ABSTRACT
Immunoaffinity columns (IACs) are a well-established tool in the determination of regulated mycotoxins in food and feed commodities. However, they also have the potential to become attractive pre-concentration and clean-up materials for the determination of masked (also called modified) mycotoxins, which have been recognised as important contributors to the toxicological hazard deriving from fungal spoilage of goods. However, the information available in the literature concerning the cross-reactivity of DON-IACs against the major conjugates (DON-3-G, 15-AcDON and 3-AcDON) is incomplete and often contradictory. We have carried out a detailed characterisation of the cross-reactivity of the four main IACs brands against DON and its conjugates as well as an assessment of the competition among the analytes. Only one IAC enabled the simultaneous analysis of all relevant DON forms while two missed 15-AcDON and the fourth one missed DON-3-G and 3-AcDON. In the case of the multivalent IAC, the analytes modified at the C-3 position compete for the antibody binding with preference for 3-AcDON (less spatially hindered) while DON-3-G has the more-hindered access to the active sites. Taking into consideration the levels of DON conjugates existing in real samples, the cross-reactivity of one DON-IAC allows a quantitative analysis of all of these analytes. Important but rather neglected aspects such as the continuous supply of IACs with identical characteristics, and of columns which are strictly blank, are also addressed in this paper.
GRAPHICAL ABSTRACT
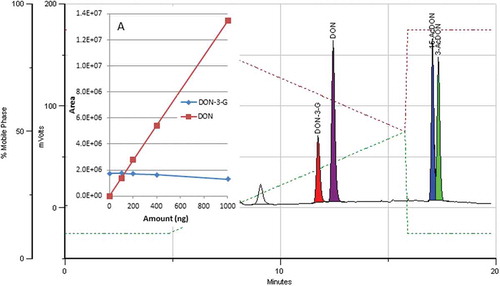
Introduction
The need to include masked mycotoxins in food safety assessments is increasingly under the attention of the scientific community and regulators (Berthiller et al. Citation2013; Guo et al. Citation2014).
Deoxynivalenol (DON) is the most frequently detected trichothecene in cereals and the one measured in highest amounts (EFSA Citation2013; Suman et al. Citation2013). Its acetylated derivatives, 3-acetyl-DON (3-AcDON) and 15-acetyl-DON (15-AcDON), are intermediate fungal metabolites in the biosynthesis of DON and have been found to occur concomitantly with DON in food/feed commodities (Berthiller et al. Citation2013; EFSA Citation2013; Habler & Rychlik Citation2016). Another pathway leading to masked DON is its conjugation with a glucose moiety during phase II metabolism, activated by the defence mechanism of the infected plants, giving rise to deoxynivalenol-3-glucoside (DON-3-G) (Desmarchelier & Seefelder Citation2011; Goryacheva & Saeger Citation2012).
It is generally accepted that the hydrolysis of the mentioned metabolites and conjugates in the digestive tract of animals consuming contaminated food might release the toxic precursor (DON), at least partially (Berthiller et al. Citation2011, Citation2013; Desmarchelier & Seefelder Citation2011; Boevre et al. Citation2012; EFSA Citation2013; Broekaert et al. Citation2014; Rychlik et al. Citation2014). Failure to detect these forms of DON can lead to significant underestimation of the toxic potential of a particular sample (Vendl et al. Citation2009).
The joint FAO/WHO expert committee on food additives considered the toxicity of the acetylated derivatives equal to that of DON (Codex Alimentarius Commission Citation2011) while Pinton et al. (Citation2012) further suggested that 15-AcDON displays even higher toxicity. The committee could not gather sufficient evidence to include DON-3-G in the group provisional maximum tolerable daily intake (PMTDI) due to a lack of toxicological data.
A study covering 12 European countries showed that 20% of the samples were positive for 15-AcDON while 8% contained 3-AcDON (European Commission Citation2003). On average, DON-3-G amounted for about 20% of DON but this figure may vary depending on the crop year and genotype of the crop. According to a survey carried out by the European Food Safety Authority (EFSA), the average concentrations of 3-AcDON and 15-AcDON in unprocessed grains of undefined end use were 12.8 and 48.5 μg kg−1, respectively. In foods, 3-AcDON and 15-Ac-DON were quantified in < 5% of the samples. Results on the occurrence of DON-3-G were scarce (EFSA Citation2013).
Immunoaffinity columns (IACs) are a popular tool in sample clean-up and enrichment prior to the determination of mycotoxins by high performance-liquid chromatography (HPLC) coupled to UV-vis or fluorescence detection (FLD) (Krska et al. Citation2008). According to Cirlini et al. (Citation2012) the cross-reactivity of IACs is a potentially interesting feature to widen the scope of IAC-based methods in terms of number of analytes. Their application to the determination of masked mycotoxins can not only allow more meaningful food safety assessments but also render mycotoxin monitoring more cost effective. Also, Guo et al. (Citation2014) stressed the need to develop generic or broad-specific monoclonal antibodies with similar affinity for DON and its analogues of interest so as to rapidly and concomitantly determine the sum of parent and masked mycotoxins. The authors propose the use of hapten heterology for hybridoma screening in order to produce such antibodies.
The results found in the literature concerning the ability of DON IACs to bind all relevant forms of DON (DON, DON-3-G, 3- and 15-AcDON) are incomplete and somewhat contradictory. Veršilovskis et al. (Citation2011) found that among the IACs tested, one product was only useful for analysing DON, while the remaining IACs cross-reacted with the other conjugated forms to a variable extent. DONPrep, Neocolum for DON and DZT MSPrep provided quantitative recovery of DON while in the best cases recoveries did not exceed 58% for DON-3-G, 54% for 3-AcDON and 13% for 15-AcDON. Suman et al. (Citation2013) exploited the cross-reactivity of the Neocolumn for DON to analyse DON-3-G in bread and mini cake obtaining recoveries between 85% and 95%. Kostelanska et al. (Citation2011) reported cross-reactivity of DONPrep for DON-3-G, 3- and 15-AcDON in the analysis of beer and intermediate brewing products but AcDONs were not chromatographically separated. Vendl et al. (Citation2009) found no cross-reactivity of DONPrep and DONTest for DON-3-G while the former IAC could still recover 3-AcDON (105%). 15-AcDON was not included in the assay. In general, the antibodies incorporated in the tested IACs were unable to retain simultaneously all four substances of interest. Guo et al. (Citation2014) attempted to produce multivalent antibodies for DON analogues but they did not demonstrate the cross-reactivity to DON-3-G.
The aim of the present work was to clarify the cross-reactivity of DON dedicated IACs supplied by the four main producers to selectively retain the DON conjugates. As the determination of all masked forms of DON in cereals and cereal products is becoming a legislative priority, we have fully assessed the quantitative features of IACs for DON and respective conjugates leading to interesting results. Aspects such as competition during sample clean-up, target-analyte free immunoaffinity columns, and continued supply of equivalent IACs will be addressed.
Materials and Methods
Materials and reagents
Ultrapure water used for extraction of samples, preparation of reagents and as HPLC mobile phase was Milli-Q grade produced by a Millipore system (Merck Millipore, Darmstadt, Germany). Acetonitrile HiPerSolv Chromanorm LC-MS grade was from VWR International (Leuven, Belgium). Reagents for the post-column derivatisation system were NaOH from Merck KGaA (Darmstadt, Germany), ammonium acetate ACS reag Ph Eur, EMSURE, from Merck and methyl acetoacetate > 99% from Acros Organics (Geel, Belgium).
Analytical standards of DON, DON-3-G, 3-AcDON and 15-AcDON were all Biopure solutions (in acetonitrile) supplied by Romer Labs Diagnostic GmbH (Tulln, Austria), except 15-AcDON which was purchased as the solid (98.8% purity).
Four brands of IACs were compared regarding their cross-reactivity and extraction efficiency: DONTest WB from Vicam, lot nr. 238 (Milford, MS, USA); Neocolumn for DON from Neogen Europe Ltd, batch nr. D1010813 (Auchincruive, UK); DONPrep from R-Biopharm Rhone Ltd, batch nr. Al922/50 (Glasgow, UK) and Immunoclean CF DON from Aokin (Berlin, Germany). Sample extracts were purified by IAC clean-up on a 12-port vacuum manifold from Alltech (Thermo Fisher Scientific Inc., Waltham, MA, USA). IAC columns were kept in the fridge at 4°C and allowed to reach room temperature before use.
Sample preparation and IAC protocol
The cross-reactivity and competition experiments were carried out with individual and mixed standard solutions in Milli-Q water, as the concerned analytes can be extracted from cereals with pure water. A total of 10 ml of standard solutions or 2 ml sample extract diluted to 10 ml with water were percolated drop-wise through the IAC columns. The IACs were desorbed by the ‘hot water elution’ technique (Stroka & Seidler Citation2014) and analysed in-line with HPLC-post-column derivatisation-fluorescence detection (HPLC-PCD-FLD). Briefly, 1.5 ml of Milli-Q water was added to the IAC followed by heating in a water bath at 85°C for 5 min, to disrupt the affinity of the antibodies to the mycotoxins. The analytes were immediately transferred to the injection loop (2.1 ml volume) by means of positive pressure on the IAC syringe body and flushed with an additional 400 µl of water. The chromatographic analysis was then started. The temperature and length of time employed in hot water elution were previously optimised to ensure quantitative elution and preserve the analytes from degradation, however further details on this matter is out of the scope of the present paper.
To demonstrate the applicability of the cross-reactivity results, cereal samples from representative matrices (barley, wheat and maize) known to contain various levels of DON were analysed. Test portions of these samples (20 g) were extracted with Milli-Q water (100 ml) and purified using DONTest IAC.
Post-column derivatisation and chromatographic analysis
The chromatographic system was composed by two pumps: model 307 (mobile phase A – Milli-Q Water) and model 306 (mobile phase B – acetonitrile) from Gilson (Villiers Le Bel, France). Mobile phases were on-line degassed by means of an ERC 3215 degasser (ERC Inc. Tokyo, Japan) and mixed in a model 811D dynamic mixer also from Gilson. The mixture was delivered to a Rheodyne injector model 7010 (Rheodyne, Rohnert Park, CA, USA) mounted with a 2.1 ml loop (installed for inline IAC elution) and then to the analytical column Zorbax SB C18 (25 cm × 4.6 mm i.d., 5 µm particle size) from Agilent (Palo Alto, CA, USA). The column was housed in the oven compartment of the post-column derivatiser Pickering PCX 5200 (Pickering Laboratories, Mountain View, CA, USA) and kept at 35°C. The derivatisation reagents: NaOH 0.3 M solution (reagent 1) and ammonium acetate 2 M + methyl acetoacetate 0.05 M (reagent 2) were delivered at 0.5 ml min−1, mixed sequentially with the column effluent and infused through a reaction loop at 120°C. Under the above conditions, DON and its modified forms undergo decomposition to formaldeyde in alkaline media (reagent 1), then formaldehyde condenses with acetoacetate, in the presence of ammonium acetate (reagent 2), to form dihydropyridine according to the Hantzsch reaction (Hantzsch Citation1881). Dihydropyridine was quantified by a scanning fluorescence detector model 474 from Waters (Milford, MA, USA). The detector was operated at 370 nm excitation and 470 nm emission wavelengths. The detector signal was converted to digital by a Gilson 506 C interface. Chromatographic resolution of the analytes was achieved with the following mobile phase gradient: 0–5 min, 10% mobile phase B; 5–16 min, gradient to 50% mobile phase B; 16.1 min, return to 10% mobile phase B and equilibration of the column until 20 min. The dead volume time of the system was approximately 4 min at a mobile phase flow rate of 1 ml min−1. System control and data acquisition was performed by a personal computer running the Unipoint LC, ver. 5.11 software from Gilson.
Results and discussion
Cross-reactivity experiments
Cross-reactivity of DON IACs to DON-3-G and AcDONs is the first critical issue to be considered when developing an efficient extraction/clean-up IAC method for the analysis of DON and conjugates, as no dedicated IACs are available for the conjugated forms (Veršilovskis et al. Citation2011; Goryacheva & Saeger Citation2012). The data available in the literature suggest that the performance of IACs from different suppliers differ considerably regarding the modified forms of DON, which is understandable as each producer uses a proprietary method for raising the antibodies that are incorporated in the IACs (Veršilovskis et al. Citation2011; Goryacheva & Saeger Citation2012; Guo et al. Citation2014). We have compared IA columns from four main suppliers at three concentration levels of the analytes. The results can be found in . The first observation to highlight is the fact that under the conditions tested here only DONTest IAC succeeded in retaining DON and the three conjugates with good recoveries. A representative chromatogram at 200 ng of each analyte on column, equivalent to a level of 100 µg kg−1 DON in a sample can be found in .
Figure 1. (colour online) Recoveries obtained with IACs columns marketed by the main suppliers tested at three loading levels (a- 40; b- 200; and c- 400/1000 ng). Error bars represent the result of duplicates. DONaokin was just tested once at each level.
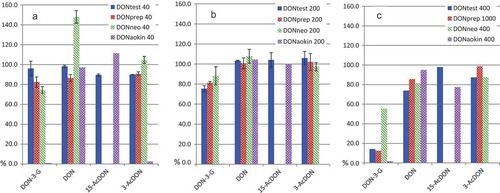
Figure 2. (colour online) Chromatogram of a mixture of DON and conjugates. The IAC column was loaded with 10 ml of an extract obtained from a sample containing 100 µg kg−1 each (corresponding to 200 ng each analyte).
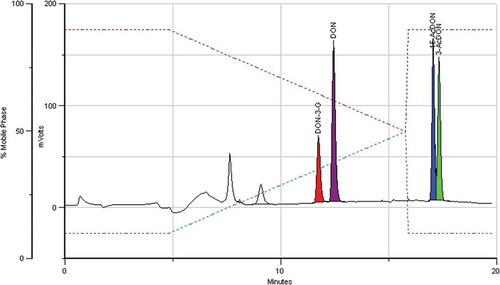
The DONPrep and DONneo columns were unable to retain 15-AcDON while the DONAokin displayed a dissimilar cross-reactivity pattern leading to the loss of DON-3-G and 3-AcDON.
At a column load of 40 ng of each compound, almost quantitative recoveries were obtained with all the columns for the analytes with which they cross-reacted. With increasing loading amounts, the recoveries of DON-3-G worsened considerably; the IAC Neocolumn for DON was the least affected. On the other hand, this IAC showed a rather high recovery of DON of 148% at 40 ng level which was due to the presence of traces of DON in one batch of columns, as delivered by the supplier. The amount of DON was estimated to be about 15 ng when eluting the column with pure methanol, and its identity was confirmed by LC-MS/MS. Such amount caused only a significant bias in the results for the lowest concentration (equivalent to 20 µg kg−1), which is far below any legislated limit for DON in food (European Commission Citation2006). It must be highlighted that the set-up we have chosen in the above assays was the complete (in-line) injection of the IAC eluate; an uncommon procedure in routine analysis. Therefore, the impact of such DON traces using the operational conditions recommended by the supplier are likely to be insignificant for routine analysis.
The apparent absence of retention capability of the Neocolumn and DONPrep IACs for 15-AcDON was further investigated by collecting the percolated solution and applying it to a DONTest IAC, which was shown to retain 15-AcDON. This set-up (tandem) allowed demonstrating the presence/lack of cross reactivity of the immobilised antibodies towards 15-AcDON, excluding other potential reasons for not finding 15-AcDON e.g. ineffective elution. In both cases, 15-AcDON leaked from the first column and was collected quantitatively in the second column. The results above suggest that the antibodies immobilised in Neocolumn for DON and DONPrep IACs only cross-react with DON congeners not altered in the C15 position (DON, DON-3-G and 3-AcDON) while, apparently, the C15 OH group is not the exclusive or main epitope for DONTest antibodies (broader selectivity) (Goryacheva & Saeger Citation2012). The same tandem approach was performed with the DONAokin column where the effluent from that IAC was percolated through a DONTest column. Once again, the analytes that were not collected in the first IAC (DON-3-G and 3-AcDON) were collected quantitatively in the second column. DONAokin antibodies only cross-react with DON congeners not substituted in the C3 position. The strategy for immunogen synthesis leading to cross-reacting antibodies (or not) to the masked compounds was described by Goryacheva and Saeger (Citation2012).
Our results are partially in agreement with Veršilovskis et al. (Citation2011), who also found that 15-AcDON was the least recovered among three IA columns, but showing DONaok had the reverse behaviour, as far as the AcDONs are concerned. The recovery values of 15-AcDON of around 10% can be explained by unspecific binding or change of antibody properties by the supplier throughout time. A dissimilar scenario was described by Vendl et al. (Citation2009) where none of the IACs DONPrep and DONTest could retain DON-3-G while DONPrep still cross-reacted with 3-AcDON. As explained by Guo et al. (Citation2014) most of the available monoclonal antibodies have much higher affinity either to 3-AcDON or to 15-AcDON, because they were produced using DON-immunogens containing the ester linkage at the C-3 or C-15 positions which mimics the acetyl groups of 3-AcDON and 15-AcDON, respectively. Antibodies cross-reacting with 3-AcDON are relatively more common, often with even higher affinity than for DON (Baumgartner et al. Citation2010).
Lattanzio et al. (Citation2016) evaluated the cross-reactivity of a lateral flow immunoassay for DON against the three conjugates and the ratio of false-positives caused by their co-occurrence in the samples. The immunoassay generated a strong response for 3-AcDON and DON-3-G leading to 100% and 70% of false-positives, respectively. Nevertheless, the authors concluded that at the levels conjugates are normally found in the samples the reliability of the assay is not significantly impaired.
The hypothesis mentioned by Senyuva and Gilbert (Citation2010) that a declared cross-reactivity of one IAC brand to 3-AcDON and 15-AcDON is likely to be true for other products could not be confirmed for the columns tested in our experiments.
As the DONTest IAC was the most promising for the simultaneous analysis of DON and major conjugates, further competition assays were performed with just this one IAC.
Competition for binding of DON and conjugates
During the assessment of linearity, recovery and working range, good linearity was obtained for DON and 15-AcDON in the range from 20 to 1000 ng (10–500 µg kg−1). However, above 400 ng of 3-AcDON and above 100 ng of DON-3-G deviation from linearity was noticed (). An indication of this behaviour could already be observed in showing that recoveries were greatly reduced at higher concentration levels. This effect can be explained by the apparent competition between DON and conjugates for the active sites on the IAC antibodies.
Figure 3. (colour online) Calibration curves obtained with IAC enrichment/clean-up followed by HPLC-PCD-FLD of aqueous solutions containing analyte amounts in the range of 20 to 1000 ng (10 to 500 µg kg−1).
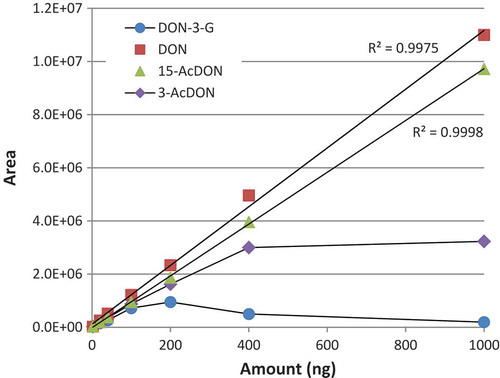
As mentioned by Senyuva and Gilbert (Citation2010), and confirmed by the supplier, the DONTest IAC capacity is 1250 ng of DON. The observed competition to the detriment of conjugated forms was noticed for DON-3-G in particular even below the nominal column capacity. Test results using binary mixtures of analytes with a constant amount of DON-3-G (200 ng) are shown in . A 5% reduced binding capacity of DON-3-G at 400 ng of DON and 24.5% at 1000 ng of DON () was seen, while no relevant competition was observed between DON-3-G and 15-AcDON (). An even more pronounced effect was observed for DON-3-G when applied together with 3-AcDON (). Above 100 ng of 3-AcDON the binding capacity of DON-3-G was greatly compromised. 3-AcDON also reached quantitative retention capacity at a loading of 400 ng. Again, in both cases, response inflexion for DON-3-G occurred far below the stated capacity of the IAC to bind DON. It can be postulated that the DONTest antibodies can bind at least two distinct epitopes, one of which is compatible with 15-AcDON, and another with DON-3-G and 3-AcDON, whereas DON is bound via all the epitopes. Competition is stronger between compounds modified on the same OH group while DON is less spatially hindered, thus can easily access various binding sites.
Figure 4. (colour online) Influence of increasing concentrations of DON (a), 15-AcDON (b) and 3-AcDON (c) on the response of DON-3-G (100 µg kg−1, 200 ng) when loaded onto an IAC with a stated capacity of 1250 ng for DON.
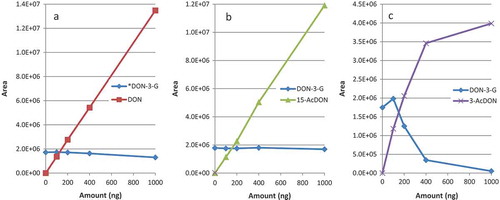
To clarify this issue further, an experiment with three DONTest IACs in sequence (stacked) was performed. The percolate of IAC I was forced to percolate IAC II and so forth, ensuring that the analytes were retained along the chain according to their avidity. As it can be seen in , when loading the IACs with 400 ng of all analytes, DON and 15-AcDON are quantitatively retained in the first column (IAC I) while 12% of 3-AcDON is still found in IAC II. Only a minor fraction of DON-3-G was collected in IAC I while about 50% was retained in IAC II and an additional 27% was found in IAC III. According to these results, the affinity of the antibody to the analytes can be ranked in the following manner: DON and 15-AcDON > 3-AcDON > DON-3-G.
Table 1. Amount of DON and conjugates retained in three IAC columns (I–III) in a stacked experiment to demonstrate competition between analytes for the antibodies in the IAC. Recoveries are also given.
A simple capacity limit explanation seems insufficient to explain the observed behaviour, as both IAC I and IAC II capacity limits were not reached and still a significant fraction of analytes leaked to IAC III. More evidence of the weaker avidity of the IAC for DON-3-G was obtained by performing a calibration just with this analyte (0–1000 ng) (). The linear range was extended but did not reach 400 ng although this value is far below the IAC capacity limit. At a load of 1000 ng about 52% of DON-3-G was not retained by the antibodies. As the amount of conjugates in real samples is generally far below DON, competition effects are minimised to levels that ensure quantitative collection of the masked forms (Lattanzio et al. Citation2016).
Figure 5. (colour online) Plot of the response obtained for increasing loading amounts of DON-3-G, in the range from 20 to 1000 ng in MilliQ water (equivalent to 10 to 500 µg kg−1).
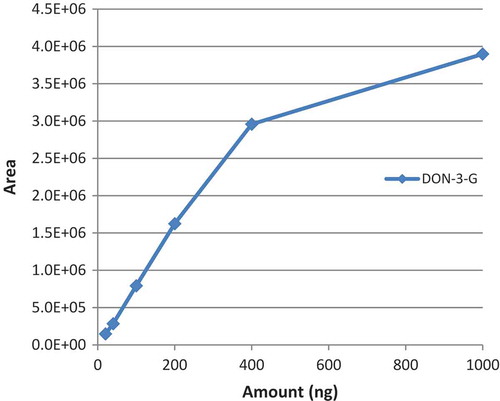
shows a IAC-HPLC-PCD-FLD chromatogram of a naturally contaminated maize sample containing all the investigated conjugates. Failure to determine 15-AcDON would result in an underestimation of ‘total DON’ content as this compound was found in four out of five maize samples and two out of five barley samples, and more frequently than 3-AcDON.
Technical challenges and findings
To make use of IACs’ cross-reactivity for method development aiming at the analysis of a family of mycotoxins, the continuous production of antibodies with the same characteristics is a basic condition, as the performance of the IAC is usually just referred to the leader analyte. During our study a manufacturer changed the antibodies incorporated in the IAC initially used in this study. The reason for this change was to achieve an improved behaviour of the column with respect to some features not relevant for this study. This, however, in return resulted in a loss of cross-reactivity to 15-AcDON using our proposed procedure. This was the only column tested that had sufficient cross-reactivity to all four investigated analytes. The manufacturer offered kindly to supply us with IACs of the old antibody type, which allowed us to complete the study.
At the moment of drafting this article the authors are aware that the IAC with the properties described here is not anymore available as a new antibody is used for this product. Our results remain however insofar of interest as new IAC products, with features demonstrated useful in this study, may become available again.
The anti-DON antibodies (Aokin) used as recognition element in the IBIS iSPR multiplex microarray sensor (IBIS Technologies, Hengelo, The Netherlands) were able to detect all DON forms herein discussed, so such antibodies could be used for an IAC with a wide cross-reactivity (Goryacheva & Saeger Citation2012).
Another aspect that we discovered in this study was that one batch of IACs contained from the outset DON at levels of around 15 ng per IAC. Traces of deepoxydeoxynivalenol (DOM – about 0.22 ng) were also found in these columns which, however, is not expected to be present in cereal samples. The identities of both analytes were confirmed by LC-MS/MS. presents the corresponding extracted ion chromatograms and respective MRM transitions. To what degree the level of DON can have a significant impact on false-positive test results when used according to the protocol recommended by the manufacturer was not investigated. However, when used according to our protocol (quantitative IAC eluate injection for chromatography) these traces had an impact due to the rather low LOD achieved. Thus, blank verification as part of quality control of IACs batches appears to be an important issue, despite the widespread use of monoclonal antibodies in current IAC columns for mycotoxin analysis.
Conclusions
The feasibility of using commercially available DON-targeted immunoaffinity columns for the determination of DON and major conjugates was investigated. The cross-reactivity of IACs against masked DON was deconvoluted through coupling to chromatographic analysis and fluorescence detection gaining on specificity and providing results for a family of DON analogues. Only DONTest IAC enabled the simultaneous analysis of DON, DON-3-G, 3- and 15-AcDON while two IACs missed 15-AcDON and another missed both DON-3-G and 3-AcDON. The competition between 3-AcDON and DON-3-G was the strongest as both molecules display a similar configuration which fits the same active site. Eventually, these dedicated sites are available in a reduced number, which restricts the take-up of all analytes by the DONTest IAC. A stacked experiment demonstrated that competition between DON analogues occurs at loading amounts below the nominal capacity for DON. The affinity of the antibody was ranked in the following order: DON = 15-AcDON > 3-AcDON > DON-3-G. As DON conjugates occur in cereal samples in limited amounts, the cross-reactivity of the IAC is still enough for the quantitative analysis of DON and its conjugates, becoming a valuable tool for evaluating the ‘total DON’ content. Potential overestimation of results arising from cross-reactivity, seen as a drawback of immunochemical methods, can instead be regarded as a useful feature relating to the global toxicological hazard of a given sample, once such cross-reactivity is fully characterised and assigned to DON conjugates.
Disclaimer
The reference to trade names or commercial products in this publication is solely for the purpose of providing factual information and does not imply recommendation or endorsement by the European Commission. The views expressed are purely those of the authors and may not in any circumstances be regarded as stating an official position of the European Commission.
Acknowledgements
Nancy Zabe (VICAM) is thanked for supplying additional DON IACs.
Disclosure statement
No potential conflict of interest was reported by the authors.
References
- Baumgartner S, Führer M, Krska R. 2010. Comparison of monoclonal antibody performance characteristics for the detection of two representatives of A- and B-trichothecenes: T-2 toxin and deoxynivalenol. World Mycotoxin J. 3:233–238.
- Berthiller F, Crews C, Dall’Asta C, Saeger SD, Haesaert G, Karlovsky P, Oswald IP, Seefelder W, Speijers G, Stroka J. 2013. Masked mycotoxins: a review. Mol Nutr Food Res. 57:165–186.
- Berthiller F, Krska R, Domig KJ, Kneifel W, Juge N, Schuhmacher R, Adam G. 2011. Hydrolytic fate of deoxynivalenol-3-glucoside during digestion. Toxicol Lett. 206:264–267.
- Boevre MD, Mavungu JDD, Maene P, Audenaert K, Deforce D, Haesaert G, Eeckhout M, Callebaut A, Berthiller F, Peteghem CV, De Saeger S. 2012. Development and validation of an LC-MS/MS method for the simultaneous determination of deoxynivalenol, zearalenone, T-2-toxin and some masked metabolites in different cereals and cereal-derived food. Food Addit Contam A. 29:819–835.
- Broekaert N, Devreese M, Mil TD, Fraeyman S, Baere SD, Saeger SD, Backer PD, Croubels S. 2014. Development and validation of an LC–MS/MS method for the toxicokinetic study of deoxynivalenol and its acetylated derivatives in chicken and pig plasma. J Chromatogr B. 971:43–51.
- Cirlini M, Dall’Asta C, Galaverna G. 2012. Hyphenated chromatographic techniques for structural characterization and determination of masked mycotoxins. J Chromatogr A. 1255:145–152.
- Codex Alimentarius Commission. 2011. Joint FAO/WHO food standards programme, codex committte on contaminants in foods, fifth session. Working document for information and use in discussions related to contaminants and toxins in the GSCTFF, CF/5 INF/1. The Hague: FAO/WHO.
- Desmarchelier A, Seefelder W. 2011. Survey of deoxynivalenol and deoxynivalenol-3-glucoside in cereal-based products by liquid chromatography electrospray ionization tandem mass spectrometry. World Mycotoxin J. 4:29–35.
- EFSA. 2013. European food safety authority scientific report: deoxynivalenol in food and feed: occurrence and exposure. EFSA J. 11:3379.
- European Commission. 2003. Collection of occurrence data of fusarium toxins in food and assessment of dietary intake by the population of EU Member States, Report on Tasks for Scientific Cooperation (SCOOP) 3.2.10 European Commission, 2003, Brussels. [accessed 2014 Mar 25]. Available from: http://ec.europa.eu/food/fs/scoop/task3210.pdf
- European Commission. 2006. Commission Regulation No 401/2006 of 23 February 2006, laying down the methods of sampling and analysis for the official control of the levels of mycotoxins in foodstuffs. Luxembourg: Official Journal of the European Union L 70/12.
- Goryacheva IY, Saeger SD. 2012. Immunochemical detection of masked mycotoxins: a short review. World Mycotoxin J. 5:281–287.
- Guo Y, Sanders M, Galvita A, Heyerick A, Deforce D, Bracke M, Eremin S, Saeger SD. 2014. Heterologous screening of hybridomas for the development of broad-specific monoclonal antibodies against deoxynivalenol and its analogues. World Mycotoxin J. 7:257–265.
- Habler K, Rychlik M. 2016. Multi-mycotoxin stable isotope dilution LC-MS/MS method for fusarium toxins in cereals. Anal Bioanal Chem. 408:307–317.
- Hantzsch A. 1881. Condensation produckte aus aldehyd ammoniak und ketonartigen verbindungen. Ber. 14:1637–1638.
- Kostelanska M, Zachariasova M, Lacina O, Fenclova M, Kollos A-L, Hajslova J. 2011. The study of deoxynivalenol and its masked metabolites fate during the brewing process realised by UPLC–TOFMS method. Food Chem. 126:1870–1876.
- Krska R, Schubert-Ullrich P, Molinelli A, Sulyok M, MacDonald S, Crews C. 2008. Mycotoxin analysis: an update. Food Addit Contam. 28:152–163.
- Lattanzio VMT, Ciasca B, Powers S, Holst C. 2016. Validation of screening methods according to regulation 519/2014/EU. Determination of deoxynivalenol in wheat by lateral flow immunoassay: A case study. Trends Anal Chem. 76:137–144.
- Pinton P, Tsybulskyy D, Lucioli J, Laffitte J, Callu P, Lyazhri F, Grosjean F, Bracarense AP, Kolf-Clauw M, Oswald IP. 2012. Toxicity of deoxynivalenol and its acetylated derivatives on the intestine: differential effects on morphology, barrier function, tight junction proteins, and mitogen-activated protein kinases. Toxicological Sci. 130:180–190.
- Rychlik M, Humpf H-U, Marko D, Dänicke S, Mally A, Berthiller F, Klaffke H, Lorenz N. 2014. Proposal of a comprehensive definition of modified and other forms of mycotoxins including “masked” mycotoxins. Mycotoxin Res. 30:197–205.
- Senyuva HZ, Gilbert J. 2010. Immunoaffinity column clean-up techniques in food analysis: a review. J Chromatogr B. 878:115–132.
- Stroka J, Seidler J. 2014. Determination of the mycotoxin deoxynivalenol by solvent free immunoaffinity clean-up. World Mycotoxin J. 7:115–120.
- Suman M, Bergamini E, Catellani D, Manzitti A. 2013. Development and validation of a liquid chromatography/linear ion trap mass spectrometry method for the quantitative determination of deoxynivalenol-3-glucoside in processed cereal-derived products. Food Chem. 136:1568–1576.
- Vendl O, Berthiller F, Crews C, Krska R. 2009. Simultaneous determination of deoxynivalenol, zearalenone, and their major masked metabolites in cereal-based food by LC–MS–MS. Anal Bioanal Chem. 395:1347–1354.
- Veršilovskis A, Huybrecht B, Tangni EK, Pussemier L, Saeger SD, Callebaut A. 2011. Cross-reactivity of some commercially available deoxynivalenol (DON) and zearalenone (ZEN) immunoaffinity columns to DON- and ZEN-conjugated forms and metabolites. Food Addit Contam. 28:1687–1693.