the tube was capped and shaken briefly by hand, then mixed on a rotary mixer (approximately 60 rpm) for 10 min. Tubes were centrifuged for 10 min at 12,800 g and 4°C. Two 1.0 ml aliquots of the supernatant were distributed into separate 15 ml disposable glass culture tubes. One aliquot was diluted with 2 ml 0.1 N HCl; the other aliquot was diluted with 2 ml 0.1 N NaOH.
Mixed-mode ion exchange/reversed phase SPE cartridges (Oasis MAX and Oasis MCX; 150 mg, 30 µm, 6 ml, Waters Corp., Milford, MA, USA) were conditioned with 5 ml MeOH followed by 5 ml water. The NaOH-diluted aliquots were applied to Oasis MAX cartridges, and the HCl-diluted aliquots were applied to Oasis MCX cartridges. All cartridges were then washed with 3 ml water, followed by 3 ml ACN and 3 ml MeOH. CYA was eluted from the MAX cartridges with 3 ml 2% acetic acid in MeOH; the other analytes were eluted from the MCX cartridges with 3 ml 5% NH4OH in MeOH. MCX and MAX eluates were evaporated to dryness under N2 in a 50°C water bath, and reconstituted in 500 µl 90:10 (v/v) ACN–water (containing 5 pg µl−1 15N13C-MEL and 50 pg µl−1 15N13C-CYA, used as performance standards to account for matrix effects on ionization). Samples were mixed well, filtered through a 0.2 µm nylon syringe filter directly into a sample vial, and stored at room temperature until instrumental analysis.
Instrumental analysis
All samples, blanks and standards were analysed using a Waters Acquity ultra-high-pressure liquid chromatograph (UPLC) coupled to a Waters Quattro-Premier XE triple-quadrupole tandem mass spectrometer (Waters Corp., Milford, MA, USA). Samples (5.0 µl injections) were chromatographed at 55°C on a Waters Acquity UPLC BEH hydrophilic interaction (HILIC) column (2.1 × 100 mm, 1.7 µm) using a binary mobile phase of (A) 2 mM ammonium formate and 0.02% (v/v) formic acid in water and (B) 100% ACN. The initial flow rate was 0.175 ml min−1. The initial mobile phase composition was 9.0% A, which was held for 2 min, then increased to 20% A at 4 min, then held for 6 min. The flow rate was increased to 0.20 ml min−1 at 10.5 min, then to 0.25 ml min−1 at 12.5 min in order to speed conditioning of the column back to the initial mobile phase composition of 9.0% A. At 13 min, the flow rate was decreased to the initial rate of 0.175 ml min−1.
The mass spectrometer (MS) source temperature was held at 120°C, and the desolvation temperature at 350°C. The cone and desolvation gas (N2) flows were 50 and 950 l h−1, respectively. The collision gas (argon) pressure was maintained at 8.47 × 10−3 mbar (0.4 ml min−1). The multiplier voltage was held at 650 V for positive ion detection and 675 V for negative ion detection. Both quadrupole mass analysers were run at baseline unit resolution.
CYA and its associated internal standards were analysed using multiple reaction monitoring (MRM) in negative ion electrospray mode (ES−), with the capillary and cone voltages held at 3.0 kV and 30 V, respectively. All other analytes were analysed using MRM in positive ion electrospray mode (ES+), with the capillary and cone voltages held at 3.5 kV and 35 V, respectively. Details of the transitions monitored and their associated conditions are provided in ; a chromatogram of a fortified infant formula sample is shown in .
Figure 2. Chromatograms of the quantitation MRM transitions for an infant formula sample, fortified at 0.05 mg kg−1 (CYA) and 0.01 mg kg−1 (MEL, AMN and AMD); internal standards are 0.25 mg kg−1 (CYA) and 0.025 mg kg−1 (MEL, AMN and AMD).

Table 1. MRM conditions for tandem mass spectrometry.
Data analysis
Data analysis was performed using the MassLynx 4.1 data system (Waters Corp., Milford, MA, USA) on the UPLC-MS/MS system. Analyte peaks were considered to be identified if the retention times of analytes in samples and blanks were within 0.3 min of the average retention time in calibration standards, the peak had a signal-to-noise ratio of greater than 9:1, and the ratio of the peak height of the quantitation transition to the peak height of the confirmation transition was within 20% of the average ratio in calibration standards.
Analytes were quantitated using a seven-point calibration curve, ranging from 0.10 to 100 ng ml−1 for MEL, AMN and AMD, and 1.0 to 1000 ng ml−1 for CYA. A 1/x weighted linear curve was used to plot (performance standard corrected) analyte response versus concentration. Native CYA, AMN, AMD and MEL were quantitated against their corresponding 13C-labelled recovery standards which were quantitated against their corresponding 15N,13C-labelled performance standards. The absolute instrument detection limit (S/N = 3) was approximately 0.5 pg per injection for MEL, AMN and AMD, and about 3 pg per injection for CYA. However, trace amounts of the analytes were frequently detected in reagent blanks. As a result, the ability of the method to reliably quantitate the analytes was more a function of blank cleanliness than instrument sensitivity. The method detection limits for each compound, calculated as the average concentration in reagent blanks (n = 12) plus three times the standard deviation, are presented in . Concentrations in samples were blank and recovery corrected by subtracting the concentration of the analyte in the associated reagent blank and dividing by the percentage of the 13C-labelled internal recovery standard present in each sample.
Table 2. Method detection limits, analyte detection rates in infant formula samples, and mean and maximum analyte concentrations (blank- and recovery- corrected) in positive samples.
Quality control
The accuracy and precision of the method were examined by fortifying three different infant formula products with different concentrations of the analytes and analysing the fortified samples. Two powdered and one liquid infant formula samples were fortified with MEL, AMN and AMD at 0.01, 0.05, and 0.25 mg kg−1; CYA was fortified at 0.05, 0.25 and 0.5 mg kg−1 (n = 5 replicates).
A combination of blanks, replicate measurements and quality control (QC) samples were used to monitor the performance of the method during sample analysis. Reagent blanks consisting of Milli-Q water were extracted and processed with every sample batch to monitor background concentrations of the analytes. Solvent blanks of 90:10 (v/v) ACN–water were run throughout the instrumental analysis to monitor for sample carryover between runs. A randomly chosen sample in each batch was run in duplicate. Two infant formula samples, one liquid and one powdered, were used as in-house reference materials; at least one was run in each sample batch. These samples had been previously analysed using the earlier MEL-only method (Tittlemier et al. Citation2009) and were known to contain approximately 0.025 mg kg−1 MEL.
Results and discussion
Method development and performance
The original acidic aqueous extraction (Tittlemier et al. Citation2009) resulted in poor recoveries of AMN, AMD and CYA. Expansion of the method to include these analytes required the use of a less specific extraction solvent, and a 1:1 ACN–water extraction has been used successfully by other laboratories (Filigenzi et al. Citation2007; Smoker and Krynitsky Citation2008). The DCM wash step (Andersen et al. Citation2008) was retained, as it reduced matrix effects without affecting analyte recoveries.
The clean-up procedure used by Smoker and Krynitsky (Citation2008) for MEL and CYA was modified slightly to increase recoveries of AMN and AMD, which do not elute from the MCX SPE cartridges with a basic ACN solution. Changing the elution solution to 5% NH4OH in MeOH resulted in nearly quantitative recovery of AMN and AMD from the MCX cartridges. In addition, the incorporation of AMN and AMD into the method required a considerable reduction in sample size. Recovery of AMN and AMD from the MCX SPE cartridges was very low unless the applied aliquot contained 0.05 g or less of infant formula. MEL and CYA appear to be relatively immune to these SPE elution and sample size effects, perhaps because MEL is purely basic and CYA is purely acidic. In contrast, AMN and AMD are amphoteric, and are retained by both MAX and MCX SPE cartridges (approximately 20% recovery of AMN and approximately 30% recovery of AMD from MAX). It is possible that these two analytes compete with matrix components for active sites on the SPE cartridges.
MCX and MAX SPE fractions were analysed separately to minimize interferences and to determine the fraction of each analyte eluting from the two SPE cartridges. However, there is sufficient chromatographic separation between CYA and the other analytes for the MS to be switched from ES− mode to ES+ mode during the run. The fractions can therefore be combined before evaporation and run as a single sample.
Absolute response of the performance standards in the infant formula samples varied considerably (17–96% for 15N13C-CYA; 28–91% for 15N13C-MEL), indicating significant matrix effects in some samples. The degree of ion suppression tended to be greatest in milk-based powders, particularly those with a first listed (non-water) ingredient of ‘partially hydrolysed reduced minerals whey protein concentrate’. Diluting the sample generally reduced the degree of ion suppression, but also adversely affected analyte detection limits. In some cases, halving the sample size doubled the performance standard response, but for most samples in which this effect was evaluated, the decrease in sensitivity from sample dilution was not completely offset by the increase in response from reduced ion suppression.
Method performance was evaluated by the analysis of fortified samples, and these results are presented in . In general, method accuracy and precision were very good, with recoveries (relative to 13C internal standards) greater than 80% and relative standard deviations (RSD) less than 10%. The only exception to this was CYA at the lowest fortification level (0.05 mg kg−1), where recoveries were more variable (11–32% RSD). This may be more related to the infant formula samples chosen for method evaluation. The sample that exhibited the highest variability (Powder B in ) also had appreciable pre-fortification levels of CYA (approximately 0.05 mg kg−1), and correcting for this relatively high background concentration likely introduced additional error into the result.
Table 3. Average recoveries (relative to 13C internal standards) of analytes ± standard deviation (%, n = 5) from fortified infant formula.
Many of the randomly chosen duplicate samples did not contain appreciable quantities of the analytes. Duplicate samples that had >0.01 mg kg−1 MEL and/or CYA (AMN and AMD were generally lower than 0.005 mg kg−1) varied in the range 1.2% to 7.6% for MEL and by 0.1% to 22% for CYA. MEL variability in the two in-house reference materials was similar (4% and 6%). Only one of the in-house reference materials had appreciable levels of CYA; results from replicate analyses exhibited variability of 18%.
Results of infant formula analysis
MEL results for this study agreed very well with those of Tittlemier et al. (Citation2009); in most cases, differences were <10%, with greater differences in samples that were <0.01 mg kg−1. This demonstrates that expansion of the method to include the other analytes did not sacrifice MEL sensitivity. It further suggests that MEL is stable at room temperature for long time periods (>1 year).
MEL and CYA were detected in almost all infant formula samples (). Concentrations were generally low: the majority of samples had total melamine-related compounds (sum of all four analytes) of <0.05 mg kg−1. Concentrations of AMN and AMD were always very low (<0.02 mg kg−1). In all samples, the sum of melamine-related compounds was below the interim standard of 0.5 mg kg−1 set by Health Canada (Citation2008a) for infant formula products. The highest concentrations observed were 0.32 mg kg−1 MEL in one sample and 0.45 mg kg−1 CYA in a different sample.
The majority of samples analysed had higher concentrations of CYA than any other analyte. Samples that were relatively high in CYA tended to be low in MEL, and vice versa: only one sample had relatively high concentrations (>0.1 mg kg−1) of both compounds. Analyte ratios were generally very different from those found in adulterated Chinese milk (Wu et al. Citation2009), suggesting that the melamine-related compounds came from a different source. MEL and CYA are very common industrial chemicals, and may be present at low concentrations in foods as a result of legitimate uses of products that contain or degrade to MEL or CYA (WHO Citation2009). For example, trichloromelamine, which degrades to MEL, is a component of solutions used to sanitize food processing equipment and other food contact articles (WHO Citation2009). Chlorinated isocyanurates, used in dishwashing detergents, sanitizing solutions and drinking water treatment products (Occidental Chemical Corporation Citation2010), hydrolyse to form CYA.
Soy-based infant formulas tended to be lower in CYA than milk-based formulas. However, a soy-based brand had the highest MEL concentrations of all analysed samples (approximately 0.3 mg kg−1). Because this brand was certified Pareve (meat and dairy free), it appears unlikely that the MEL had a milk-based source. Similarly, degradation of the pesticide cyromazine is unlikely to be a source of MEL for a certified organic product. In Canada, cyromazine is approved for use on many leafy green vegetables, celery and mushrooms (Health Canada Citation2010), which are not typical ingredients of infant formula. MEL has been shown to migrate from melamine-formaldehyde plastic tableware (Bradley et al. Citation2005; Lund and Petersen Citation2006), but these articles are generally used for occasional food contact rather than long-term storage. MEL was not detected in extracts of the interior surfaces of infant formula containers (Tittlemier et al. Citation2009). MEL has also been found in non-dairy protein and in ammonium bicarbonate (Gossner et al. Citation2009), but the origin of the relatively low MEL and CYA concentrations observed in the infant formula samples analysed for this study is unknown. Triazine-based cleansers or disinfectants, used on food contact surfaces during food preparation, are a potential but unconfirmed source.
In general, liquid formulas were lower in MEL and CYA: the highest concentrations were always observed in powdered formula. Much of the difference can be attributed to a sample dilution effect: the powdered samples were not diluted according to the label directions, and were analysed as received. Correcting the results for sample type (using dilution factors of 2 for concentrated liquids and 7 for powdered formula) did not reveal any discernible trend for MEL, but the ten products that were highest in CYA were all milk-based powders. Calculations of chemical exposure from food consumption would take formula dilution into account, but this study focuses on concentration trends in infant formula rather than determining population exposure.
References
- Andersen , WC , Turnipseed , SB , Karbiwnyk , CM , Clark , SB , Madson , MR , Gieseker , CM , Miller , RA , Rummel , NG and Reimschuessel , R . 2008 . Determination and confirmation of melamine residues in catfish, trout, tilapia, salmon, and shrimp by liquid chromatography with tandem mass spectrometry . J Agric Food Chem , 56 : 4340 – 4347 .
- Bradley , EL , Boughtflower , V , Smith , TL , Speck , DR and Castle , L . 2005 . Survey of the migration of melamine and formaldehyde from melamine food contact articles available on the UK market . Food Addit Contam A , 22 : 597 – 606 .
- Canadian Food Inspection Agency (CFIA). 2009. Report on testing results for melamine in foods containing milk and milk-derived ingredients from China [Internet]. Ottawa (ON): Canadian Food Inspection Agency; [cited 2010 Jun 16]. Available from: http://www.inspection.gc.ca/english/fssa/concen/2008melinfoe.shtml/
- Dobson , RLM , Motlagh , S , Quijano , M , Cambron , RT , Baker , TR , Pullen , AM , Regg , BT , Bigalow-Kern , AS , Vennard , T Fix , A . 2008 . Identification and characterization of toxicity of contaminants in pet food leading to an outbreak of renal toxicity in cats and dogs . Toxicol Sci , 106 : 251 – 262 .
- Ehling , S , Tefera , S and Ho , IP . 2007 . High-performance liquid chromatographic method for the simultaneous detection of the adulteration of cereal flours with melamine and related triazine by-products ammeline, ammelide, and cyanuric acid . Food Addit Contam A , 24 : 1319 – 1325 .
- Filigenzi , MS , Tor , ER , Poppenga , RH , Aston , LA and Puschner , B . 2007 . The determination of melamine in muscle tissue by liquid chromatography/tandem mass spectrometry . Rapid Commun Mass Spectrom , 21 : 4027 – 4032 .
- Food Standards Australia New Zealand (FSANZ). 2008. Melamine in foods from China [Internet]. Canberra (Australia): FSANZ; [cited 2010 Jun 16]. Available from: http://www.foodstandards.gov.au/scienceandeducation/factsheets/factsheets2008/melamineinfoodsfromchina/
- Gossner , CM-E , Schlundt , J , Ben Embarek , P , Hird , S , Lo-Fo-Wong , D , Beltran , JJO , Teoh , KN and Tritscher , A . 2009 . The melamine incident: implications for international food and feed safety . Environ Health Perspect , 117 : 1803 – 1808 .
- Health Canada. 2008a. Health Canada has re-assessed its maximum tolerable limit for melamine in infant formula [Internet]. Ottawa (ON): Health Canada; [cited 2010 Jun 16]. Available from: http://www.hc-sc.gc.ca/fn-an/securit/chem-chim/melamine/index-eng.php/
- Health Canada. 2008b. Survey and health risk assessment of background levels of melamine in infant formula allowed for sale in Canada [Internet]. Ottawa (ON): Health Canada; [cited 2010 Jun 16]. Available from: http://www.hc-sc.gc.ca/fn-an/pubs/melamine_survey-enquete_hra-ers-eng.php/
- Health Canada. 2010. List of maximum residue limits regulated under the Pest Control Products Act [Internet]. Ottawa (ON): Health Canada; [cited 2010 Jul 29]. Available from: http://www.hc-sc.gc.ca/cps-spc/alt_formats/pdf/pest/part/protect-proteger/food-nourriture/mrls-lmr-eng.pdf/
- Lund , KH and Petersen , JH . 2006 . Migration of formaldehyde and melamine monomers from kitchen- and tableware made of melamine plastic . Food Addit Contam A , 23 : 948 – 955 .
- Occidental Chemical Corporation. 2010. Detergent, bleach, cleaner and sanitizer applications handbook [Internet]. Dallas (TX) USA: Occidental Chemical Corporation; [cited 2010 Jul 21]. Available from: http://www.oxy.com/Our_Businesses/chemicals/Documents/isocyanurates/ACLHB%5B1%5D.pdf/
- Puschner , B , Poppenga , RH , Lowenstine , LJ , Filigenzi , MS and Pesavento , PA . 2007 . Assessment of melamine and cyanuric acid toxicity in cats . J Vet Diagn Invest , 19 : 616 – 624 .
- Smoker M, Krynitsky AJ. 2008. Interim method for determination of melamine and cyanuric acid residues in foods using LC-MS/MS: Version 1.0. Washington (DC): US Food and Drug Administration. Library Information Bulletin No. 4422
- Tittlemier , SA , Lau , BPY , Ménard , C , Corrigan , C , Sparling , M , Gaertner , D , Pepper , K and Feeley , M . 2009 . Melamine in infant formula sold in Canada: occurrence and risk assessment . J Agric Food Chem , 57 : 5340 – 5344 .
- US Food and Drug Administration (USFDA). 2009. Melamine contamination in China [Internet]. Washington (DC): USFDA; [cited 2010 Jun 16]. Available from: http://www.fda.gov/NewsEvents/PublicHealthFocus/ucm179005.htm/
- World Health Organization (WHO) . 2009 . Toxicological and health aspects of melamine and cyanuric acid , Geneva (Switzerland) : WHO .
- Wu , Y-N , Zhao , Y-F and Li , J-G . 2009 . A survey on occurrence of melamine and its analogues in tainted infant formula in China . Biomed Environ Sci , 22 : 95 – 99 .
- Yokley , RA , Mayer , LC , Rezaaiyan , R , Manuli , ME and Cheung , MW . 2000 . Analytical method for the determination of cyromazine and melamine residues in soil using LC-UV and GC-MSD . J Agric Food Chem , 48 : 3352 – 3358 .