ABSTRACT
To investigate the potential transfer of pyrrolizidine alkaloids (PAs), laying hens were fed for 14 days with diets containing 0.5% of dried common ragwort, common groundsel, narrow-leaved ragwort or viper’s bugloss, or 0.1% of common heliotrope. This resulted in total PA levels in feed of respectively 5.5, 11.1, 53.1, 5.9 and 21.7 mg kg–1, with varying composition. PAs were transferred to eggs, in particular yolk, with steady-state levels of respectively 12, 21, 216, 2 and 36 µg kg–1. Overall transfer rates for the sum of PAs were estimated between 0.02% and 0.23%, depending on the type of PAs in the feed. In animals slaughtered shortly after the last exposure, levels in meat were slightly lower than those in eggs, levels in livers somewhat higher. When switched to clean feed, levels in eggs gradually decreased, but after 14 days were still above detection limits in the hens exposed to higher PA levels. Similar was the case for meat and especially kidneys and livers. It is concluded that the intake of PA containing herbs by laying hens may result in levels in eggs and meat that could be of concern for consumers, and as such should be avoided.
Introduction
Pyrrolizidine alkaloids (PAs) occur in a large number of plants in many parts of the world. They are not produced by plants consumed by humans, but nevertheless are being detected in our food, most likely due to contamination (Edgar et al. Citation2011; EFSA 2011). Recently a survey was performed for EFSA in which a large number of food samples were analysed (Mulder et al. Citation2015). Higher levels of PAs in particular were observed in various teas and food supplements. In animal-derived products like milk, meat and eggs, PAs were only present at low levels and in some of the samples. However, the project investigated a limited number of samples and from only six countries.
Various herbs may be present in pastures and fields where animals are foraging. They may also be present in fresh or dried products fed to animals, as shown in particular for alfalfa, a widely used feed product (EFSA 2011). When consumed by animals, part of the PAs will be transferred to animal-derived food products (Dickinson et al. Citation1976; Edgar & Smith Citation2000; Hoogenboom et al. Citation2011). Although the transfer and levels in animal-derived products seem relatively low, exposure via such products may still be relevant. This is due to the fact that various PAs were shown to contain genotoxic and carcinogenic properties, meaning that even intake of low levels by humans may result in adverse effects (Fu et al. Citation2004; EFSA 2011; Edgar et al. Citation2015). The common policy is to reduce the presence of genotoxic carcinogens in our food as much as possible.
With respect to chickens, there is very limited information on the transfer of PAs to eggs and meat. Edgar and Smith (Citation2000) followed-up a case where feed had become contaminated with PAs, probably from seeds of common heliotrope but possibly also from other plants. Levels in contaminated grain were estimated to be 26 mg kg–1 (primarily heliotrine, europine and lasiocarpine); levels in two egg samples taken during the incident were on average 157 µg kg–1. Eröksüz et al. (Citation2003) provided laying hens for 210 days with feed containing up to 4% dried eastern groundsel (Senecio vernalis). The reported feed level of 56 mg kg–1 feed (primarily N-oxides of senecionine and to a lesser extent senecivernine, seneciphylline and integerrimine) did not result in detectable levels of PAs in eggs. Diaz et al. (Citation2014) included 1%, 2% or 3% of seeds of Crotalaria pallida in feed of laying hens for a period of 35 days. Seeds contained primarily usaramine and some N-oxide, resulting in feed levels of 18, 36 and 54 mg kg–1. Usaramine was detected in the eggs at steady-state levels between 100, 150 and 225 µg kg–1 for the three doses respectively. The results of the study by Eröksüz et al. (Citation2003) seem contradictive of the other two but might be explained by the sensitivity of the analytical methods applied. The GC-MS method applied by Eröksüz et al. (Citation2003) had, for example, a rather high LOQ (Eröksüz et al. Citation2008). However, also differences in PA composition may play a role. Previously it was shown that the overall transfer rate of PAs from a mixture of common ragwort (Jacobaea vulgaris, syn. Senecio jacobaea) and narrow-leaved ragwort (Senecio inaequidens) to milk in dairy cows was relatively low (0.1%), but that this rate was quite different for the various PAs (Dickinson et al. Citation1976; Hoogenboom et al. Citation2011). The N-oxides, for example, which contribute largely to the overall content in the herbs, were not detected at all in milk, whereas jacoline showed a transfer rate of 8%. Therefore, the overall transfer to animal-derived products largely depends on the type of PA profile in the herbs present in the feed. The overall balance in the study by Hoogenboom et al. (Citation2011) was rather poor, suggesting the large-scale formation of unknown metabolites (including protein adducts) or retention of PAs in the body of the cows.
The aim of the present study was to examine further the transfer of PAs to eggs, but also livers and meat of laying hens fed with herbs containing different PA profiles, more specifically, common ragwort (Jacobaea vulgaris), common groundsel (Senecio vulgaris), narrow-leaved ragwort (Senecio inaequidens), viper’s bugloss (Echium vulgare) and common heliotrope (Heliotropium europaeum). Also, the overall balance was studied. The results show the transfer of certain PAs to eggs, although with a low overall transfer rate, but also the presence of PAs in meat, liver and kidney. Overall, only part of the PAs were recovered, suggesting extensive metabolism.
Materials and methods
Materials
Common ragwort (J. vulgaris, JV), common groundsel (S. vulgaris, SV), narrow-leaved ragwort (S. inaequidens, SI) and viper’s bugloss (E. vulgare, EV) were collected in the vicinity of Wageningen, the Netherlands, in the summer of 2014. Common heliotrope (H. europaeum, HE) does not occur in the Netherlands and was collected in the French Luberon region in August 2014. Materials were air dried and subsequently milled and homogenised using a Peppink 200 AN Grinding machine (Veerman, Olst, the Netherlands). Feed for laying hens containing 0.5% of those materials (either common ragwort, common groundsel, narrow-leaved ragwort or viper’s bugloss) or 0.1% (common heliotrope) of the dried herbs were prepared by Research Diet Services (Wijk bij Duurstede, the Netherlands). Standards of PAs were obtained from Phytoplan (Heidelberg, Germany), Phytolab (Vestenbergsgreuth, Germany) and Latoxan (Valence, France). Epi-jacobine, a synthetic epimer of jacobine, was obtained from Mercachem (Nijmegen, the Netherlands).
Animal study
A protocol was prepared and approved by the Animal Ethics Committee. Sixty laying hens of about 24 weeks old (Novocen, van Beek, Lunteren, the Netherlands) were randomly distributed over 12 ground cages (five hens per cage). An additional five hens were put in another cage to study the overall balance. Light was on 16 h per day; temperature was around 20°C. After 14 days (day 0–13) on blank feed, birds (10, divided over two cages) were fed ad libitum with either blank feed or one of the feeds containing one of the five different herbs for a period of 14 days (days 14–27). The amount of dried herbs was 0.5% except for common heliotrope (0.1%) since in the latter herb the PA levels were higher. Table S1 in the supplemental data online shows the composition of the basal diet.
After 14 days of treatment (day 28), half the hens (two from the first cage, three from the second cage) from each group were killed by electrocution and debleeding, and the livers, kidneys and breast muscle were weighed and collected for further analysis. Also plasma was collected. Plasma and liver samples were stored at –80°C, kidneys and muscle at –20°C. The other half of the hens was switched to clean feed for 2 weeks (days 28–41) to study the decrease in the potential levels in meat and eggs. These hens were subsequently killed on day 42, and samples collected as described above. Eggs were collected during the whole study period. Animals were tagged and weighed on days 0, 14 (start of exposure), 28 (end of exposure) and 42 (end of study). Feed remains were weighed on days 7, 14, 21, 28, 35 and 42.
The remaining five hens were fed sequentially with each of the experimental feeds for 5 days followed by 2 days on clean feed. Feed consumption was registered and faeces were collected during the last 2 days of each 5-day period on contaminated feed. The order was JV, SV, SI, EV, finishing with HE.
Analysis of PAs in feed material
The PA composition and homogeneity of the prepared animal feed materials was assessed by the analysis of 10 samples randomly taken from each feed material. Of each sample 2 g was transferred to 50 ml test tubes. Epi-jacobine (20 µl of a 1000 µg l–1 solution in methanol) was added as internal standard. Of a 2% formic acid solution in water 40 ml was added and the samples extracted by rotary tumbling for 1 h. After centrifugation for 15 min at 3500g, 5-ml aliquots of the extracts were subjected to SPE clean-up over StrataX 60 mg/3 cc cartridges (Phenomenex, Torrance, CA, USA). Cartridges were conditioned with 3 ml methanol followed by 3 ml water. After application of 5 ml extract, the cartridges were washed with 3 ml 1% formic acid solution followed by 3 ml 1% ammonium hydroxide solution. The SPE cartridges were dried by applying reduced pressure using a vacuum manifold for 5–10 min and the analytes were eluted with 3 ml methanol into 10 ml polypropylene test tubes. The samples were evaporated under a gentle flow of nitrogen in a water bath kept at 50°C (TurboVap, Zymark, Uppsala, Sweden) and the dry residues were reconstituted in 500 µl water/methanol 9:1 (v/v) and filtered using 0.45 µm PTFE 500 µl mini UniPrep filtervials (Whatman, GE Healthcare, Maidstone, UK). Sample extracts were injected (5 µl) on the LC-MS/MS in a randomised order. Quantification was performed against a six-point calibration curve of PA standards (0–500 µg l–1) in a diluted extract of blank feed. One control sample of blank feed was spiked with PA standards at 1000 µg kg–1 and carried through the sample preparation procedure to determine the recovery of the method. The LOQ for individual PAs and their N-oxides was between 2 and 5 µg kg–1.
Analysis of PAs in egg
The PA content in eggs (whole egg, egg white, egg yolk) was assessed by means of LC-MS/MS. Test portions of 3.0 ml of homogenised whole egg, egg white or egg yolk were transferred to 50 ml test tubes and 30 µl of epi-jacobine IS solution (1000 µg l–1 in methanol) were added, followed by 27 ml of 0.2% formic acid solution and 15 ml hexane. The mixture was agitated for 30 min on a rotary tumbler and centrifuged for 15 min at 3500g. The hexane layer and (most of) the solid middle layer (containing most of the non-soluble proteins) was removed by suction. Concentrated ammonia solution (25%) was added to adjust the pH to 9–10 using a pH test strip. The samples were centrifuged for another 15 min at 3500g and 5 ml of the remaining aqueous extract was used for further clean-up by SPE over a StrataX 200 mg, 6 cc cartridge (Phenomenex). The cartridges were conditioned with 6 ml methanol and 6 ml ammonia solution (0.1%). The cartridges were loaded with 5 ml of extract, washed with 6 ml ammonia solution (0.1%) and dried under vacuum using a vacuum manifold for 5–10 min. PAs were eluted from the cartridges with 6 ml of methanol. The eluates were dried under a nitrogen stream at 50°C in a water bath (TurboVap) and reconstituted in 500 µl of methanol/water (10/90, v/v). The reconstituted sample extracts were filtered using 0.45 µm PTFE 500 µl filtervials (UniPrep, Whatman). Subsequently, 5 µl were injected on the LC-MS/MS system. The sample extracts were quantified against an eight-point calibration curve of PA standards (0–100 µg kg–1) in blank whole egg homogenate, blank egg white or blank egg yolk prepared from eggs from the blank control group. The LOQ in egg matrix for the individual PAs and their N-oxides was between 0.1 and 0.25 µg kg–1.
Analysis of PAs in meat, liver and kidney
For muscle tissue, liver and kidney samples, a slightly modified protocol was applied as described for egg. To test portions of 3 g homogenised chicken filet, liver or kidney in 50 ml test tubes 30 µl epi-jacobine IS solution (1000 µg l–1 in methanol) were added, followed by extraction with 30 ml of 0.2% formic acid solution and 15 ml hexane. After removal of the hexane top layer and the solid middle layer, 10 ml of extract were transferred to a new test tube. Concentrated ammonia solution (25%) was added to adjust the pH to 9–10 using a pH test strip. The samples were centrifuged for another 15 min at 3500g and 5 ml of the remaining aqueous extract was used for further clean-up using the same SPE procedure as described for egg. The sample extracts of muscle tissue were quantified against an eight-point calibration curve of PA standards (0–25 µg kg–1) in blank chicken fillet from the control group, those of liver tissue against an eight-point calibration curve of PA standards (0–50 µg kg–1) in blank liver tissue homogenate from the control group. And those of kidney tissue against an eight-point calibration curve of PA standards (0–50 µg kg–1) in blank kidney homogenate from the blank control group. The LOQ in muscle tissue, liver and kidney for the individual PAs and their N-oxides was between 0.1 and 0.5 µg kg–1 for muscle tissue and between 0.25 and 1 µg kg–1 for liver and kidney tissue.
Analysis of PAs in excrements
For the analysis of PAs in excrements a slightly modified procedure was followed. Excrements were lyophilised by means of freeze drying (REVO RD-85S5, Millrock Technologies, Kingston, NY, USA). To 2 g lyophilised material in 50 ml test tubes, 20 µl epi-jacobine IS solution (1000 µg l–1) were added, followed by 20 ml of 0.2% formic acid solution. The mixture was agitated for 30 min on a rotary tumbler and centrifuged for 15 min at 3500g and 10 ml of the extract was transferred to a new test tube and concentrated ammonia solution (25%) was added to adjust the pH to 9–10 using a pH test strip. The samples were centrifuged for another 15 min at 3500g and 5 ml of the remaining aqueous extract was used for further clean-up using the same SPE procedure as described for egg. Sample extracts were quantified against a nine-point calibration curve of PA standards (0–250 µg kg–1) in blank lyophilised excrements homogenate from the control group. LOQs in lyophilised excrements ranged between 1 and 5 µg kg–1 for most PAs. In general, the free bases were somewhat more sensitive than the N-oxides.
Instrumentation
Analysis of feed extracts were performed on a Waters Acquity UPLC coupled to a Waters Quattro Premier XE tandem mass spectrometer (Waters, Milford, MA, USA), operated in positive electrospray mode. Analysis of egg, muscle, liver, kidney and excrement samples was performed on a Waters Acquity UPLC coupled to a Waters Xevo TQ-S tandem mass spectrometer (Waters, Milford, MA, USA), operated in positive electrospray mode. With both systems, compounds were separated on a Waters UPLC BEH C18 150 × 2.1 mm, 1.7 µm analytical column, kept at 50°C and run at 0.4 ml min–1 with an acetonitrile/water gradient containing 6.5 mM ammonia. The gradient started at 100% water and was changed to 50% acetonitrile in 12 min. Total runtime of the method was 15 min. For both systems the MS/MS settings were optimised for each individual compound using reference standards or plant extracts when standards were not available. Two precursor product ion transitions were selected and incorporated in an MRM method. The dwell time for each transition was set at 10 ms for the Quattro Premier and at 3 ms for the Xevo TQ-S. Two MS/MS methods were constructed, one covering all potentially relevant PAs from common ragwort and common groundsel and one covering the PAs present in viper’s bugloss and common heliotrope. and present an overview of the mass spectrometric settings used for the detection of the relevant PAs. All compounds could be characterised on the basis of retention time and fragmentation transitions. PA metabolites showing the typical fragmentation patterns characteristic for PAs, but for which no structure or tentative name could be derived were labelled according to their protonated mass and retention time. With the final method over 90 compounds (including several metabolites) were monitored. For those compounds for which no reference standard was available, a semi-quantitative (indicative) value could be obtained by comparison with a closely related analogue (often an isomer), which exhibited a similar MS/MS fragmentation spectrum. In these cases the same transitions were used. For some compounds (e.g., jaconine and its N-oxide) and several metabolites of unknown structure, no closely related standard with similar MS/MS spectrum could be identified. In such cases the concentration was estimated by taking the sum of the two most intense fragments and comparing this with the sum area of the transitions selected for the most closely related standard (e.g., jacobine and its N-oxide), as indicated in and .
Table 1. Mass spectrometric conditions used for the analysis of pyrrolizidine alkaloids (PAs) in common ragwort (JV), common groundsel (SV) and narrow-leafed ragwort (SI).
Table 2. Mass spectrometric conditions used for the analysis of PAs in viper’s bugloss (EV) and common heliotrope (HE).
Stability of PAs in eggs
To test the stability of PA residues in egg, 18 eggs of each treatment were collected at days 23, 24 and 26. Eggs were stored for 2, 4 or 8 weeks at RT or at 4–6°C. At each time point three eggs were taken, the contents were pooled and the homogenates stored at –20°C until analysis.
Histology
Liver samples were taken directly after dissecting the animals. All tissues were fixed in 4% buffered formaldehyde. Routine histology was performed on 5 µm thick haematoxylin and eosin (HE)-stained paraffin sections. Histological parameters were described and/or specific parameters were scored semi-quantitatively based on the degree of change. For liver of each bird, 10 fields using objective magnification 40× were scored for anisokaryosis, apoptosis and the number of mitoses per view using a semiquantitative 0+, 1+, 2+, 3+ system.
From all sections photographs were made. Per printed photograph the diameters of the liver nuclei were measured using a ruler. Mean measurement results (in arbitrary units) per photograph, made using objective 40×, were noted.
Statistics
The observed differences in body weight, and absolute and relative liver weights were tested for statistical significance using a Student’s t-test (two-sided). It should be noticed that this was performed on an individual basis whereas the hens were kept in two cages per treatment. This could have introduced some bias, as will be discussed.
Results and discussion
Animal study
After an initial adaptation period of 2 weeks, groups of 10 laying hens were given feed containing 0.5% dried common ragwort (JV), common groundsel (SV), narrow-leaved ragwort (SI) or viper’s bugloss (EV), or containing 0.1% common heliotrope (HE) for 14 days. After this period, half the animals were sacrificed and half the animals were put on clean feed for another 14 days. gives an overview of the body weights, feed intake, egg production and feed conversion. Table S2 in the supplemental data online gives an overview of individual data for body and liver weights. Feed intake gradually decreased in the group fed with SI containing feed.
Table 3. Body weight, feed intake and egg production during the adaptation (days 0–14), exposure (days 14–28) and washout period (days 28–42). Treatment was either with common ragwort (JV), common groundsel (SV), narrow-leaved ragwort (SI), viper’s bugloss (EV) or common heliotrope (HE).
Body weights of the 10 hens fed SI were significantly reduced (p < 0.05) at the end of the treatment period (day 28). The effect was still observed in the five remaining hens after the additional 2 weeks on clean feed (day 42), but no longer significant (p = 0.07). In this group, also the weight change per hen was significant (p < 0.01) at day 28 and borderline at day 42 (p < 0.05). As the effect was observed in both cages and in none of the others, it seems unlikely that it is a cage related effect (changes of –126 ± 146 and –225 ± 76 g in the two SI cages, versus 33 ± 34 and –45 ± 64 g in the control cages). There was also a significant decrease (p < 0.01) in liver weights of SI-treated hens at the end of the exposure period, showing weights of 25 ± 6 versus 40 ± 5 g in the controls (mean ± SD, n = 5) ( and Table S2 in the supplemental data online). The effect did not disappear after the additional 2 weeks on clean feed, being 28 ± 7 g for SI versus 42 ± 8 g for the controls, although being less significant (p < 0.05). This effect could not be explained by the decrease in body weights, since also the relative liver weights were significantly decreased, being 1.6 ± 0.4% for SI versus 2.2 ± 0.1% in the controls at day 28 (p < 0.01), and 1.9 ± 0.3% for SI versus 2.4 ± 0.3% in the controls (p < 0.05) at day 42. The other treatments had no significant effect on the body and liver weights. Kidney weights were not affected by any of the treatments. Diaz et al. (Citation2014) also observed a dose- and time-related decrease in body weights and relative liver weights in all three dose groups (feed levels of 18, 36 and 54 mg kg–1), the highest concentration being comparable with the one in the feed with SI (see below).
As shown in , there was a decrease in laying frequency and as a result also in the mean egg production per cage in hens fed with the diet containing SI. This started during the treatment period but further continued in the period on clean feed. Similar effects were observed in the hens used for the balance study. None of the effects was statistically significant, also due to the fact that egg production was recorded per cage and hence the statistical power was rather low. A decrease in egg production was also observed by Diaz et al. (Citation2014), who ascribed it to potential liver damage, regarding the important role of the liver in production of the egg yolk.
The primary aim of the study was to investigate the transfer of PAs, and to a lesser extent potential effects of the treatment. Nevertheless, the SI supplemented feed, containing the highest PA levels, showed clear indications of a reduction in feed consumption, body weight, both absolute and relative liver weight and in egg production.
Histology
Livers of hens slaughtered just after the last treatment were examined histologically for a number of different effects (see for means and Table S3 in the supplemental data online for individual hens). Because of the limited accuracy of the semi-quantitative measurements, statistical testing was not applicable. However, clear differences were observed between SI and the control group: an increase in anisokaryosis (differences in nuclear size), apoptosis, mitosis and increased nuclear diameter. For the other treatments almost no effects were seen.
Table 4. Histological effects (using a 0+ to 3+ semiquantitative system, summed for five animals) in livers of hens treated with different herbs for 14 days.
Earlier studies with PAs in chickens also reported histological effects on the liver. Groups of 10 laying hens were fed diets with 0.5, 2 or 4% Senecio vernalis for 210 days (Eröksüz et al. Citation2003). Hens fed 2 or 4% plant diets showed chronic liver changes consisting of periportal or septal fibrosis, megalocytosis, bile duct hyperplasia and early regenerative nodule formation. Huan et al. (Citation1992) studied effects of 5% common ragwort (JV) in the diet in broilers. Anisokaryosis and enlargement of liver nuclei was observed in both studies. The effect has also been frequently described for domestic animals in Australia after consumption of Lantana species (Pass et al. Citation1979). Gopinath and Ford (Citation1977) performed feed experiments with 7% dried and ground common ragwort in 1-week-old chickens for 6 weeks. This led to megalocytosis, focal necrosis and portal fibrosis. Nuclear changes were also observed. These authors stated explicitly that occlusion of the smaller blood vessels was not observed in the livers. Also in the present experiment this well-known pathological effect of PAs on blood vessels in humans (Fan & Crawford Citation2014) was not observed.
PA levels in animal feeds
A number of PAs were determined in the feeds and animal-derived products. This included the free and N-oxide forms of various PAs but also some unknown PAs, which showed masses and mass fragments typical for PAs and were all assigned an abbreviation based on retention time and mass (see Tables S4–S8 in the supplemental data online). The PAs present in the different herbs were in accordance with those reported previously (Hartmann & Witte Citation1995; El-Shazly & Wink Citation2014).
PA levels in the animal feeds prepared with different herbs are shown in the third column of Tables S4–S8 in the supplemental data online, which also show the levels in eggs, muscle, liver and kidney of hens slaughtered just after the last treatment (discussed below). For all diets the N-oxides clearly dominated compared with the free bases. Overall PA levels for the diets fortified with JV, SV, SI, EV and HE were respectively 5.5, 11.1, 53.1, 5.9 and 21.7 mg kg–1.
There are clear differences in the PA patterns of the different diets. In the case of JV the N-oxides of erucifoline (21%), jacobine (16%), senecionine (15%), seneciphylline (11%) as well as the free base of erucifoline (10%) were the major components, together 72% of total identified PAs. To some extent these PAs were also detected for SV and SI but in a different composition. In the SV diet the major PAs were the N-oxides of seneciphylline (44%), retrorsine (16%), senecionine (15%) and integerrimine (6%), and the free base of seneciphylline (6%), together 86% of the sum. For SI these were the N-oxides of retrorsine (45%), senecivernine (16%), integerrimine (9%) and usaramine (7%), together 77% of total PAs. Contrary to the other diets, this feed also contained some otonecines like otosenine and florosenine. For EV the diet contained primarily the N-oxide of echimidine (86%), an unknown retronecine diester N-oxide (7%) and some free base echimidine (6%), together accounting for 99% of the PA content. In the case of HE the major PAs present were the N-oxides of heliotrine (37%), europine (27%) and lasiocarpine (20%), together accounting for 84% of total PAs.
PA levels in eggs
Eggs were pooled per day and in most cases analysed as whole egg homogenates.No changes in PA content were observed for eggs stored for up to 8 weeks at RT or under cooled (4–6°C) conditions, indicating that no significant metabolism or degradation takes place after the eggs are laid (data not shown).
shows the total PA concentrations for each type of herb at various time points during the 14-day treatment and after the switch to clean feed. Administration of the contaminated feed material started on day 14 and ended on day 28. For all treatments there was a gradual increase in the levels during the treatments up to a certain maximum, and also a gradual decrease when switched to clean feed. Highest PA levels were observed for SI, around 200 µg kg–1, lowest levels for EV, around 2 µg kg–1. The differences in eggs levels roughly reflected those in the feed.
Figure 1. Transfer of pyrrolizidine alkaloids (PAs) to whole egg (pooled samples of six eggs). Treatments: (a) common ragwort (JV), (b) common groundsel (SV), (c) narrow-leafed ragwort (SI), (d) viper’s bugloss (EV) and (e) common heliotrope (HE). Administration via feed started on day 14 and ended on day 27. Jl, jacoline; Jb, jacobine; Er, erucifoline; Rt, retrorsine; Sp, seneciphylline; Sn, senecionine; RtNO, retrorsine N-oxide; Ot, otosenine; Em, echimidine; FB316, retronecine monoester with protonated mass 316; Eu, europine; Ht, heliotrine.
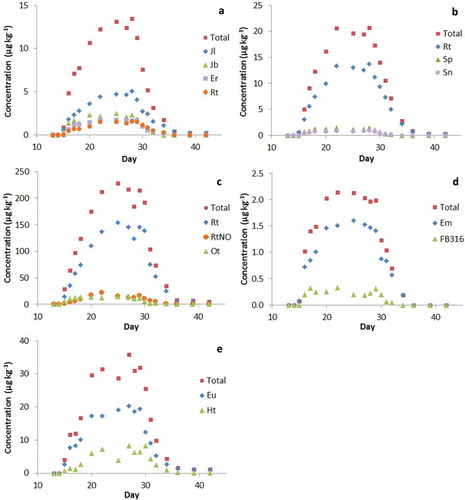
Tables S4–S8 in the supplemental data online include the levels of individual PAs in eggs sampled after reaching the plateau level. The PAs contributing most for each treatment are included in , showing in principle the same patterns as the total PAs. shows the PA patterns for feed, eggs and different tissues as well as excrements for JV and HE, including all PAs contributing at least 1% as free base or N-oxide to the sum in one of the matrices. PA patterns for all treatments can be found in Figure S1 in the supplemental data online. Although some N-oxides were detected in eggs, the majority of PAs was present in their free base form. In the case of JV ((a) and Table S4 in the supplemental data online), jacoline (38%), jacobine (17%), erucifoline (14%) and retrorsine (11%) were most relevant (together 80%), which in the feed were actually rather minor components (17% of total PAs). To some extent (for jacobine and erucifoline), this might be explained by the conversion of the N-oxides to the free bases. However, that does not apply for retrorsine and jacoline. This suggests a very selective excretion of certain PAs to the eggs, possibly related to a higher stability against metabolic degradation. A similar situation was observed in dairy cows fed with common ragwort where jacoline was by far the most important PA in milk, but also in urine (Hoogenboom et al. Citation2011).
Figure 2. PA patterns for animal feed, eggs, tissues and excrements of hens fed with (a) common ragwort (JV) or (b) common heliotrope (HE). Only PAs contributing at least 1% as free base or N-oxide in one of the matrices are included. Upper red part of the bars presents the N-oxides.
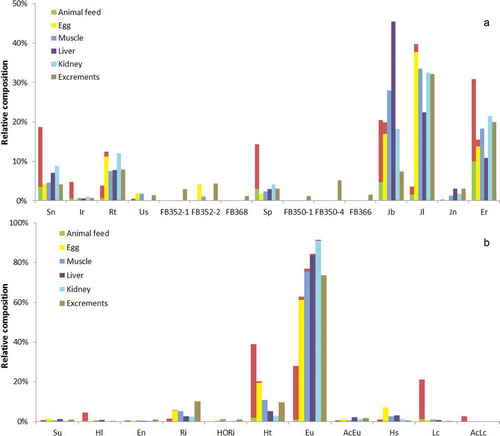
Despite the high contribution of seneciphylline in feed containing SV, the free base contributed for only 6% in the eggs (see Table S5 in the supplemental data online). The most predominant PAs were actually retrorsine (58%), its N-oxide (7%), seneciphylline (6%) and an unknown retronecine macrocyclic ester with protonated mass 338 (10%). Together these four PAs contributed for 81% to the total PA level.
Eggs from hens fed SI showed the highest levels for retrorsine (67%), usaramine (8%) and otosenine (7%). Retrorsine N-oxide, being the most important PA in feed (see Table S6 in the supplemental data online), also contributed to some extent (6%). Together these PAs contributed for 89% to the sum.
For EV (see Table S7 in the supplemental data online), the free base echimidine (75%) was by far the most important PA in eggs, possibly resulting from the conversion of the N-oxide being the prominent PA in the feed. There were also contributions from a retronecine monoester metabolite with mass 316 (9%) and an unknown retronecine diester (12%), altogether amounting to 96% of the total PA level.
A conversion of the major PA N-oxides to the corresponding free bases, and subsequent transfer to eggs seems also to take place for HE ((b) and Table S8 in the supplemental data online). In this case, europine (61%) and heliotrine (20%) are the most important PAs. The order clearly changed between feed and eggs. Two other PAs detected in eggs, heliosupine (7%) and rinderine (5%), were clearly less important in the feed. Together, the four major PAs in eggs contributed for 93% to the total PA level. Lasiocarpine, another major PA in the feed, was hardly detected in the eggs. Similar was observed by Eröksüz et al. (Citation2008) for quails fed with Heliotropum dolosum, with europine being the only PA detected in eggs, whereas in the herb lasiocarpine and its N-oxide were by far the most dominant PA.
Distribution of PAs over egg yolk and egg white
The gradual increase during the treatment and gradual decrease after the switch to clean feed suggested a difference in the distribution of PAs in egg white and egg yolk. The yolk of eggs is formed during a period of about 10 days, contrary to the white which is added at a late stage (Donoghue et al., Citation1996). To investigate the distribution of PAs over egg white and yolk, a number of individual egg samples were analysed (). It was shown that the PA concentrations were considerably higher in the yolk than in the egg white for all five herbs. The differences became larger with longer treatment, in line with the duration of yolk and white formation. This is also reflected by the ratio between egg yolk and white, as shown in Figure S2 in the supplemental data online. The ratio steadily increased during the treatment, due to the 10-day production of the egg yolk, as compared with 1 day for the white. For the same reasons, the ratio rapidly further increased after the switch to clean feed, since the levels in the white of new eggs decreases very rapidly whereas those in the yolk still represent the exposure period. In the case of hens fed with viper’s bugloss, the differences between egg yolk and egg white were much smaller.
Figure 3. Total PA concentration in egg yolk and white (individual samples). Treatments: (a) common ragwort (JV), (b) common groundsel (SV), (c) narrow-leafed ragwort (SI), (d) viper’s bugloss (EV) and (e) common heliotrope (HE). Administration via feed started on day 14 until day 27. Clean feed was provided on day 28 until day 42.
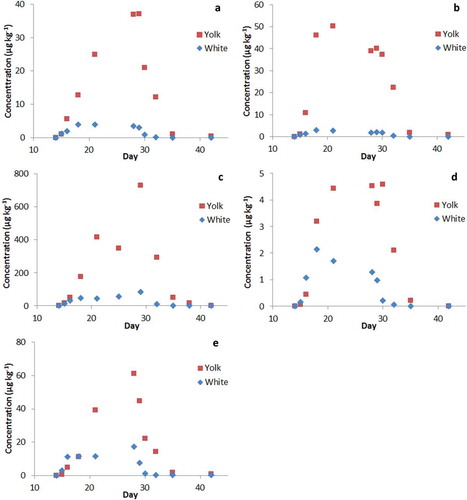
PA levels in meat, liver, kidney
Breast muscle, livers and kidneys were collected from the hens slaughtered either after the last treatment (day 28) or after the additional 2 weeks on clean feed (day 42). shows the mean total PA concentrations in muscle, liver and kidney tissue at the two slaughter moments. The results for whole egg, sampled the day before slaughter, are included for comparison. It is evident that PAs could be detected in all meat samples just after the last exposure, with the highest level of 67 µg kg–1 in the hens with the highest exposure, i.e., those fed with SI. Levels in livers and kidneys were in general much higher than those in breast muscle. Even after a 14-day period on clean feed, PAs were still detectable in tissues from the animals with the highest exposure (SI and HE).
Table 5. Total PA concentrations (µg kg–1) in animal tissues (days 28 and 42: n = 5, and eggs collected on days 27 and 41 (n = 6) (mean ± SD).
The concentrations of individual PAs in muscle, liver and kidney tissue at day 28 are included in Tables S4–S8 in the supplemental data online. In general the PAs that contribute most to the total levels are the same as observed in the eggs (see for JV and HE, and Figure S1 in the supplemental data online for all treatments).
Mass balance
A separate group of hens was subsequently exposed to various herbs for a number of days in order to quantitatively collect the excrements. The concentrations of individual PAs in excrements are included in Tables S9 (JV, SV, SI) and S10 (EV, HE) in the supplemental data online and were used to calculate the excretion. shows the calculated amounts of ingested PAs and the amounts of PAs excreted via the excrements. In addition, the absolute amounts excreted via the eggs or remaining in the various tissues at the end of the exposure period are given, based on the experimental groups that received only one herb. The overall balance varies between 3 and 27% and is rather poor. The fact that not all tissues were sampled and analysed, is unlikely to explain this poor recovery. More likely is the formation of unknown metabolites which may both be free metabolites but also metabolites bound to tissue proteins. Very little is known about the potential adverse effects of such metabolites and further studies are required to identify and characterise these metabolites, in particular if they are excreted into eggs or present as residues in edible tissues.
Table 6. Amounts administered via feed (µg total PAs) and recovered in animal tissues and excrements (mean ± SD). Amounts are based on levels and weights of tissues and excrements, so in the case of muscle only the breast tissue of around 200 g.
Transfer rates
includes the estimated transfer rate for total PAs from feed to eggs at the plateau level, based on the levels measured in feed, eggs at day 27, feed consumption and egg production. This shows that the rates vary between 0.02% for the hens fed EV and 0.23% for the hens fed SI. This latter transfer rate is similar to those reported by Edgar and Smith (Citation2000) and Diaz et al. (Citation2014), who reported levels around a few hundred µg kg–1 after exposure of hens to feed containing 26 and 18–54 mg kg–1, respectively. This is comparable with the 216 µg kg–1 observed in the SI group with 53 mg PAs kg–1 feed.
As shown before in the case of dairy cows, the transfer rates clearly depend on the PA composition of the herbs. shows transfer rates for a number of different PAs from feed to eggs, combining the free bases and N-oxides. In some cases, levels in feed were too low to result in detectable levels in eggs, even if the transfer rate were in a similar range as those for other PAs or observed with other herbs. Transfer rates vary between PAs with a factor of up to 1000. In general transfer rates for PAs present in JV, SV and SI are comparable. Highest rates were observed for usaramine, retrorsine, otosenine, senkirkine and especially jacoline and sceleratine. The transfer rate for echimidine in EV was much lower. In the case of HE, supinine, rinderine and heliosupine showed relatively high transfer rates, while that of lasiocarpine was very low.
Table 7. Transfer ratesa for individual PAs for feed to eggs, expressed as the fraction of the daily dose excreted in eggs (%). Levels of the free base and N-oxide were combined for each PA.
Tables S11 (JV, SV and SI) and S12 (EV and HE) in the supplemental data online show bioconcentration factors (BCFs) for eggs, meat, liver and muscle, being the ratio between the level in the respective food product and the feed (again free bases and N-oxides combined). It is clear that PAs with relatively high BCFs in eggs are also the most important ones in meat, liver and kidney. In particular jacoline shows relatively high BCFs in all matrices. This may indicate that these PAs are relatively stable against metabolic degradation. BCFs in liver and kidney are in general higher than those in eggs and breast muscle.
Risks for consumers
The relatively low transfer rates to eggs may raise the question whether consumption of PA containing herbs could be of potential concern for consumers. Although little is known about consumption of PA-containing herbs by laying hens, the actual amounts consumed in the present study were not very high, being around 0.1 g day–1 for HE and 0.5 g day–1 for the other herbs. This resulted in total PA levels in eggs at steady state between 2 and 216 µg kg–1, or 0.12–13 µg per egg of 60 g. Daily consumption of one egg by a person of 70 kg would thus result in an intake of 0.002–0.185 µg kg–1 bw day–1. EFSA derived a BMDL10 for tumours in rats exposed to lasiocarpine of 70 µg kg–1 bw day–1. In the absence of specific data on other PAs, this BMDL10 was also applied for evaluating exposure to other 1,2-unsaturated PAs. The above-mentioned exposure from eggs would thus result in margins of exposure (MoE) of 41,000 for the lowest level to 378 for the highest level as compared with a desired factor of 10,000 or more for compounds with genotoxic and carcinogenic properties.
As shown in , PA levels in breast muscle were three to seven times lower than in eggs. The portion size will in general be higher but the frequency of consumption lower. Nevertheless, consumption of, for example, 150 g breast muscle per week with the highest observed level of 67 µg kg–1 would still imply an average daily exposure of (0.15 × 67)/(70 × 7) = 0.02 µg kg–1 bw and an MoE of 3500. For livers and kidneys, portion sizes or consumption frequencies may be lower than for breast muscle but levels were clearly higher.
This implies that regular consumption of herbs with high PA levels by laying hens, and probably also other chickens, should be prevented, despite the rather low transfer rates.
Conclusions
The current study confirms results from previous studies, showing the transfer of PAs to eggs and in particular egg yolk. Following the switch to clean feed, levels gradually decreased. Breast muscle, kidneys and livers also contained elevated levels of PAs, and PAs were still detectable in the tissues of the higher exposed animals after 14 days. There were clear differences between the various PAs, which also affected the overall transfer rates of total PAs. The observed levels in eggs and tissues of the higher exposed hens would imply a potential risk for consumers, showing that such intakes should be avoided. This includes removal of PA containing herbs in courtyards where laying hens forage, The contamination of dried feed materials like alfalfa, but also the deliberate feeding of certain PA containing herbs, including the potential use for medical reasons.
TFAC1241430__Supplementary_Data.docx
Download MS Word (272.1 KB)Disclosure statement
No potential conflict of interest was reported by the authors.
Supplemental data
Supplemental data for this article can be accessed here.
Additional information
Funding
References
- Diaz GJ, Almeida LX, Gardner DR. 2014. Effects of dietary Crotalaria pallida seeds on the health and performance of laying hens and evaluation of residues in eggs. Res Vet Sci. 97:297–303.
- Dickinson JO, Cooke MP, King RR, Mohamed PA. 1976. Milk transfer of pyrrolizidine alkaloids in cattle. J Am Vet Med Assoc. 169:1192–1196.
- Donoghue DJ, Hairston H, Gaines SA, Bartholomew MJ, Donoghue AM. 1996. Modelling residue uptake by eggs: 1. Similar drug residue patterns in developing yolks following injection with ampicillin or oxytetracycline. Poult Sci. 75:321–328.
- Edgar JA, Colegate SM, Boppré M, Molyneux RJ. 2011. Pyrrolizidine alkaloids in food: a spectrum of potential health consequences. Food Addit Contam Part A. 28:308–324.
- Edgar JA, Molyneux RJ, Colegate SM. 2015. Pyrrolizidine alkaloids: potential role in the etiology of cancers, pulmonary hypertension, congenital anomalies, and liver disease. Chem Res Toxicol. 28:4–20.
- Edgar JA, Smith LW. 2000. Transfer of pyrrolizidine alkaloids into eggs: food safety implications. In: Tu AT, Gaffield W, editors. Natural and selected synthetic toxins, biological implications. ACS symposium series 745. Washington (DC): American Chemical Society; p. 118–128.
- [EFSA] European Food Safety Authority. 2011. Scientific opinion on pyrrolizidine alkaloids in food and feed. EFSA panel on contaminants in the food chain (CONTAM). Scientific opinion on pyrrolizidine alkaloids in food and feed. EFSA J. 9:2406.
- El-Shazly A, Wink M. 2014. Diversity of pyrrolizidine alkaloids in the boraginaceae – structures, distribution and biological properties. Diversity. 6:188–282.
- Eröksüz H, Eröksüz Y, Özer H, Yaman I, Tosun F, Akyüz Kizilay C, Tamer U. 2003. Toxicity of Senecio vernalis to laying hens and evaluation of residues in eggs. Vet Hum Toxicol. 45:76–80.
- Eröksüz Y, Çeribaşi AO, Çervik A, Eröksüz H, Tosun F, Tamer U. 2008. Toxicity of Heliotropium dolosum, Heliotropium circinatum, Senecio vernalis in parental quail and their progeny, with residue evaluation of eggs. Turkish J Vet Anim Sci. 32:475–482.
- Fan CQ, Crawford JM. 2014. Sinusoidal obstruction syndrome (hepatic veno-occlusive disease). J Clin Exp Hepatol. 4:332–346.
- Fu PP, Xia Q, Lin G, Chou MW. 2004. Pyrrolizidine alkaloids – genotoxicity, metabolism, enzymes, metabolic activation, and mechanisms. Drug Metab Rev. 36:1–55.
- Gopinath C, Ford EJ. 1977. The effect of ragwort (Senecio jacobea) on the liver of the domestic fowl (Gallus domesticus): a histopathological and enzyme histochemical study. Br Poult Sci. 18:137–141.
- Hartmann T, Witte L. 1995. Chemistry, biology and chemoecology of the pyrrolizidine alkaloids. In: Pelletier SW, editor. Chemical and biological perspectives. Vol. 9. Oxford: Pergamon Press; p. 155–233.
- Hoogenboom LAP, Mulder PPJ, Zeilmaker MJ, van den Top HJ, Remmelink GJ, Brandon EFA, Klijnstra M, Meijer GAL, Schothorst R, van Egmond HP. 2011. Carry-over of pyrrolizidine alkaloids from feed to milk in dairy cows. Food Addit Contam Part A. 28:359–372.
- Huan J, Cheeke PR, Lowry RR, Nakaue HS, Snyder SP, Whanger PD. 1992. Dietary pyrrolizidine (Senecio) alkaloids and tissue distribution of copper and vitamin A in broiler chickens. Toxicol Lett. 62:139–153.
- Mulder PPJ, López Sánchez P, These A, Preiss-Weigert A, Castellari M. 2015. Occurrence of pyrrolizidine alkaloids in food. EFSA Supporting Publication EN-859; p. 1–114. [cited 2016 Oct 14]. Available from: www.efsa.europa.eu/publications
- Pass DA, Hogg GG, Russell RG, Edgar JA, Tence IM, Rikard-Bell L. 1979. Poisoning of chickens and ducks by pyrrolizidine alkaloids of Heliotropium europaeum. Aust Vet J. 55:284–288.