ABSTRACT
Recently, traces of brominated flame retardants (BFRs) have been detected in black plastic food-contact materials (FCMs), indicating the presence of recycled plastics, mainly coming from waste electric and electronic equipment (WEEE) as BFRs are one of the main additives in electric applications. In order to evaluate efficiently and preliminary in situ the presence of WEEE in plastic FCMs, a generic procedure for the evaluation of WEEE presence in plastic FCMs by using defined parameters having each an associated importance level has been proposed. This can be achieved by combining parameters like overall bromine (Br) and antimony (Sb) content; additive and reactive BFR, rare earth element (REE) and WEEE-relevant elemental content and additionally polymer purity. In most of the cases, the WEEE contamination could be confirmed by combining X-ray fluorescence (XRF) spectrometry and thermal desorption/pyrolysis gas chromatography-mass spectrometry (GC-MS) at first. The Sb and REE content did not give a full confirmation as to the source of contamination, however for Sb the opposite counts: Sb was joined with elevated Br signals. Therefore, Br at first followed by Sb were used as WEEE precursors as both elements are used as synergetic flame-retardant systems. WEEE-specific REEs could be used for small WEEE (sWEEE) confirmation; however, this parameter should be interpreted with care. The polymer purity by Fourier-transform infrared spectrometer (FTIR) and pyrolysis GC-MS in many cases could not confirm WEEE-specific contamination; however, it can be used for purity measurements and for the suspicion of the usage of recycled fractions (WEEE and non-WEEE) as a third-line confirmation. To the best of our knowledge, the addition of WEEE waste to plastic FCMs is illegal; however, due to lack on screening mechanisms, there is still the breakthrough of such articles onto the market, and, therefore, our generic procedure enables the quick and effective screening of suspicious samples.
KEYWORDS:
- Inductively coupled plasma optical emission spectroscopy
- thermal desorption
- pyrolysis mass spectrometry
- brominated flame retardants
- food-contact materials
- waste electric and electronic equipment
- attenuated total reflectance Fourier-transformed infrared spectroscopy
- X-ray fluorescence spectroscopy
- rare earth elements
Introduction
Plastic food-contact materials (FCMs) are a crucial medium for storing, packing, protecting, delivering, preparing, cooking and consuming foodstuffs. Due to their specific contact to food, all plastic FCMs are therefore directly influencing the quality of food and beverages by potentially releasing a certain level of multiple chemical migrants (constituents) to which individuals are exposed. For the European market the requirements of Regulation (EC) 1935/2004 of the European Parliament and of the Council (further as regulation (EC) 1935/2004; European Union Citation2004) apply, implementing that all materials and articles, including active and intelligent materials, should be manufactured in compliance with good manufacturing practice so that, under normal or foreseeable conditions of use, they do not transfer their constituents to food in quantities that could endanger human health, bring about an unacceptable change in the composition of the food or bring about a deterioration in the organoleptic characteristics. All plastic FCMs should be manufactured only from substances, listed in Annex 1 (positive list approach) of Commission Regulation (EU) 10/2011 (further as Regulation (EU) 10/2011; European Commission Citation2011a) and its amendments (European Commission Citation2011b, Citation2011c, Citation2012a, Citation2014a, Citation2014b, Citation2015, Citation2016). Other substances different from those listed in the Regulation (EU) 10/2011 positive list can be intentionally used in the manufacture of plastics intended to come into contact with foodstuffs: (1) colorants (a special type of additives); (2) solvents (a special type of polymer-production aids – PPAs); (3) certain non-listed PPAs (provided that these three points are subjected to member states’ national laws); (4) substances mentioned in Article 6(3) (a–d), subject to rules in certain Articles of Regulation (EU) 10/2011; and (5) aids to polymerisation (AP). The positive list is still expanding because new toxicological data are available and new chemicals are appearing on the market. For some of these substances, the positive list establishes purity criteria, concentration limits of the substance in the plastic FCMs, and also specific migration limits (SMLs) (set by taking into account toxicological data as the tolerable daily intake (TDI) of the substance). The purpose of these SMLs is to control the migration of substances of toxicological interest from the plastic FCMs to the foodstuffs or simulants. Regulation (EU) 10/2011 requires that plastic FCMs intended to come into contact with almost all types of food, must comply with the overall migration limit (OML), after performing standardised migration tests. This OML is set (Annex III of Regulation (EU) 10/2011) in order to prove the resistance of the plastic FCM against food. For substances not listed in the positive list, a risk assessment should be done in order to define a safe SML. An SML of 0.01 mg kg–1 is considered as a safe enough for most substances, except those classified as carcinogenic, mutagenic and toxic to reproduction (CRM). These substances are totally banned for the intentional use in plastic FCMs. Waste electrical and electronic (WEEE) is a post-consumer waste stream based on all kinds of plastics containing additives such as antioxidants, brominated flame retardants (BFRs) and inorganic hazardous compounds (Dimitrakakis et al. Citation2009; Vilaplana et al. Citation2008; Goodship & Stevels, Citation2012; UNEP Report Citation2013). Electronic devices contain, by their application, organobromine substances like BFRs which have an inhibitory effect on the ignition of combustible organic materials and fire spread. Generally, by quick interaction chemistry of the bromine (Br) functions, BFRs decrease the flammability of the final product.
In order to achieve sufficient flame retardancy, BFRs are needed to be added at a percentage level (Wäger et al. Citation2009; Binnemans et al. Citation2016). Practically, BFR levels can be reduced by adding Sb2O3 (Sb = antimony) as a synergist because during the thermal degradation process Sb2O3 releases water by H-abstraction from the polymer chains combined with the production of SbBr3 after partial debromination of the BFRs (Camino et al. Citation1991). Jakab et al. (Citation2003) studied the effect of Sb2O3 addition to two brominated flame retarded high-impact polystyrene (HIPS) samples and discovered a higher thermal stability for polymers containing Sb2O3 and BFRs than the polymers containing only the BFRs at same concentration. Generally, Sb2O3 synergist is added to the polymer in the lower percentage range, typically containing 5–10 weight %, increasing later in WEEE the overall Sb content. Dimitrakakis et al. (Citation2009) reported that from all analysed WEEE samples (n = 161), half where Br positive, with a majority containing Sb as well. Plastic FCMs having a direct contact with food do not require fireproofing and, therefore, should not contain a significant level of Sb. However, Sb in the form of Sb2O3 is allowed and used mainly in polyethylene terephthalate (PET) bottles at a concentration level of 0.2–0.3 weight % of Sb2O3 (Keresztes et al. Citation2009). For FCM purposes, this parameter is covered by specific elemental migration according to Regulation (EU) 10/2011 (Substance No. 398; CAS No. 0001309-64-4; Sb2O3; SML 0.04 mg kg−1 expressed as Sb). There are other inorganic fire retardants (FRs) with extinguishing properties, as BFRs inhibit the gas-phase combustion by free-radical scavenging, the inorganic FRs (e.g., hydroxides and carbonates) function in the gas and condensed phases by releasing non-flammable substances (H2O, CO2), which dilutes the fuel and cools the polymer surface. Inorganic FRs during combustion form a crust on the polymer surface preventing the access of oxygen to the polymer fuel. Other commercial inorganic (synergist) FRs are based on iron oxide, zinc borate, zinc stannate, zinc phosphate, magnesium hydroxide, aluminium hydroxide, calcium carbonate and magnesium carbonate (Pritchard Citation1998; Segev et al. Citation2009).
WEEE is predominantly based on polymer matrices which are used in terms of significance and prominence. The most abundant WEEE matrices are based on a majority of polystyrene (PS), polyacrylonitrile-butadiene-styrene terpolymer (ABS), polyolefins like polyethylene and/or polypropylene (PE/PP) and polycarbonate (PC), either as blends or as different grades of PC (Martinho et al. Citation2012; Buekens & Yang Citation2014). Casings of large household products (mainly PS) will have a higher impact than covers or components from small WEEE (sWEEE, mainly ABS and PP) on the composition of WEEE in general. WEEE plastics contain inorganic hazardous compounds coming from the fillers, wires, printed circuit boards and other components including beside ‘base metals’ as well rare earth elements (REEs) (Groß et al. Citation2008; Wienold et al. Citation2011; Tan et al. Citation2017).
Based on the presence of harmful elements like Hg, Pb, Cd and Cr6+, the EU introduced the Restriction of Hazardous Substances (RoHS) 2002/95/EC (European Commission Citation2002a), which recast Directive Citation2011/65/EU of the European Parliament and of the Council (European Commission Citation2011d), effective from 2013 limiting Hg, Pb and Cr6+, including polybrominated biphenyls (PBBs) and polybrominated diphenylethers (PBDEs) at a threshold level of 0.1 weight % of homogeneous material and 0.01 weight % for Cd. Harmonised methods like the six standards from the International Electrotechnical Commission (International Electrotechnical Commission (IEC), Citation2008) in the 62321 series (2013) for evaluating WEEE recommend an orientation screening at first, followed by more precise and time-demanding methods for evaluation. This approach of screening at first implements the use of spectral methods in order to evaluate quickly the compliance of each parameter preferable in situ without dismantling or destroying the electric device.
Within our paper we will take over this approach for the evaluation of WEEE contamination in plastic FCMs. This is due to the need for such methods as recently BFRs have been detected in many consumer goods like toys, plastic FCMs, VHS and polymer-based necklaces (Hirai et al. Citation2007; Miller et al. Citation2016; Guzzonato et al. Citation2016a). We would like to bring this appearance of WEEE in plastic FCMs and their constituents into an actual perspective, therefore as introduction an overview of relevant available literature and findings. BFRs have a wide range of applications dating back many centuries (Hindersinn Citation1990); however, nowadays they are developed with the same aim but evolved to a numerous amount of individual chemicals.
Bergman et al. (Citation2012) unified the abbreviations used for commercial BFRs as well as chlorinated flame retardants (CFRs) and phosphorus-based flame retardants (PFRs) which cover the majority of the organic flame retardants, although melamine or PVC do not contain either phosphorus or halogen-based flame retardants. As the number of chemicals of concern has increased, they made an overview of abbreviations and proposed one to be generally used, which resulted in a list of nearly 70 different halogenated FRs (mainly BFRs) and nearly 30 PFRs. Due to higher migration rates and the aim to increase the material’s fire resistance in the environment, FRs are either incorporated as additives (moulded blend), additive polymeric BFR or reactive components, e.g., tetrabromobisphenol A (TBBPA) with bisphenol A for PC production. Since 1990 a substitute for technical decabromodiphenylether (decaBDE, based on a majority of decaBDE-209 congener), decabromodiphenylethane (DBDPE) was introduced (US Patent 5077334, 1991) which when implemented over a certain time came to be found in sediments (Kierkegaard et al. Citation2004; Ricklund et al. Citation2010), household dust (Stapleton et al. Citation2008a) and air (Egebäck et al. Citation2012). Because the long service time of several electric household products, large amounts of older BFRs will still appear in the environment (Vyzinkarova & Brunner Citation2013). The presence of BFRs in plastic FCMs can be explained by the bad waste management of WEEE into virgin polymers to make the final product intentionally cheaper or might be accidental contamination; however, it is a violation of European law (Regulations (EU) 10/2011 and 1935/2004/EC) as these substances are not on the positive list and, as a consequence, not authorised for food contact. Moreover, WEEE plastic materials are used in other fields for other technical purposes, containing non-food-grade substances (having not been risk assessed for food contact), and are not contemplated in the groups of substances that are allowed to be used for food contact, even without being included on the positive list of Regulation (EU) 10/2011. The usage of recycled polymer materials for manufacturing plastic FCMs is allowed in the European market, but only under strict conditions (Commission Regulation (EC) 282/2008; further (EC) 282/2008; European Commission Citation2008) when implementing the reuse of raw materials from a carefully and efficiently sorted waste stream. The associated risks are the migration of potential contaminants from an unknown waste stream. The sorting efficiency of such a waste stream can be achieved in controlled closed-product loops like the PET bottles cycle. The sorting efficiency necessary for each material should be identified on a case-by-case basis and conditions for use of recycled polymeric material (either in whole or in part) as FCM implements appropriate scientific evidence that the process is able to reduce any contamination of the plastic input to a concentration that does not pose a risk to human health (Barthélémy et al. Citation2014); the quality of the recycled plastic must be characterised and controlled in accordance with pre-established criteria that ensure compliance of the final recycled plastic material performed by government authorities (Regulation (EC) 1935/2004/EC). From the economical/environmental point of view, for producers of polymer articles, the use of a recycled polymer fraction implements a decrease of the price of the final products and a lower environmental impact. However, as a potential risk, plastic packaging waste may contain residues from previous use, contaminants from misuse and contaminants from non-authorised substances. Therefore, it is necessary to lay down special requirements (e.g., testing by chemical analysis) to ensure that materials and articles produced from recycled plastics and intended for food contact respect the requirements of Regulation (EC) 1935/2004/EC. Recycled polymers intend to be grey or brown (e.g., in case of WEEE-contaminated plastic FCMs), as a solution, by colour unification generally the mixture gets modified into black: the polymeric article again becomes fashionable. Muncke (Citation2009) confirmed that food packaging is a major source of food contaminants summarising that there is a strong influence from chemicals behaving as endocrine-disrupting compounds. For our purposes, this relation between food packaging and human health issues can be taken over for the presence of BFRs in FCM as BFRs have an influence on human health (Szymańska et al. Citation1999; Covaci et al. Citation2006; Harju et al. Citation2007) when they enter the human food chain (Darnerud Citation2003; Schecter et al. Citation2009; Gieroń et al. Citation2010) or migrate into foodstuffs by their presence in plastic FCMs.
As BFRs are persistent, bioaccumulative and toxic to both humans and the environment, and suspected to cause neurobehavioral effects and endocrine disruption, the EFSA recommended monitoring BFRs in food of animal origin (Commission Recommendation; European Commission Citation2014c). Analytical approaches for the detection of BFRs and WEEE-related elements (REEs and non-REEs) in polymer matrices were evaluated in certain cases using state-of-the-art methods; however, several studies are briefly given in .
Table 1. Overview of THE available analytical methods for the quantification of selected parameters for waste electric and electronic equipment (WEEE) evaluation in polymer matrices.
As this current paper is closely connected to our previous publications, therefore we give an overview of the conclusions done so far. The first study (Samsonek & Puype Citation2013) mainly focused on black plastic FCMs in order to identify the source of the WEEE-recycled polymer fractions. The comparison of the BFRs found in black thermocups and the absence of BFRs in the green and red thermocups confirmed the hypothesis that black items are ‘risky’. By Fourier-transform infrared spectrometer (FTIR) identification the Br-positive matrices were generally based on PE/PP and styrenic matrices like ABS. A motivation for this appearance is that styrene-based polymers and co-polymers/blends of PP/PE are easy to be mechanically recycled by melting technology. Another interesting fact in this study was the occurrence of BFR-positive samples all over Europe. The majority of the samples were purchased in the Czech Republic, but also eight samples from other EU countries were collected. From 22 samples purchased in the Czech Republic, 10 were positive for BFRs, while for other EU countries from eight samples three were positive for BFRs (purchased in Belgium, Germany and the Slovak Republic). The Br-positive samples contained mainly technical decaBDE. Newer types of BFRs such as tetrabromobisphenol A (TBBPA), tetrabromobisphenol A bis(2,3-dibromopropyl), ether (TBBPA-BDBPE) and DBDPE, replacing the PBDEs and PBBs, were also identified. In all samples PBBs or hexabromocyclododecane (HBCD) were absent.
The second study (Puype et al. Citation2015) confirmed the possibility that recycled fractions from WEEE streams were illegally entering the European market focusing again on black plastic FCMs. Evidence that WEEE, and its relevant contaminants, are presented in plastic FCMs was achieved by applying the following parameters: Br quantification, BFR identification combined with WEEE-relevant elemental analysis and polymer impurity analysis were performed. From the 10 selected plastic FCMs, seven samples contained a Br level ranging from 50 to 6000 mg kg−1, which is lower than expected to achieve flame retardancy. The BFRs found were TBBPA, decaBDE, DBDPE and 1,2-bis(2,4,6-tribromophenoxy)ethane (BTBPE). Typical elements used in electronic equipment and present in WEEE were detected either at trace level or at elevated concentrations. In all cases when Br was detected at higher concentrations, concurrently Sb was also detected, which confirms the synergetic use of Sb in combination with BFRs. We describe as well the measurement of REEs where combinations of cerium (Ce), dysprosium (Dy), lanthanum (La), neodymium (Nd), praseodymium (Pr) and yttrium (Y) were detected in four of the seven BFR-positive samples. Additionally, the polymer purity was investigated where in all cases foreign polymer fractions were detected. Despite the fact that this study was carried out only on a very small number of samples, there is a significant likelihood that WEEE has been used for the production of plastic FCMs.
In a third study, Knoop et al. (Citation2014) evaluated the presence of REEs in completely milled and digested WEEE samples detecting by inductively coupled plasma-optical emission spectroscopy (ICP-OES) concentrations of Nd, La, Pr, Dy, Y, Ce and erbium (Er) ranging from 1 to 100 mg kg−1. In all nine samples (mobile phone, LCD screen and seven circuit boards), Y was detected followed by Ce as the most abundant present REEs (in three samples).
In this paper we present a generic methodology combining analytical tools that can be used by the authorities in order to monitor compliance to Regulation (EU) 10/2011. Generic procedures are not yet available and, therefore, we offer our approach in order to apply uniform quality criteria and result interpretation for the detection of WEEE fractions in polymeric plastic FCMs. At first, rapid and essential screening for common synergists like Br and Sb, preferably screened by an X-ray spectral method in order to allow measurements in real time of the selected samples on site, e.g., for consumer goods control in situ, can preselect suspicious samples. The intention is to have an effective screening method based on preselected qualifiers without having an a priori knowledge of the samples. The selection of suspected samples (Br and Sb positive) can significantly increase the efficiency of the whole control process, saving time and money-demanding analysis. In this way, samples that have the highest probability to fail the requirements of Regulation (EU) 10/2011 are not going to be subjected to further analysis, and clearing the market from non-food-grade products can be done quickly and effectively. For further evaluation, in laboratorium approaches should be used to determine WEEE-specific parameters.
Materials and methods
Sampling, statement and testing strategy
Within our previous studies, mainly black plastic FCMs were evaluated on WEEE-related parameters as they are likely to be made from polymeric waste fractions. For our studies, samples of polymeric kitchen utensils and thermocups were selected from the European market without having a priori knowledge of their material composition. The aim of the results is to propose a deduction method using relevant parameters for the evaluation of the presence of WEEE in plastic FCMs. The described method is not an ‘all-in-one’ approach but requires multiple measurements () linked to an importance level. At a highest importance level is the evaluation of Br (BFR) and Sb presence; a direct indication for WEEE presence, this criterion will discard a majority of the WEEE-contaminated plastic FCMs. At a second level, WEEE-related elements can be measured (including REEs for potential sWEEE contamination).
XRF analysis: screening for Br and Sb
The importance of this measurement should not be underestimated as this will discard a majority of WEEE-contaminated FCMs from the market as BFRs are intensively used in electrical and electronic applications. Energy-disperse XRF spectroscopy (ED-XRF) is already widely used for the detection of RoHS-restricted elements in WEEE. This technique has the advantage of being non-destructive, quick and possibly applicable in situ; however, benchtop instruments deliver superior resolution. Although the LODs are relatively low, the method is limited by its specificity; there is the inherent risk that the Br signal might not come from the BFRs and the Sb signal does not belong to the Sb2O3 additive. Taking into account the Br–Sb synergic relationship, there will be an indication. Therefore, the methodical coupling to chromatographic techniques is required to confirm the presence of BFR, implicating Br content as a proxy for specific BFRs. There are many hand-held and benchtop XRF devices on the market, and they have proven their practical use (Fink et al. Citation2000; Wolksa et al. Citation2005; Samsonek et al. 2007; Stapleton et al. Citation2011; Guzzonato et al. Citation2016b).
Guzzontao et al. (2016c) improved the precision of the XRF measurement by using thickness correction and correlating this to laser ablation inductively coupled plasma spectrometry-mass spectrometry (LA-ICP-MS) on standards and real samples. They proposed a practical solution to the need for an accurate, yet rapid and inexpensive, technique for the quantification of BFRs in waste materials in terms of Br rather than BFR concentration (based on a conservative assumption about the BFR presence). Commercial (XRF) instruments are capable of monitoring preferably in situ, meeting the required LODs from in the lower mg kg−1 region. The XRF analyses methods are described in our previous studies; however, briefly for explanation, the 2015 study was performed using a Shimadzu EDX-800P spectrometer (Kyoto, Japan) equipped with an Si(Li) semiconductor detector cooled by liquid nitrogen. The instrument parameters were optimised for measuring total Br in a hydrocarbon matrix with an acceleration voltage of the X-ray tube with an Rh target set to 50 kV. The current towards the filament was approximately 120 µA, having the X-rays filtered by a primary Ag filter for background reduction. For Br, the Kα spectral line at 11.92 KeV was the most sensitive and, therefore, chosen as the analytical line for quantification. Special attention should be taken as this line can be overlapped by the Kβ lines from As and also by the Lβ1 and Lβ2 lines from Hg. Such possible overlaps can be corrected by using the data-processing functionality of Shimadzu’s PCEDX software (v.1.02). Interferences are then automatically subtracted from the Kα peak of Br if necessary. For Sb measurement, the Kα spectral line at 26.36 KeV is generally used because it is expected to be free of significant interferences. For calibration of the XRF spectrometer, an RoHS standard set of Sumika Chemical Analysis Service, Ltd (Osaka, Japan) was used containing six PE standards with a calibration range of approximately 0–1200 mg kg−1 Br. To obtain intensity data, the peak area between 11.66 and 12.16 KeV was fitted to a Gaussian curve implementing an internal standard background compensation. It is recommended to use as a control measurement the certified reference materials ERM®-EC591 (Voorspoels et al. Citation2008; JRC-IRMM, Citation2009), which is a PBDE/PBB/Sb2O3-doped PP in pellet form with an overall Br concentration of 2.08 ± 0.07 g kg−1 and 0.713 ± 0.022 g kg−1 Sb. The certified reference material ERM-EC680K PE pellets (JRC-IRMM, 2007) spiked with inorganic pigments have an elemental Br concentration of 96 ± 4 mg kg−1, and 10.1 ± 1.6 mg kg−1 of Sb (from Sb2O3) is suitable for this application as well. The conversion of the reference pellets into a thick film is recommended to unify the measurement design as test points of isolated samples will have the same geometric appearance.
Thermal desorption (pyrolysis) GC-MS: identification of BFRs
The combination of methods using XRF and thermal desorption GC-MS as a substance-specific method has been evaluated as being efficient for RoHS screening purposes, including several common BFRs at a major level (De Percin Citation1995; Hosaka et al. Citation2005; Samsonek et al. Citation2007; Kemmlein et al. Citation2009), but also can be used to detect traces of BFRs. This method includes drawbacks based on sample-to-sample matrix variance resulting in different recovery rates and in many cases dissolution of the polymer and its impurities (e.g., styrenic polymers). As the thermal desorption measurement is able to screen common BFRs, the threshold can be measured by a combination of methods like XRF and LA.
Thermal desorption using a semi-quantitative method overcomes analytical challenges like different solvent extraction efficiencies between samples (unknown polymer hardness) and analyte dependent solubility variations are omitted (polar–apolar solvent discrimination effects). Our procedure is described in previous work (Samsonek & Puype. Citation2013; Puype et al. Citation2015), but briefly explained here. Thermal desorption as an extraction and sample introduction technique uses heat at a moderate level (up to 350ºC) in order to evolve non-covalent bound additives like additive BFRs. When the same hardware is used at an elevated temperature (at 650ºC), the carbon covalent bounds are cleaved by pyrolysis processes including radical chain reaction and H abstraction; accordingly, the fragments are swept on the analytical column for separation and detection with MS. Pyrolysis GC-MS will further be described as a method identifying sample matrices and macromolecular contaminants, but can be used to identify newer polymeric BFRs like brominated polystyrene or when adding tetramethyl ammonium hydroxide (TMAH)-reactive pyrolysis can be used to confirm end-capped-brominated epoxy-based polymers based on tetrabromobisphenol A diglycidyl ether (Cheng-Yu Wang Citation2000; Herrera et al. Citation2003; Puype & Samsonek Citation2013; Puype et al. Citation2014).
Practically, from the selected test points, samples were taken by cutting about 2 mm by side, and 0.2 g of sample were dissolved in 1 ml of toluene (GC/ECD-grade residue analysis; Chromservis s.r.o., Prague, Czech Republic), which is a solvent enabling high extraction yields for all targeted BFRs. All extracts were kept static for a few days at RT under darkness in order to avoid photodegradation (Stapleton et al. 2008b) of the higher brominated PBDEs and shaken regularly. From the supernatant, 80 μl of toluene solution were spiked into the sample cup and dried at RT before injection. Extracts are a cleaner way to introduce sample into the GC-MS; however, small solid samples of a few mg could be introduced as well. Within our studies we used a thermal desorption unit (Multi-Shot Pyrolyzer EGA/PY-3030D, Frontier Laboratories Ltd, Koriyama, Japan) equipped with a 48-position autosampler (Auto-Shot Sampler AS-1020E, Frontier Laboratories) hyphenated to GC-MS (GC-MS QP2010 Plus, Shimadzu). Initially, the toluene extract from the polymer sample or the solid sample was spiked in a metallic 80-μl sample cup coated with fused silica (< 1 μm thickness) and evaporated till dry at RT. The great advantage of using extracts is that a very thin film of oligomers and non-volatile target analytes remains in the sample cup, which is giving significantly cleaner chromatograms than direct liquid sample injections or thermal desorption of the polymer sample itself. By a free-fall injection the cup enters quickly the heated furnace of the thermal desorption unit, which is sequentially heated using a temperature program up till 350ºC. BFRs like higher brominated PBDEs are heat sensitive and tend to debrominate at elevated temperatures; therefore, a special separation column achieving a short residence time is used (Ultra ALLOY-PBDE; 0.25 mm inner diameter × 15 m; 0.05 μm film of dimethyl polysiloxane, Frontier Laboratories). The peaks were monitored using the fast automated full-scan single-ion monitoring technique (FASST) mode, which enables one to acquire alternate both full-scan (SCAN) and single-ion monitoring (SIM) data on one peak. As an advantage, this method enables the detection of non-target analytes at the same time and additional information can be collected from one measurement. This additional possibility to obtain information about other non-target peaks can lead to conclusions such as the presence of polymeric BFRs (like oligomers from dibromostyrene copolymer or fragments of TBBPA-based epoxy resins), and reactive BFRs (like tetrabromobisphenol A, tetrabromophtalic anhydride and derivates). The FASST mode can be used for BFR determination in recycled polymers very effectively as BFRs appear there mostly as debrominated products due to the thermal reprocessing or photodegradation during their previous lifetime. As an example, TBBPA in recycled plastics gives in the chromatogram mostly peaks of mono-, di- and tri-bromobisphenol A due to thermal debromination of the target analyte TBBPA. The SIM method, as a part of the FASST mode, has been programmed so that most of the actual abundant BFRs could be monitored including PBDEs, PBBs, TBBPA and DBDPE (Puype & Samsonek Citation2008).
In order to reflect realistic conditions, for the purpose of checking sensitivity and carryover, and mainly as a proof of concept, polymer reference material doped with BFRs was used. Each measurement is joined with a measurement of the certified reference material ERM®-EC591 (the European Commission, Joint Research Centre – Institute for Reference Materials and Measurements (JRC-IRMM), Geel, Belgium; JRC-IRMM Citation2009), which are PP pellets containing 700 mg kg−1 technical pentaBDE, 200 mg kg−1 technical octaBDE, 700 mg kg−1 technical decaBDE and 700 mg kg−1 technical decaBB. From the analytical point of view, this method is limited to BFR identification and is not recommended for precise BFR quantification as polymers have a very diverse hardness/polarity and result in variable extraction efficiencies for each BFR, which makes the calibration for the quantitative measurement of unknown BFRs in different polymer matrices nearly impossible. Fortunately, exact quantification is not the key factor during market control as there is no limitation in BFR (or WEEE) dosing; the simple presence of such substances means that the sample does not fulfil the requirements of Regulation (EU) 10/2011.
ICP-OES analysis: REEs and WEEE-relevant elements
In order to enclose the elements from the polymeric matrix, acid digestion was performed in our previous study by use of a microwave-assisted digestion system (Speedwave four with DAK-100/4 polytetrafluoroethylene closed vessels; Berghof, Eningen, Germany). All samples were acid digested and measured in triplicate in order to overcome inhomogeneity as WEEE fractions mixed into a polymer are based on smaller parts after a shredding step before extrusion. A minimal sample weight of 0.25 g was acid digested by adding 8 ml of HNO3 (67 weight %; Analpure, Analytica, Czech Republic) and 2 ml of hydrogen peroxide (30 weight %; Lachner, Czech Republic). Joined with the samples, two certified reference materials were acid digested (ERM-EC591 and ERM-EC680K) (JRC-IRMM), which is a polyolefin matrix containing As, Cd, Cr, Hg, Pb, Sn, Zn and Sb achieving a recovery of 80–120% interval for a triple measurement. Note that there was no certified reference material available for polymeric WEEE containing all target REEs. The selected WEEE-relevant elements for monitoring were As, Be, Cd, Cu, Cr, Fe, Hg, Ni, Pb, Sb, Zn (non-REEs) and Ce, Dy, Er, La, Nd, Pr, Y (REEs) due to their abundance in WEEE. All measurements were performed by ICP-OES (ICPE-9820, Shimadzu) having a simultaneous axially and radially emission path. Both plasma observations can be combined in a single method, which means traces can be measured using a real axial view and major elements for the same sample by using real radial view. Special attention should be taken for possible spectral interferences between the analytes themselves. They were considered using an external-permuting calibration model implementing several calibration levels randomly. By this calibration strategy the use of interfering spectral lines can be eliminated. Moreover, the evaluation of REEs’ analytical spectral lines/analytical lines has been done in solutions containing high levels of Fe, Al, Ti, Cr, Mn, Co, Ni, Cu, Zn, Zr, Nb, Ag, Sn, Au and Pb, respectively. For major elements (Cr, Cu, Sb) with suspected interferences, decadal dilutions were used by checking the signal linearity. The hydride-forming elements at trace levels (Hg, As) were analysed by using the hydride vapour generator technique (HVG) by using the HVG-1 device (Shimadzu) connected to the ICP-OES. Using this technique, matrix interferences are almost unexpected due to the effective matrix separation.
ATR-FTIR analysis and pyrolysis GC-MS for the identification of macromolecular impurities
A small part of the sample was taken from the sample matrix and measured for ATR-FTIR and pyrolysis GC-MS. The quickest but less sensitive method is ATR-FTIR, while pyrolysis GC-MS requires a more complicated hardware and interpretation (preferably knowledge of possible pyrolysis processes). ATR-FTIR analysis was performed using a Shimadzu IRPrestige FTIR spectrophotometer equipped with a single-reflectance diamond ATR crystal achieving a wave number resolution of 2 cm−1 by using the Happ–Genzel transformation as apodisation function. The water peaks were subtracted using the atmosphere correction mode. All spectra were compared with standard spectra from databases which are commercially available, e.g., RoHS, ATR-Polymer2, IRs Polymer2 and T-Polymer2, all running on LabSolutions IR software (Shimadzu) combined with in-house libraries. For the identification of the main polymers, the match with the libraries was 90% or higher. Pyrolysis GC-MS can detect monomers and bigger pyrolysis degradation products (oligomers and their specific chromatographic pattern) to obtain a full compositional overview of the polymer matrix. The hardware configuration is the same as used for the thermal desorption GC-MS experiment (PY-2020iD, Frontier Laboratories; GC-MS QP2010 Plus, Shimadzu). The peaks from the pyrograms were identified by using the NIST 05 library (National Institute for Standards and Technology, USA) with a minimal match of 80% in combination with retention time comparison and oligomeric distribution patterns from pyrograms of known polymer matrices. Generally, in our previous study we focused on certain groups of polymers: HIPS, co-polymer of acrylonitrile-styrene (SAN), ABS, polybutylene terephthalate (PBT), PET, polyamide-6 (PA6), polymethylmethacrylate (PMMA) and PP/PE. The pyrograms and FTIR spectra gave in certain cases spectral patterns that might come from co-polymers or other polymer fractions. These data should be interpreted with care. The direct relation between polymer purity and WEEE additions cannot be confirmed; therefore, we used this parameter with the aim to detect foreign polymeric material (both WEEE and non-WEEE factions).
Results and discussion
Based on the combined data from our two previous studies (Samsonek & Puype Citation2013; Puype et al. Citation2015) measuring WEEE-relevant contaminants in WEEE, from the 40 selected samples (including 49 test points), 22 samples were positive for Br (XRF data). As an advantage, in order to build an efficient control process mainly based on the detection of Br via XRF spectroscopy, XRF has very well optimised measurement conditions in the range from approximately 7 to 12 keV. In this spectral region the Br lines have very high sensitivity. The same counts for as Sb as well. Taking Br detection as a proxy for BFRs, the presence of BFRs could be confirmed for 21 samples. From these 21 samples, decaBDE and TBBPA gave the highest abundance (15 times) where they appeared together in 11 samples. The recent decaBDE replacement, DBPDE appeared five times generally joined as a BFR mixture with decaBDE. Newer BFRs like BTBPE and TBBPA-BDBPE were detected as well (three times), but generally, except for one sample, they joined with its precedent decaBDE. The appearance of small concentrations of BFRs might indicate small additions of WEEE; moreover, the mixture of BFRs indicates a mixture of several samples.
As an example (), the chromatogram of a black thermocup cover (sliding stopper) made of ABS containing 1521 mg kg−1 Br using thermal desorption in the FASST mode gave a majority of TBBPA (a) detected with traces of decaBDE (b) and its commercial replacement DBDPE (c). All obtained peaks were joined with their full-scan electron-impact mass spectrum. As this plastic FCM contains a mixture of BFRs, this sample is supposed to contain small additions of WEEE.
Figure 2. Fast automated full-scan single-ion monitoring technique (FASST) data obtained by thermal desorption GC-MS from a black thermocup cover (sliding stopper) purchased on the European market.
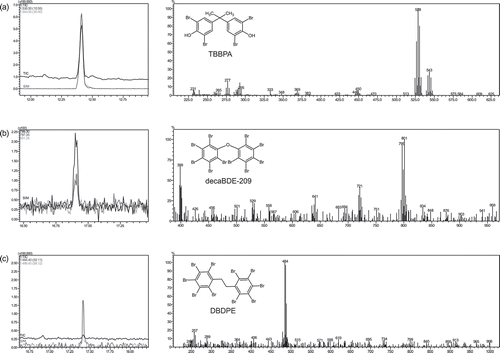
From seven Br-positive samples, four contained Sb (taking the data from our study from 2015). The presence of Sb should be considered as complementary WEEE indications as the Br concentration must be elevated enough in order to detect Sb. The concentration of Sb is generally lower than the overall Br content and might fall below the LOD of the instrument (being generally 2 mg kg−1 for Br and 10 mg kg−1 for Sb), depending on the spectrometer’s sensitivity. The opposite could be stated: when Sb was detected, generally Br (and BFRs) was detected as well. Note that except for PET matrices, Sb has no reason to be added to a polymer; moreover, in conjunction with Br, there is no reason to make a plastic FCM flame retardant – this is also illegal.
Concerning the detection of REEs, four of the seven BFR-positive samples contains one to five of the selected REEs. The presence of REEs should be taken with care as not all WEEE does contain sWEEE (mobile phones, printed circuit boards, LED lights, monitors); however, there are indications when detecting several combinations of REEs: Ce, Dy, La and Y are used in LEDs; Ce, La and Y are used in fluorescent powder from lighting components and television sets; batteries from consumer goods like cameras and laptop computers contain Ce, La, Nd and Pr; Nd is a specific element used for permanent magnets in microphones, professional loudspeakers, in-ear headphones and computer hard disks; and Pr is used in some applications together with Nd due to their excellent magnetic properties (Goodship & Stevels, Citation2012). There is currently a high economical interest in urban mining of REEs, where REE waste fractions are recollected and recycled. Despite the huge efforts to perform this practice in a controlled way, many illegal domestic and backyard recycling is still occurring, generally in overseas countries (Yoshida Citation2011; Duan et al. Citation2016). This results in a growing uncontrolled trade with WEEE-related polymeric waste streams, aluminium, ferrous and non-ferrous metals (Chancerel et al. Citation2009; Guo et al. Citation2009; Dwivedy & Mittal Citation2010; Kahhat & Williams Citation2012).
The appearance of non-REEs like Cu might indicate traces from cabling and connectors, while ferrous metals are mainly used in castings, magnets and magnetic coils. Be is used mainly in metalloid applications like Be/Cu alloys, Be oxide-ceramic or as metallic Be. REEs generally confirm sWEEE contamination.
At a third level, polymer purity could not fully cover WEEE and sWEEE detection as this parameter also indicates polymer fractions in non-BFR-containing samples. Note that foreign polymer recycled fractions might have been used without a relation to WEEE. On the other hand, the polymers might be a co-polymer and in certain samples this information is unknown. Even though the sampling was focused on mainly dark items, the outcome confirmed that 40% of the samples selected from the European market contain BFRs that are typical for WEEE plastic material. High-risk polymers are co-polymers of ABS and PP/PE. This is not a strict indication for WEEE, but might come from other polymeric waste fractions (non-WEEE). Guzzonato et al. (Citation2017; data not published yet) performed a study on WEEE-relevant element presence (including REEs) and inorganic filler identification (CaCO3 and TiO2) using principal component analysis (PCA) with a categorisation into defined polymer classes. The outcome of the study was that the bi-plot gave a similar grouping vector of mainly two elements: Br and Sb.
Conclusions
Based on the measured data and recent available literature a generic procedure for WEEE evaluation in plastic FCMs has been proposed (). After taking into consideration criteria such as colour, smell, fragility or availability of documentation, the sample/test points for evaluation are selected. It is recommended to follow the proposed importance-level structure methodology and, therefore, at first the Br content should be evaluated preferably in situ with optionally screening for Sb as added in a parallel confirmatory loop. When BFRs are confirmed to be present, it is obvious that WEEE has been used implementing directly that the Br content was a proxy for BFRs. In the scheme also PFRs are added to be screened delivering optional information about the sample addition as PFRs form another class of FRs as well. We also need to take into account, when BFRs could not be detected, that Br signals might come from certain brominated substances appearing in Annex 1 of Regulation (EU) 10/2011 and, therefore, specific migration tests should be performed in case of doubt or lack of information. The same scenario counts if the sample is positive for Sb, then the migration test according to Regulation (EU) 10/2011 should be applied. The advantage of this first importance level is that both parameters can be primary measured in situ. Another aspect is the lead time of the evaluation, although this method is not a precise measurement, the results are rather in-time readable and, therefore, do not require a time-consuming acid digestion or other sample preparation (like extractions and sample clean-up) in advance. If none of these substances is detected, it is recommended to measure WEEE-related elements and relevant REEs at a second importance level. There is a potential link to sWEEE; however, this parameter should be cleared out in a next step by the recommended conformity assessment procedure. At a third importance level the purity of the polymer must be evaluated, with general knowledge of the availability of commercial co-polymers, foreign fractions might be determined. The obtained FTIR or pyrolysis GC-MS data will never distinguish with a high level of confidence the source being a WEEE or non-WEEE-recycled fraction.
Figure 3. Generic procedure for the evaluation of waste electric and electronic equipment (WEEE) in plastic food-contact materials (FCMs), including an antimony (Sb) loop for the synergetic confirmation, taking into consider principles of Regulation (EU) 10/2011 (plastic FCMs) and Regulation (EC) 282/2008 (recycled plastic FCMs).
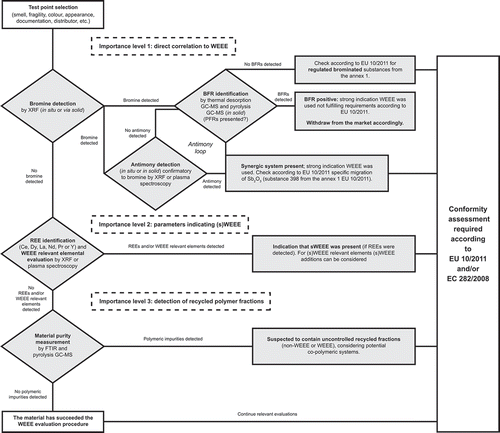
In all cases, if at least one of the importance levels’ evaluation gives a positive result, then consulting the available documentation as a part of the conformity assessment is recommended. If the materials comply with the proposed parameters evaluated by this multi-methodical approach, the sample can be considered as WEEE free.
Further guidance on conformity assessment of plastic FCMs can be evaluated according to Regulation (EU) 10/2011 Chapter II, Section 1, Article 5, describing the EU list of authorised substances, where only the substances included in the EU list of authorised substances (hereinafter referred to as the Union list) set out in Annex I may be intentionally used in the manufacturing of plastic layers in plastic materials and articles. The Union list should contain: (1) monomers or other starting substances; (2) additives excluding colorants; (3) PPAs excluding solvents; and (4) macromolecules obtained from microbial fermentation. The Union list may be amended in accordance with the procedure established by Articles 8–12 of Regulation (EC) 1935/2004/EC. When BFRs are detected in the sample, the evaluated sample clearly does not conform to Chapter II, Section 1, Article 5, ‘EU list of authorised substances’, as no BFRs are allowed to be used in the manufacturing of the plastic FCMs.
Another piece of legislation is the conformity assessment procedure for recycled material according to Regulation (EC) 282/2008, where under Clause 14 it is described that plastic waste may be contaminated by substances from the previous use or incidental misuse of the plastics or by substances originating from non-food contact-grade plastic. As it is not possible to know all possible types of contamination and as different types of plastics have different capacities to retain and release contaminants, it is not possible to set defined characteristics for the final product applicable to all types of recycled plastics. Therefore, a combination of input characterisation together with an adequate process to remove possible contamination is necessary to control the safety of the final product. Article 16 of Regulation (EC) 1935/2004/EC requires a declaration of compliance (DoC) for FCMs and articles. The converter of recycled plastic material and articles should declare that he is using only recycled plastic from an authorised process and that the final product respects European Community and national provisions applicable to it, in particular Regulation (EC) 1935/2004/EC and Regulation (EU) 10/2011 replacing Directive 2002/72/EC (European Commission Citation2002b). The recycler should provide the converter with the information that the recycled plastic is produced by an authorised process and specify its field of application. Therefore, both the finished recycled plastic materials and articles and the recycled plastic should be accompanied by a DoC. All required information to be provided in the DoC is already laid down in Annex IV of Regulation (EU) 10/2011 and Annex I of Regulation (EC) 282/2008. As the recycling of WEEE is not an authorised process, the presence of WEEE must be seen as a violation against Regulation (EC) 1935/2004/EC and Regulation (EC) 282/2008.
Our generic procedure can be applied for the evaluation of WEEE plastic waste streams in plastic FCMs as an evaluation tool. Mainly sanitary authorities who are dealing with consumer safety should take action when it concerns putting safe, regulated products onto the market within the EU. In order to minimise the health risks, we propose to the sanitary authorities that each consignment of such products should be accompanied by appropriate documentation, including analytical results showing that it meets the requirements concerning our generic procedure by at least mandatory Br measurement by XRF spectroscopy for suspicious plastic FCMs at first.
A further step would be to follow the approach from Commission Regulation (EU) (European Commission Citation2011e) No. 284/2011, which monitors consumer goods on selected parameters like primary aromatic amines and formaldehyde for polyamide and melamine plastic kitchenware originating in or consigned from overseas countries entering the EU (European Commission Citation2011d).
Acknowledgements
The authors thank the International Life Sciences Institute (ILSI) scientific committee and, especially, Dr Marsaux for the invitation to present our data and findings in this journal.
Disclosure statement
No potential conflict of interest was reported by the authors.
References
- Ballesteros-Gómez A, De Boer J, Leonards PE. 2016. Novel analytical methods for flame retardants and plasticizers based on gas chromatography, comprehensive two-dimensional gas chromatography, and direct probe coupled to atmospheric pressure chemical ionization-high resolution time-of-flight-mass spectrometry. Anal Chem. 85:9572–9580.
- Barthélémy E, Spyropoulos D, Milana MR, Pfaff K, Gontard N, Lampi E, Castle L. 2014. Safety evaluation of mechanical recycling processes used to produce polyethylene terephthalate (PET) intended for food contact applications. Food Addit Contam Part A. 31:490–497.
- Bergman Å, Rydén A, Law RJ, De Boer J, Covaci A, Alaee M, Birnbaum L, Petreas M, Rose M, Sakai S, et al. 2012. A novel abbreviation standard for organobromine, organochlorine and organophosphorus flame retardants and some characteristics of the chemicals. Environ Int. 49C:57–82.
- Binnemans K, Dupont D, Arnout S, Jones PT. 2016. Antimony recovery from end-of-life products and industrial process residues: a critical review. J Sustain Metall. 2–1:79–103.
- Buekens A, Yang J. 2014. Recycling of WEEE plastics: a review. J Mater Cycles Waste. 16:415–434.
- Camino G, Costa L, Luda Di Cortemiglia MP. 1991. Thermal stabilization and flammability of polymers and composites: overview of fire retardant mechanisms. Polym Degrad Stab. 33:131–154.
- Chancerel P, Meskers CEM, Hagelüken C, Rotter VS. 2009. Assessment of precious metal flows during pre-processing of waste electrical and electronic equipment. J Ind Ecol. 13:791–810.
- Cheng-Yu Wang F. 2000. Polymer additive analysis by pyrolysis–gas chromatography: II. J Chromatogr. 886:225–235.
- Christian B, Romanova I, Turbini L 2012. Elemental compositions of over two dozen cell phones, as originally published in the IPC APEX EXPO proceedings. Available from: https://www.smtnet.com/library/files/upload/cell-phones-composition.pdf
- Covaci A, Gerecke AC, Law RJ, Voorspoels S, Kohler M, Heeb NV, Leslie H, Allchin CR, de Boer J. 2006. Hexabromocyclododecanes (HBCDs) in the environment and humans: a review. Environ Sci Technol. 40:3679–3688.
- Darnerud PA. 2003. Toxic effects of brominated flame retardants in man and in wildlife. Environ Int. 29:841–853.
- De Percin PR. 1995. Application of thermal desorption technologies to hazardous waste sites. J Hazard Mater. 40:203–209.
- Dimitrakakis E, Janz A, Bilitewski B, Gidarakos E. 2009. Small WEEE: determining recyclables and hazardous substances in plastics. J Hazard Mater. 161:913–919.
- Duan H, Hu J, Tan Q, Liu L, Wang Y, Li J. 2016. Systematic characterization of generation and management of e-waste in China. Environ Sci Pollut Res. 23:1929–1943.
- Dwivedy M, Mittal RK. 2010. Estimation of future outflows of e-waste in India. Waste Manag. 30:483–491.
- Egebäck AL, Sellström U, McLachlan MS. 2012. Decabromodiphenyl ethane and decabromodiphenyl ether in Swedish background air. Chemosphere. 86:264–269.
- European Commission. 2002a. Directive (EC) no. 2002/95/EC of the European parliament and of the council of 27 January 2003 on the restriction of the use of certain hazardous substances in electrical and electronic equipment. Off J Eur Union L. 37:19.
- European Commission. 2002b. Commission Directive 2002/72/EC of 6 August 2002 relating to plastic materials and articles intended to come into contact with foodstuffs (Text with EEA relevance). Oj L 220. 15.8:18–58.
- European Commission. 2008. European regulation (EC) No 282/2008 of 27 March 2008 on recycled plastic materials and articles intended to come into contact with foods and amending regulation (EC) no 2023/2006. Off J Eur Union L. 86:9.
- European Commission. 2011a. Regulation (EC) No. 10/2011 of 14 January 2011 on plastic materials and articles intended to come into contact with food. Off J Eur Union. L. 39:41.
- European Commission. 2011b. Regulation (EC) No. 321/2011 of 2 April 2011 amending and correcting Regulation (EU) No 10/2011 on plastic materials and articles intended to come into contact with food. Off J Eur Union. L87:1.
- European Commission. 2011c. Regulation (EC) No. 1282/2011 of 28 November 2011 amending and correcting Regulation (EU) No 10/2011 on plastic materials and articles intended to come into contact with food. Off J Eur Union. L328:22.
- European Commission. 2011d. Directive (EC) No. 2011/65/EU of the European Parliament and of the Council of 8 June 2011 on the restriction of the use of certain hazardous substances in electrical and electronic equipment. Off J Eur Union. L179:88.
- European Commission. 2011e. Commission regulation (EU) No 284/2011 of 22 March 2011 laying down specific conditions and detailed procedures for the import of polyamide and melamine plastic kitchenware originating in or consigned from the People’s Republic of China and Hong Kong special administrative region, China. Off J Eur Union L. 77:22–25.
- European Commission. 2012. Directive (EC) No. 1183/2012/EU of 30 November 2012 amending and correcting Regulation (EU) No 10/2011 on plastic materials and articles intended to come into contact with food. Off J Eur Union. L338:11.
- European Commission. 2014a. Regulation (EC) no. 202/2014 of 3 March 2014 amending European commission regulation (EU) no. 10/2011 on plastic materials and articles intended to come into contact with food. Off J Eur Union. L 62:13.
- European Commission. 2014b. Regulation (EC) no. 865/2014 of 8 August 2014 amending European commission regulation (EU) no. 10/2011 on plastic materials and articles intended to come into contact with food. Off J Eur Union. L 238:1.
- European Commission. 2014c. Recommendation No. 2014/118/EU of 3 March 2014 on the monitoring of traces of brominated flame retardants in food. Off J Eur Union L. 65:39.
- European Commission. 2015. Regulation (EC) no. 2015/174 of 5 February 2015 amending European commission regulation (EU) no. 10/2011 on plastic materials and articles intended to come into contact with food. Off J Eur Union L. 30:2.
- European Commission. 2016. Commission regulation (EU) 2016/1416 of 24 August 2016 amending and correcting regulation (EU) no 10/2011 on plastic materials and articles intended to come into contact with food (Text with EEA relevance). Off J Eur Union L. 230:22–42.
- European Commission, Joint Research Centre – Institute for Reference Materials and Measurements (JRC-IRMM). 2007. CERTIFICATION Report The certification of the mass fractions of As, Br, Cd, Cl, Cr, Hg, Pb, S and Sb and the assignment of indicative values for Sn and Zn in two polyethylene reference materials Certified Reference Materials, ERM®-EC680k and ERM®-EC681k, ISSN 1018-5593, Available from: http://publications.jrc.ec.europa.eu/repository/bitstream/JRC37540/7540%20-%20ERM-EC680k%20%20ERM-EC681k_report_complete.pdf
- European Commission, Joint Research Centre – Institute for Reference Materials and Measurements (JRC-IRMM). 2009. CERTIFICATION Report Certification of the mass fractions of various polybrominated diphenyl ethers (PBDEs), decabrominated biphenyl and total Br and total Sb in two polymer reference materials Certified Reference Materials ERM®-EC590 & ERM®-EC591, EUR 23677 EN, ISBN 978-92-79-11105-1, ISSN 1018-5593, DOI 10.2787/9764, Available from: https://ec.europa.eu/jrc/sites/default/files/rm/ERM-EC590_report.pdf
- European Union. 2004. EU parliament and council directive 1935/2004 of 27 October 2004. Oj L. 338/4. [ cited 2004 Nov 13].
- Fink H, Panne U, Theisen M, Niessner R, Probst T, Lin X. 2000. Determination of metal additives and bromine in recycled thermoplastics from electronic waste by TXRF analysis. Fresen J Anal Chem. 368:235–239.
- Gallen C, Banks A, Brandsma S, Baduel C, Thai P, Eaglesham G, Heffernan A, Leonards PE, Bainton P, Mueller JF. 2014. Towards development of a rapid and effective non-destructive testing strategy to identify brominated flame retardants in the plastics of consumer products. Sci Total Environ. 491–492:255–265.
- Gieroń J, Grochowalski A, Chrząszcz R. 2010. PBB levels in fish from the Baltic and North seas and in selected food products from Poland. Chemosphere. 78:1272–1278.
- Goodship V, Stevels A. 2012. Waste electrical and electronic equipment (WEEE) handbook. Woodhead publishing series in Electronic and optical materials. ISSN 2050-1501. Woodhead Publishing. Available from: https://www.elsevier.com/books/waste-electrical-and-electronic-equipment-weee-handbook/goodship/978-0-85709-089-8
- Groß R, Bunke D, Gensch CO, Zangl S, Manhart A. 2008. Study on hazardous substances in electrical and electronic equipment, not regulated by the RoHS directive. Study made at the Öko-Institut e.V. (Head Office Freiburg). Contract No. 070307/2007/476836/MAR/G4. Final report. Freiburg: Öko-Institut. Available from http://ec.europa.eu/environment/waste/weee/pdf/hazardous_substances_report.pdf
- Guo J, Guo J, Xu Z. 2009. Recycling of non-metallic fractions from waste printed circuit boards: a review. J Hazard Mater. 168:567–590.
- Guzzonato A, Mehlmann H, Krumwiede D, Harrad S. 2016a. A novel method for quantification of decabromodiphenyl ether in plastics without sample preparation using direct insertion probe – magnetic sector high resolution mass spectrometry. Anal Methods. 8:5487–5494.
- Guzzonato A, Puype F, Harrad S. 2016c. Pushing the limits of hand-held X-ray fluorescence spectrometers to quantify BFRs in WEEE. Organohalogen Compd. 78:15–18.
- Guzzonato A, Puype F, Harrad SJ. 2016b. Improving the accuracy of hand-held X-ray fluorescence spectrometers as a tool for monitoring brominated flame retardants in waste polymers. Chemosphere. 159:89–95.
- Guzzonato A, Puype F, Harrad SJ. 2017. Evidence of bad recycling practices: BFRs in children’s toys and food-contact articles. Environmental Science: Processes & Impacts.
- Harju M, Hamers T, Kamstra JH, Sonneveld E, Boon JP, Tysklind M, Andersson PL. 2007. Quantitative structureactivity relationship modeling on in vitro endocrine effects and metabolic stability involving 26 selected brominated flame retardants. Environ Toxicol Chem. 26:816–826.
- Herrera M, Matuschek G, Kettrup A. 2003. Fast identification of polymer additives by pyrolysis-gas chromatography/mass spectrometry. J Anal Appl Pyrolysis. 70:35–42.
- Hindersinn RR. 1990. Historical aspects of polymer fire retardance. In: Nelson GL, editor. Fire and polymers hazard identification and prevention. American chemical society symposium series. New York (NY): American Chemical Society.
- Hirai Y, Sakai S 2007. Brominated flame retardants in recycled plastic products. BFR 2007. Paper Presented at: the 4th International Symposium on Brominated Flame Retardants; Amsterdam, the Netherlands.
- Hosaka A, Watanabe C, Tsung S. 2005. Rapid determination of decabromodiphenyl ether in polystyrene by thermal desorption- GC/MS. Anal Sci. 21:1145–1147.
- International Electrotechnical Commission (IEC), 2008, IEC 62321 Ed.1: ‘Electrotechnical products – Determination of levels of six regulated substances (lead, mercury, cadmium, hexavalent chromium, polybrominated biphenyls, polybrominated diphenyl ethers)’, IEC/FDIS 2008. Available from: http://webstore.iec.ch/webstore/webstore.nsf/Artnum_PK/42411
- Jakab E, Uddin M, Bhaskar T, Sakata Y. 2003. Thermal decomposition of flame-retarded high-impact polystyrene. J Anal Appl Pyrolysis. 68–69:83–99.
- Kahhat R, Williams E. 2012. Materials flow analysis of e-waste: domestic flows and exports of used computers from the United States. Resour Conserv Recycl. 67:67–74.
- Kemmlein S, Herzke D, Law RJ. 2009. Brominated flame retardants in the European chemicals policy of REACH-Regulation and determination in materials. J Chromatogr. 1216:320–333.
- Keresztes S, Tatár E, Mihucz VG, Virág I, Majdik C, Záray G. 2009. Leaching of antimony from polyethylene terephthalate (PET) bottles into mineral water. Sci Total Environ. 407:4731–4735.
- Kierkegaard A, Björklund J, Fridén U. 2004. Identification of the flame retardant decabromodiphenyl ethane in the environment. Environ Sci Technol. 38:3247–3253.
- Knoop J, Opperman U, Schram J. 2014. Interference-free determination of REEs in electronic waste using ICP optical emission spectroscopy. J Chem Chem Eng. 8:635–640.
- Martinho G, Pires A, Saraiva L, Ribeiro R. 2012. Composition of plastics from waste electrical and electronic equipment (WEEE) by direct sampling. Waste Management. 32:1213–1217.
- Miller GZ, Tighe ME, Peaslee GF, Peña K, Gearhart J. 2016. Toys, décor, and more: evidence of hazardous electronic waste recycled into new consumer products. J Environ Prot (Irvine, Calif). 7:341–350.
- Muncke J. 2009. Exposure to endocrine disrupting compounds via the food chain: is packaging a relevant source? Sci Total Environ. 407:4549–4559.
- Paine MR, Rae ID, Blanksby SJ. 2014. Direct detection of brominated flame retardants from plastic e-waste using liquid extraction surface analysis mass spectrometry. Rapid Commun Mass Spectrom. 28:1203–1208.
- Pöhlein M, Bertran RU, Wolf M, Van Eldrik R. 2005. Rapid identification of RoHS relevant flame retardants from polymer housings by ultrasonic extraction and RPHPLC/UV. J Chromatogr A. 1066:111–117.
- Pritchard G. 1998. plastics additives. Chapman & Hall, ISBN. 0:72720 X.
- Puype F, Samsonek J. 2008. Identification of decabromodiphenyl ethane (DBDPE) in plastics by thermal desorption GC-MS. Organohalogen Compd. 70:914–917.
- Puype F, Samsonek J. 2013. The application of thermal desorption and reactive pyrolysis – gas chromatography – mass spectrometry for the identification of end-capped brominated expoxy based polymers based on tetrabromobisphenol A diglycidyl ether in PBT. Proceedings from PMA 2013 & SRC 2013. The official journal of the Association of Czech Chemical Societies.
- Puype F, Samsonek J, Ambros J. 2014. Internal standardization for analytical pyrolysis, thermal desorption and quantitative in-source desorption by electron impact ionization: improvements and strategies for polymer science. Proceedings from PLASTKO 2014. Zlín: Univerzita Tomáše Bati ve Zlíně (UTB)
- Puype F, Samsonek J, Knoop J, Egelkraut-Holtus M, Ortlieb M. 2015. Evidence of waste electrical and electronic equipment (WEEE) relevant substances in polymeric food-contact articles sold on the European market. Food Addit Contam: Part. 32:410–426.
- Ricklund N, Kierkegaard A, McLachlan MS. 2010. Levels and potential sources of decabromodiphenyl ethane (DBDPE) and decabromodiphenyl ether (decaBDE) in lake and marine sediments in Sweden. Environ Sci Technol. 44:1987–1991.
- Samsonek J, Puype F. 2013. Occurrence of brominated flame retardants in black thermos cups and selected kitchen utensils purchased on the European market. Food Addit Contam: Part. 30:1976–1986.
- Samsonek J, Puype F, Vít D. 2007. Rapid determination of certain BFRs in plastics by X-ray fluorescence spectrometry (XRF) and thermal desorption GC-MS (TD-GC-MS) for the RoHS directive. Organohalogen Compd. 69:2789–2792.
- Sato Y, Oki M, Kondo A, Takenaka M, Satake H. 2010. Rapid analysis of polybrominated diphenyl ethers by ion attachment mass spectrometry. Anal Methods. 2:701–706.
- Schecter A, Haffner D, Colacino J, Patel K, Päpke O, Opel M, Birnbaum L. 2009. Polybrominated diphenyl ethers (PBDEs) and hexabromocyclodecane (HBCD) in composite U.S. food samples. Environ Health Perspect. 118:357–362.
- Schlummer M, Gruber L, Mäurer A, Wolz G, van Eldik R. 2007. Characterisation of polymer fractions from waste electrical and electronic equipment (WEEE) and implications for waste management. Chemosphere. 67:1866–1876.
- Segev O, Kushmaro A, Brenner A. 2009. Review environmental impact of flame retardants (Persistence and biodegradability), int. J Environ Res Public Health. 6:478–491.
- Sindiku O, Babayemi J, Osibanjo O, Schlummer M, Schluep M, Watson A, Weber R. 2015. Polybrominated diphenyl ethers listed as Stockholm Convention POPs, other brominated flame retardants and heavy metals in e-waste polymers in Nigeria. Environ Sci Pollut Res Int. 22:14489–14501.
- Stapleton HM, Allen JG, Kelly SM, Konstantinov A, Klosterhaus S, Watkins D, McClean MD, Webster TF. 2008a. Alternate and new brominated flame retardants detected in U.S. house dust. Environ Sci Technol. 42:6910–6916. PMID.
- Stapleton HM, Dodder NG. 2008b. Photodegradation of decabromodiphenyl ether in house dust by natural sunlight. Environ Toxicol Chem. 27:306–312.
- Stapleton HM, Klosterhaus S, Keller A, Ferguson PL, van Bergen S, Cooper E, Webster TF, Blum A. 2011. Identification of flame retardants in polyurethane foam collected from baby products. Environ Sci Technol. 45:5323–5331.
- Szymańska JA, Piotrowski JK, Frydrych B. 1999. Hepatotoxicity of tetrabromobisphenol-A: effects of repeated dosage in rats. Toxicology. 142:87–95.
- Tan Q, Dong Q, Liu L, Song Q, Liang Y, Li J. 2017. Potential recycling availability and capacity assessment on typical metals in waste mobile phones: A current research study in China. J Clean Prod. 148:509–517.
- UNEP Report. 2013. Metal recycling: opportunities, limits, infrastructure, a report of the working group on the global metal flows to the international resource panel. Reuter. MA, Hudson C, van Schaik A, Heiskanen K, Meskers C, Hagelüken C. ISBN: 978-92-807-3267-2. Available from: http://www.unep.org/resourcepanel/Portals/24102/PDFs/Metal_Recycling_Full_Report.pdf
- US Patent 5077334, patent 1991. Flame-retarded formulations of thermoplastics and decabromodiphenylethane. from Ethyl Corp. [ cited 1991 Dec 31].
- Vilaplana F, Karlsson P, Ribes-Greus A, Ivarsson P, Karlsson S. 2008. Analysis of brominated flame retardants in styrenic polymers. Comparison of the extraction efficiency of ultrasonication, microwave-assisted extraction and pressurised liquid extraction. J Chromatogr A. 1196–1197:139–146.
- Voorspoels S, Linsinger T, Birgersson-Liebig A, Pellizzato F, Venelinov T, Lamberty A. 2008. Towards the first certified reference material for polybrominated diphenyl ethers (PBDEs) and polybrominated biphenyls (PBBs) in two types of polymers. Organohalogen Compd. 70:2248–2251.
- Vyzinkarova D, Brunner HP. 2013. Substance flow analysis of wastes containing polybrominated diphenyl ethers: the need for more information and for final sinks. J Ind Ecol. 17:900–911.
- Wäger P, Böni H, Buser A, Morf L, Schluep M, Streicher M. 2009. Recycling of plastics from waste electrical and electronic equipment (WEEE)—tentative results of a swiss study. In: R’09 twin world congress & world resources forum. Davos: EMPA Zurich.
- Wienold J, Recknagel S, Scharf H, Hoppe M, Michaelis M. 2011. Elemental analysis of printed circuit boards considering ROHS regulation. Waste Manag. 31:530–535.
- Wolksa J. 2005. Safeguarding the environment – XRF analysis of heavy metals in polyethylene. Plast Addit Compd. 7:36–39.
- Yamasue E, Nakajima K, Daigo I, Hashimoto S, Okumura H, Ishihara KN. 2007. Evaluation of the potential amounts of dissipated rare metals from WEEE in Japan. Mater Trans. 48–9:2353–2357.
- Yoshida A. 2011. Recyclable waste and goods trade of mainland China. Economic integration and recycling in asia: an interim report). Chosakenkyu Hokokusho, Institute of Developing Economies. Available from: http://www.ide.go.jp/Japanese/Publish/Download/Report/2010/pdf/2010_431_03.pdf