ABSTRACT
Fish meal is an accepted ingredient in compound feed. Unauthorised application is primarily enforced by visual inspection, i.e., microscopy. In order to document the visually available diversity, fragments of bones and scales of 17 teleost fish species belonging to seven different orders were investigated for their diversity in the presence of structural elements: lacunae and canaliculae in bone fragments and type of growth rings and teeth of scale fragments. Despite the classical division into cellular bones and acellular bones of teleost fish, i.e., whether or not possessing osteocytes, the current examinations revealed patterns of lacunae, in some types accompanied with canaliculae, in all 17 species investigated. In total seven types of bone structures were defined, and six types of scale structures. Profiles with the relative frequency of each bone type per species were established. The share of acellular bone fragments appeared to be related to the evolutionary position of the species. Results of proficiency tests for the detection of fish meal reveal that in most cases the sensitivity and specificity for the detection of fish meal ranges from sufficient to perfect. Only some specified circumstances can hamper proper recognition and identification, most notably salmon bone fragments mimicking bone fragments from terrestrial animals, and pieces of hydrolysed proteins or minerals mimicking acellular fish bone fragments. The expertise gained in this study would help to improve the distinction between fish meal and terrestrial animal material in compound feed, and it supports the application of the species-to-species ban with respect to the valorisation of by-products from fish farms in aquafeed. In a broader perspective, the current expertise might be helpful to detect fraud throughout the feed/food production chain. The matrix of characteristics versus species is implemented in a data model running in the expert system ‘Determinator’ for facilitating identification.
Introduction
Processed material originating from fish is a legal ingredient in a diverse range of different compound feeds. Fish meal is exclusively prohibited in ruminant feeds with the exception of dedicated feeds for weaning ruminant (calves) where fish meal might be used as an ingredient in milk replacer (Margry & de Jong Citation2012; European Commission Citation2013a). There is a long track record for the detection and identification of fish meal particles in proficiency tests of animal feeds in either the presence or absence of processed animal proteins of terrestrial animals (PAPs) (Veys et al. Citation2015; previous reports; van Raamsdonk et al. Citation2015; previous reports).
Fragments of vertebrate bones are the major marker for the microscopic detection of animal by-products in animal feed and feed ingredients (Gizzi et al. Citation2003; Liu et al. Citation2011). European legislation requires discrimination between terrestrial animals (mammals and birds) and fish (teleost fish, cartilaginous fish, marine arthropods). Visible characteristics of bone fragments can be observed at the level of the entire bone fragments, at the level of inclusions, i.e., the lacunae as formed by the osteocytes during life, or at the level of sub-cellular structures (canaliculae). The presence of other objects besides bone fragments such as muscle fibres, gills, scales, teeth and otholiths might add to the recognition of fish (van Raamsdonk et al. Citation2012a). The difficulty in recognising teleost fish bones is acknowledged, most recently by van Raamsdonk et al. (Citation2016) at a spike level of 0.1%, but still not explicitly examined.
In 1859, the year of the first publication of Charles Darwin’s On the Origin of Species, a presentation was held during a meeting of the Royal Society on the absence of osteocytes in fish bones of a series of fish species (Kölliker Citation1859). This absence results in the lack of (visible) lacunae in bone fragments in teleost fish meals used in compound feed. Extrapolated to the current classification of ray-finned fish based on molecular data (Actinopterygii; Near et al. Citation2012; Broughton et al. Citation2013), the most advanced group, the Acanthopterygii, shows the highest share of fish species with acellular (anosteocytic) bones. The implications of the absence of osteocysts for fish development and function were studied (e.g., Kranenbarg et al. Citation2005; Currey Citation2010; Shahar & Dean Citation2013). The consequence for monitoring the presence of fish meal in feed is that of the three levels of characteristics for fish bone identification in compound feed (van Raamsdonk et al. Citation2012a), only the level of the entire fragment characterisation is applicable to fish meal containing acellular bone fragments. The mentioned track record in proficiency testing still indicates that fish meal at a level of 1% or higher in a compound feed is detected without almost any error (high sensitivity). Notwithstanding a large range of studies into acellular bone structures and the implications of this state (Currey Citation2010), the absence of osteocytes throughout all bone structures of many fish species is disputed (Hughes et al. Citation1994; Atkins et al. Citation2015).
Voehringer (Citation1958; partly reproduced in Hohmann Citation2006) published an elaborate study of diversity in bone fragments and scales as found in fish meals regularly on the market at that time. Acellular structures were not mentioned in his study. The lack of discussion of this phenomena, combined with the suggested homogeneity of structural characteristics among all bones within each individual species in the Voehringer study, stimulates investigation of the fish bone structures of a series of teleost species commercially available in fish meals in more detail. Moreover, some recent encountered cases indicate the need for further examination of specific characteristics. These cases indicate at first that in specific situations it appears to be difficult to enforce the legal requirement to discriminate between material from fish and from terrestrial animals. Especially bone fragments from salmon can mimic those of terrestrial animals (Veys et al. Citation2011; van Raamsdonk et al. Citation2012b). Furthermore, on certain occasions detection at the required level of 0.1% seems difficult. This is indicated by recent results of proficiency tests (van Raamsdonk et al. Citation2016). Finally, the species-to-species ban (European Commission Citation2011) does apply to by-products resulting from hatcheries or fish farms. A by-product of a certain species is not allowed to be fed to fish of the same species, which needs expertise to identify fish species.
This study examines and documents the diversity necessary for monitoring the presence of fish meal in compound feed and at identifying fragments from a range of commercially used teleost fish species. The collected data are discussed in the light of a range of proficiency test results and of the evolutionary development of acellular structures.
Materials and methods
Specimen from 13 economically important fish species (FAO Globefish Citation2016) were collected from a private company and identified by barcoding of the cytb-R region (set A). The samples are listed in . Annotated materials from the RIKILT reference collection were added to the set of specimen (, set B). Samples from series 52## and 69## were collected in the past as complete fish specimen from a fishing port, and processed as a whole fish. In addition, tuna meal and salmon meal were used for establishing the fish bone types, but a frequency profile was not obtained from these two meals. Commercial fish meals originating from Chile, Peru, Denmark and Norway were used for collecting data on sensitivity and recovery.
Table 1. Overview of material used in the study.
The materials of the 13 species in set A were dissected in separate subsamples with head, body and tail, and the flesh was partly removed. Gills were prepared separately. The remaining materials of the head, body and tail subsamples per species were freeze dried, ground and subjected to a sedimentation procedure to separate the bone fraction from the other tissues. Material was observed in slides mounted with water, paraffin oil or Norland adhesive by light microscopy in bright field and polarisation, and by differential interference contrast (DIC). This procedure simulates the obligatory analytical method for monitoring animal proteins in compound feed in the European Union (European Commission Citation2013b). The entire gills were examined prior to grinding.
A sediment of an ordinary fish meal consists of bone fragments including fin rays, and in addition gill fragments, scales and scale fragments, teeth and otholiths or fragments thereof. The characteristics as described by Schweizer (Citation1931) and Voehringer (Citation1958) for bone fragments (based on presence of lacunae and canaliculae, and if so, their appearance) and for scale types (based on growth rings and connected teeth) were used as starting point for defining two sets of types, seven types for bone fragments and five types for scales. Additional elements (otholiths, teeth) were excluded from categorisation. The gills as extracted from set A were examined separately. In order to get a representative frequency distribution for every sample and species over the seven categories of bones, the numbers of bone fragments per category were balanced over the subsamples of head, body and tail of the samples in set A.
Slides were prepared from all reference materials and approximately 50 randomly selected bone fragments per sample were examined and characterised with respect to the presence of internal structures (lacunae indicating the presence of osteoblasts, canaliculae) and with respect of recognisability as fish meal. In addition, scale types were examined. The examined bone fragments and scales were categorised according to the respective types. Randomly encountered fragments with a pattern of orthogonal lines, partly originated from otholiths, were considered as an extra residual category. The frequency profiles per species are averaged over the samples per species. Although examination at lower magnifications using a binocular would enhance the opportunities to detect fish material, it would not add to the identification of microstructures. This type of examinations are therefore excluded in this study.
The documentation was used as basis for a data model for identification of processed animal proteins in compound feeds, running in the expert system platform Determinator (van Raamsdonk et al. Citation2012c).
An additional experiment was based on slides prepared from fish meals originating from Denmark, Norway, Chile and Peru. Sediments of these four fish meals were sieved and the fraction with particle size between 80 and 250 µm was used. A total of 1 mg of each of the four fish meals was evenly distributed over two slides, paraffin oil was added and the number of particles was counted independently by two technicians. After that, 4.5 mg of the fraction finer than 250 µm of a PAP-free mineral premix were added to each of the eight slides and mixed, making eight slides with a concentration of 10% fish meal. Two other technicians counted independently again the number of fish meal particles in the eight slides. The a priori and a posteriori numbers were used to calculate the recovery. The examination of the entire set of four fish meals was carried out with untreated sediments as well with sediments stained with Alizarin according to the European Union procedure (European Commission Citation2013b). Pearson’s correlation coefficient was calculated in order to support the interpretation of the results of the experiment.
Results
Seven different internal bone structures were defined. The types are illustrated in . The herring pattern (type 1) shows elliptical, elongated or linear lacunae in a dense or dispersed pattern. When elongated or linear, the lacunae are organised parallel to each other. The canaliculae are clearly visible and are branched. The salmon pattern (type 2) consists of elliptical or elongated lacunae, moderate dispersed. The border of the lacunae is irregular due to the visibility of only the basis of the canaliculae. A parallel organisation of the lacunae is visible. The tuna pattern (type 3) is based on an irregular zigzag pattern of elliptical or elongated lacunae. Canaliculae are hardly or not visible. The cod pattern (type 4) shows long linear lacunae in a parallel pattern. Canaliculae are not visible. Without air inclusion the lacunae are hardly visible as well. Type 5 is based on a, mostly dense, pattern of circular micro-lacunae. Canaliculae are not visible. Micro-lacunae are well discriminated from the lacunae in the other types by their size: < 5 μm versus (much) > 10 μm. A pattern of cross-pigmentation in linear bones (type 6) is visible in material primarily originating from flat fish (order Pleuronectiformes). Acellular bones, without any visible structure of lacunae and canaliculae (type 7), are found in a range of fish species.
Figure 1. Seven types of bone fragments in teleost fish: a, b: type 1 (herring and anchovy); c: type 2 (trout); d, e: type 3 (tuna and mackerel); f: type 4 (cod); g: type 5 (plaice); h: type 6 (flounder); and i: type 7 (sea robin). Bar = 50 µm.
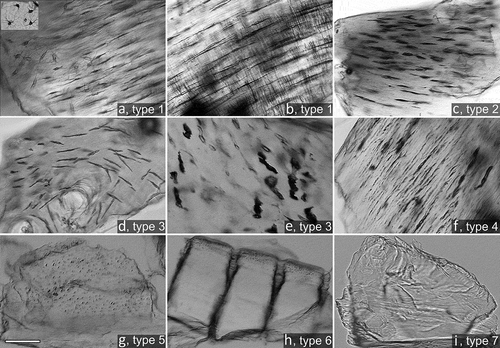
A pattern of orthogonal lines can be visible in fragments from a variety of species. Fragments showing such a pattern are assigned to a residual category, since such fragments can originate from either scales or from otholiths. The number of fragments in this extra category was chosen to be included in the calculation of the frequency distributions per species. The share of this extra category was < 10% in all cases.
The overview of the distribution of the seven categories of bone types over the total set of 17 species is shown in . There is a clear distribution of certain bone types among teleost fish orders. Type 1 (herring and sardine) is present with high shares in the frequency profiles of species of the Anguiliformes and Clupeiformes. Type 2 (salmon) is dominant in the trout profile, as representative of the Salmoniformes. Some of the other types are linked to one of the other orders, but at much lower shares in the frequency profiles. Type 4 (cod) is primarily found in the species of the orders Gadiformes and Perciformes at levels around 20%, and hardly in the other species as investigated. The segmented bone fragments (type 6) primarily occur in the species belonging to the order Pleuronectiformes and are predominantly present in the subsamples with tail material. There is a co-occurrence of type 5 (micro-lacunae) with the presence of bone fragments without any visual structure (type 7). Only in anchovy is the presence at a level of 40% of type 7 bone fragments not accompanied by a considerable share of fragments with micro-lacunae. The residual type did not have a share > 10% in any of the profiles.
Figure 2. (colour online) Profiles of seven fish bone types for 17 different species. Indications of orders are arranged according to evolutionary development.
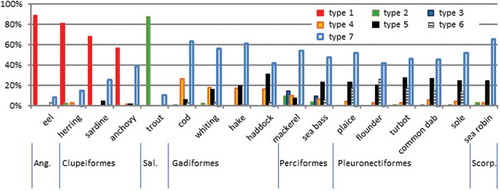
The sum of quantities of types 1–5 represents the total amount of cellular bone fragments, whereas the quantities of types 6 and 7 represent the portion of acellular fragments. The distribution of the portions of cellular and acellular bones of the investigated species is shown in . Evidence for a full absence or presence of one of these two portions was not found. A majority of bones (over 60%) appeared to be cellular in the representatives of the orders of Anguiliformes, Clupeiformes and Salmoniformes. The species belonging to Pleuronectiformes and Scorpaeniformes showed over 60% of acellular bones. Intermediate amounts were taken by species of the orders Gadiformes and Perciformes. Here the portions of acelluar bone fragments were between 45% and 66%, with a share of cellular bone fragments over 33%.
Figure 4. Different types of gill filament fragments: (top) anchovy, (bottom) cod. Note the presence of the distinct palisade layer (top image). Bar = 50 µm.
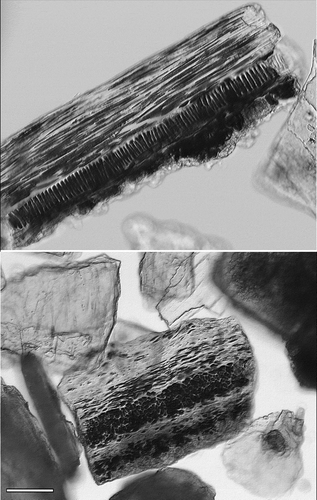
Figure 5. Share of cellular (types 1–5) and acellular (types 6 and 7) fish bone types for 17 different species. Indications of orders are arranged according to evolutionary development.
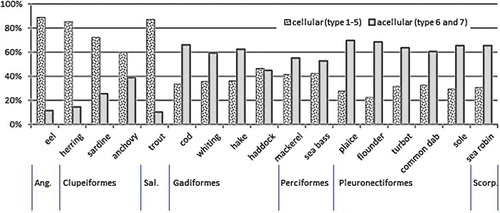
Three basic types of teleost fish scales were recognised. The clupoid type shows growth rings that have an approximate linear structure. The cycloid type is characterised by concentric growth rings. In the gadoid type the concentric rings are intermittent, which results in a radial pattern. Intermediate types are found between clupoid and cycloid, and between cycloid and gadoid. A special type is characterised by large teeth at the basal side of the scale. This feature is mostly superimposed on the cycloid/gadoid type in specific cases. The several types are illustrated in .
Table 2. Scale types and their distribution over the teleost fish orders represented in this study.
As for bone fragment types, the scale diversity is preferentially distributed over the examined teleost fish orders. Within one species only one scale type occurs. The distribution of the scale types is presented in . The parallel growth rings (clupoid type) are found in some species of Clupeiformes. Cycloid scales are found in species belonging to the orders Salmoniformes and Perciformes. The cycloid/gadoid type is found in most representatives of the orders Perciformes, Pleuronectiformes and Scorpaeniformes. The teeth as indication of the ctenoid type are present in selected species from several different orders. These scale ‘teeth’ show a principal other appearance than the real teeth of fish. Some teleost fish orders, most notably the Perciformes, show several scale types among their representatives.
The extracted gill filaments show a dense pattern of cellular structures. The observations revealed an organisation of layers with different characteristics. The filaments are tapering towards the end and some layers are gradually vanishing. The filaments of the representatives of the Clupeiformes show three layers in the ventral part, reducing to two layers in the dorsal end. The filament organisation of sardine is shown in . A clear characteristic is the presence of a layer of palisade cells. Although three layers can be recognised in the filaments of the species belonging to the orders Gadiformes, Pleuronectiformes and Scorpaeniformes as well, the middle layer shows another, more randomly, organised appearance.
Four mixed fish meals from two fishing regions in the world were investigated with respect to recognisability using stained and unstained sediment material. Two slides of each of the fish meals with a known number of particles (a priori count) were investigated blind to recover the fish bone fragments from a mixture with 90% minerals. The results are shown in . The recovery percentage of the fish meals originating from the Southern Pacific is acceptable or good. In contrast, the recovery of the meal originating from the Northern Atlantic is < 65%. Examination of the bone fragments revealed a high share of acellular (type 7) bone fragments in the fish meals originating from the North Atlantic. The use of stained segment material resulted in recovery rates without a clear relationship with the type of material nor with the recovery as achieved with unstained material. The sodium hypochlorite treatment, as part of the staining procedure, resulted in a high friability and, hence, shattering of the fragments. It appeared to be impossible to count the shattered fragments of the weighted 0.5 mg. As an alternative, a series of bone fragments was selected representing the 0.5 mg per slide. Even then, the handling of the material resulted in some fragmentation. Notwithstanding these complications, the standard deviations for the a posteriori counts (i.e., with minerals added) of the stained material were comparable with those of the unstained material. Two control counts of the mineral mix used to prepare the a posteriori samples revealed that approximately 1% of the mineral particles appeared to be stained. The number of stained particles with a clear mineral origin in the eight samples, as revealed in the a posteriori examination and representing 4.5 mg of mineral mix, appeared to be between 25 and 49 for technician 1 and between 24 and 40 for technician 2.
Table 3. Recovery of fish bone fragments in mixtures of mineral:fish of 9:1 with four different fish meals. Results are shown for unstained material and material stained with alizarin. Type means share of acellular bone fragments.
Discussion
Definition of types
Some basic bone types, of which the characteristics are found in herring, sardine, tuna, mackerel and some species not included in the current set of species, were described by Voehringer (Citation1958). The current inventory of a larger set of species resulted in a larger diversity in bone structural elements. Some bone types include a diversity in appearance, although the basic characteristic per type is clear. Type 1 (herring, sardine and relatives) is defined by the clearly visible and branched canaliculae, although the shape of the lacunae shows a large diversity from elliptical (herring) to almost linear (sardine). The elongated shape was found in herring bone fragments as well (). The co-occurrence of the elliptical and the elongated lacunae in herring bone fragments approves that this diversity belongs to one type. Type 3 (tuna and mackerel) is characterised by an irregular zigzag pattern of lacunae. At the same time these lacunae show a larger diversity from an elongated shape with a clear border (tuna) to a disorderly shape (mackerel). Also the bone fragments of type 6, or more precise the fin ray elements, show a large diversity in segmentation patterns. The bone fragments included in type 7 show still a large diversity ranging from translucent particles with clear borders to irregular, frayed or fibrous pieces. In this way type 7 seems to be a collection of fragments not otherwise typified. On the other hand, if any structural feature in the form of only a few lacunae in a fragment can be confirmed, a proper classification can be achieved. The effect is that type 7 fragments did not reach a share of more than 65% in any of the profiles.
Several authors report the existence of micron-sized tubules in fish bones (Kölliker Citation1859; Hughes et al. Citation1994; Atkins et al. Citation2015). The appearance of these structures (Atkins et al. Citation2015, figure 3) might be comparable with the here described types 4 (longitudinal view: linear lacunae in cod and relatives) and type 5 (transverse view: micro-lacunae). It can be expected that both longitudinal and transverse views of the same structure would be encountered in more or less equal frequencies when examining particles randomly. However, type 5 with micro-lacunae takes a dominant share among the cellular bone types in the species belonging to Perciformes, Pleuronectiformes and Scorpaeniformes, with mackerel as only exception (). In several studies selected bones were used for investigating biomechanical and histological properties. For example, Cohen et al. (Citation2012) and Atkins et al. (Citation2015) selected the operculum for their experiments, vertebrae were studied by Cao et al. (Citation2011), and mandibles were studies by Hughes et al. (Citation1994). The necessary targeted analysis of one bone type will not provide an overview of diversity within a specimen, since this was not the goal in these studies. The aim of the current investigations, being the identification of ground and, therefore, randomised material of fish, is quite different from those studies into fish bone properties. Elaborated studies are recommended both for histological features as well as for material properties (Currey Citation2010).
Scales are usually present in commercial fish meals as fragments due to grinding. Distinction between the three principal types, clupoid, cycloid and gadoid, is then hampered by the existence of the two intermediate types. For example, the scale fragment depicted in Makovsky et al. (Citation2011, figure 4.13) can originate either from a gadoid type scale or from a cycloid/gadoid-type scale. Especially the teeth from the ctenoid scale type can be found as clusters in commercial fish meals. The ctenoid scale type is found in a limited set of species, sometimes restricted to adult specimen (e.g., common dab). Together with the additional information obtained from Voehringer (Citation1958) included in , the scale diversity can add to the identification of fish species in fish meals.
Examination of commercial fish meals over a range of years revealed that gill fragments are present in two different structures (): a composition with and a composition without a layer of palisade cells. Fish meals based on herring and relatives and tuna and relatives show the palisade layer (to be indicated as the clupoid type), while cod and relatives show the pattern without the mentioned layer (to be indicated as the gadoid type). Although this principal difference can be related to the current observations of whole gill filaments (), the diversity as found in the entire filaments seems not fully represented in ordinary fish meals. It has to be noted that a dense pattern of cellular structures in gill filaments was found as well in species which were assumed to be acellular.
Acellularity and the sensitivity of microscopic detection of fish meal in compound feed
Information from the literature suggests that a species contains either bones with a visible histological structure or acellular bones (Kölliker Citation1859; Kranenbarg et al. Citation2005). Past studies into the characteristics of fish material used as feed ingredient focus exclusively on the visible histological features (lacunae and canaliculae) or other characteristics such as scales, teeth and otholites (Schweizer Citation1931; Voehringer Citation1958; Makovsky et al. Citation2011). The current study reveals that within a specimen different bone structures can be observed, and that the share of each histological type can vary within a species. The total amount of cellular structures (types 1–5) and that of acellular structures (types 6 and 7) is shown in . In all cases a mixture of both basic types exists. The majority of bone fragments per species assigned in this study to either cellular or acellular types corresponds to the classification of the teleost fish orders with predominant cellular or acellular species, and the tendency of an increase of acellular bone fragments is in line with the evolutionary position of the orders (Kranenbarg et al. Citation2005) (). The current study, however, contradicts assumptions that a fish species possess exclusively either a cellular or an acellular bone structure.
Figure 6. Simplified evolutionary tree of teleost fish, based on Near et al. (Citation2012) and Broughton et al. (Citation2013). Rectangle sizes indicate the relative portion of species per group, with the share of cellular species (left, dashed) and acellular species (right, blank) after Kranenbarg (2005). The Ostariophysi is a paraphyletic superorder according to molecular evidence, but is maintained here for its relation with data on (a) cellular bone occurrence.
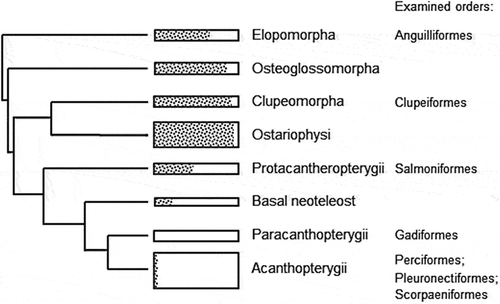
The samples used for examining the recognisability of fish particles in the sediment () represent the fishing regions south-east Pacific and north-east Atlantic, which are the main producing areas of fish meal (FAO Globefish Citation2016). The blind examination of these four fish meals () showed a quite strong connection between acellularity and recovery. The recovery of 105.5% of fish bones in slide D2 showed that a small share of false-positive conclusions on a fish identity can exist, i.e., that some mineral particles were erroneously identified as fish. Presumably this erroneous identification of mineral particles as fish occurs in all cases, also at recovery levels < 100%. After staining with alizarine, generally large differences appeared to exist among the duplicate analyses of the individual samples. This could be caused by the fragmentation as methodological artefact of the handling of the fragments. Considering a high number of particles (e.g., sample D1: 181.5 particles on average) as a sign of fragmentation, a correlation between the a priori mean of the number of particles and the recovery could be assumed. However, this correlation is only −0.34. The smallest difference between two duplicates after staining was found for sample B (Norway). The mean recovery over two duplicates was 60.7% (unstained) versus 84.5% (stained). This could indicate that recognition was improved after applying alizarine staining. Nevertheless, this was not found for the other samples. Alizarine is also used as a stain to indicate calcium carbonate minerals such calcite and aragonite (Green Citation2001). Since such minerals can also be present in mineral mixtures, confusion might be an issue. The overall results and information concerning staining reveals that further testing and validation of staining as part of official monitoring is recommended. In terms of recognising fish meal particles, the use of a binocular would assist in the first phase of the examination of a sediment. The application of a binocular is mentioned in the official procedure (European Commission Citation2013b). It is particularly useful for the detection of fish meal material generally lacking microstructures (i.e., type 7 fragments), since examinations at low magnifications can provide information at the level of the entire bone fragments as described by van Raamsdonk et al. (Citation2012a).
Assuming a share of 50% acellular but still recognisable bone fragments (type 7) in an ordinary fish meal, then a spike percentage of 0.1% of that fish meal in a compound feed would result in an effective percentage of 0.05%. Past results in proficiency tests showed that spike levels of 0.1% generally did not result in low specificity scores (). A large study on quantification of fish meal in compound feeds gave also good specificity scores (Veys & Baeten Citation2008). However, in the presence of 0.1% meat and bone meal (MBM) a suboptimal result was found at the spike level for fish of 0.1% (). At higher spike levels the sensitivity of fish meal detection is good to perfect under several circumstances (), implying that detection of fraud, i.e., illegal use of fish meal at economically beneficial levels, is fully achievable. Shares of acellular bone fragments apparently do not have a considerable influence on the detection at those higher spike levels. Investigation of six fish feeds containing fish meals did not show principal differences for a range of lacuna characteristics, although geographical origin nor a discussion of acellular bone fragments was given (Pinotti et al. Citation2016). The lack of large differences among the six samples might possibly be due to relatively high shares of cellular bone fragments in the fish feeds.
Table 4. Specificity (column indicated with 0%) and sensitivity (columns indicated with percentages above 0%) related to fish meal detection in proficiency tests over a period of six years, organised by EURL AP or RIKILT on behalf of IAG section feed microscopy. EURL results are recalculated to a ‘zero tolerance’ approach. EURL and IAG reports are referenced by their first author, Veys and van Raamsdonk, respectively.
Mimics of fish bone fragments
The results of proficiency tests () show that two major sources of mimicking fragments exist. A considerable part of the participants in proficiency tests erroneously report terrestrial animal material in the presence of salmon meal (Veys et al. Citation2011: 35%; van Raamsdonk et al. Citation2012b: 30%). Certain fragments originating from salmon can be erroneously identified as terrestrial animal material due to the shape of the lacunae, which can be oval with a visible basis of some canaliculae ()).
Hydrolysed feather meal partly consists of pieces of transparent material without internal structural elements, which might mimic acellular fish bone fragments. Proficiency tests revealed confusion (). A total of 31% of the participants did not detect 0.5% of hydrolysed feather meal in fish feed containing fish meal (Veys et al. Citation2012), and a number of the participants in two tests erroneously reported fish meal in two different matrices containing feather material (van Raamsdonk et al. Citation2011: 12%; Veys & Berben Citation2013: 25%). Assuming that bodies of fully hydrolysed feather meal mimic acellular fish bone fragments, these results imply that the participants are aware of the existence of acellular bone fragments. Nevertheless, it remains necessary that proper distinction has to be made between hydrolysed feather meal and fish bone fragments.
The experiment with four 1:9 fish:mineral mixtures () revealed that confusion between acellular fish bone fragments and mineral particles can occur. In the absence of internal structures such as lacunae and canaliculae, the external characteristics (shape, transparency) provide the only information for identification.
Implications for detection of fish meals in compound feeds
Caught mixtures of fish meals used as ingredient in fish feed are exempted from the species-to-species ban, even if material from the target fish species in the fish farm might be present in the mixture. However, by-products from fish farms are prohibited as feed ingredient in feed intended for the same species (European Commission Citation2011). It is therefore necessary to have rapid tools for fish identification in feed. Monitoring programmes for feed are installed by member states of the European Union for a variety of reasons and detection of fish material is routine practice.
The characteristics of bone and scale fragments as found in commercial fish meals can be attributed to the diversity described in this study pertaining to bone and scale structures. The combination of specific features from bone and scale fragments might give the opportunity to identify fish material primarily at the level of orders. The two basic types of gill filaments as usually found in fish meals are apparently only a fraction of the diversity found after investigating full gill racks. In specific cases further discrimination might be possible based on specific features of bone and scale fragments, i.e., between herring and sardine/anchovy, tuna versus mackerel, and species having ctenoid scales. Non-specific combinations of characteristics not found in one species might point to a mixture of two or more species. Examples are the co-occurrence of type 1 bone fragments (herring, sardine and relatives) with non-clupoid scale elements, of micro-lacunae (type 6) together with either type 1 or type 2 bone fragments or with clupoid-scale elements, and the combination of clupoid and gadoid-scale elements. Due to the mixed profiles for all species included in this study, traces of types of bone fragments might hamper the identification of mixtures. The matrix of characteristics versus species is implemented in a data model running in the expert system Determinator (van Raamsdonk et al. Citation2012c) in order to facilitate identification.
Conclusions
The current study reveals that in an overview of 17 species originating from seven teleost fish orders, none of the species showed exclusively cellular or acellular bone structures. Especially micro-lacunae are found to be present in a part of the bone fragments of the species belonging to teleost fish orders indicated to possess acellular bone structures. The application of the entire set of characteristics of bone and scale fragments allows one to identify fish species at the level of orders. In addition, combination of specific features which are not attributed to a single species might point to a mixture of species. A high share of acellular bone fragments in commercial fish meals resulted in recovery rates < 65%. Alizarine staining of sediments did not result in reliably higher recovery rates. It is recommended to document the alizarine staining further before the staining procedure can be validated as part of official control.
The expertise gained in this study will help to improve the distinction between fish meal and terrestrial animal material in compound feed, and to support the application of the species-to-species ban with respect to the valorisation of by-products from fish farms. In a broader perspective, the current expertise might be helpful to detect fraud throughout the feed and food production chain.
Acknowledgments
The authors thank Dr L. van der Geest, Dr J. de Jong and Dr A. Peijnenburg for their valuable comments.
Disclosure statement
No potential conflict of interest was reported by the authors.
Additional information
Funding
References
- Atkins A, Reznikov N, Ofer L, Masic A, Weiner S, Shahar R. 2015. The three-dimensional structure of anosteocytic lamellated bone of fish. Acta Biomat. 13:311–323.
- Broughton RE, Betancur-R R, Li C, Arratia G, Ortí G. 2013. Multi-locus phylogenetic analysis reveals the pattern and tempo of bony fish evolution. PLOS Curr Tree Life. 5. doi: 10.1371/currents.tol.2ca8041495ffafd0c92756e75247483e.
- Cao L, Moriishi T, Miyazaki T, Iimura T, Hamagaki M, Nakane A, Tamamura Y, Komori T, Yamaguchi A. 2011. Comparative morphology of the osteocyte lacunocanalicular system in various vertebrates. J Bone Miner Metab. 29:662–670.
- Cohen L, Dean M, Shipov A, Atkins A, Monsonego-Ornan E, Shahar R. 2012. Comparison of structural, architectural and mechanical aspects of cellular and acellular bone in two teleost fish. J Exp Biol. 215:1983–1993.
- Currey JD. 2010. Mechanical properties and adaptations of some less familiar bony tissues. J Mech Behav Biomed Mat. 3:357–372.
- European Commission. 2011. Commission Regulation (EU) No 142/2011 of 25 February 2011 implementing Regulation (EC) No 1069/2009 of the European Parliament and of the Council laying down health rules as regards animal by-products and derived products not intended for human consumption and implementing Council Directive 97/78/EC as regards certain samples and items exempt from veterinary checks at the border under that Directive. Off J Eur Commun. L 54:1–254.
- European Commission. 2013a. Commission Regulation (EU) No 56/2013 of 16 January 2013 amending Annexes I and IV to Regulation (EC) No 999/2001 of the European Parliament and of the Council laying down rules for the prevention, control and eradication of certain transmissible spongiform encephalopathies Text with EEA relevance. Off J Eur Commun. L 21:3–16.
- European Commission. 2013b. Commission Regulation (EU) No 51/2013 of 16 January 2013 amending Regulation (EC) No 152/2009 as regards the methods of analysis for the determination of constituents of animal origin for the official control of feed. Off J Eur Commun. L 20:33–43.
- FAO Globefish. 2016. Globefish highlights, annual issue including 2015 statistics [Internet]. [cited 2016 Apr]. Available from: http://www.fao.org/3/a-i5803e.pdf.
- Gizzi G, van Raamsdonk LWD, Baeten V, Murray I, Berben G, Brambilla G, Von Holst C. 2003. An overview of tests for animal tissues in animal feeds used in the public health response against BSE. In: risk analysis of BSE and TSEs: update on BSE and use of alternatives to MBM as protein supplements. Rev Scitech Off Int Epiz. 22:311–331.
- Green OR. 2001. A manual of practical laboratory and field techniques in palaeobiology. Berlin (Heidelberg): Springer; p. 56.
- Hohmann B. 2006. Mikroskopische Untersuchung pflanzlicher Lebensmittel und Futtermittel. Hamburg: Behr Verlag.
- Hughes DR, Bassett JR, Moffat LA. 1994. Histological identification of osteocytes in the allegedly acellular bone of the sea breams Acanthopagrus australis, Pagrus auratus and Rhabdosargus sarba (Sparidae, Perciformes, Teleostei). Anat Embryol. 190:163–179.
- Kölliker A. 1859. On the different types in the microscopic structure of the skeleton of osseous fishes. Proc Biol Sci. 1859:1–14.
- Kranenbarg S, van Cleynenbreugel T, Schipper H, van Leeuwen J. 2005. Adaptive bone formation in acellular vertebrae of sea bass (Dicentrarchus labrax L.). J Exp Biol. 208:3493–3502.
- Liu X, Han L, Veys P, Baeten V, Jiang X, Dardenne P. 2011. An overview of the legislation and light microscopy for detection of processed animal proteins in feeds. Micr Res Techn. 74:735–743.
- Makovsky J, Vary N, McCutcheon M, Veys P. 2011. Microscopic analysis of agricultural products. Urbana (IL): AOCS Press.
- Margry RFJM, de Jong J. 2012. Detection, identification and quantification of processed animal proteins in feedingstuffs. Namur: Les éditions namuroises. Chapter 1, Feed chain: Processed animal proteins and their use in animal feed; p. 9–16.
- Near TJ, Eytan RI, Dornburg A, Kuhn KL, Moore JA, Davis MP, Wainwright PC, Friedman M, Smith WL. 2012. Resolution of ray-finned fish phylogeny and timing of diversification. Pnas. 109:13698–13703.
- Pinotti L, Ottoboni M, Caprarulo V, Giromini C, Gottardo D, Cheli F, Fearn T, Baldi A. 2016. Microscopy in combination with image analysis for characterization of fishmeal material in aquafeed. An Feed Sci Techn. 215:156–164.
- Schweizer G. 1931. Mikroskopische Bilder der wichtigsten Futtermittel tierischer Herkunft. Stuttgart: Verlag von Eugen Ulmer.
- Shahar R, Dean MN. 2013. The enigmas of bone without osteocytes. Bonekey Rep. 2. Article number: 343. doi:10.1038/bonekey.2013.77.
- van Raamsdonk LWD, Pinckaers VGZ, Scholtens IMJ, Prins TW, van der Voet H, Vliege JJM. 2014. IAG ring test animal proteins 2014. Report 2014.011. Wageningen: RIKILT; p. 47.
- van Raamsdonk LWD, Pinckaers VGZ, Vliege JJM. 2012b. Animal proteins in feed. IAG ring test 2012. Report 2012.009. Wageningen: RIKILT; p. 39.
- van Raamsdonk LWD, Pinckaers VGZ, Vliege JJM. 2013. Animal proteins in feed. IAG ring test 2013. Report 2013.016. Wageningen: RIKILT; p. 35.
- van Raamsdonk LWD, Pinckaers VGZ, Vliege JJM, van Ruth SM. 2011. Animal proteins in feed. IAG ring test 2011. Report 2011.015. Wageningen: RIKILT; p. 42.
- van Raamsdonk LWD, Pinotti L, Veys P, Campagnoli A, Paltanin CL, Belinchón Crespo C, Jørgensen JS. 2012a. Detection, identification and quantification of processed animal proteins in feedingstuffs. Namur: Les éditions namuroises. Chapter 6: Markers for microscopic detection; p. 59–69.
- van Raamsdonk LWD, van de Rhee N, Scholtens IMJ, Prins TW, Vliege JJM, Pinckaers VGZ. 2015. IAG ring test animal proteins 2015. Report 2015.016. Wageningen: RIKILT; p. 32.
- van Raamsdonk LWD, van de Rhee N, Scholtens IMJ, Prins TW, Vliege JJM, Pinckaers VGZ. 2016. IAG ring test animal proteins 2016. Report 2016.008. Wageningen: RIKILT; p. 31.
- van Raamsdonk LWD, van der Vange S, Uiterwijk M, Groot MJ. 2012c. Dr. Chiang Jao (ed.), Decision support systems reliability and evaluation of identification models exemplified by a histological diagnosis model. Rijeka: Intech. ISBN 980-953-307-529-2.
- Veys P, Baeten V. 2008. CRL-AP interlaboratory study 2007. Final report. Gembloux (Belgium): CRL-AP, CRA-W.
- Veys P, Baeten V, Berben G. 2014. EURL-AP proficiency test microscopy 2013. Final version. Gembloux (Belgium): European Union Reference Laboratory for Animal Proteins in feedingstuffs, Walloon Agricultural Research Centre.
- Veys P, Baeten V, Berben G. 2015. EURL-AP proficiency test microscopy 2014. Final version. Gembloux (Belgium): European Union Reference Laboratory for Animal Proteins in feedingstuffs, Walloon Agricultural Research Centre.
- Veys P, Berben G. 2013. EURL-AP proficiency test microscopy 2012. Final version. Gembloux (Belgium): European Union Reference Laboratory for Animal Proteins in feedingstuffs, Walloon Agricultural Research Centre.
- Veys P, Berben G, Baeten V. 2011. EURL-AP interlaboratory study microscopy 2010. Final version. Gembloux (Belgium): European Union Reference Laboratory for Animal Proteins in feedingstuffs, Walloon Agricultural Research Centre.
- Veys P, Berben G, Baeten V. 2012. EURL-AP proficiency test microscopy 2011. Final version. Gembloux (Belgium): European Union Reference Laboratory for Animal Proteins in feedingstuffs, Walloon Agricultural Research Centre.
- Veys P, Fumière O, Marien A, Baeten V, Berben G. 2016. Combined microscopy-PCR EURL-AP proficiency test 2015. Final version. Gembloux (Belgium): European Union Reference Laboratory for Animal Proteins in feedingstuffs, Walloon Agricultural Research Centre.
- Voehringer H. 1958. Die mikroskopische Untersuchung von Fishmehlen auf Echtheit und Reinheit [ Dissertation]. Hohenheim: Landwirtschaftlichen Hochschule.