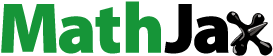
ABSTRACT
Insects are promising sources of protein and lipid in feeds for farmed animals. In the European Union, the use of insect meal (IM) and insect oil is permitted in fish feed. However, the European Food Safety Authority has highlighted the lack of data regarding the chemical safety of insects and products thereof. In this study, Atlantic salmon (Salmo salar) were fed diets in which fish meal (FM) was partially or fully substituted with IM, resulting in four diets with an FM replacement of 0%, 33%, 66% and 100% by IM. The IM was produced from Black soldier fly (Hermetia illucens) larvae fed media containing 60% seaweed (Ascophyllum nodosum). After 16 weeks of feeding, fish fillet samples were collected. The concentrations of undesirable substances, e.g., heavy metals, arsenic, dioxins, mycotoxins, pesticides, in the IM, the diets and fillets were determined. The concentrations of the analysed compounds in the IM were all below EU maximum levels for feed ingredients, except for arsenic. However, for complete feeds the concentrations of these compounds in the feeds, including arsenic, were all below EU MLs. Arsenic was transferred from seaweed to IM, resulting in arsenic levels in IM similar to what has been documented for FM. Transfer of arsenic from feed to fillet was observed; however, total arsenic concentrations in the fillet significantly decreased when fish were fed diets with more IM and less FM. Arsenic speciation analysis of the diets showed that although total arsenic levels were similar, the arsenic species were different. Arsenobetaine was the major organoarsenic species in the diets containing FM, while in diets containing IM several unidentified arsenic species were detected. The results suggest that the lower feed-to-fillet transfer of arsenic when FM is replaced by IM may be due to the presence of arsenic species with low bioavailability in the IM.
Graphical Abstract
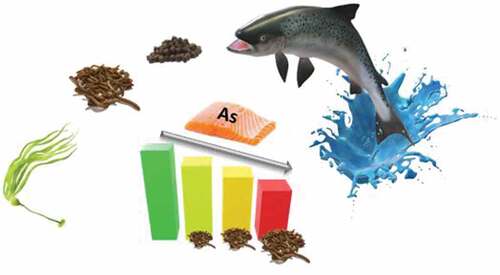
Introduction
Research on the use of insect meal (IM) in aquafeeds has developed rapidly in the last years, leading to an increased number of scientific contributions on this topic (van Huis Citation2013; Lock et al. Citation2016; Borgogno et al. Citation2017; Magalhães et al. Citation2017; Belghit et al. Citation2018a, Citation2018b). The European Union (EU) has recently allowed the use of insect-processed animal protein (PAP) in feed for aquaculture animals (EU Citation2017). The insect species allowed for the production of insect PAP are: Black soldier fly (Hermetia illucens, L.; BSF), Common Housefly (Musca domestica), Yellow Mealworm (Tenebrio molitor), Lesser Mealworm (Alphitobious diaperinus), House cricket (Acheta domesticus), Banded cricket (Gryllodes sigillatus) and Field Cricket (Gryllus assimilis). Among these species, BSF is considered as one of the most relevant species for the production of IM to be used in feed for salmonids, i.e., rainbow trout (Onchorhynchus mykiss) and Atlantic salmon (Salmo salar) (St-Hilaire et al. Citation2007; Henry et al. Citation2015; Lock et al. Citation2016, Citation2018; Renna et al. Citation2017; Belghit et al. Citation2018b). These fish species are typically fed on high-energy diets characterized by high levels of protein and lipid, and low levels of carbohydrates (Kaushik et al. Citation1989; Lock et al. Citation2018). Larvae of BSF typically contain high amounts of protein (~40% of dry weight, dw) and essential amino acids, the profile thereof is similar to fish meal (FM) (Barroso et al. Citation2014; Makkar et al. Citation2014). Moreover, BSF larvae can be a good source of lipid (~30% of dw), essential minerals (e.g., calcium, iron, magnesium, zinc, and phosphorus) and a variety of vitamins (A, D, E, K, C and B group vitamins), depending on the feeding medium (van Huis Citation2013; Henry et al. Citation2015; Kouřimská and Adámková Citation2016; Liland et al. Citation2017; Nogales-Mérida et al. Citation2018). Rearing BSF larvae on substrates of marine origin, such as seaweed, increases the levels of marine nutrients in the larvae, e.g., omega-3 polyunsaturated fatty acids, iodine and vitamin E (Liland et al. Citation2017). This could improve the quality of the IM used in feeds, and meet the dietary requirement of Atlantic salmon for these nutrients (Torstensen et al. Citation2004; Tocher Citation2010). It was recently demonstrated that IM and insect lipid from BSF larvae reared on seaweed-enriched media could be a potential future feed ingredient for Atlantic salmon (Belghit et al. Citation2018b). In an 8-week feeding trial, no negative effects on growth performance, body composition and nutrient digestibility were observed (Belghit et al. Citation2018b). When insects and products thereof are used in aquafeeds, they must meet the EU feed regulations. The EU has set maximum levels (MLs) for undesirable substances in feed ingredients and complete animal feed, covering several compounds such as heavy metals, arsenic, mycotoxins and persistent environmental pollutants (POPs) (e.g. dioxins and dioxin-like polychlorinated biphenyl (DL-PCBs)) (EC Directive 2002/32 and amendments) (EU Citation2002). Feed ingredients produced from insects can contain undesirable substances. These can be naturally present in insects or can be accumulated during rearing processes. However, knowledge on the potential hazards associated with farming insects is scant (Charlton et al. Citation2015; Diener et al. Citation2015; EFSA Citation2015; van der Fels-Klerx et al. Citation2016; Biancarosa et al. Citation2018b), as highlighted by the European Food Safety Authority (EFSA) in their risk profile (EFSA Citation2015).
In this study, seawater Atlantic salmon were fed diets in which FM was partially or fully replaced with IM, which was produced from BSF larvae fed on seaweed-enriched media. Seaweeds are known to accumulate heavy metals and the metalloid arsenic from the surrounding water (Villares et al. Citation2002; Rose et al. Citation2007; Maehre et al. Citation2014; Biancarosa et al. Citation2018a). It has previously been shown that BSF larvae accumulate heavy metals and arsenic from media containing seaweeds (Biancarosa et al. Citation2018b). Also, other studies on BSF have shown accumulation of heavy metals and arsenic in larvae fed feeding media spiked with these substances (Diener et al. Citation2015; Scheibelberger et al. Citation2017; Biancarosa et al. Citation2018b). Arsenic is an element of concern for feed and food safety, as some forms of arsenic, mainly inorganic arsenic, can be toxic to living organisms (EFSA Citation2009). Both bioavailability and toxicity of arsenic largely depend on the arsenic species; in general, organic arsenic species (e.g., arsenobetaine (AB), arsenocholine) are considered to be of a lower toxicity than inorganic arsenic for living organisms (EFSA Citation2009). Arsenosugars are the major arsenic species in seaweeds (Rose et al. Citation2007; Díaz et al. Citation2012), but also high levels of inorganic arsenic can occur in some seaweed species (Almela et al. Citation2006; Biancarosa et al. Citation2018a). In marine fish, more than 90% of total arsenic is AB (Edmonds and Francesconi Citation2003). To the best of our knowledge, no data is available on arsenic species in insects and products thereof.
The aim of the present study was to document the occurrence of undesirable substances, including heavy metals (cadmium, lead, mercury) and arsenic, as well as fluorine, POPs (dioxins and furans (PCDD/F), DL-PCBs, non-dioxin like polychlorinated biphenyls (NDL-PCBs), polybrominated diphenyl ethers (PBDE), organochlorine pesticides (OCPs)) and mycotoxins in IM produced from BSF larvae fed on a substrate containing 60% seaweed, and diets containing IM. Further, aim was to study the potential transfer of these undesirables from feed to fish fillet, with particular focus on arsenic and arsenic species. We discuss the results in light of the current EU MLs for these substances in feed materials and animal feed (EU Citation2002). By rearing Atlantic salmon up to market size of ~4 kg, we could gain consumer-relevant knowledge on feed and food safety concerning the use of IM in feed for Atlantic salmon.
Materials and methods
Experimental diets and feeding trial
A detailed description of the feeding trial has been published elsewhere (Belghit et al. Citation2018a). In brief, the IM was produced by Protix Biosystems BV (Dongen, The Netherlands) from BSF larvae reared on media containing powdered seaweed (60%) mixed with a plant-based (wheat) medium used as reference material for BSF larvae growth (40%). The seaweed biomass belonged to the brown alga Ascohpyllum nodosum (Ocean Harvest, County Galway, Ireland). At the end of an eight-day growth period, the larvae were separated from the feeding media, washed and partially defatted before being dried and powdered into IM. The nutritional composition of the IM is available elsewhere (Belghit et al. Citation2018a). The formulation of the experimental diets, produced by Cargill (Dirdal, Norway), are shown in . The control diet (IM-0) contained FM and plant-based proteins (20:80, wet weight, ww) as protein sources. In the three experimental diets, FM was replaced with 33% (IM-33), 66% (IM-66) or 100% IM (IM-100), corresponding to IM inclusion levels of 50 g kg−1, 100 g kg−1 and 150 g kg−1, respectively. The lipid sources in all four diets were fish oil and rapeseed oil (33:66, ww). Additional fish oil was included in the experimental diets (IM-33, IM-66 and IM-100) to cover the dietary requirements of long-chained highly unsaturated fatty acids. The relative percentage of the ingredients in each diet was adjusted to make the four diets isonitrogenous (39% crude protein), isolipidic (29% crude lipid) and isoenergetic (25 MJ kg−1 dry matter). All the diets were supplemented with 1% yttrium oxide as inert digestibility marker.
Table 1. Formulation of the four experimental diets. IM-0 = control diet without insect meal (IM) inclusion; IM-33, IM-66 and IM-100 = 33%, 66% and 100% replacement level of FM with IM, respectively.
The feeding trial was conducted at Gildeskål Research Station (GIFAS) in Langholmen, Inndyr, Norway, for 114 days between August and December 2017, following national guidelines for care and use of animals based on the EU Directive 2010/63/EU. Post-smolt Atlantic salmon (~1500 g) were randomly distributed among 12 sea-cages (n = 3 per dietary treatment, 90 fish per cage) and acclimated to the environment for two weeks prior to start the feeding trial. The fish was fed daily by hand, until visual satiation.
Sampling
At the start of the trial, samples of IM and feed were taken, and stored at −30°C until further use. Prior to analysis, the feed samples were grounded into a powder using a blender (Knife Mill Grindomix GM 100, Retsch, Germany). At the end of the trial, a standardized muscle sample, the Norwegian Quality Cut (NQC) as described by Johnsen and colleagues (Johnsen et al. Citation2011) was taken from six fish per tank, pooled and homogenized at sampling, then transported on ice to the laboratory and stored at −30° C until further use. In this paper, the term “fillet” refers to the NQC, unless otherwise stated. Prior to analysis, the fillet samples were freeze-dried for 72 h (FreeZone 18 Liter Console, Labconco, USA).
Chemical analyses
Heavy metals, arsenic and fluorine
Analyses of cadmium, lead, mercury and total arsenic in IM, feed and fish fillet, were performed by inductively coupled plasma mass spectrometry (ICPMS) after microwave-assisted digestion, as described earlier (Julshamn et al. Citation2013; Biancarosa et al. Citation2018b). Briefly, samples (approximately 0.2 g) were digested in 69% nitric acid (2 mL; Sigma-Aldrich, St. Louis, USA) and 30% hydrogen peroxide (0.5 mL; Merck Millipore, Billerica, USA) using a microwave digestion system (UltraWAVE, Milestone, Sorisole, Italy). The solutions were diluted to 25 ml with Milli-Q water (Merck Millipore). Cadmium, lead, mercury and total arsenic concentrations in the samples were quantified by ICPMS (iCapQ ICP-MS, Thermo Scientific, Waltham, USA) equipped with an autosampler (FAST SC-4Q DX, Elemental Scientific, Omaha, USA). Data were collected and processed using the Qtegra ICPMS software (Thermo Scientific). Two certified reference materials were used to assess the accuracy of the method: lobster hepatopancreas (TORT-3; National Research Council Canada, Ottawa, Ontario, Canada) and oyster tissue (SMR 1566b; National Institute of Standards and Technology, Gaithersburg, USA). The limit of quantification (LOQ) of the method was 0.005 mg kg −1 (dw) for cadmium and mercury, 0.03 mg kg −1 (dw) for lead and 0.01 mg kg −1 (dw) for total arsenic.
Fluorine in IM and feed samples were determined using alkali fusion and a fluoride ion-selective electrode (Kjellevold Malde et al. Citation2001). Briefly, the sample (approximately 0.25–0.5 g) was first digested in 8M NaOH, then dried on a hot plate at 110°C for two days, and finally ashed at 525 ± 5°C in a muffle furnace for 3 h. The sample was then diluted in water and neutralized with concentrated HCl until pH in the solution reached 7.5. Aliquots (5 ml) of the sample solution were analysed using a fluoride ion-selective electrode. For quality control, a standard reference material of fluoride in vegetation (material 2695, National Institute of Standards and Technology, NIST, Gaithersburg, MD, USA) and an in-house reference sample of krill meal were used.
Inorganic and organic arsenic species
Inorganic arsenic was determined using an anion-exchange HPLC-ICPMS (1260 HPLC, 7900ICP-MS, Agilent Technologies, Wilmington, DE, USA), as described earlier (Sloth et al. Citation2005a; Julshamn et al. Citation2012), based on a CEN method (European Committee for standardization, NS-EN 16802:2016). In brief, approximately 0.2 g of sample was weighed into a 13 ml centrifuge tube (Sarstedt, Nümbrecht, Germany), and a solution of 0.1 mol L−1 nitric acid (Merck Millipore) and 3% of hydrogen peroxide (Merck Millipore) was added. The samples were subjected to a water bath (90°C for 60 min), cooled to room temperature and centrifuged (2.3 × 103 g for 10 min; Eppendorf® Centrifuge 5702, Hamburg, Germany). Prior to analysis, the samples were filtered through a 0.45 µm disposable syringe filter (Sartorius, Gӧttingen, Germany). The sample was separated on a IonPac AS7 column (2 × 25 mm; Dionex, Sunnyvale, USA) using a mobile phase of 50 mM ammonium carbonate (J.T. Baker, Philipsburg, NJ, USA) in an aqueous 3% (v/v) methanol solution (Merck), with pH 10.3 (adjusted with 25% (v/v) aqueous ammonia (Merck)). During the extraction procedure As (III) was oxidized to As (V), and inorganic arsenic (sum of arsenite (As (III)) and arsenate (As (V))) was determined as As (V), using an external calibration curve of arsenic (As (V), Spectrascan, Oppegaard, Norway). The LOQ of the method was 0.01 mg kg −1 dw. A certified reference material of rice (ERM-BC211; Institute for Reference Materials and Measurements, IRMM, Geel, Belgium) was used to assess the accuracy of the method. The obtained value of 128.3 ± 0.6 µg/kg (mean ± standard deviation, n = 2) was within the 2xSD of the certified value of 124 ± 11 µg/kg ww.
The water-soluble organic arsenic species AB and dimethylarsinic acid (DMA) were determined using a cation-exchange HPLC-ICPMS method, as described earlier (Sloth et al. Citation2003; Sele et al. Citation2015). In brief, approximately 0.5 g of IM and diets, and 0.25 g of fillet samples, was weighed in three replicates into a 50 ml centrifuge tube (polypropylene, VWR, West Chester, PE, USA). Then, a solution of methanol and Milli-Q water (1:1) was added, and the sample was shaken overnight on a shaking board. The samples were centrifuged (3800 × 103 g, 20 min), and 10 ml of the soluble sample extract was transferred to a new 50 ml centrifuge tube. The sample was evaporated to dryness in a laboratory drying oven (Termaks, Bergen, Norway) set at 50°C for 1–2 days, reconstituted in 2 mL of Milli-Q water and filtered through a 0.45 µm single-use syringe filter (Sartorius) prior to analysis. The samples were analysed by a gradient elution program using a Chrompack IonSpher-5C column (3 mm × 100 mm, 5 mm; Agilent Technologies, Wilmington, DE, USA). Mobile phases of 0 mM pyridine (A) and 50 mM pyridine (B) in aqueous 3% (v/v) methanol, pH 2.7 (adjusted with formic acid), were used. The instrumental settings are described elsewhere (Sloth et al. Citation2003; Sele et al. Citation2015). Standard solutions of AB using BCR 626 (certified reference material, IRMM) and DMA (Supelco Analytical, Bellefonte, PA, USA) were prepared, and AB and DMA were quantified using an external calibration curve. The certified reference material tuna fish tissue (BCR-627, IRMM) was used to assess the accuracy of the method. The obtained results for AB (average ± standard deviation, SD) 3.2 ± 0.2 mg As/kg, n = 3, and DMA (mean ± SD) 0.14 ± 0.01 mg As/kg, n = 3, were within the 2xSD of the certified reference values of 3.9 ± 0.2 mg As/kg and 0.15 ± 0.02 mg As/kg, respectively. The ICP-MS is an element-specific detector, and a limitation in this instrumentation is the lack of information regarding chemical structures for the compounds detected. Selected samples (n = 3) were therefore spiked with a standard mixture of methylarsenate (MA), DMA, AB, and tetramethylarsonium ion (TMAO) to assess the identities (chemical structure) of the arsenic peaks detected by ICP-MS. In this way, the arsenic species were identified by correlation to retention times of a standard.
POPs
Samples were analysed for polychlorinated dibenzo-p-dioxins (PCDD) which include: 2,3,7,8-TCDD, 1,2,3,7,8-PeCDD, 1,2,3,4,7,8-HxCDD, 1,2,3,6,7,8-HxCDD, 1,2,3,7,8,9-HxCDD, 1,2,3,4,6,7,8-HpCDD and OCDD; polychlorinated dibenzofurans (PCDF) which include: 2,3,7,8-TCDF, 1,2,3,7,8-PeCDF, 2,3,4,7,8-PeCDF, 1,2,3,4,7,8-HxCDF, 1,2,3,6,7,8-HxCDF, 1,2,3,7,8,9-HxCDF, 2,3,4,6,7,8-HxCDF, 1,2,3,4,6,7,8-HpCDF, 1,2,3,4,7,8,9-HpCDF and OCDF. In this paper, the term “dioxins” will refer to all dioxins and furans mentioned above, unless otherwise stated. The DL-PCBs analysed were the non-ortho polychlorinated biphenyls (noPCB) CB 77, 81, 126 and 169, and the mono-ortho polychlorinated biphenyls (moPCB) CB 105, 114, 118, 123, 156, 157, 167 and 189. For dioxins and DL-PCBs, the mass fraction of each congener was converted to toxicity equivalents (TEQ), ng TEQ kg−1 PCDD ww (van den Berg et al. Citation2006). When the sum of dioxins and DL-PCBs was calculated, mass fractions that were lower than the LOQ were set equal to the LOQ (upper bound LOQ). Feed samples were also analysed for PCB6 which represents six congeners of NDL-PCBs: CB 28, 52, 101, 138, 153 and 180; and PBDEs which include: BDE 28, 47, 99, 100, 153, 154 and 183. The methods for analysis of dioxins, DL-PCBs, NDL-PCBs and PBDEs have been previously described (Lundebye et al. Citation2017). In short, internal standards were added to homogenized sample material, then analytes were extracted with hexane by an accelerated solvent extractor. The sample extracts were purified using a three sequenced solid phase extraction column (silica-, basic alumina- and carbon column) (Power Prep system, FMS, Waltham, MA, USA). Dioxins and DL-PCB analysis was performed by high-resolution gas chromatography/high-resolution mass spectrometry (HRGC-HRMS). MoPCBs and PCB6 were determined by gas chromatography/tandem mass spectrometry (GC-MS/MS). PBDEs were analysed by negative chemical ionization gas chromatography/mass spectrometry (GC/MS).
Samples of feed were analysed for organochlorine pesticides (OCPs) at Eurofins GfA Lab Service (Hamburg, Germany) using an accredited method (accreditation number D-PL-14629-01-00 according to DIN EN ISO/IEC 17025:2005). The compounds analysed were: aldrin; chlordane (cis-, oxy-, trans-) dieldrin; endosulfan (alpha-, beta-, sulfate); endrin; hexachlorocyclohexane (HCH) (alpha-, beta-, gamma-, delta-); heptachlor; heptachlor epoxide (cis-, trans-); hexachlorobenzene (HCB); mirex; trans-nonachlor; o, p’-DDD; o, p’-DDE; o, p’-DDT (dichlorodiphenyltrichloroethane); octachlorstyrene; p, p’-DDD; p, p’-DDE; p, p’-DDT; pentachlorobenzene; toxaphene (parlar 26, 50, 62).
Mycotoxins
Samples of feed were analysed for the presence of mycotoxins at Eurofins GfA Lab Service (Hamburg) using an accredited method (accreditation number D-PL-14602-01-00). The following compounds were analysed: aflatoxin (AFB1, AFB2, AFG1 and AFG2), beauvericin, deoxynivalenol (DON), enniatin (ENNA, ENNA1, ENNB and ENNB1), HT-2 toxin (HT2), ochratoxin A (OTA), T-2 toxin, fumonisin B1 and B2 (FB1, FB2) and zearalenone (ZEN). HPLC coupled with fluorescence detection was used for determination of aflatoxins and OTA, while the other compounds were quantified by liquid chromatography-mass spectrometry (LC-MS).
Statistical analysis
All statistical analyses were performed using the free software environment R (R Development Core Team Citation2011). All data were statistically evaluated by a regression design using a linear model (lm) and one-way ANOVA (Tukey test) to find differences due to dietary treatments. All data were analysed for homogeneity of variance using a Levene’s test and for normality using a Shapiro Wilk’s test, as well as being evaluated graphically by QQ-plots before utilizing parametric tests. Differences were regarded as significant when P≤ 0.05. All data are presented as mean ± SD, if not otherwise stated.
Calculations
The digestibility of total arsenic was calculated as apparent digestibility coefficient (ADC), according to this formula:
where Y is yttrium concentration and As is total arsenic concentration.
Results
The proximate composition of the diets is described in detail elsewhere (Belghit et al. Citation2018b). Overall, all diets were similar in dry matter, protein, fat, energy and carbohydrates levels and identical concentrations of essential amino acids were found. Increasing dietary IM inclusion led to some changes in the fatty acid composition of the diets, such as an increase in the concentrations of lauric- and eicosapentaenoic-docosahexaenoic acids (Belghit et al. Citation2018a).
The replacement of FM with IM did not affect dietary concentrations of the undesirable substances analysed. Similar concentrations of heavy metals, POPs, pesticides and mycotoxins were found in the four diets, and the concentrations were all below current EU MLs for these substances in complete feed (EU Citation2002). Arsenic speciation analysis of the diets showed decreasing concentrations of AB with increasing inclusion of IM. Feed to fillet transfer of arsenic was observed, although total arsenic concentrations in the fillet significantly decreased when fish were fed diets with higher inclusion of IM.
Heavy metals and fluorine
The concentrations of the heavy metals cadmium, lead and mercury, and of fluorine in the IM were all below the EU MLs for these contaminants in feed materials (). By replacing FM with IM in the diet, cadmium concentrations increased, although low concentrations were observed in all the diets (0.13 and 0.30 mg kg−1 in IM-0 and IM-100, respectively) (). Lead was not quantified (<LOQ) in the control diet (IM-0), and its concentration slightly increased with higher dietary IM inclusion (0.08 mg kg−1 in IM-100) (). Mercury concentrations were low in all the diets (0.02 mg kg−1 in IM-0) and were not quantified (<LOQ) in the diet with the highest IM inclusion (IM-100) (). Dietary concentrations of fluorine decreased with increasing inclusion of IM in the diets (38 and 24 mg kg−1 in IM-0 and IM-100, respectively) ().
Table 2. Concentrations (mg kg−1) of cadmium (Cd), mercury (Hg), lead (Pb) and fluorine (F) converted to moisture content of 12%, in the experimental diets. EU maximum levels in complete feed for fish relative to a feed with moisture content of 12 %, according to Directive 2002/32/EC (and amendments) on undesirable substances in animal feed. Actual concentrations in the samples in parentheses (mg kg−1 wet weight basis). IM-0 = control diet without insect meal (IM) inclusion; IM-33, IM-66 and IM-100 = 33%, 66% and 100% replacement level of FM with IM, respectively.
Cadmium and lead were not quantified (<LOQ) in any of the fillet samples (data not shown). The concentrations of mercury decreased in the fillet of Atlantic salmon fed diets with increasing IM inclusion, although concentrations were very low (0.02 ± 0.001 and 0.009 ± 0.001 mg kg−1 ww in IM-0 and IM-100, respectively) (data not shown).
Arsenic
The concentration of total arsenic in the IM was 4.7 mg kg−1 (), thus above the EU ML of 2 mg kg−1 set for feed materials (EU Citation2002). Total arsenic concentrations in the experimental diets were comparable (2.1 and 1.8 mg kg−1 in IM-0 and IM-100, respectively) despite higher inclusion of IM, and all concentrations were below the ML of 10 mg kg−1 for fish feed (). The digestibility of total arsenic was highest in the control diet, while it decreased in the IM-rich diets (ADC was 77% in IM-0, 68 in IM-33, 53% in IM-66 and 43% in IM-100) (data not shown). The concentrations of total arsenic in the fillet samples decreased significantly with increasing inclusion of IM in the diets (1.1 ± 0.06 and 0.3 ± 0.01 mg kg−1 ww in IM-0 and IM-100, respectively) (P< 001) (, ).
Table 3. Concentrations (mg kg−1) of total arsenic (totAs), inorganic arsenic (iAs), arsenobetaine (AB), dimethylarsenate (DMA) and unidentified arsenic species (UAs) in the insect meal (IM), the experimental diets and the fish fillet. Concentrations in the experimental diets are converted to moisture content of 12%, while actual concentrations in the samples in parentheses (mg kg−1 wet weight basis). IM-0 = control diet; IM-33, IM-66 and IM-100 have 33%, 66% and 100% replacement level of FM with IM, respectively.
Figure 1. Concentrations (mg kg−1 ww) of total arsenic in fillet (NQC) of Atlantic salmon fed diets with increasing inclusion of insect meal. IM-0 = control diet without insect meal (IM) inclusion; IM-33, IM-66 and IM-100 = 100% replacement level of FM with IM, respectively. Values are means, with their standard deviations represented by vertical bars. Different letters indicate significant differences (P< .05; linear regression) (R2 =Adjusted R-squared).
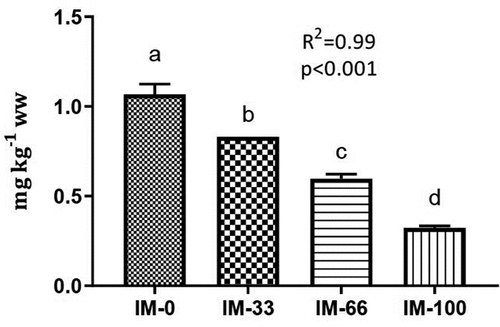
By speciation analysis of arsenic in the IM, inorganic arsenic (0.28 mg kg−1), AB (0.06 mg kg−1) and DMA (0.88 mg kg−1) were found to account for 6%, 1.2% and 19% of total arsenic in IM, respectively (). The majority of the arsenic species found in IM was unidentified species, eluting in the void of the chromatogram (<2 min) or later (retention time 8 to 12 min) in the chromatogram (). Spiking experiments showed that MA elute in the void of the chromatogram, suggesting that MA could be present in the IM. However, other neutral and anionic compounds will also co-elute in the void. The samples were therefore not quantitatively determined for MA. Arsenic peaks eluting later in the chromatogram did not correlate with TMAO, and standard of other arsenic species are unfortunately not commercially available for spiking experiments.
Figure 2. Chromatogram of the insect meal (IM) analysed by cation-exchange HPLC-ICPMS. The major arsenic peaks are dimethylarsinate (DMA) and unidentified arsenic species, eluted in the void and later in the chromatogram (retention time (r.t.) 8–12 min). Arsenobeatine (AB) is the minor arsenic peak with r.t. 6 min.
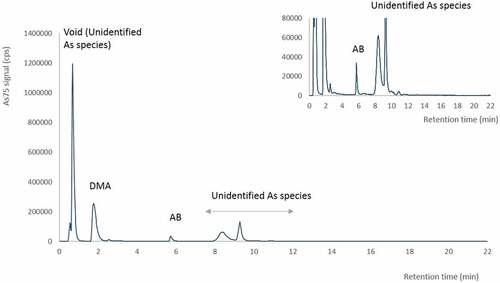
In the diets, higher inclusion of IM corresponded to decreased concentrations of AB and increased concentrations of inorganic arsenic, DMA and unidentified arsenic species (; ). In the salmon fillet, inorganic arsenic was not detected in any of the samples (<LOQ), while AB was the major arsenic species for all dietary groups. AB accounted for 86% and 61% of total arsenic in the fillet from fish fed the control diet (IM-0) and the highest IM diet (IM-100), respectively ().
Figure 3. Chromatograms of the organic arsenic species in the diets (overlay of diet IM-0, IM-33, IM-66 and IM-100) analysed by cation-exchange HPLC-ICPMS. The major arsenic peaks are in the void of the chromatogram (< r.t. 1 min) being unidentified arsenic species, dimethylarsinate (DMA) and arsenobetaine (AB). Minor unidentified arsenic species are detected later in the chromatogram (r.t. 8–15 min). The enlargement shows the full signal scale for AB.
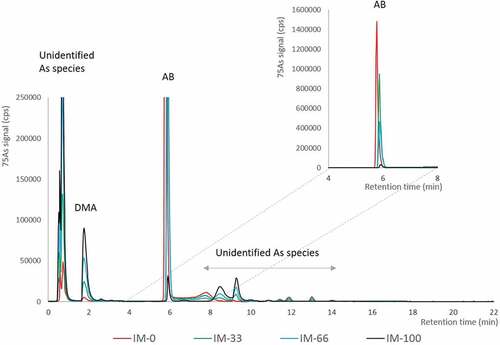
POPs
The concentrations of the sum of PCDD and PCDF, referred to as “dioxins”, DL-PCBs (the sum of four non-ortho and eight mono-ortho PCB congeners) and the sum of dioxins and DL-PCBs, were low in all the experimental diets and below the EU MLs (). Similar concentrations of these compounds were found in all the four diets. The concentrations of NDL-PCBs were low in all the diets and well below the EU ML set for complete feed for fish (EU Citation2002). Polybrominated diphenyl ethers were found in the diets (); however, no EU MLs for these compounds are set.
Table 4. Concentrations of POPs converted to moisture content of 12% in the experimental diets: the sum of polychlorinated dibenzodioxins (PCDD), polychlorinated dibenzofurans (PCDF), dioxin-like polychlorinated biphenyls (DL-PCBs) (ng WHO05 TEQ kg−1 wet weight), non-dioxin like polychlorinated biphenyls (NDL-PCBs) and polybrominated diphenyl ethers (PBDEs) (µg kg−1 wet weight), organochlorine pesticides (ng g−1 wet weight). EU maximum levels (ML) in compound feed for fish relative to a feed with moisture content of 12 %, according to Directive 2002/32/EC (and amendments) on undesirable substances in animal feed. Actual concentrations in the samples in parentheses. IM-0 = control diet without insect meal (IM) inclusion; IM-33, IM-66 and IM-100 = 100% replacement level of FM with IM, respectively.
The concentrations of OCPs analysed in the feed samples were all below the LOQ, except for HCB and DDT which were found in all the diets (). The concentrations of HCB were however very low in all the four diets (maximum value was 1.09 ng g−1 in IM-100) and well below the EU ML of 10 ng g−1 in compound feed (EU Citation2002). The concentration of sum of DDT (upper-bound concentrations of DDT-, DDD- and DDE-isomers) was low in all the experimental diets (highest value was 5 ng g−1 in IM-66) and below the EU ML (EU Citation2002) ().
Mycotoxins
Of the mycotoxins analysed in the feed samples (), the concentrations of AFB1, OTA, ENNA, ENNA1 and ENNB1 were below the LOQs. The concentrations of DON, FB1, FB2 and ZEN were similar in the four diets and well below the recommended EU guidance levels set for these compounds in animal feed (Commission Recommendation 2006/576/EC and amendments) (EU Citation2006). By replacing FM with IM in the diet, the concentrations of beauvericin slightly increased, whereas the concentration of ENNB slightly decreased (). No guidance values or MLs for beauvericin and ENNB exist.
Table 5. Concentration (µg kg−1) mycotoxins converted to moisture content of 12%, in the experimental diets. EU maximum levels in complete feed for farmed animals relative to a feed with moisture content of 12 %, according to Directive 2002/32/EC (and amendments) on undesirable substances in animal feed. Actual concentrations in the samples in parentheses (µg kg−1 wet weight basis). IM-0 = control diet without insect meal (IM) inclusion; IM-33, IM-66 and IM-100 = 100% replacement level of FM with IM, respectively.
Discussion
Heavy metals and fluorine
In the present study, the heavy metals cadmium, lead and mercury were detected in the IM of BSF larvae. This was expected as the IM was obtained from larvae fed media containing 60% seaweed (the brown alga, A. nodosum). Seaweeds are known to accumulate heavy metals from the marine environment, especially brown algae species (Almela et al. Citation2006; Yamada et al. Citation2007; Hwang et al. Citation2010; Duinker et al. Citation2016; Biancarosa et al. Citation2018a), as applied in this study. It has previously been shown that BSF larvae fed seaweed-enriched media accumulate cadmium, lead and mercury from the feeding media (Biancarosa et al. Citation2018b). Specifically, a higher inclusion of seaweed in the feeding media causes higher concentrations of heavy metals in the larvae. In the current study, concentrations of heavy metals in the IM were overall low, with concentrations of 0.98, 0.36, 0.016 mg kg−1 for cadmium, lead and mercury, respectively. The low levels are likely linked to the low concentrations of the metals in the feeding media for the larvae. The concentrations of heavy metals in the brown alga A. nodosum were ~0.2, ~0.1 and ~0.01 mg kg−1 dw for cadmium, lead and mercury, respectively (data not shown). These levels are among the lowest concentrations found in brown algae species (Almela et al. Citation2006; Yamada et al. Citation2007; Hwang et al. Citation2010; Duinker et al. Citation2016; Biancarosa et al. Citation2018a). Previous studies have also shown that accumulation of heavy metals in BSF strongly depends on the concentrations of the metals in the feeding media (Diener et al. Citation2015; van der Fels-Klerx et al. Citation2016; Gao et al. Citation2017; Scheibelberger et al. Citation2017). Comparing the heavy metals concentrations in IM in the current study and in BSF fed seaweed-enriched media from our previous study (Biancarosa et al. Citation2018b), we found similar values. This suggests that processing whole BSF larvae into IM may not affect metal concentrations. In other words, the heavy metals seemed to be bound to the protein fraction of the larvae, as fat was largely removed while processing larvae into IM. The concentrations of cadmium, lead and mercury in the IM did not exceed any of the current EU MLs for these metals in feed materials (EU Citation2002).
The concentrations of heavy metals in the diets were comparable to concentrations found in commercial fish feeds for Atlantic salmon in the last few years, which are found to be from 0.08 to 1.1, 0.02 to 0.05, 0.005 to 0.042 mg kg−1 for cadmium, lead and mercury, respectively (Berntssen et al. Citation2010; Sele et al. Citation2018). Replacing dietary FM with IM led to a three-fold increase of cadmium concentrations in the diet with the highest IM inclusion (IM-100) compared to the control diet (IM-0). Cadmium is considered a heavy metal of major concern in farmed insects (Charlton et al. Citation2015; EFSA Citation2015), as it can easily accumulate in insect larvae (Diener et al. Citation2015; van der Fels-Klerx et al. Citation2016; Gao et al. Citation2017; Scheibelberger et al. Citation2017; Biancarosa et al. Citation2018b). Similarly, the concentrations of lead slightly increased in the diets with higher IM inclusion. A previous study testing insect-based diets for freshwater Atlantic salmon, also showed higher cadmium and lead concentrations when more IM was included in the diet (Belghit et al. Citation2018b). Lead is often present in BSF larvae, although concentrations are generally low (up to 4 mg kg−1), unless the feeding media are spiked with high concentrations of lead (Charlton et al. Citation2015; Diener et al. Citation2015; Biancarosa et al. Citation2018b). The concentrations of mercury were low in the diets, with 0.03 mg kg−1 in the control diet (IM-0) and under the LOQ in the diet where FM was fully replaced by IM (IM-100). This was expected as marine ingredients (e.g., FM) are the main sources of mercury in fish feeds (Berntssen et al. Citation2010). Overall, the concentrations of the heavy metals cadmium, lead and mercury in the diets did not exceed any of the current EU MLs set for these contaminants in fish feed (EU Citation2002). Moreover, cadmium and lead were not detected in any of the fish fillet samples, as they have a limited capacity to accumulate in fish muscle (Handy Citation1992; Atobatele and Olutona Citation2015). Similarly, mercury was found in the fillet samples at low concentrations: 0.02 ± 0.001 and 0.009 ± 001 in IM-0 and IM-100, respectively (data not shown).
IM and the diets were also analysed for the content of fluorine. Excessive exposure to this element is associated with dental and skeleton abnormalities in various animal species, including fish (EFSA Citation2004). In the EU, MLs for fluorine in feed are set to 150 and 350 mg kg−1 for feed materials and complete feed for fish, respectively (Directive 2002/32/EC and amendments) (EU Citation2002). The concentrations of fluorine in IM and the diets were well below the current EU MLs for this element in feed materials and complete feed, thus not posing a feed safety issue.
Arsenic
Arsenic was detected with relatively high concentrations, 4.7 mg kg−1, in the IM. This was expected as arsenic is known to easily accumulate in seaweeds (Rose et al. Citation2007) and can be transferred to BSF larvae fed seaweed-enriched media (Biancarosa et al. Citation2018b). Concentrations of total arsenic in the IM was above the current EU ML of 2 mg kg−1 set for feed materials (EU Citation2002). These results show that using IM from BSF larvae fed on seaweed-enriched media as feed ingredient may be limited due to elevated arsenic levels in the IM. The EU feed legislation on undesirable substances in animal feed is based on total concentrations of arsenic. However, authorities can request documentation showing that the concentration of inorganic arsenic in feed ingredients is below 2 mg kg−1 (EU Citation2002). The concentration of inorganic arsenic in the IM (0.28 mg kg −1) accounted for less than 6% of total arsenic concentrations and below the EU ML. Previous studies have shown that inorganic arsenic only contributed a minor fraction (less than 1%) of total arsenic in BSF larvae fed on seaweed-enriched media (Biancarosa et al. Citation2018b). These findings suggest that BSF larvae do not convert the organic arsenic forms present in the seaweed into inorganic arsenic.
The main sources of arsenic in fish feed are marine ingredients, mainly FM and fish oil (Sloth et al. Citation2005a; Berntssen et al. Citation2010). Interestingly, the replacement of FM with IM in this study did not impact the concentrations of total arsenic in the four diets. Based on the concentrations of total arsenic in the IM and its inclusion levels in the diets, IM contributed only to the 12% of total arsenic concentrations in the IM-33 diet, while to 26% and 44% of total arsenic in IM-66 and IM-100, respectively. This could partially explain the similar levels of total arsenic in the four diets. In a previous study, the increased inclusion of IM and decreased inclusion of FM in the diets for freshwater Atlantic salmon caused lower total arsenic concentrations in the diets (Belghit et al. Citation2018b). This difference could be due to using additional fish oil in the diets containing IM in the current study. High concentrations of arsenic have previously been found in fish oils, thus contributing considerably to the arsenic concentrations in commercial fish feeds (Sloth et al. Citation2005a; Sissener et al. Citation2013; Sele et al. Citation2014). Overall, the concentrations of total arsenic in the diets were all below the EU ML of 10 mg kg−1 in complete feed for fish (Directive 2002/32/EC and amendments) (EU Citation2002). The concentrations of inorganic arsenic in the diets were also below the EU ML of 2 mg kg−1 feed.
Despite similar total arsenic concentrations in the four diets, the fish fillet showed a significant decrease of total arsenic concentrations with increasing inclusion of IM in the diets, compared to the control diet (IM-0). This could be due to the presence of arsenic species in the IM which are not bioavailable or accumulated in the fish muscle. In FM, AB is considered the dominant arsenic species (Sloth et al. Citation2003). In fish oil, the lipid-soluble arsenic species, the arsenolipids, are the major forms (Francesconi and Kuehnelt Citation2004; Sele et al. Citation2014, Citation2015). Speciation analysis of organic arsenic (water-soluble) species in the IM showed that AB accounted for only 1.2% of the total arsenic. DMA was the major arsenic species detected in the IM, accounting for 18% of the total arsenic. A major fraction of arsenic in the IM was still-unidentified arsenic species, eluting in the void of the chromatogram or later in the chromatogram (r.t. from 8 to 10 min; ). There are limitations to the ICP-MS as it cannot provide structural information on the arsenic peaks detected, and hence, commercial standards are a prerequisite for the identification of compounds when analysing with HPLC-ICPMS. Due to the lack of commercial standards, one can only speculate regarding the chemical forms of the unidentified arsenic compounds. The unidentified arsenic species detected in the IM could be metabolic products produced by the BSF larvae fed on the seaweed substrate, e.g., metabolites of arsenosugars. Possible metabolic products of arsenosugars could be DMA, dimethylarsinoylacetate, dimethylarsinoylethanol and their thio-analogues, as shown by Feldmann and Krupp (Citation2011). Also, arsenocholine has been proposed as intermediate of the reduction/methylation of arsenosugars to the end-product AB (Edmonds and Francesconi Citation1987). The unidentified arsenic species in the void (>2 min) of the IM chromatogram could be MA, but also other neutral or anionic compounds, e.g., inorganic arsenic and arsenosugars, could account for this peak. A. nodosum was not analyzed for arsenic species in this study. However, seaweeds are considered to take up arsenate in place of the phosphate, and then rapidly transform it to form a number of arsenic species (Ronan et al. Citation2017). The most common species in algae are the arsenosugars (Francesconi and Edmonds Citation1998; Edmonds et al. Citation1997; Feldmann and Krupp Citation2011), but also inorganic arsenic, arsenolipids and DMA can be present (Francesconi and Edmonds Citation1998; Rose et al. Citation2007). The metabolism of arsenic by the BSF larvae is currently not known and it would presumably also be linked to the arsenic species present in the seaweeds in the feeding media. There is also a fraction (13%) of the total arsenic in the IM that was not extracted, which could be arsenolipids or arsenic forms related to the chitins (exoskeleton) in insects. To our knowledge, this is the first study of arsenic species in a product of BSF larvae fed on seaweed-enriched media; therefore, further studies on this topic are suggested.
The digestibility of total arsenic is decreased with higher inclusion of IM in the diet. Speciation analysis of the diets showed that the concentration of AB decreased with increasing inclusion of IM (). At the same time, inorganic As, DMA, and unidentified arsenic species increased in the diets containing more IM. In the fish fillets, AB was the major arsenic species for all dietary groups. However, AB accounted for a higher percentage of total arsenic in the control group compared to the insect-based diets. These results suggest that although total arsenic levels in the diets are approximately similar, the type of arsenic species present in the diets affect the accumulation of arsenic in the fish.
POPs
Dietary concentrations of POPs (i.e., dioxins (PCDD/F), DL-PCBs, NDL-PCBs and PBDEs) were all below current EU MLs, and they were comparable to concentrations found in commercial fish feeds for Atlantic salmon in the last few years (Berntssen et al. Citation2010; Sele et al. Citation2018). POPs are lipid soluble and mainly associated with marine ingredients (mainly fish oil), thus their presence in fish feeds has decreased, following the increased use of plant-based feed ingredients (i.e., replacing fish oils with vegetable oils) (Berntssen et al. Citation2010). A low inclusion level of fish oil (10–15%) was used in the diets in the current study. Similar concentrations of POPs were found between experimental diets; therefore, the inclusion of IM in the diets seemed not to affect the concentration of these contaminants in the diets. Low concentrations of POPs have been found in different-farmed insect species (Charlton et al. Citation2015; EFSA Citation2015; Poma et al. Citation2017). However, no data are available on the presence of POPs in IM.
Of the analysed pesticides, only HCB and DDT were detected in the diets. In a recent study, published by Charlton et al. (Citation2015), no pesticide residues were detected in BSF larvae. The use of HCB in agriculture was banned in Europe in the 1980s, but it is still released to the environment as by-product through industrial processes. The average concentration of 1 ng g−1 HCB found in the diets is comparable to the average concentration (0.8 ng g−1) found in 40 commercial fish feed in 2017 (Sele et al. Citation2018). DDT is a very low-biodegradable pesticide and has been considered an environmental problem since the late 1960s. The use of DDT is banned in many countries, but in some countries, it is still used in the fight against malaria. The concentrations of DDT in the diets (upper bound concentrations) were lower than average concentrations found in commercial fish feeds for salmonids (Berntssen et al. Citation2010; Sele et al. Citation2018). The concentrations of pesticides found in the diets were overall below current EU MLs set for fish feed and were not affected by the inclusion of IM in the diets.
Mycotoxins
The mycotoxins DON, FB1, FB2, ZEN, beauvericin and ENNB were found in the diets. The concentrations of mycotoxins were comparable to the ones of commercial fish feeds for Atlantic salmon (Sele et al. Citation2018). Mycotoxins are produced by fungi and can naturally be found in a wide variety of crops, making plant-based feed materials the main sources of these substances in animal feed (Matejova et al. Citation2017). Replacing dietary FM with IM did not affect the concentrations of mycotoxins in the diets, as the concentrations were similar among the four experimental diets. Low concentrations of mycotoxins have earlier been found in farmed insect species (Charlton et al. Citation2015; EFSA Citation2015). Also, insect larvae can easily metabolize and excrete mycotoxins from contaminated feeding media (Scheibelberger et al. Citation2017; Van Broekhoven et al. Citation2017; Camenzuli et al. Citation2018). Therefore, similar levels of mycotoxins in all diets were expected, as IM would not be a major dietary source of these compounds. The EU has set an ML of 10 µg kg−1 for aflatoxin B1 in complete feed (Directive 2002/32/EC and amendments) (EU Citation2002), while guidance values are set for DON, FB1+ FB2, ZEN and OTA (Commission Recommendation 2006/576/EC) (EU Citation2006). The concentrations of mycotoxins found in the diets were all below the MLs and the guidance values.
Conclusions
The IM and the diets contained the heavy metals cadmium, lead and mercury, and fluorine; however, levels of these contaminants in the IM, and the diets were all below current MLs for feed materials and complete fish feed. Also, the concentrations of POPs, pesticides and mycotoxins in the diets were below the current MLs set by the EU. While total arsenic concentrations in IM-based diets were all below the current ML for fish feed, the IM contained arsenic at a concentration above the current ML for feed materials. The use of BSF larvae fed seaweed-enriched media for production of IM could, therefore, be a challenge, in relation to arsenic. Transfer of arsenic from feed-to-fillet occurred, although there was a clear reduction of total arsenic concentrations in the fillet of Atlantic salmon fed diets with increasing inclusion of IM. This could be due to the IM in the diets contributing with arsenic species which are not accumulating into the muscle tissue of the fish. Based on our findings, we can conclude that replacing FM with IM in the diet of Atlantic salmon did not impact the amount of contaminants in the feed. IM from BSF larvae fed on seaweed-enriched media is overall a safe source of protein for seawater Atlantic salmon, with respect to the quantity of contaminants observed in this study that were present below the MLs stipulated in the EU legislation.
Acknowledgments
This work was supported by the Norwegian Research Council project AquaFly [grant number 23889] and RAPFINN [grant number 220634]. Special thanks to Snorri Gunnarsson at the Institute of Marine Research (Bergen, Norway) for the technical work on arsenic speciation analyses. No potential conflict of interest was reported by the author(s).
Additional information
Funding
References
- Almela C, Jesús Clemente M, Vélez D, Montoro R. 2006. Total arsenic, inorganic arsenic, lead and cadmium contents in edible seaweed sold in Spain. Food Chem Toxicol. 44(11):1901–1908.
- Atobatele OE, Olutona GO. 2015. Distribution of three non-essential trace metals (Cadmium, Mercury and Lead) in the organs of fish from Aiba reservoir, Iwo, Nigeria. Toxicol Rep. 2:896–903.
- Barroso FG, de Haro C, Sanchez-Muros MJ, Venegas E, Martinez-Sanchez A, Perez-Banon C. 2014. The potential of various insect species for use as food for fish. Aquaculture. 422:193–201.
- Belghit I, Liland NS, Gjesdal P, Biancarosa I, Menchetti E, Li Y, Waagbø R, Krogdahl Å, Lock E-J. 2018a. Black soldier fly larvae meal can replace fish meal in diets of sea-water phase Atlantic salmon (Salmo salar). Aquaculture. 503:609–619.
- Belghit I, Liland NS, Waagbø R, Biancarosa I, Pelusio N, Li Y, Krogdahl Å, Lock E-J. 2018b. Potential of insect-based diets for Atlantic salmon (Salmo salar). Aquaculture. 491:72–81.
- Berntssen MHG, Julshamn K, Lundebye A-K. 2010. Chemical contaminants in aquafeeds and Atlantic salmon (Salmo salar) following the use of traditional- versus alternative feed ingredients. Chemosphere. 78(6):637–646.
- Biancarosa I, Belghit I, Bruckner CG, Liland NS, Waagbø R, Amlund H, Heesch S, Lock E-J. 2018a. Chemical characterization of 21 species of marine macroalgae common in Norwegian waters: benefits of and limitations to their potential use in food and feed. J Sci Food Agric. 98(5):2035–2042.
- Biancarosa I, Liland NS, Biemans D, Araujo P, Bruckner CG, Waagbø R, Torstensen BE, Lock E-J, Amlund H. 2018b. Uptake of heavy metals and arsenic in black soldier fly (Hermetia illucens) larvae grown on seaweed-enriched media. J Sci Food Agric. 98(6):2176–2183.
- Borgogno M, Dinnella C, Iaconisi V, Fusi R, Scarpaleggia C, Schiavone A, Monteleone E, Gasco L, Parisi G. 2017. Inclusion of Hermetia illucens larvae meal on rainbow trout (Oncorhynchus mykiss) feed: effect on sensory profile according to static and dynamic evaluations. J Sci Food Agric. 97(10):3402–3411.
- Camenzuli L, Van Dam R, De Rijk T, Andriessen R, Van Schelt J, Van der Fels-Klerx JH. 2018. Tolerance and excretion of the mycotoxins aflatoxin B1, zearalenone, deoxynivalenol, and ochratoxin A by alphitobius diaperinus and hermetia illucens from contaminated substrates. Toxins. 10(2):91.
- Charlton AJ, Dickinson M, Wakefield ME, Fitches E, Kenis M, Han R, Zhu F, Kone N, Grant M, Devic E, et al. 2015. Exploring the chemical safety of fly larvae as a source of protein for animal feed. J Insects Food Feed. 1(1):7–16.
- Díaz O, Tapia Y, Muñoz O, Montoro R, Velez D, Almela C. 2012. Total and inorganic arsenic concentrations in different species of economically important algae harvested from coastal zones of Chile. Food Chem Toxicol. 50(3–4):744–749.
- Diener S, Zurbrügg C, Tockner K. 2015. Bioaccumulation of heavy metals in the black soldier fly, Hermetia illucens and effects on its life cycle. J Insects Food Feed. 1(4):261–270.
- Duinker A, Roiha IS, Amlund H, Dahl L, Lock E-J, Kögel T, Måge A, Lunestad BT 2016. Potential risks posed by macroalgae for application as feed and food-a Norwegian perspective. Technical report National Institute of Nutrition and Seafood Research (NIFES).
- Edmonds JS, Francesconi KA. 1987. Transformations of arsenic in the marine environment. Experientia. 43(5):553–557.
- Edmonds JS, Francesconi KA. 2003. Organoarsenic compounds in the marine environment. New Jersey (USA): John Wiley & Sons, Ltd; p. 195–222.
- Edmonds JS, Shibata Y, Francesconi KA, Rippingale RJ, Morita M. 1997. Arsenic transformations in short marine food chains studied by HPLC‐ICP MS. Appl Organomet Chem. 11(4):281–287.
- [EFSA] European Food Safety Authority. 2004. Opinion of the scientific panel on contaminants in the food chain [CONTAM] related to fluorine as undesirable substance in animal feed. EFSA J.
- [EFSA] European Food Safety Authority. 2009. Scientific opinion on arsenic in food EFSA panel on contaminants in the food chain (CONTAM). EFSA J. 7(10):1351.
- [EFSA] European Food Safety Authority. 2015. Risk profile related to production and consumption of insects as food and feed. EFSA J. 13(10):4257.
- [EU] European Union. 2002. Directive 2002/32/EC of the European Parliament and the Council of 7 May 2002 on undesirable substances in animal feed.
- [EU] European Union. 2006. Commission Recommendation of 17 August 2006 on the presence of deoxynivalenol, zearalenone, ochratoxin A, T-2 and HT-2 and fumonisins in products intended for animal feeding.
- [EU] European Union. 2017. Commission Regulation (EU) 2017/893 of 24 May 2017 amending Annexes I and IV to Regulation (EC) No 999/2001 of the European Parliament and of the Council and Annexes X, XIV and XV to Commission Regulation (EU) No 142/2011 as regards the provisions on processed animal protein.
- Feldmann J, Krupp EM. 2011. Critical review or scientific opinion paper: arsenosugars—a class of benign arsenic species or justification for developing partly speciated arsenic fractionation in foodstuffs? Anal Bioanal Chem. 399(5):1735–1741.
- Francesconi KA, Edmonds JS. 1998. Arsenic species in marine samples. Croatica Chemica Acta. 71(2):343–359.
- Francesconi KA, Kuehnelt D. 2004. Determination of arsenic species: A critical review of methods and applications, 2000–2003. Analyst. 129(5):373–395.
- Gao Q, Wang X, Wang W, Lei C, Zhu F. 2017. Influences of chromium and cadmium on the development of black soldier fly larvae. Environ Sci Pollut Res. 24(9):8637–8644.
- Handy RD. 1992. The assessment of episodic metal pollution. II. The effects of cadmium and copper enriched diets on tissue contaminant analysis in rainbow trout (Oncorhynchus mykiss). Arch Environ Contam Toxicol. 22(1):82–87.
- Henry M, Gasco L, Piccolo G, Fountoulaki E. 2015. Review on the use of insects in the diet of farmed fish: past and future. Anim Feed Sci Technol. 203:1–22.
- Hwang YO, Park SG, Park GY, Choi SM, Kim MY. 2010. Total arsenic, mercury, lead, and cadmium contents in edible dried seaweed in Korea. Food Addit Contam: Part B. 3(1):7–13.
- Johnsen CA, Hagen Ø, Adler M, Jönsson E, Kling P, Bickerdike R, Solberg C, Björnsson BT, Bendiksen EÅ. 2011. Effects of feed, feeding regime and growth rate on flesh quality, connective tissue and plasma hormones in farmed Atlantic salmon (Salmo salar L.). Aquaculture. 318(3):343–354.
- Julshamn K, Maage A, Norli HS, Grobecker KH, Jorhem L, Fecher P, Dowell D. 2013. Determination of arsenic, cadmium, mercury, and lead in foods by pressure digestion and inductively coupled plasma/mass spectrometry: first action 2013.06. J AOAC Int. 96(5):1101–1102.
- Julshamn K, Nilsen BM, Frantzen S, Valdersnes S, Maage A, Nedreaas K, Sloth JJ. 2012. Total and inorganic arsenic in fish samples from Norwegian waters. Food Addit Contam: Part B. 5(4):229–235.
- Kaushik SJ, Medale F, Fauconneau B, Blanc D. 1989. Effect of digestible carbohydrates on protein/energy utilization and on glucose metabolism in rainbow trout (Salmo gairdneri R.). Aquaculture. 79(1):63–74.
- Kjellevold Malde M, Bjorvatn K, Julshamn K. 2001. Determination of fluoride in food by the use of alkali fusion and fluoride ion-selective electrode. Food Chem. 73(3):373–379.
- Kouřimská L, Adámková A. 2016. Nutritional and sensory quality of edible insects. Nfs J. 4:22–26.
- Liland NS, Biancarosa I, Araujo P, Biemans D, Bruckner CG, Waagbø R, Torstensen BE, Lock E-J. 2017. Modulation of nutrient composition of black soldier fly (Hermetia illucens) larvae by feeding seaweed-enriched media. PLoS One. 12(8):e0183188.
- Lock E-J, Biancarosa I, Gasco L. 2018. Insects as raw materials in compound feed for aquaculture. In: Halloran A, Flore R, Vantomme P, Roos N, editors. Edible insects in sustainable food systems. Cham (UK): Springer International Publishing; p. 263–276.
- Lock E-J, Arsiwalla T, Waagbø R. 2016. Insect larvae meal as an alternative source of nutrients in the diet of Atlantic salmon (Salmo salar) postsmolt. Aquac Nutr. 22(6):1202–1213.
- Lundebye A-K, Lock E-J, Rasinger JD, Nøstbakken OJ, Hannisdal R, Karlsbakk E, Wennevik V, Madhun AS, Madsen L, Graff IE, et al. 2017. Lower levels of Persistent Organic Pollutants, metals and the marine omega 3-fatty acid DHA in farmed compared to wild Atlantic salmon (Salmo salar). Environ Res. 155:49–59.
- Maehre HK, Malde MK, Eilertsen KE, Elvevoll EO. 2014. Characterization of protein, lipid and mineral contents in common Norwegian seaweeds and evaluation of their potential as food and feed. J Sci Food Agric. 94(15):3281–3290.
- Magalhães R, Sánchez-López A, Leal RS, Martínez-Llorens S, Oliva-Teles A, Peres H. 2017. Black soldier fly (Hermetia illucens) pre-pupae meal as a fish meal replacement in diets for European seabass (Dicentrarchus labrax). Aquaculture. 476:79–85.
- Makkar HPS, Tran G, Heuzé V, Ankers P. 2014. State-of-the-art on use of insects as animal feed. Anim Feed Sci Technol. 197:1–33.
- Matejova I, Svobodova Z, Vakula J, Mares J, Modra H. 2017. Impact of mycotoxins on aquaculture fish species: A review. J World Aquac Soc. 48(2):186–200.
- Nogales-Mérida S, Gobbi P, Józefiak D, Mazurkiewicz J, Dudek K, Rawski M, Kierończyk B, Józefiak A. 2018. Insect meals in fish nutrition. Rev Aquacult. doi:10.1111/raq.12281
- Poma G, Cuykx M, Amato E, Calaprice C, Focant JF, Covaci A. 2017. Evaluation of hazardous chemicals in edible insects and insect-based food intended for human consumption. Food Chem Toxicol. 100:70–79.
- R Core Team. 2011. R: a language and environment for statistical computing. Vienna (Austria): R Foundation for Statistical Computing. http://www.R-project.org/.
- Renna M, Schiavone A, Gai F, Dabbou S, Lussiana C, Malfatto V, Prearo M, Capucchio MT, Biasato I, Biasibetti E, et al. 2017. Evaluation of the suitability of a partially defatted black soldier fly (Hermetia illucens L.) larvae meal as ingredient for rainbow trout (Oncorhynchus mykiss Walbaum) diets. J Anim Sci Biotechnol. 8(1):57.
- Ronan JM, Stengel DB, Raab A, Feldmann J, O'Hea L, Bralatei E, McGovern E. 2017. High proportions of inorganic arsenic in laminaria digitata but not in ascophyllum nodosum samples from Ireland. Chemosphere. 186:17–23.
- Rose M, Lewis J, Langford N, Baxter M, Origgi S, Barber M, MacBain H, Thomas K. 2007. Arsenic in seaweed—forms, concentration and dietary exposure. Food Chem Toxicol. 45(7):1263–1267.
- Scheibelberger R, Axmann S, Adler A, Jäger H. 2017. Impact of substrate contamination with mycotoxins, heavy metals and pesticides on the growth performance and composition of black soldier fly larvae (Hermetia illucens) for use in the feed and food value chain AU - Purschke, Benedict. Food Addit Contam: Part A. 34(8):1410–1420.
- Sele V, Sanden M, Berntssen MHG, Lunestad BT, Espe M, Lie KK, Amlund H, Lundebye A-K, Hemre G-I, Waagbo R, et al. 2018. Program for overvåking av fiskefôr – Årsrapport for prøver innsamlet i 2017. Institute of Marine Research. https://www.imr.no.
- Sele V, Sloth JJ, Holmelid B, Valdersnes S, Skov K, Amlund H. 2014. Arsenic-containing fatty acids and hydrocarbons in marine oils – determination using reversed-phase HPLC–ICP-MS and HPLC–qTOF-MS. Talanta. 121:89–96.
- Sele V, Sloth JJ, Julshamn K, Skov K, Amlund H. 2015. A study of lipid- and water-soluble arsenic species in liver of Northeast Arctic cod (Gadus morhua) containing high levels of total arsenic. J Trace Elem Med Biol. 30:171–179.
- Sissener NH, Julshamn K, Espe M, Lunestad BT, Hemre GI, Waagbø R, Måge A. 2013. Surveillance of selected nutrients, additives and undesirables in commercial Norwegian fish feeds in the years 2000–2010. Aquac Nutr. 19(4):555–572.
- Sloth JJ, Julshamn K, Lundebye A-K. 2005a. Total arsenic and inorganic arsenic content in Norwegian fish feed products. Aquac Nutr. 11(1):61–66.
- Sloth JJ, Larsen EH, Julshamn K. 2003. Determination of organoarsenic species in marine samples using gradient elution cation exchange HPLC-ICP-MS. J Anal At Spectrom. 18(5):452–459. doi: 10.1039/B300508A
- St-Hilaire S, Sheppard C, Tomberlin JK, Irving S, Newton L, McGuire MA, Mosley EE, Hardy RW, Sealey W. 2007. Fly prepupae as a feedstuff for rainbow trout, oncorhynchus mykiss. J World Aquac Soc. 38(1):59–67.
- Tocher DR. 2010. Fatty acid requirements in ontogeny of marine and freshwater fish. Aquacult Res. 41(5):717–732.
- Torstensen BE, Frøyland L, Lie Ø. 2004. Replacing dietary fish oil with increasing levels of rapeseed oil and olive oil – effects on Atlantic salmon (Salmo salar L.) tissue and lipoprotein lipid composition and lipogenic enzyme activities. Aquac Nutr. 10(3):175–192.
- Van Broekhoven S, Gutierrez JM, De Rijk TC, De Nijs WCM, Van Loon JJA. 2017. Degradation and excretion of the Fusarium toxin deoxynivalenol by an edible insect, the Yellow mealworm (Tenebrio molitor L.). World Mycotoxin J. 10(2):163–169.
- Van den Berg M, Birnbaum LS, Denison M, De Vito M, Farland W, Feeley M, Fiedler H, Hakansson H, Hanberg A, Haws L, et al. 2006. The 2005 World Health Organisation re-evaluation of human and mammalian toxic equivalency factors for dioxins and dioxin-like compounds. Toxicol Sci. 93(2):223–241.
- van der Fels-Klerx H, Camenzuli L, Van Der Lee M, Oonincx D. 2016. Uptake of cadmium, lead and arsenic by tenebrio molitor and hermetia illucens from contaminated substrates. PLoS One. 11(11):e0166186.
- van Huis A. 2013. Potential of insects as food and feed in assuring food security. Annu Rev Entomol. 58:563–583.
- Villares R, Puente X, Carballeira A. 2002. Seasonal variation and background levels of heavy metals in two green seaweeds. Environ Pollut. 119(1):79–90.
- Yamada M, Yamamoto K, Ushihara Y, Kawai H. 2007. Variation in metal concentrations in the brown alga undaria pinnatifida in Osaka Bay, Japan. Phycol Res. 55(3):222–230.