ABSTRACT
In the framework of sustainability and a circular economy, new ingredients for feed are desired and, to this end, initiatives for implementing such novel ingredients have been started. The initiatives include a range of different sources, of which insects are of particular interest. Within the European Union, generally, a new feed ingredient should comply with legal constraints in terms of ‘yes, provided that’ its safety commits to a range of legal limits for heavy metals, mycotoxins, pesticides, contaminants, pathogens etc. In the case of animal proteins, however, a second legal framework applies which is based on the principle ‘no, unless’. This legislation for eradicating transmissible spongiform encephalopathy consists of prohibitions with a set of derogations applying to specific situations. Insects are currently considered animal proteins. The use of insect proteins is a good case to illustrate this difference between a positive, although restricted, modus and a negative modus for allowing animal proteins. This overview presents aspects in the areas of legislation, feed safety, environmental issues, efficiency and detection of the identity of insects. Use of insects as an extra step in the feed production chain costs extra energy and this results in a higher footprint. A measure for energy conversion should be used to facilitate the comparison between production systems based on cold- versus warm-blooded animals. Added value can be found by applying new commodities for rearing, including but not limited to category 2 animal by-products, catering and household waste including meat, and manure. Furthermore, monitoring of a correct use of insects is one possible approach for label control, traceability and prevention of fraud. The link between legislation and enforcement is strong. A principle called WISE (Witful, Indicative, Societal demands, Enforceable) is launched for governing the relationship between the above-mentioned aspects.
GRAPHICAL ABSTRACT
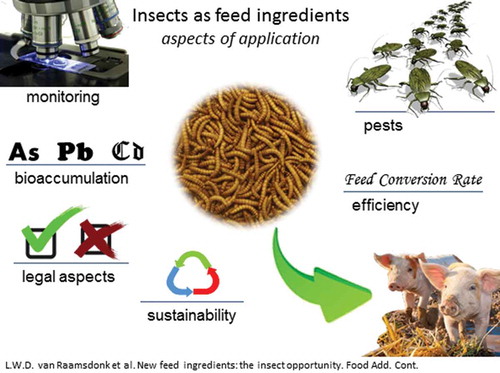
Introduction
There is a growing interest for insects as an alternative protein source for feed production. Insects can supplement traditional feed sources such as soy, maize, grain and fishmeal. Insects with the largest immediate potential for large-scale feed production are black soldier fly (Hermetia illuscens) larvae, common housefly (Musca domestica) larvae, house crickets (Acheta domesticus) and the yellow mealworm (Tenebrio molitor) (Veldkamp et al. Citation2012). Producers in China, South Africa, Spain and the United States are already rearing large quantities of flies for aquaculture and poultry feed by bioconverting organic waste (FAO Citation2013).
Figure 1. Trend lines for feed-conversion rates for several farmed animals. X-axis = year; y-axis = feed-conversion rate (kg feed/kg body weight gain).
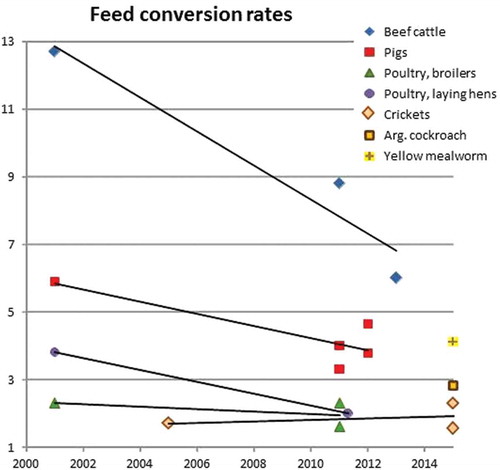
Insects are a group of very diverse animals, belonging to the phylum Arthropods. They take their share in a large array of different animals (see Appendix A) serving at several stages of the feed and food production chain. The systematic position and mutual relationship influence legislation and usefulness when used as a protein source. There are approximately 1.5 million animal species, of which the insects comprise more than two-thirds (950,000). Estimates of yet undescribed insects range from 2.6 million to 7.8 million species (Vié et al. Citation2009). More than 1900 species are used as traditional human food source (Huis Citation2013). This number and diversity is an indication of the complexity when applying insects in the feed and food production chain.
Insects are assumed to be incapable of expressing prions. In cases of contained cultivation prions are currently not considered an issue, but the related legislation does apply. One major fundament of current legislation on the ban of animal proteins is the principle of ‘prohibit, unless’. This precautionary approach has a relevant basis in the bovine spongiform encephalitis (BSE) crisis and subsequent legislation. Insects are not specifically mentioned in the legislation and, therefore, being animal proteins in a broad sense, prohibited in animal feed (Spiegel et al. Citation2013; EFSA Citation2015). Krill, as group of animals related to insects (see Appendix A), is nevertheless allowed in feed since it is included in the definition of fishmeal (Regulation (EC) 142/2011: ‘“fishmeal” means processed animal protein (PAP) derived from aquatic animals, except sea mammals’).
The application of insects in feeds has several goals, such as optimal feed conversion (Oonincx et al. Citation2015), use of alternative substrates and specifically produced products such as insect proteins, fat fractions and chitin (Veldkamp et al. Citation2012). Different insect species have different protein levels, and the amino structures also vary widely (Makkar et al. Citation2014; Oonincx et al. Citation2015). It is necessary to review these opportunities in the framework of applicable legislation, environmental constraints and monitoring requirements.
Several reviews and overviews of specific aspects related to the utilisation of insects as feed ingredient have been published recently (Veldkamp et al. Citation2012; Belluco et al. Citation2013; FAO Citation2013; Spiegel et al. Citation2013; Makkar et al. Citation2014; EFSA Citation2015). This paper aims to discuss the issues related to insects as feed material in the area of legal constraints, feed safety, sustainability, environmental impacts and monitoring, with a specific focus on the relation between these five aspects.
Framework
In 2015, the European Commission (EC) started a large programme for stimulating a circular economy with implications for a range of market sectors, agriculture amongst them. One of the goals is to ‘take measures to clarify European Union (EU) legislation relating to waste, food and feed and facilitate food donation and the use of former foodstuff and by-products from the food chain in feed production without compromising food and feed safety’.Footnote1 The mentioned measures focus on valorisation as much as possible, and include, in this order, prevention of waste, reuse of material, recycle material and energy recovery up to disposal (Directive 2008/98/EC). This study will translate these goals and prospects to the specific topic of rearing insects as feed material for animal feeding and to recycling commodities at the highest level of value.
The term ‘commodity’ – including but not restricted to feeding stuffs (general food law – GFL), feed materials (feed catalogue) or substrates (EFSA opinion on insects – is used here to indicate every material that can theoretically be considered feed for farmed animals, whether or not covered in current legislation.
Legal aspects
This section briefly lists the general safety requirements for feed materials, followed by the specific restrictions for animal by-products. The main EU legislation for the entire food production chain is the GFL (Regulation (EC) 178/2002). It is the basis for general principles in the area of food safety, for the establishment of the EFSA and it applies to production, processing and distribution. Food as well as feed are defined separately, and specific categories such as medicinal products and narcotics, cosmetics, tobacco and contaminants are excluded from the definitions.
Based on this general fundament, several other legal documents are in force in the EU. In principle, several categories of hazards are regulated for feed materials. These include:
Chemical hazards: Directive 2002/32/EC addresses a range of undesirable substances, such as the classical pesticides, fluorine, heavy metals, gossypol and dioxins. Another regulation applies to residues of plant protection products (Regulation (EC) 396/2005).
Chemical hazards from plant origin: the presence of several plant materials such as seeds from Ricinus, Jatropha, Datura and Brassica in feed and feed materials is regulated according to Directive 2002/32/EC.
Microbiological hazards: rules on hygiene are implemented by Regulation (EC) 183/2005, including those for feed material and feed business operators. The principle of good practice is laid down in this regulation as well.
Physical hazards: several materials that could cause physical damage to animals, such as household and solid urban waste and packaging materials in former food products intended as feed materials, are prohibited according to Regulation (EC) 767/2009.
Combined hazards: wood treated by wood preservatives, hide treated with tanning substances, contents of digestive tracts and faeces are prohibited (Regulation (EC) 767/2009). These hazards combine a specific type of material with a chemical compound.
This set of safety requirements applies to a range of feeding stuffs as defined in the referred legislation. A range of different feed materials is listed in the feed catalogue (Regulation (EC) 68/2013). This list is not compulsory or conclusive, but when a listed feed material is being used it should comply with the specific definition in the catalogue and the good practice rules should be followed. The definition of new feed materials as well as the procedures for their inclusion in the catalogue are defined in Regulation (EC) 767/2009. So far feed materials are allowed to enter the feed chain with the requirement that legal limits are not exceeded. This can be described as a principle ‘yes, provided that’ a range of constraints are met. These constraints are discussed for novel feed materials such as seaweed (Burg et al. Citation2013) and several other novel feed materials (Spiegel et al. Citation2013). In the case of animal products, insects among them, an extra set of requirements apply following the ban on the use of PAPs. These regulations are based on a few general bans, for which ranges of exemptions and derogations are installed. This can be indicated as the principle ‘no, unless …’.
The set of bans on the use of PAPs consists basically of three parts:
Feeding of ruminants with PAPs is prohibited (Regulation (EC) 999/2001, article 7, part 1). As derogation fish meal may be used as a milk replacer for unweaned ruminants (Regulation (EC) 999/2001, article 7, part 3).
Feeding of PAPs originating from a species (or group) may not be fed to the same species (or group) (species-to-species or anti-cannibalism ban; Regulation (EC) 1069/2009, article 11). Exemptions are fur animals and the utilisation of caught fish in fish feed (Regulation (EC) 1069/2009, article 11, parts a and d, respectively).
Prohibition of feeding PAPs is extended to other animals (Regulation (EC) 999/2001, article 7, part 2 and annex IV). This is commonly indicated as the extended feed ban. A large range of very specific exemptions and derogations is in force. Annex IV of Regulation (EC) 999/2001 lists a series of additional prohibitions (part I) and a series of derogations (part II).
Regulation (EC) 999/2001 considers processed insectsFootnote2 as PAPs for the simple reason that insects are currently not specifically exempted. This should imply that using insects as feed material for other farmed animals is prohibited. The transmissible spongiform encephalopathy (TSE) road map 2 of the EC (2010–15) would suggest otherwise, but insects are not specified in that document either (see the comment in Spiegel et al. Citation2013). The feed catalogue makes a distinction between animal by-products of warm-blooded animals and terrestrial invertebrates; PAPs are indicated as originating from animal by-products of warm-blooded animals (see Appendix B). In addition, crustaceans and krill are listed as separate feed materials.
Another principal aspect is the classification of animal proteins in three categories (Regulation (EC) 1069/2009). Short indications of these categories are:
Category 1 (article 8): ill animals, treated animals, specified risk material, material collected from waste water, catering waste originating from international transport.
Category 2 (article 9): manure and gut content, treated animals and material collected from waste water as far as not listed as category 1 material, foetuses, dead-in-shell poultry, fallen stock.
Category 3 (article 10): basically all by-products from the production of products fit for human consumption, hides and skins, eggs and egg material, blood, raw milk, feather material, pig bristles, all originating from healthy animals, products and by-products from aquatic animals without any signs of sickness, certain types of catering waste, with the exemption of all materials listed as categories 1 or 2 material. Aquatic animals and their by-products are listed under parts (i) and (j), and insects are part of the indication of aquatic and terrestrial invertebrates (l). This means that crustaceans can either be classified as aquatic animals or as aquatic invertebrates.
There are seven procedures for processing PAPs from animal by-products (Regulation (EC) 142/2011, annex IV, chapter III). Material of mammalian origin needs to be treated according to procedure 1: particles smaller than 50 mm are treated at 133°C for 20 min at at least 3 bar. Treatment procedure 7 is of particular interest. The description of this procedure allows the competent authority to authorise any treatment method, provided that an identification of relevant hazards linked to the starting material has been carried out, that the processing method has been shown to be able to reduce these hazards to acceptable levels, and that the microbiological safety is sufficiently proven. In the case of insects this possibility could imply the development of a suited procedure for processing.
Neither the classification of insect products as category 3 material nor the possibility to apply a specific processing procedure will overrule the prohibition as deducted from Regulations (EC) 999/2001 and (EC) 1069/2009. The major issue is the current absence of ‘insects’ in any derogation of the ban on PAPs in feed, which causes the principal adjective ‘unless’ to have no content. This changes the principle of ‘no, unless ….’ into ‘no.’ without any further derogation. It would be profitable to harmonise the definitions of animal by-products and PAPs as used in the feed catalogue with those in the other regulations. The legislative elements have a major impact on the design of the analytical tools for monitoring.
The EU recently circulated a draft regulation amending Regulation (EC) 999/2001 and Regulation (EC) 142/2011 to allow material of six insect species as an ingredient in fish feed. The mentioned species are black soldier fly (Hermetia illucens), yellow mealworm (Tenebrio molitor), lesser mealworm (Alphitobius diaperinus), house cricket (Acheta domesticus), banded cricket (Gryllodes sigillatus) and field cricket (Gryllus assimilis). These species are already indicated as candidates for large-scale rearing (Veldkamp et al. Citation2012; EFSA, Citation2015). Special emphasis is given in the EU draft regulation to pathogenic effects and invasiveness. A list of allowed insect species for cultivation implies the establishment of a white list. Although stated that insects were allowed in aquafeed from 2013 onwards (Regulation (EC) 56/2013) by Lähteenmäki-Uutela and Grmelová (Citation2016), the main restriction remained to be the obligation that insects should be processed in official and registered slaughterhouses (Regulation (EC) 999/2001, annex IV, chapter IV, section D). The current proposal of the EU now provides an exemption from this obligation for insects.
Safety
Recently, several papers on safety issues related to insects as feed and food ingredient have been published (Belluco et al. Citation2013; Spiegel et al. Citation2013; EFSA Citation2015). The restrictions on the use of animal by-products as feed ingredients are primarily focusing on the hazard of prion diseases.
BSE is the main representative form of TSEs caused by the presence of prions, which are modified forms of host-specific proteins. The pathological form of the prion protein accumulates primarily in nervous system organs because of its resistance to proteolytic enzymes. The epidemiology and background of prions as a feed and food contaminant differ principally from those of chemical or (micro-)biological contaminations: prion diseases always have a progressive and irreversible nature, there is a genetic basis and resistance or a cure does not exist (Prusiner Citation1998; Prince et al. Citation2003; Collins et al. Citation2004).
Elaborate analyses revealed that the most likely route for the infection of susceptible animals is by oral ingestion of prions. These prions originate from animal by-products used as a feed ingredient (Morley et al. Citation2003; Prince et al. Citation2003). Plants such as alfalfa, corn and tomatoes can take up prions, and parts of these plants are capable of infecting mice after injection (Pritzkow et al. Citation2015).
Thackray et al. (Citation2012) have demonstrated that prions (PrP) cannot be expressed in genomes of insects. Lupi (Citation2005, Citation2006) studied the possibility of transferring prions consumed by insects and concluded that dissemination of both scrapie and chronic wasting disease (CWD) appears possible. Larvae of common flesh fly (Sarcophaga carnaria) fed with hamster brain material containing PrPSc appeared to be infectious after consumption by hamsters (Post et al. Citation1999). The probability of insects being a vector of prions depends apparently exclusively on the prions that might be present in their rearing substrate. Commodities based on feed- and food-grade materials can be considered safe, whereas animal by-products and human waste might need precautionary measures. Furthermore, a division can be made between ruminant and non-ruminant origin of feed commodities for rearing insects (EFSA Citation2015).
With respect to other classical categories of hazards (chemical and microbiological) a comparable division can be made: passive transfer from their rearing commodity, active bioaccumulation from their rearing commodity, production of indigenous toxins by the insects themselves, and effects of processing insects to feed materials (see also Veldkamp et al. Citation2012; Belluco et al. Citation2013). As for any other feed ingredient intended for the consumption by the current farmed animals, insects are a step in the food processing chain adding value as well as causing probably specific hazards.
A recent study investigated the possible presence of a wide range of contaminants – amongst others, residues of veterinary drugs and pesticides, mycotoxins, plant toxins, heavy metals, and dioxins – in larvae of four different fly species. The larvae were produced in different geographical locations, with diverse rearing methods, using different waste materials, being manure in most cases (Charlton et al. Citation2015). Levels of the contaminants in the larvae were below recommended maximum concentrations (as suggested by bodies such as the EC, the WHO and Codex) (Charlton et al. Citation2015).
Specific bioaccumulation or sequestration of some heavy metals in selected species was shown for mercury and cadmium in grasshoppers (Devkota & Schmidt Citation2000), and lead in grasshoppers (Handley et al. Citation2007), and for the trace element selenium in yellow mealworm (Hogan & Razniak Citation1991). This active mechanism is also known for seaweed, where arsenic is accumulated most notably in brown algae (Burg et al. Citation2013).
Insects can produce toxins or accumulate toxins from their specific host plant for defence mechanisms, which is considered to be a major factor (Finke et al. Citation2015). Mealworms and other members of the family Tenebrionidae can produce (benzo)quinones and alkenes (Crespo et al. Citation2011). Cyanogenic glycosides are known from moths of the genus Zygaena (Zagrobelny et al. Citation2009). In this respect insects should be considered as any other feed material, since a range of plants can produce these compounds as well (EFSA Citation2012). The group of cyanogenic glycosides is currently the compound of concern with the highest exposure to free grazing animals (van Raamsdonk et al. Citation2015a).
Besides the situation that mycotoxins present in processed insects used as feed material will contribute to the exposure to the targeted species, mycotoxins can affect the insects during rearing as well (McMillian et al. Citation1981). Allergenic activity is also a matter of concern when insects are being consumed by humans (Belluco et al. Citation2013); any reaction of animals is still not reported (FAO Citation2013). Further details as part of a risk assessment of insects are given by EFSA (Citation2015). It is necessary to assess risks and impacts more specifically in case-by-case approaches.
Environment
The impact of rearing insects on the environment has several implications. Principally a food chain or web (in nature as well directed by man) consists principally of one trophic level of organisms which can harbour energy from a non-biological source (primarily sunlight captured by chlorophyll in plants) and which gain in biomass, followed by one or more trophic levels of consuming organisms (herbivores followed by carnivores) which transfer a part of the biomass back into energy (Hui Citation2012). The more levels of consuming organisms there are in a food web, the higher the loss of biomass and the higher the production of greenhouse gases will be. Therefore, in an ideal case, insects are directly used as food, which would maximise the gain because insects are thus not an extra trophic level in the food web but replace another trophic level. The effect on food webs of using insects as feed ingredient will be addressed further below.
The rearing of insects results in a product, waste from the substrate and one or more by-products. Chitin as a by-product of insect protein production could be a high-value source for carbohydrate-rich products (Veldkamp et al. Citation2012). Remains of the substrate have to be processed and recycled, which could result in pollution when used as a fertiliser (EFSA Citation2015).
Several already cultivated insects are considered pests globally or in certain parts of the world (FAO Citation2013). Especially when insects are being reared in regions where they are not indigenous, escape from cultivation can lead to undesired outbreaks (Kumschick et al. Citation2016). In order to ensure that only approved insect species are cultivated, a white list can be designed and gradually extended. A white list seems to be practical, since unexpected issues with non-listed species and groups are automatically addressed as possessing no immediate risk of plagues because these species are already prohibited, and extensions to the list can gradually be achieved. A white list can also help to enforce traceability or label control. The draft regulation as currently proposed by the EC implies the adoption of a white list. The alternative, a black list, would give a range of insects prohibited for rearing in a certain region. This could be difficult to maintain since a large range of insects might be theoretically hazardous for their invasiveness or otherwise, which is hard to predict. Most species on the global list of invasive species of the Invasive Species Specialist GroupFootnote3 only appeared to be invasive afterwards. The choice of maintaining either a white list or a black list has a major impact on the monitoring of using insects.
Sustainability
Basically the use of insects as feed material implies that an extra trophic level in the food production chain or web is plugged in. This extra trophic level costs of energy and produces waste, which has to be compensated for in a certain way.
In order to analyse the effect of using insects as feed ingredient and to compare insects with other farmed animals, the feed-conversion rate (FCR) is used as one out of several possible parameters suited for the purpose. The FCR is the ratio between the feed consumed (kg) and the gain in body weight (kg) for a given animal. Principally, the FCR is larger than 1 for all animals. The inverse is the feed efficiency, which, logically, is below 1 in all cases. Other measures focus on, for example, dry matter conversion and nitrogen efficiency, which can be used as efficiency parameters as well (Oonincx et al. Citation2015). Values for FCRs of nine farmed animals, with four insect species among them, spanning the last 15 years have been collected and analysed. An overview of these FCRs is given in .
Table 1. Feed-conversion rates for a series of farmed animals.
To access the type of animal, the FCR is influenced by age (development stage; e.g., Stender Citation2012). This can be clarified by the growth curve of animals, where young animals gain weight at a much higher speed compared with adult ones. Furthermore, feed composition (e.g., Fahmy et al. Citation1992; Dijkstra et al. Citation2013) and the genetic basis of the animal (Eenennaam Citation2013) are also factors in the optimisation of the physiology of farmed animals. In the last decades much effort has been put into animal breeding for efficiency to convert feed in biomass in order to maximise production (Hayes et al. Citation2013). The effect of the progress in domestication and management systems for farmed animals during the last 15 years is illustrated in , based on data from . It can be assumed that improvements in FCR can be more easily achieved at higher levels of FCR compared with lower levels, since lines will asymptotically approach the ideal FCR of 1. It is also shown that the FCR levels for insects are low compared with older data for classically farmed animals (see also FAO Citation2013, fig. 5.1), but are at levels comparable with the modern levels of pigs and poultry. Ideally, insect domestication could result in even lower levels of FCR.
Consider the following scenarios:
An insect with an FCR of 2 and a target animal, e.g., poultry, with an FCR of 3.
The total consumption of the target animal is 10 kg of feed, either composed of traditional feed materials (scenario A) or of a share of 20% insects (2 kg of the total 10 kg; scenario B).
The final amount of animal product in scenario A is 3.33 kg (10/3), and in scenario B is 3 kg (8/3 + (2/2)/3). In other words, the efficiency of the feed transfer is 9% lower in scenario B. In order to get the original production of 3.33 kg of the target animal, a total amount of 11.11 kg feed is necessary when using insects as step in the feed production.
Based on this example it should be questioned what the benefit is of using insects in the feed production chain in view of increased sustainability. At first, particular reasons for rearing and using insects as feed material can be the production of special proteins for niche markets (e.g., specific amino acid composition), or a better digestibility of the insect proteins compared with those contained in the substrates. Secondly, a low FCR can be achieved when the insects are reared on high-quality substrates. Lundy and Parrella (Citation2015) found an FCR of only 1.3 when rearing house crickets on poultry feed. Relatively low values were found by Oonincx et al. (Citation2015) when using control feeds for four species (). Three of these control feeds were optimised and supplied by the insect farm; in the fourth case poultry feed was used. However, in those cases only a sustainable advantage can be reached when those insects are used directly for human consumption. Used as feed ingredient, the insects are only a biomass consuming an intermediate trophic level between the poultry feed and the poultry. Alternatively, the use of new commodities such as former food products containing meat or fish, by-products not entering the food chain otherwise, catering and household waste containing meat, animal manure and intestine content, gardening and forestry material, and human manure (EFSA Citation2015, summarised by Finke et al. Citation2015; Lähteenmäki-Uutela & Grmelová Citation2016) would result in high-value proteins from low value or currently not used as substrates. Manure was also mentioned by European Feed Manufacturers' Federation (FEFAC) in their October 2015 meeting. Category 2 animal material, by principle free of prions, can be added to this list. The use of these commodities would fit in with the EU initiative to promote a circular economy. In those cases, however, the FCR values can be substantially higher, up to 10 when using a high-protein–low-fat diet for house crickets or a value of 10.9 when using a low-protein–low-fat diet with carrot addition for yellow mealworm (Oonincx et al. Citation2015). These high FCR values could only be acceptable when otherwise non-valued substrates are being used. An interesting exception is the results of the experiments with Argentinean cockroach, which showed lower FCRs when reared on suboptimal substrates () (Oonincx et al. Citation2015).
Insects are cold blooded, which is assumed to be an important factor in their low FCRs (Veldkamp et al. Citation2012; FAO Citation2013). Nevertheless, it is demonstrated that some warm-blooded animals can achieve comparable low FCRs (Figure 1). An important factor in this comparison should be the situation that warm-blooded animals generally can heat their own facility, whereas rearing of cold-blooded animals might need additional heating. These additional energy costs, also those caused by cooling stables of warm-blooded animals, if applicable, should be included in the calculation of the energy balances when comparing the efficiency of different protein production systems (e.g., Kil et al. Citation2013; Shepon et al. Citation2016). Therefore, a measure for energy conversion should be used to complement the FCR, especially in the view of low FCRs for both cold- and warm-blooded systems (). Life cycle assessments (LCA) of insect rearing would include these and other aspects in a total overview of losses and gains (Salomone et al. Citation2017).
Detection and identification
Finally, considering all the possibilities and constraints to legalise insects as feed material, an approach for the proper and legal use of insects needs to be designed. Both the legal framework and a scenario for monitoring the correct application of insects as feed material should ideally be developed in close cooperation and communication. Detection of insects as part of the composition of a feed can be carried out for several objectives: label control (Regulation (EC) 767/2009), traceability (Regulation (EC) 178/2002) and detection of fraud (Regulation (EC) 882/2004; Decision (EU) 2015/1918). The major recent reviews of the use of insects as feed material (Veldkamp et al. Citation2012; Belluco et al. Citation2013; FAO Citation2013; Spiegel et al. Citation2013; Makkar et al. Citation2014; EFSA Citation2015) all lack a chapter on monitoring. However, it could be argued that a risk is higher when monitoring is not (fully) achievable. Administrative control or assurance regimes could be options in a range of circumstances. These options, however, will not ensure that the documents and label represent the content of a batch or party in all cases (an example is the horse meat incidence – for a review, see Elliott 2013). In those cases that physical control seems to be appropriate, analytical methods for detection and identification need to be available. This section will highlight several scientific aspects for such methods.
There are two principal methods for detection and identification of PAPs: classical light microscopy and PCR (Regulation (EU) 152/2009, Annex VI as amended by Regulation (EU) 51/2013). In addition, several other methods are published as being either fit for purpose or in a conceptual sense, including immunoassays (Bremer et al. Citation2013; van Raamsdonk et al. Citation2015b), in situ PCR of bone fragments (Lecrenier et al. Citation2014), in situ immunoassay detection of troponin I in muscle fibres (van Raamsdonk et al. Citation2011), and MS (Lecrenier et al. Citation2016). Some general overviews present other alternative methods (van Raamsdonk et al. Citation2007; Liu et al. Citation2011).
Classical light microscopy
RIKILT on behalf of the IAG section Feed Microscopy, organises proficiency tests for animal proteins on an annual basis. The 2014 test (van Raamsdonk et al. Citation2014) consisted of a feed sample fortified with 1% grasshopper meal. The results are summarised in .
Table 2. Overview of the analysis of a sample containing 1% grasshopper meal.
The reports of the participants are a mix of observations (e.g., muscle, chitin) and evaluations (e.g., insects, Crustacea). The observation of chitin is pooled with the more general indication of krill and Crustacea, since all arthropods (see Appendix A) possess an exoskeleton based on chitin. The report of the presence of muscle without any interpretation is correct since muscle fibres originating from fish and terrestrial animals cannot be distinguished either (van Raamsdonk et al. Citation2012). Nevertheless, European legislation requires that one distinguish between the categories of fish and terrestrial animals (Regulation (EC) 999/2001). Furthermore, marine arthropods (e.g., krill) are considered part of fish meal and, as discussed, insects are currently prohibited as feed ingredient. This situation implies that insects should be distinguished from either terrestrial animals, fish and marine arthropods and, hence, be reported as such. Therefore, the category ‘insects’ is separately included in .
The proteins (muscles) and chitin (outer scale, exoskeleton) of insects will not be part of the most important fraction of a sample (i.e., the sediment), which is examined microscopically (see Regulation (EC) 152/2009, Annex VI as amended by Regulation (EU) 51/2013; Liu et al. Citation2011). Two remarks can be made based on the IAG test results:
Sensitivity: in a future approach where monitoring of the presence of insects might be a standard objective, the large share of negative reporting (31%) will be much lower, since more attention will be given to the other fraction in a sample (i.e., the flotate).
Specificity: the discrimination between insects and krill is apparently difficult. In all those cases where muscle fibres were found (31%), these can up to now not be attributed to a specific animal group but to animals in general. This is also an issue when differentiating between ruminant and non-ruminant PAPs, where no other particles are found. DNA identification is then the next option.
The heavy and light fractions (high and low specific density) are usually separated by using the solvent tetrachloroethylene (D = 1.62). The heavy particles in the feed (minerals, bone fragments if present) are descending in the solvent, together forming the sediment, and all other particles remain floating, together forming the flotate. The sediment is usually less than 2% of the total amount of material used, resulting in a concentration of possibly present bone fragments of more than a factor 50. Experiments were carried out by RIKILT in the framework of the EU project SAFEED-PAP to find other (mixtures of) solvents for getting another distribution of the particles over the fractions, using several feeds and bovine and porcine meat meal (Bremer et al. Citation2012). Muscle fibres were chosen as target for in situ identification with an immunoassay, and these fibres appear to have a very low specific density. Therefore, several solutions have been tested to separate the majority of the matrix (vegetable ingredients) and the muscle material. The solvents used ranged from D = 0.62–0.66 (petroleum ether) to 1.32 (dichloromethane). Requirements additional to the specific density included non-toxicity, chemical inertness and a hydrophobic nature in order to be able to mix the extra solvent with TCE. Finally, the best results have been achieved with a mixture of TCE:heptane in a combination of 7:3 (v/v), where most muscle fibres remained floating and most other materials appeared to form the large sediment (Bremer et al. Citation2012). With respect to insects, a fair separation might be expected between muscle fibres and chitin parts in the flotate and other, denser, particles in the sediment. This would greatly enhance the capability of detecting insect parts in animal feeds. The next challenge of the application of light microscopy is the separation between the different arthropod classes: insects versus crustacean, including krill.
PCR
A lot of molecular data are available for insects in the tree of life (Meusemann et al. Citation2010), which can be used as a source for establishing suitable DNA targets. Alternatively, full sequence analysis of either nuclear or mitochondrial DNA can also provide information on the preferred DNA targets, e.g., the mitochondrial DNA genome of yellow meal worm (Liu & Wang Citation2014). Group-specific primers were developed for a range of insect species groups (Sint et al. Citation2014).
With a huge number of insect species (see Appendix A) and an expectation of a similarly large biodiversity, detection and identification by means of DNA might be complicated. Species group-directed identification might ease this task. In this respect it is necessary to consider at first the need of having a white or a black list of allowed versus prohibited species and to anticipate any future prohibition at all. At a higher level of classification, the distinction between insects and other arthropods, primarily crustaceans and krill, can be expected to be achieved with molecular targets, possibly supported by microscopic examinations (see the contribution of Ottoboni et al. 2017). Driven by economic importance and ethical considerations, a database with DNA sequence information or fingerprints of prohibited plague insects can be developed in concordance with the existing genetically modified organisms (GMO) databaseFootnote4 (Bonfini et al. Citation2012) and EUginiusFootnote5 with detection methods, events, constructs and specific taxon information.
Monitoring of insects as feed material
Several approaches for monitoring could be considered:
Processed insect remains prohibited as a feed material (current situation): a general DNA target for insects is sufficient. Possibly light microscopy can be applied as well.
A white list of a limited number of allowed species: establishment of sufficient DNA targets for all non-listed, i.e., prohibited species can be expected to be very complicated. Focus on the species mentioned in the white list would support label control and traceability. Pre-screening by light microscopy might be an opportunity.
A black list of a limited number of prohibited species, e.g., pest insects: DNA target per single species or genus needs to be developed. New targets have to be developed every time the list is extended.
No prohibition of insects throughout the feed chain: identification at species level is not necessary as the presence of all other feed (plant) materials for label control is established at a general level as well. Light microscopy could be sufficient. All other safety, hygiene and environmental issues remain.
Principally physical monitoring for authenticity or fraud detection is targeting the presence of prohibited material. Although a white list seems to be most practical as discussed, this would result in the need to detect almost all insects, since those unlisted species are prohibited. The alternative is the detection of the listed and, hence, allowed species. In those cases where an allowed species is encountered, still no information is available of any prohibited species co-occurring with the allowed one. When considering the balance between the profits and disadvantages of either a white or a black list, the objective(s) needs to be taken into account: either label control, traceability or fraud detection.
Discussion
In the view of promoting the recycling of by-products (EU initiative) and the need to use alternative commodities, legislative requirements apply when accommodating those alternatives. It is interesting to discuss here briefly the rearing of earthworms. These animals can be compared with insects in several aspects: invertebrate animals, included in category 9.16.1 of the feed catalogue (see Appendix B), and not specified in the regulations on the animal by-products ban. The commodity for raising earthworms is directed by the specialised nature, namely humus-rich, soils. Such commodities are not particularly regulated, and the monitoring of feed safety is carried out on the final (processed) product. Insects can be treated in the same way by considering their rearing, including the commodities used, as a ‘pre-chain’ additional to the main feed production chain. The products resulting from this pre-chain, as any other feed material, have to comply with the full set of requirements and restrictions for feed safety and hygiene. The resulting waste and by-products should comply with the appropriate measures. The exclusion of insects (and other invertebrates such as earthworms) from the definition of PAPs could be considered, with the effect that a larger range of low-quality commodities can be used for rearing insects. This is an important way to achieve a sustainable cultivation. Several reviews (e.g., FAO Citation2013; Lähteenmäki-Uutela & Grmelová Citation2016) point out two advantages of using insects: (1) a low feed-conversion rate; and (2) the possibility of rearing insects on low-quality or waste substrates. Current data point out that these advantages are mutually exclusive (). The use of low-quality or waste substrates is expected to result in higher FCRs compared with high-quality substrates, in some cases even higher than the modern values for cattle, although exceptions might exist ().
Based on these viewpoints, it has to be emphasized that using insects for feed production is not a goal on itself, but can be an instrument to achieve goals in biowaste reduction and conversion, improving sustainability and optimising food webs. These goals should be confirmed before large-scale cultivation of insects is implemented. Two basic strategies can be envisioned:
Find and legalise new commodities for rearing already-accepted insects. This could include those commodities with an ethical constraint such as category 2 material, manure, and catering waste and household waste containing meat (see also Finke et al. Citation2015).
Find new insects to apply alternative commodities. Gardening and forestry products are mentioned by EFSA (Citation2015). The insect species currently getting attention in the EU cannot digest these kinds of commodities, but termites and some comparable insects are capable of doing so (FAO Citation2013).
The need to assess the environmental impact and to prohibit the cultivation of plague insects remain. In those cases where different feed-producing systems show comparably low FCRs, a complementary measure for energy conversion should be used. Any diversification of allowed versus prohibited species, either a white or a black list, will have implications on the design of the analytical methods.
In order to find the balance between maximising the benefits and minimising the risks and the ecological footprint of the use of insects in a legal framework, a principle called WISE is proposed:
Witfull: implementation of new legal regulations or measures should be based on reasonable legal principles and well embedded in the framework of existing legislation. This legal constraint is usually well assured. With respect to insects, the link between the feed catalogue, the definition of PAP and the animal by-product bans might need further attention.
Indicative: the border between legal and illegal application should be indicated by clear limits. Definitions should be clear and precise in order to facilitate enforcement strategies and the underlying methods for control. This applies primarily to issues in the area of identity and authenticity of insects; legal limits on contaminants and undesirable impurities are well established.
Societal demands: the regulation or measure should match the demands from the perspectives of public health, environmental constraints and market/trade desires, and it should seek a balance among these perspectives. In the view of the large diversity among insect species, assessments of feed and food safety, of environmental impact and of the efficiency of rearing systems are most appropriately made in a case-by-case approach, which should include enforcement policies.
Enforceable: a definition, restriction or measure should facilitate enforcement practices. These can include administrative control, assurance regimes and physical monitoring. In the case of the latter option, a suited control method is desired. Ideally such regulations and the development of the monitoring method should be twinned until finally putting it into force. The way insects are defined and a possible application of either a white or a black list, or more generally placing partial bans into force and make derogations to it, has a major impact on the design of analytical methods for monitoring authenticity, label control and detection of fraud.
Acknowledgements
The authors thank Dr L. van der Geest, Dr A. Peijnenburg, Ms M. Noordam and Dr T. W. Prins for their valuable comments.
Disclosure statement
No potential conflict of interest was reported by the authors.
Additional information
Funding
Notes
2. Live insects are not subjected to the mentioned legislation and whole insects are considered as not being processed.
References
- Belluco S, Losasso C, Maggioletti M, Alonzi CC, Paoletti MG, Ricci A. 2013. Edible insects in a food safety and nutritional perspective: a critical review. Comp Rev Food Sci Food Saf. 12:296–313.
- Best P. 2011. Poultry performance improves over past decades. Available from: WATTagnet.com November 24.
- Bonfini LVD, Bulcke MH, Mazzara M, Ben E, Patak A. 2012. GMOMETHODS: the European union database of reference methods for GMO analysis. J AOAC Intl. 95:1713–1719.
- Bremer M, Fumière O, Hekman W, Marien A, Kemmers-Vonken A, Fernández Pierna JA, Vliege J, Baeten V, Raamsdonk LWD van, Berben G. 2012. Combination methods for PAP detection and species determination of animal particles. In: Detection, identification and quantification of processed animal proteins in feedingstuffs. Namur: Les éditions namuroises. p. 139–149. ISBN 978-2-87551-029-7.
- Bremer MGEG, Margry RJCF, Vaessen JCH, Doremalen AMH van, Palen JGP van de, Kaathoven RGC van, Kemmers-Vonken AEM, Raamsdonk LWD van. 2013. Evaluation of a commercial ELISA for detection of ruminant processed animal proteins in non-Ruminant processed animal proteins. J AOAC Intl. 96:552–559.
- Burg SWK, Stuiver M, Veenstra FA, Bikker P, Lopez Contreras AM, Palstra AP, Broeze J, Jansen HM, Rg J, Gerritsen AL, et al. 2013. A Triple P review of the feasibility of sustainable offshore seaweed production in the North Sea. LEI Report 13-077. Wageningen: Wageningen UR; p. 108. ISBN 9789086156528.
- Charlton AJ, Dickinson M, Wakefield ME, Fitches E, Kenis M, Han R, Zhu F, Kone N, Grant M, Koko GKD, et al. 2015. Exploring the chemical safety of fly larvae as a source of protein for animal feed. J Insects Feed Food. 1:7–16.
- Collavo A. Glew RH, Huang YS, Chuang LT, Bosse R, Paoletti MG. 2005. House cricket small-scale farming. In MG Paoletti, editor. Ecological implications of minilivestock: potential of insects, rodents, frogs and snails. New Hampshire: Science Publishers, p. 519–544.
- Collins SJ, Lawson VA, Masters CL. 2004. Transmissible spongiform encephalopathies. Lancet. 363:51–61.
- Crespo R, Villaverde ML, Girotti JR, Güerci A, Juárez MP, Bravo MGD. 2011. Cytotoxic and genotoxic effects of defence secretion of defence secretion of Ulomoides dermestoides on A549 cells. J Ethnopharm. 136:204–209.
- Devkota B, Schmidt GH. 2000. Accumulation of heavy metals in food plants and grasshoppers from the Taigetos Mountains, Greece. Agric Ecosyst Environ. 78:85–91.
- Dijkstra J, France J, Ellis JL, Strathe AB, Kebreab E, Bannink A. 2013. Production efficiency of ruminants: feed, nitrogen and methane. In: Kebreab E, editor. Sustainable animal agriculture. Wallingford: CAB International; p. 10–25.
- Eenennaam ALV. 2013. Genetics and sustainable animal agriculture. In: Kebreab E, editor. Sustainable animal agriculture. Wallingford: CAB International; p. 53–66.
- Elliott review. 2013. Available from: https://www.gov.uk/government/publications/elliott-review-into-the-integrity-and-assurance-of-food-supply-networks-interim-report
- European Food Safety Authority (EFSA). 2012. Compendium of botanicals reported to contain naturally occurring substances of possible concern for human health when used in food and food supplements. EFSA J. 10:2663–2722.
- European Food Safety Authority (EFSA). 2015. Scientific opinion on a risk profile related to production and consumption of insects as food and feed. EFSA J. 13:4257–4317.
- Fahmy MH, Boucher JM, Pose LM, Grégoire R, Butler G, Comeau JE. 1992. Feed efficiency, carcass characteristics, and sensory quality of lambs, with or without prolific ancestry, fed diets with different protein supplements. J Anim Sci. 70:1365–1374.
- Finke MD, Rojo S, Roos N, van Huis A, Yen AL. 2015. The European food safety authority scientific opinion on a risk profile related to production and consumption of insects as food and feed. J Insects Food Feed. 1:245–247.
- Handley MA, Hall C, Sanford E, Diaz E, Gonzalez-Mendez E, Drace K, Wilson R, Villalobos M, Croughan M. 2007. Globalization, binational communities, and imported food risks: results of an outbreak investigation of lead poisoning in Monterey County, California. American J Public Health. 97:900–906.
- Hayes BJ, Lewin HA, Goddard ME. 2013. The future of livestock breeding: genomic selection for efficiency, reduced emissions intensity, and adaptation. Tr Genetics. 29:206–214.
- Hogan GR, Razniak HG. 1991. Selenium-Induced mortality and tissue distribution studies in Tenebrio molitor (Coleoptera: tenebrionidae). Environ Entomol. 20:790–794.
- Hui D. 2012. Food web: concept and Applications. Nat Educ Knowledge. 3:6.
- Huis A van. 2013. Potential of insects as food and feed in assuring food security. Ann Rev Entomol. 58:563–583.
- Kil DY, Kim BG, Stein HH. 2013. Feed energy evaluation for growing pigs. Asian-Australas J Anim Sci. 26:1205–1217.
- Kumschick S, Devenish A, Kenis M, Rabitsch W, Richardson D, Wilson J. 2016. Intentionally introduced terrestrial invertebrates: patterns, risks, and options for management. Bio Invasions. 18:1077–1088.
- Lähteenmäki-Uutela A, Grmelová N. 2016. European law on insects in food and feed. Eur Food Feed Law Review. 11:2–8.
- Lecrenier MC, Ledoux Q, Berben G, Fumiére O, Saegerman C, Baeten V, Veys P. 2014. Determination of the ruminant origin of bone particles using fluorescence in situ hybridization (FISH). Nat Scientific Rep. 4:5730.
- Lecrenier MC, Marbaix H, Dieu M, Veys P, Saegerman C, Raes M, Baeten V. 2016. Identification of specific bovine blood biomarkers with a non-targeted approach using HPLC ESI tandem mass spectrometry. Food Chem. 213:417–424.
- Liu L-N, Wang CY. 2014. Complete mitochondrial genome of yellow meal worm (Tenebrio molitor). Zool Res. 35:537−545.
- Liu X, Han L, Veys P, Baeten V, Jiang X, Dardenne P. 2011. An overview of the legislation and light microscopy for detection of processed animal proteins in feeds. Micros Res Techn. 74:735–743.
- Lundy ME, Parrella MP. 2015. Crickets are not a free lunch: protein capture from scalable organic side-streams via high-density populations of Acheta domesticus. Plos ONE. 10:e0118785.
- Lupi O. 2005. Risk analysis of ectoparasites acting as vectors for chronic wasting disease. Med Hypotheses. 65:47–54.
- Lupi O. 2006. Myiasis as a risk factor for prion diseases in humans. J Eur Acad Dermatol Venereol. 20:1037–1045.
- Makkar HPS, Tran G, Heuze V, Ankers P. 2014. State-of-the art on use of insects in animal feed. Anal Feed Science Techn. 197:1–33.
- McMillian WW, Widstrom NW, Wilson DM. 1981. Rearing the maize weevil on maize genotypes when aflatoxin-producing Aspergillus flavus and A. parasiticus isolates were present. Environ Entomol. 10:760–762.
- Meusemann K, von Reumont BM, Simon S, Roeding F, Strauss S, Kück P, Ebersberger I, Walzl M, Pass G, Breuers S, et al. 2010. A phylogenomic approach to resolve the arthropod tree of life. Mol Biol Evol. 27:2451–2464.
- Morley RS, Chen S, Rheault N. 2003. Assessment of the risk factors related to bovine spongiform encephalopathy. OIE Revue Scientifique Technique. 22:157–178.
- Oonincx DGAB, van Broekhoven S, van Huis A, van Loon JJA. 2015. Feed conversion, survival and development, and composition of four insect species on diets composed of food by-products. Plos ONE. 10:e0144601.
- Pârvu M, Bogdan AT, Andronie ICAmfim A. 2011. Aspects of energy metabolism in mangalitsa pigs exposed at thermic neutral temperature. An Sci Biotech. 2011:44–46.
- Post K, Riesner D, Walldorf V, Mehlhorn H. 1999. Fly larvae and pupae as vectors for scrapie. Lancet. 354:1969–1970.
- Prince MJ, Bailey JA, Barrowman PR, Bishop KJ, Campbell GR, Wood JM. 2003. Bovine spongiform encephalopathy. OIE Revue Scientifique Technique. 22:37–60.
- Pritzkow S, Morales R, Moda F, Khan U, Telling GC, Hoover E, Soto C. 2015. Grass plants bind, retain, uptake, and transport infectious prions. Cell Reports. 11:1–8.
- Prusiner SB. 1998. Prions. Proc Natl Acad Sci USA. 95:13363–13383.
- Raamsdonk LWD van, Holst C van, Baeten V, Berben G, Boix A, Jong J de. 2007. New developments in the detection of animal proteins in feeds. Feed Sci Techn. 133:63–83.
- Raamsdonk LWD van, Margry RJCF, Kaathoven RGC van, Bremer MGEG. 2015b. Inter-laboratory study of two immunochemical methods for detection of ruminant animal proteins. Food Chem. 185:333–339.
- Raamsdonk LWD van, Ozinga WA, Hoogenboom LAP, Mulder PPJ, Mol JGJ, Groot MJ, Fels-Klerx HJ van der, Nijs M den. 2015a. Exposure assessment of cattle via roughages to plants producing compounds of concern. Food Chem. 189:27–37.
- Raamsdonk LWD van, Pinckaers VGZ, Scholtens IM, Prins TW, Voet H van der, Vliege JJM. 2014. IAG ring test animal proteins 2014. Report 2014.011. Wageningen: RIKILT; p. 47.
- Raamsdonk LWD van, Pinotti L, Veys P, Bremer M, Hekman W, Kemmers A, Campagnoli A, Paltanin C, Belinchón CC, Vliege J, et al. 2011. New developments in classical microscopy; what can be expected for the official control? Biotechnol Agron Soc Environ. 15:15–24.
- RaamsdonkLWD van, Pinotti L, Veys P, Campagnoli A, Paltanin C, Belinchón CC, Jørgensen JS. 2012. Markers for microscopic detection. In: Detection, identification and quantification of processed animal proteins in feedingstuffs, Namur, Les éditions namuroises, Ch 6. p. 59–69. ISBN 978-2-87551-029-7.
- Salomone R, Saija G, Mondello G, Giannetto A, Fasulo S, Savastano D. 2017. Environmental impact of food waste bioconversion by insects: application of life cycle assessment to process using Hermetia illucens. J Cleaner Prod. 140:890–905.
- Shepon A, Eshel G, Noor E, Milo R. 2016. Energy and protein feed-to-food conversion efficiencies in the US and potential food security gains from dietary changes. Environ Res Lett. 11:105002.
- Shike DW. 2013. University of Illinois at urbana-champaign driftless region beef conference 2013. Available from: http://www.beefusa.org/CMDocs/BeefUSA/Resources/cc2012-Beef-Feed-Efficiency--Dan-Shike.pdf
- Sint D, Niederklapfer B, Kaufmann R, Traugott M. 2014. Group-specific multiplex PCR detection systems for the identification of flying insect prey. Plos ONE. 9:e115501.
- Smil V. 2002. Nitrogen and food production: proteins for human diets. AMBIO: J Human Environ. 31:126–131.
- Spiegel M van der, Noordam M, Fels-Klerx HJ van der HJ. 2013. Safety of novel protein sources (Insects, Microalgae, Seaweed, Duckweed, Rapeseed) and legislative aspects for their application in food and feed production. Comp Rev Food Science Food Safety. 12:662–678.
- Stender DR. 2012. Iowa State University extension. IPIC 25h. Swine Feed Efficiency: Influence of Market Weight 2012. Ames: Iowa State University. Available from: https://web.archive.org/web/20150909053400/http://www.swinefeedefficiency.com/factsheets/IPIC25h%20SFE%20Influence%20of%20Market%20Weight.pdf
- Thackray AM, Muhammad F, Zhang C, Denyer M, Spiropoulos J, Crowther DC, Bujdoso R. 2012. Prion-induced toxicity in PrP transgenic drosophila. Exp Mol Path. 92:194–201.
- FAO - Food and Agriculture Organization of the United Nations. 2013. Edible insects. Future prospects for food and feed security. In: Huis A. van, Itterbeeck J van, Klunder H, Mertens E, Halloran A, Muir G, Vantomme P, editors Rome. Available from: http://www.fao.org/docrep/018/i3253e/i3253e00.htm
- Veldkamp T, van Duinkerken G, van Huis A, Lakemond CMM, Ottevanger E, Bosch G, Boekel MAJS van. 2012. Insects as a sustainable feed ingredient in pig and poultry diets–a feasibility study. Report 638. Wageningen: Wageningen UR Livestock Research; p. 48.
- Vié J-C, Hilton-Taylor C, Stuart SN, editors 2009. Wildlife in a changing world – An analysis of the 2008 IUCN red list of threatened species. Gland: IUCN; p. 180.
- Wilkinson J. 2011. Re-defining efficiency of feed use by livestock. Animal. 5:1014–1022.
- Zagrobelny M, Dreon AL, Gomiero T, Marcazzan GL, Glaring MA, Moller BL, Paoletti MG. 2009. Toxic moths: source of a truly safe delicacy. J Ethnobiol. 29:64–76.
Appendix A: Brief selection of some major animal groups.
Appendix B: Relevant definitions in Regulation (EC) 68/2013: feed catalogue.