ABSTRACT
Selective androgen receptor modulators (SARMs) are a class of new emerging “designer” steroid compounds gaining popularity over more well established anabolic-androgenic steroids (AAS) amongst both non-professional and elite athletes. Moreover, due to their anabolic activity, SARM compounds may also potentially be abused in livestock animals to increase meat production. Consequently, SARM residues should be monitored as a part of routine testing employed within both anti-doping and drug residue laboratories. Since only a limited amount of information on SARM compound stability is currently available within the peer-reviewed literature, this study reports a practical approach to assess optimal storage conditions for 15 SARM compounds in solvent solutions (standard stock and intermediate mixed standard solutions) stored at −20°C for up to 1 year, as well as in a range of urine test matrices (bovine, equine, canine and human) under frozen (−20°C, −80°C) storage for up to 20 weeks and post freeze-thaw cycles. Moreover, SARM storage stability within solvent extracts was assessed at −20°C (0–4 weeks) and 4°C (0–2 weeks). Findings demonstrate that SARM analytes are stable in reference solutions when stored at −20°C, apart from PF-06260414 (stock solution) which should be stored at lower temperatures (e.g. −80°C). A limited degree of compound instability was observed for a number of SARM analytes in urine both when stored at −20°C, and after repeated freeze-thaw cycles. Moreover, SARM compounds within reconstituted urine solvent extracts were found to be effectively stable when stored for up to 4 weeks at −20°C and for 2 weeks at 4°C. The long-term stability testing data reported here will inform the more timely and effective development and validation of analytical methods for SARM residue detection and analysis, ensuring confidence in findings from monitoring of livestock animals and anti-doping processes.
Graphical Abstract
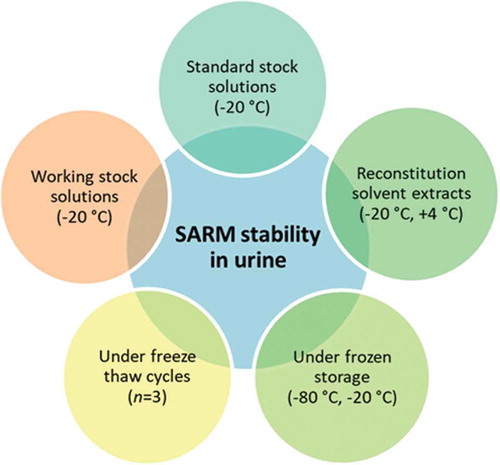
Introduction
Selective androgen receptor modulators (SARMs) are an emerging class of drug compounds first proposed in the late 1990s (Dalton et al. Citation1998) as potential new treatments for a range of human diseases, e.g. benign prostatic hyperplasia, androgen-deficiency related disorders and conditions involving muscle and bone wasting (Thevis and Volmer Citation2018). Whilst many SARM compounds are still currently in development or under clinical evaluation and have not yet been approved as pharmaceutical drugs, their anabolic properties, high tissue selectivity (bone and muscle), high rate of gastrointestinal absorption and restricted detection window after oral administration (Sobolevsky et al. Citation2015; Cesbron et al. Citation2017; Hansson et al. Citation2018), as well as reduced anti-androgen effects relative to other well-established anabolic-androgenic steroids (AAS) (Choi and Lee Citation2015; Narayanan et al. Citation2018) has led to SARM compounds being rapidly introduced within black market sources (Geldof et al. Citation2017) as a new more appetible class of “designer” steroids. Consequently, with a view to counteracting their misuse in both human and animal sports, SARMs have been banned by various controlling bodies (FEI Citation2019; IFHA Citation2019; WADA Citation2019). Moreover, the potential application of SARMs to improve carcase characteristics increases lean mass gain and so reduce production costs in livestock (e.g. cattle and pig) through animal feed incorporation has been proposed in various patents (Dalton et al. Citation2016). Nevertheless, any use of SARMs in livestock is considered an illicit practice under EU legislation through Council Directive 96/22/EC (EC Citation1996) which prohibits the use of growth-promoting agents in animal husbandry.
Fair sport performances and foods free from SARM residue contamination can only be ensured through reliable and sensitive analytical methods which can detect SARMs in a range of biological matrices. Even if various analytical procedures have been developed and validated for the identification of SARM compounds in a range of matrices including urine (Ventura et al. Citation2019), blood (Hansson et al. Citation2016, Citation2018), muscle (Gadaj et al. Citation2019) and faeces (Cesbron et al. Citation2017; Rojas et al. Citation2017), there remains a consistent lack of information concerning the stability of SARM residues and matrix constituents during test sample storage. Stability is defined as the ability of material when stored under specific conditions to maintain a stated property (e.g. concentration) within specified limits for a specific period of time (Ventura et al. Citation2003). Generally, stability studies are considered a fundamental parameter during the validation of new assays, as insufficient analyte stability may lead to significant deviation in the final outcome and interpretation of analytical findings (EC Citation2002; Croubels et al. Citation2003). Moreover, studies of stability both in solution and in biological samples constitute part of the standard ISO/IEC 17025 accreditation system in a state-of-the-art laboratory (EC Citation2002; Croubels et al. Citation2003), and experimental evidence of analyte stability is frequently demanded during accreditation audit (Stefanelli et al. Citation2015). As stability testing is time-consuming, the assessment of analyte stability can only be avoided in the case where this is well described in the peer-reviewed literature or has already been characterised in the laboratory (EC Citation2002), but unfortunately, this is not the case for many SARM compounds. In fact, to date, limited publications are available regarding SARM stability with two studies reporting the stability assessment of three arylpropionamide SARM compounds (andarine (S-4), bicalutamide and ostarine (S-22)) in solvent solutions and in bovine urine (de Rijke et al. Citation2013; Schmidt and Mankertz Citation2018), in addition to a third highlighting the stability of three SARMs (andarine, ostarine and S-1) in equine plasma following freeze-thaw cycles (Hansson et al. Citation2016). Furthermore, Gadaj et al. (Citation2019) recently reported on stability of a range of SARM compounds in bovine muscle highlighting the impact of frozen sample storage and freeze-thaw cycles on SARM residue stability in test matrix as well as in extracted samples.
Stability of SARM compounds in solvent solutions should also be determined to assess if standards used during routine analysis can assure accurate and reliable results. If analytes are not stable in solvent solutions, quantitative analytical assays may result in an underestimation of the true concentration of the analyte in the biological sample being tested, and consequently, this could lead to deviation in the correct interpretation of acquired results. Concurrently, the stability of SARM analytes within biological matrices also needs to be investigated as the analysis of samples collected during anti-doping drug testing and food or animal inspections is rarely performed immediately after collection (Ventura et al. Citation2003), with delays of often days or weeks between sampling, drug screening and confirmation of collated specimens (Adamowicz and Malczyk Citation2019). Additionally, laboratories may be obligated to preserve biological samples for a certain period post examination in order to allow further re-analysis to confirm previous findings (Cuhadar et al. Citation2013). Consequently, it is important to verify that analyte instability does not affect analytical outputs during the re-testing period, particularly for substances having a threshold concentration as positive criteria (Ventura et al. Citation2003). Moreover, preanalytical parameters such as transport conditions, improper storage and handling, may also alter analyte concentration within samples (Adamowicz and Malczyk Citation2019). Additional factors which may also alter analyte stability over time include, but not limited to, temperature, matrix type, pH, substance chemical structure (e.g. presence of esters and sulphur groups or photolabile moieties), concentration, addition of preservatives and storage container type (Jiménez et al. Citation2004; Kerrigan Citation2011; Adamowicz and Malczyk Citation2019). Consequently, analyte stability in biological specimens has to be determined under different storage conditions within the time frame comprising the period between collection and day of analysis. Such a process will guarantee that detected analyte concentration corresponds to the actual concentration at time of collection, and that it is not an underestimation due to analyte instability or interference from biological matrix components. Furthermore, since biological materials are commonly stored in frozen conditions until analysis, analyte stability needs to be assessed also after repeated freeze-thaw cycles (Jiménez et al. Citation2004). The daily demand and overload in sample analysis within laboratories can lead to the situation whereby sample extracts cannot be analysed directly and consequently extracted samples need to be stored until analysis at a later point. Thus, the stability of SARM residues in solvent extracts should also be investigated to ensure high precision and accuracy of test results. From a food safety perspective, even if stability studies are required in the frame of method validation as stated in several EU technical documents (e.g. 2002/657/EC (EC Citation2002), CRL 20/1/2010 (CRL Citation2010), SANTE/11813/2017 (European Commission Directorate General for Health and Food Safety Citation2017) and Codex Alimentarius (CAC Citation2014), the manner in which these studies are performed and how associated experimental data is evaluated is arbitrary, with only a general guidance commonly provided for such procedures. Following the EU Commission Decision 2002/657/EC guidelines, stability in matrix should be tested whenever possible in incurred samples; however, when these are not available, matrix fortified with the analyte of interest is deemed acceptable (EC Citation2002). Consequently, different approaches have been adopted to the performance of stability studies on analytes in reference solutions and in biological materials for both veterinary drug residue analysis (Berendsen et al. Citation2011; Gaugain et al. Citation2013) and anti-doping drug testing (Ventura et al. Citation2003; Jiménez et al. Citation2004).
Urine is one of the conventional test matrices utilised within the anti-doping arena, and likewise is the specimen of choice for the purpose of residue control (i.e. banned and/or non-authorised compounds) in food producing animals in accordance with current EU legislative guidelines (CRL Citation2007). This study therefore seeks to investigate the stability of 15 emerging SARM compounds in urine from various species (bovine, equine, canine and human) after storage at −20°C and −80°C, as well as under conditions of freeze-thaw cycles. Moreover, stability at 4°C and room temperature (18°C) was assessed solely for human urine, with urine sample extract stability in final injection solvent also evaluated at −20°C and 4°C. Furthermore, the stability of compound reference solutions was evaluated in both individual standard stock solutions and intermediate mixed standard solutions at −20°C.
Materials and methods
Reference standards
AC-262536 (P/N 96443–25MG), andarine (S-4, P/N 78986–25MG), bicalutamide (P/N PHR-1678-1G), LGD-2226 (P/N 07682–25MG), Ly2452473 (P/N CDS025139-50MG), PF-06260414 (P/N PZ0343-5MG), S-1 (P/N 68114–25MG), S-6 (P/N 79260–25MG) and S-23 (P/N 55939–25MG) were purchased from Sigma-Aldrich (Dublin, Ireland). LGD-4033 (P/N CAY9002046-50mg), ostarine (S-22, P/N MK-2866) and RAD140 (P/N CAY18773-1mg) were purchased from Cambridge Bioscience Ltd. (Cambridge, UK). BMS-564929 (10 mM solution in DMSO, P/N HV-12111) and GLPG0492 (10 mM solution in DMSO, P/N HY-18102) were purchased from MedChem Express (Sollentuna, Sweden). S-9 (P/N D289535), Bicalutamide-D4 (P/N B382002) and S-1-D4 (P/N D289532) were purchased from Toronto Research Chemicals (TRC; Toronto, Canada).
Samples
Urine samples were collected and previously tested as reported (Ventura et al. Citation2019): bovine urine samples from abattoirs across Ireland, equine urine samples donated by the Irish Equine Centre (IEC), canine urine samples provided by the Irish Greyhound Board and human urine samples donated by non-professional volunteer athletes. A pool of respective samples (n = 5–20) was used to execute stability studies.
Sample preparation for stability studies
Urine samples (bovine, equine, canine and human) were prepared and analysed as detailed elsewhere (Ventura et al. Citation2019). Briefly, SARM residues were extracted from urine (200 µL) with tert-butyl methyl ether (TBME) following the addition of 50 mM NH4OH pH 10.5 (200 µL), without further clean-up. Extracts concentrated under nitrogen and reconstituted in H2O:MeCN (4:1, v/v, 100 µL), were analysed by means of UHPLC-MS/MS (9 µL).
UHPLC-MS/MS SARM compound analysis
Detailed procedures for SARM analysis by means of UHPLC-MS/MS are described elsewhere (Ventura et al. Citation2019). Stable isotope-labelled analogues of bicalutamide and S-1 (bicalutamide-D4 and S-1-D4) were used as internal standards for arylpropionamide residues as reported previously (Ventura et al. Citation2019). The response factor was obtained for arylpropionamides as a ratio between analyte peak area and internal standard peak area, while in the case of other SARM residues, peak area was used as the response.
SARM compound stability assessment
Fridge and freezers stability was monitored and logged daily, whereas storage at room temperature (18°C) was carried out in a laboratory supplied with an air-conditioning system.
SARM solvent stability
The stability of 15 emerging SARMs was evaluated in both individual stock standard solutions (1 mg mL−1) and intermediate mixed standard solutions (1/2/5 µg mL−1), respectively. Since not all analytes of interest were available at the start of this study, initially the stability of single standard stock solutions was assessed for 13 SARMs during storage at −20°C for 12 months. All individual standard stock solutions were prepared at a concentration of 1 mg mL−1, by dissolving the accurately weighed quantity of a standard (taking into account the purity (%) of the standard in the particular batch of material as per Certificate of Analysis) in an appropriate solvent in a volumetric flask (5 or 10 mL). The following solvents were used: DMSO (AC-252636, andarine (S-4), LGD-2226, LGD-4033, Ly2452473, PF-06260414, RAD140 and S-23), acetonitrile (bicalutamide, ostarine (S-22) and S-1), and ethanol (S-6 and S-9). BMS-564929 and GLPG0492 were purchased as 10 mM standard solutions in DMSO and were diluted with DMSO to give a concentration of 1 mg mL−1. 1 mL aliquots of each individual standard stock solution (13 analytes) were stored in 2 mL amber autosampler vials capped with PTFE/silicone septa tops at −20°C for 12 months (t = 12). Prior to analysis (t = 0), an aliquot of each standard stock solution was removed from storage and allowed to equilibrate to room temperature. Thirteen analytes were combined in a single-mixed standard solution and diluted by serial dilutions with acetonitrile to 200 ng mL−1. Freshly prepared individual standard stock solutions were mixed and diluted with acetonitrile to the same concentration, and both mixed standard solutions were alternately analysed against each other (1 µL, n = 5 replicates) by UHPLC-MS/MS. The stability of the standard stock solutions of Ly2452473 and PF-06260414, which were acquired at a later stage of the study, was assessed separately as previously reported by Berendsen et al. (Citation2011). Respective standard stock solutions (1 mg mL−1) were aliquoted (5 × 0.5 mL) into 2 mL amber autosampler vials capped with PTFE/silicone septa tops. For each analyte at t = 0 (beginning of the stability study), one amber vial was placed at −80°C (reference temperature at which no degradation is assumed to take place) and four at −20°C (storage temperature), respectively. After 3, 6, 9 and 12 months, the respective amber vials were moved from −20°C to −80°C. After 12 months (t = 12, end of the stability study), all amber vials – one per analyte for each time point (0, 3, 6, 9 and 12 months) were removed from storage and placed at room temperature to equilibrate. Respective standard stock solutions of Ly2452473 and PF-06260414 were combined and diluted by serial dilutions with acetonitrile to give a total of five solutions at a concentration of 200 ng mL−1, and subsequently analysed against the control solution stored at −80°C from the beginning of the experiment (1 µL, n = 3 repeated injections) by means of LC-MS/MS.
Stability of the 15 SARM compounds in an intermediate mixed standard solution in acetonitrile was assessed at a concentration of 1 µg mL−1, except for andarine (2 µg mL−1) and BMS-564929 (5 µg mL−1), respectively (Ventura et al. Citation2019). The stability study was scheduled as detailed above for Ly2452473 and PF-06260414 stock solutions. Briefly, after 12 months (t = 12, end of the stability study), 2 mL amber autosampler vial (0.5 mL aliquot) for each time point (0, 3, 6, 9 and 12 months) was removed from the storage and placed at room temperature to equilibrate. Respective intermediate mixed standard solutions were diluted with acetonitrile to give a total of five solutions at a concentration of 200/400/1000 ng mL−1 and subsequently analysed against the control solution stored at −80°C from the beginning of the experiment (1 µL, n = 3 repeated injections) by LC-MS/MS.
In respect of stability studies detailed above, all relevant samples were analysed by UHPLC-MS/MS following randomisation within the same analytical batch performed at the end of the stability study period. The average peak area and relative standard deviation (CV %) were calculated for each storage time.
SARM stability within urine matrix
A number of studies were executed to assess the stability of SARMs in a range of test sample matrices, i.e. bovine, equine, canine and human urine – the first assessed the impact of frozen storage (−20°C, −80°C) over time with the second examining the effect of freeze-thaw cycles. Moreover, stability at 4°C, as well as room temperature (18°C), was determined solely for human urine as biological material could be transported at undefined conditions. Stability was determined in blank urine samples (pool n = 5–20) fortified at the concentration equal to five times the screening target concentration (5 × Cval, n = 5 replicates), namely: 5 ng mL−1 excluding andarine (10 ng mL−1) and BMS-564929 (25 ng mL−1). In the frozen storage study, fortified urine samples were analysed following storage in 2 mL polypropylene tubes at −20°C and −80°C, after 0, 1, 2, 4, 8, 12 and 20 weeks, respectively. In the freeze-thaw cycle study, the fortified urine was subjected to 0–3 freeze-thaw cycles. Samples were thawed for 1 h at room temperature and the consecutive freeze-thaw cycles were repeated following 24 h intervals. In the case of stability studies performed in test matrices, results were expressed as absolute recovery (%) as determined by comparing results from fortified samples to those of negative samples spiked post-extraction at 5 × Cval (n = 2).
SARM stability within extracted sample
A further study investigated the stability of SARMs in sample extracts within final injection solvent under storage in autosampler vials at −20°C (0–4 weeks) and 4°C (0–2 weeks), respectively. Stability was assessed in blank urine (bovine, equine, canine and human) samples (pool n = 5–20) spiked post-extraction at 5 × Cval (n = 5 replicates).
In relation to stability studies carried out either in test matrices or sample extracts, all relevant samples were analysed by means of UHPLC-MS/MS following randomisation within the same analytical run performed at the end of the stability study period, with samples fortified and/or spiked at the day of analysis (day 0) used as the control.
Acceptability criteria of stability
Although stability studies of analytes in reference solutions and biological materials are strongly demanded to fulfil the criteria of method validation, no EU technical document provides a guideline on how such studies should be carried out and which criteria need to be considered to assess analyte (in)stability. A general guide on how to evaluate the stability of individual stock solutions is given solely by the SANTE/11813/2017 legislation (SANTE Citation2017), which was applied in the current study: “the stability of single stock solutions should be verified by comparing the average signals (e.g. peak areas) of individual components of the old and freshly prepared standard solutions, obtained by alternately injecting the old and the new ones, with a minimum of five replicates. The average signals should not normally differ by more than ±10% if the average signal of the new standard is 100% and the relative difference is calculated”. On the other hand, no guidelines are available to assess the stability of analyte in biological matrices, and consequently, the choice of procedures and criteria remains entirely arbitrary. Consequently, some information can be deduced from various stability studies already reported in the scientific literature in the arena of veterinary drug residue analysis. Berendsen et al. (Citation2011) assessed the stability of a range of antibiotics in matrix through a quantitative confirmatory analysis applying 10% criterion and assuming an analyte instability in a case of the relative response drop below 90%. However, Gaugain et al. (Citation2013) stated that the application of only a t-test is not sufficient to evaluate the stability of antibiotics in matrix, and that a criterion of ±20% also has to be taken into consideration to determine what difference of averages is detectable accounting for repeatability parameters. Moreover, this limit of ±20% should not be more restrictive than the limits applied to the precision and accuracy of analytical methods as described in the Commission Decision 2002/657/EC (EC Citation2002). Therefore, the ±20% criterion is a quite sufficient parameter to assess the stability of fortified biological matrix when applying a multi-residue screening assay. Consequently, in the current study, a compound was considered unstable in the reference solution if the relative response dropped below 90% (Croubels et al. Citation2003; Berendsen et al. Citation2011; Gaugain et al. Citation2013; Stefanelli et al. Citation2015; SANTE Citation2017) together with the low variability of replicate injections (CV%) as acceptance criteria (Gaugain et al. Citation2013; SANTE Citation2017). A criterion parameter of ±20% was applied to assess analyte stability within biological matrix, and repeated measures one-way Analysis of Variance (ANOVA) with Tukey’s multiple comparison post-test performed on stability study datasets using GraphPad Prism version 5.00 (GraphPad Software, San Diego, California, USA), and a confidence level of 95% set as a further acceptable criterion. Consequently, compounds were considered unstable if the (relative) response varied more than ±20% (Gaugain et al. Citation2013) together with p < .05.
Results and discussion
SARM stability in solvent
Stability of SARM compounds in solvent was assessed in individual standard stock solutions (1 mg mL−1) stored at −20°C for 12 months, excluding Ly2452473 and PF-06260414 monitored following storage for 3, 6, 9 and 12 months, and in an intermediate mixed standard solution (1/2/5 µg mL−1) stored at −20°C for 1, 3, 6, 9 and 12 months, respectively. The relative response was calculated by dividing the average response at t = n (where n = 1, 3, 6, 9, 12 months) by the average response at t = 0 and expressed as %.
Applying the criteria discussed above (Acceptability Criteria of Stability Section), all SARM analytes were found to be stable at −20°C in the individual standard stock solutions for at least 12 months (CV≤3.2%) (Supplementary data – Figure S1), except for PF-06260414 which showed significant instability (17.6%, CV≤1.5%) after 12 months of storage (Supplementary data – Figure S2). Consequently, it would be recommended to store PF-06260414 standard stock solution at a lower temperature (e.g. ≤−80°C). All SARMs of interest were found to be stable in an intermediate mixed standard solution when stored at −20°C for 12 months (CV≤4.3%) (Supplementary data – Figure S3). These observations are in agreement with findings reported by de Rijke et al. (Citation2013) and Schmidt and Mankertz (Citation2018) regarding the stability of solvent solutions of selected arylpropionamides (andarine (S-4), bicalutamide and ostarine (S-22)) with all analytes described to be stable in individual stock solutions (1 mg mL−1 in EtOH) when stored at −18°C for at least 12 months (de Rijke et al. Citation2013). Furthermore, the same analytes were reported to show sufficient stability in solvent solution (10 ng mL−1 in MeOH) even when stored at 20°C in light for 3 months (Schmidt and Mankertz Citation2018).
SARM stability in matrix
The stability of SARMs was assessed in fortified urine samples stored for 1, 2, 4, 8, 12 and 20 weeks at −20°C (Supplementary data – Figure S4) and −80°C (Supplementary data – Figure S5), respectively, except for bovine urine stability () which was monitored only at −20°C. AC-262536 showed instability (response variation more than ±20% with p < .05) in the case of canine (8 weeks) and human (2 weeks), urine, respectively, stored at −20°C. Moreover, AC-262536 was the only compound investigated found to be significantly unstable in urine stored at −80°C, i.e. canine (2 weeks) and human (4 weeks). Furthermore, LGD-4033 showed instability in human urine after 20 weeks of storage at −20°C. In addition, some compounds (i.e. AC-262536, Ly2452472 and S-1 in bovine urine at −20°C; bicalutamide in equine urine at −20°C) presented a trend towards instability even if set criteria (response variation more than ±20% with p < .05) were not fulfilled. Therefore, it is recommended that urine samples are kept at ≤−80°C when long-term storage is required and furthermore, whenever SARM compound incurred urine samples are available stability under frozen storage conditions should be examined. Schmidt and Mankertz (Citation2018) previously reported findings regarding the stability of selected arylpropionamides (andarine (S-4), bicalutamide and ostarine (S-22)) in bovine urine with no instability of analytes fortified at a concentration of 1 ng mL−1 observed in samples stored at −30°C for 4 weeks.
Figure 1. Stability of selected SARMs in bovine urine under frozen storage (−20°C). Mean recovery (and standard deviations, shown by error bars, n = 5) obtained from fortified blank bovine urine. Where p < .05 (*), < .01 (**) and < .001 (***), and – – response limits (±20%).
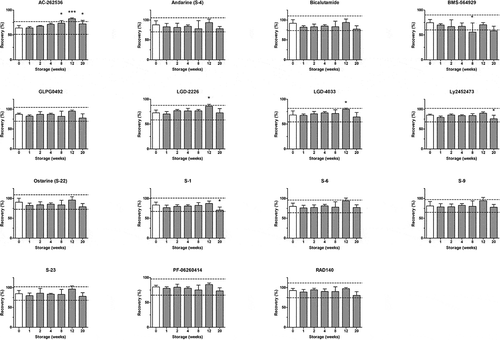
Investigation of SARM compound stability at 4°C as well as room temperature (18°C) (Supplementary data – Figure S6) highlighted that storage and/or shipping of human urine at these conditions should be kept to a minimum or avoided where possible, as significant instability (response variation more than ±20% with p < .05) was observed for both examined temperatures for AC-262536, whereas PF-06260414 was found to be significantly unstable in samples stored at room temperature. Moreover, some compounds (i.e. BMS-564929, GLPG0492 and RAD140 at room temperature; Ly2452473 and LGD-4033 at both 4°C and room temperature) showed a tendency towards instability even though defined criteria (response variation more than ±20% with p < .05) were not met.
Investigation of SARM residue stability in urine (bovine, equine, canine and human) during 0 to 3 freeze-thaw cycle conditions ( and Supplementary data – Figure S7) revealed instability (response variation more than ±20% with p < .05) solely for ostarine (S-22) in equine urine following two cycles. Moreover, some compounds (i.e. bicalutamide and S-6 in equine urine; LGD-2226 in bovine urine) showed a tendency towards instability even if set criteria (response variation more than ±20% with p < .05) were not met. Thus, repeated freeze-thaw cycles should be avoided, and it is recommended that test samples are sub-aliquoted on the day of acquisition for subsequent analysis. Moreover, as mentioned previously, whenever SARM incurred urine samples become available, the effect of freeze-thaw cycles should be assessed.
Processed sample SARM stability
The stability of SARM residues was also evaluated in urine (bovine, equine, canine and human) extracts ( and Supplementary data – Figure S8) within final injection solvent following storage at −20°C for 1, 2 and 4 weeks, and at 4°C for 1 and 2 weeks, respectively. No significant instability (relative response ±20% with p < .05) was observed in biological specimens of interest. Nevertheless, some compounds within urine extracts (i.e. PF-06260414 in equine urine at 4°C and −20°C; AC-262536, BMS-564929, GLPG0492, LGD-2226, LGD-4033 and Ly2452473 in equine urine at −20°C; BMS-564929 and PF-06260414 in canine urine at both 4°C and −20°C; andarine in canine urine at 4°C; and PF-06260414 in human urine at 4°C) showed tendency towards instability even if set criteria (relative response ±20% with p < .05) were not met. Consequently, this would indicate that urine extracts could be stored at −20°C for at least 4 weeks, and at 4°C for at least 2 weeks prior to analysis.
Figure 3. Stability of selected SARMs in bovine sample extracts – final injection solvent. Mean response (and standard deviations, shown by error bars, n = 5) obtained from blank bovine urine samples spiked post extraction. Control samples expressed as 100%. Where p < .05 (*), < .01 (**) and < .001 (***), and – – relative response limits (±20%).
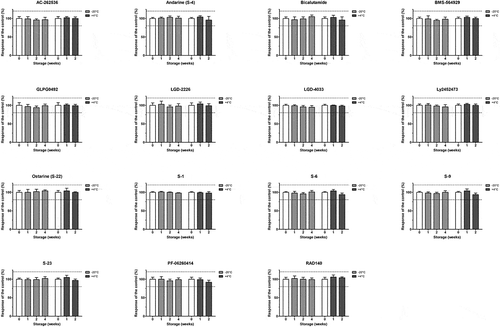
de Rijke et al. (Citation2013) reported the stability of selected arylpropionamide residues (andarine (S-4), bicalutamide and ostarine (S-22)) in bovine urine extracts obtained by liquid-liquid extraction with TBME following enzymatic hydrolysis and pH adjustment using K2CO3/NaHCO3 buffer (de Rijke et al. Citation2013), with all analytes shown to be stable post storage at −18°C for 1 week.
Conclusions
As a consequence of the fact that generally a considerable time interval can elapse between sample collection and analysis of biological material, instability of SARMs during storage may affect the integrity of analytical findings. Moreover, knowledge on the decomposition of SARM compounds in reference standard solutions is an essential requirement while validating analytical methods to allow correct interpretation of findings during routine testing within laboratories. Therefore, the stability of SARMs in solvent solutions and test matrices of interest (urine) was investigated demonstrating that individual SARM standard stock solutions can be stored at −20°C for at least 1 year, except for PF-06260414 which should be stored at lower temperatures (e.g. ≤ −80°C). Furthermore, all SARMs were found to be stable in intermediate mixed standard solution under the same storage conditions. Acquired stability data of SARM compounds in biological materials suggest that it is preferable to keep test urine samples at ≤−80°C where long-term storage is required. Moreover, storage and/or shipping of human urine at 4°C or room temperature should be kept to a minimum or avoided where practically possible. Furthermore, findings suggest repeated freeze-thaw cycles should be avoided when managing urine specimens, and moreover, urine sample extracts in final injection solvent were found to be sufficiently stable and may be stored prior to analysis at −20°C for up to 4 weeks or for 2 weeks at 4°C.
The considerable amount of data presented in this study can be useful and time-saving to support the validation process of future analytical methodologies focused on detection of SARM compounds in urine matrices, as stability studies are laborious, time-consuming, and expensive. Moreover, stability data are often part of the quality assurance system in a reference laboratory and so rarely published within the scientific literature (Stefanelli et al. Citation2015). Consequently, these studies will support future residue controls in livestock, as well as anti-doping drug testing methodologies in human and animal sports competition, and be of benefit for any laboratory currently running analytical methods for SARM detection. However, acquisition of stability data of SARMs remains an ongoing process because other matrices (e.g. faeces) and other species (e.g. porcine) may also be studied, and as more SARM compounds become available on the black market additional studies will be required. In conclusion, the authors of this paper would like to propose that more specific guidelines and criteria of acceptability to assess the analyte stability in biological specimens should be defined and incorporated within existing legislative guidelines of widespread use in the validation of analytical methods.
Supplemental Material
Download MS Word (678.2 KB)Acknowledgments
The authors Emiliano Ventura and Anna Gadaj contributed equally to the work in this paper.
Disclosure statement
The authors declare no potential conflict of interest.
Supplementary material
Supplemental data for this article can be accessed on the publisher’s website.
Additional information
Funding
References
- Adamowicz P, Malczyk A. 2019. Stability of synthetic cathinones in blood and urine. Forensic Sci Int. 295:36–45. doi:10.1016/j.forsciint.2018.12.001.
- Berendsen BJA, Elbers IJW, Stolker AAM. 2011. Determination of the stability of antibiotics in matrix and reference solutions using a straightforward procedure applying mass spectrometric detection. Food Addit Contam. 28:1657–1666. doi:10.1080/19440049.2011.604045.
- Cesbron N, Sydor A, Penot M, Prevost S, Le Bizec B, Dervilly-Pinel G. 2017. Analytical strategies to detect enobosarm administration in bovines. Food Addit Contam. 34:632–640. doi:10.1080/19440049.2016.1258122.
- Choi SM, Lee B-M. 2015. Comparative safety evaluation of selective androgen receptor modulators and anabolic androgenic steroids. Expert Opin Drug Saf. 14:1773–1785. doi:10.1517/14740338.2015.1094052.
- [CAC] Codex Alimentarius Commission. 2014. Guidelines for the design and implementation of national regulatory food safety assurance programme associated with the use of veterinary drugs in food producing animals. CAC/GL 71-2009. p. 1–42.
- [CRL] Community Reference Laboratories. 2007. Community Reference Laboratories (CRL) Guidance Paper (7 December 2007). CRLs View on State of the Art Analytical Methods for National Residue Control Plans. p. 1–8.
- CRL. 2010. Community Reference Laboratories Residues (CRLs) 20/1/2010. Guidelines for the validation of screening methods for residues of veterinary medicines (initial validation and transfer). p. 1–18.
- Croubels S, De Baere S, De Backer P. 2003. Practical approach for the stability testing of veterinary drugs in solutions and in biological matrices during storage. Anal Chim Acta. 483:419–427. doi:10.1016/S0003-2670(02)01479-4.
- Cuhadar S, Koseoglu M, Atay A, Dirican A. 2013. The effect of storage time and freeze-thaw cycles on the stability of serum samples. Biochemia Med. 23:70–77. doi:10.11613/BM.2013.009.
- Dalton JT, Miller DD, Kearbey JD 2016. SARMs and method of use thereof. US009278914B2, United States Patent. https://patentimages.storage.googleapis.com/5e/d2/77/51ddcb0a4e882e/US9278914.pdf.
- Dalton JT, Mukherjee A, Zhu Z, Kirkovsky L, Miller DD. 1998. Discovery of nonsteroidal androgens. Biochem Biophys Res Commun. 244:1–4. doi:10.1006/bbrc.1998.8209.
- de Rijke E, Essers ML, Rijk JCW, Thevis M, Bovee TFH, van Ginkel LA, Sterk SS. 2013. Selective androgen receptor modulators: in vitro and in vivo metabolism and analysis. Food Addit Contam. 30:1517–1526. doi:10.1080/19440049.2013.810346.
- [EC] European Commission. 1996. Council Directive 96/22/EC of 29 April 1996 concerning the prohibition on the use in stockfarming of certain substances having a hormonal or thyrostatic action and of beta-agonists, and repealing Directives 81/602/EEC, 88/146/EEC and 88/299/EEC.
- [EC] European Commission. 2002. Commission Decision 2002/657/EC of 12 August 2002 implementing Council Directive 96/23/EC concerning the performance of analytical methods and interpretation of results. Off J Eur Commun L. 221:8–36.
- [FEI] Fédération Equestre Internationale. 2019. 2019 Equine prohibited substances list. https://inside.fei.org/system/files/2019%20Equine%20Prohibited%20Substances%20List.pdf
- Gadaj A, Ventura E, Ripoche A, Mooney MH. 2019 Dec 30. Monitoring of selective androgen receptor modulators in bovine muscle tissue by ultra-high performance liquid chromatography-tandem mass spectrometry. Food Chem. 4:100056. doi:10.1016/j.fochx.2019.100056.
- Gaugain M, Chotard M-P, Verdon E. 2013. Stability study for 53 antibiotics in solution and in fortified biological matrixes by LC/MS/MS. J AOAC Int. 96:471–480. doi:10.5740/jaoacint.12-062.
- Geldof L, Pozo OJ, Lootens L, Morthier W, Van Eenoo P, Deventer K. 2017. In vitro metabolism study of a black market product containing SARM LGD-4033. Drug Test Anal. 9:168–178. doi:10.1002/dta.1930.
- Hansson A, Knych H, Stanley S, Berndtson E, Jackson L, Bondesson U, Thevis M, Hedeland M. 2018. Equine in vivo-derived metabolites of the SARM LGD-4033 and comparison with human and fungal metabolites. J Chromatogr B. 1074–1075:91–98. doi:10.1016/j.jchromb.2017.12.010.
- Hansson A, Knych H, Stanley S, Thevis M, Bondesson U, Hedeland M. 2016. Investigation of the selective androgen receptor modulators S1, S4 and S22 and their metabolites in equine plasma using high-resolution mass spectrometry. Rapid Commun Mass Spectrom. 30:833–842. doi:10.1002/rcm.7512.
- [IFHA] International Federation of Horseracing Authorities. 2019. International Agreement on Breeding, Racing and Wagering (IABRW). Article 6A Prohibited substances. https://www.ifhaonline.org/resources/ifAgreement.pdf
- Jiménez C, Ventura R, Segura J, De La Torre R. 2004. Protocols for stability and homogeneity studies of drugs for its application to doping control. Anal Chim Acta. 515:323–331. doi:10.1016/j.aca.2004.03.045.
- Kerrigan S 2011. Sampling, storage and stability. Clarke´s Analysis of Drug and Poisons. p. 445–457. https://pdfs.semanticscholar.org/a919/b24c9667844c627ab9f61df157bc0856dff2.pdf.
- Narayanan R, Coss CC, Dalton JT. 2018. Development of selective androgen receptor modulators (SARMs). Mol Cell Endocrinol. 465:134–142. doi:10.1097/OGX.0000000000000256.Prenatal.
- Rojas D, Dervilly-Pinel G, Cesbron N, Penot M, Sydor A, Prévost S, Le Bizec B. 2017. Selective androgen receptor modulators: comparative excretion study of bicalutamide in bovine urine and faeces. Drug Test Anal. 9:1017–1025. doi:10.1002/dta.2113.
- European Commission Directorate General for Health and Food Safety. 2017. SANTE/11813/2017. Guidance document on analytical quality control and method validation procedures for pesticide residues and analysis in food and feed. https://ec.europa.eu/food/sites/food/files/plant/docs/pesticides_mrl_guidelines_wrkdoc_2017-11813.pdf
- Schmidt KS, Mankertz J. 2018. In-house validation of a liquid chromatography–tandem mass spectrometry method for the determination of selective androgen receptor modulators (SARMS) in bovine urine. Food Addit Contam. 35:1292–1304. doi:10.1080/19440049.2018.1471222.
- Sobolevsky T, Dikunets M, Rodchenkov G 2015. Metabolism study of the selective androgen receptor modulator LGD-4033. 33rd Cologne Workshop on Dope Analysis, Manfred-Donike Institute, Cologne, Germany. p. 75–79.
- Stefanelli P, Barbini DA, Amendola G, Generali T, Girolimetti S, Pelosi P, Santilio A. 2015. A survey on long-term stability of stock standard solutions in pesticide residue analysis. Food Anal Methods. 8:1164–1172. doi:10.1007/s12161-014-9994-4.
- Thevis M, Volmer DA. 2018. Mass spectrometric studies on selective androgen receptor modulators (SARMs) using electron ionization and electrospray ionization/collision-induced dissociation. Eur J Mass Spectrom. 24:145–156. doi:10.1177/1469066717731228.
- Ventura E, Gadaj A, Monteith G, Ripoche A, Healy J, Botrè F, Sterk SS, Buckley T, Mooney MH. 2019 Apr 22. Development and validation of a semi-quantitative ultra-high performance liquid chromatography-tandem mass spectrometry method for screening of selective androgen receptor modulators in urine. J Chromatogr A. 1600:183–196. doi:10.1016/j.chroma.2019.04.050.
- Ventura R, Jiménez C, Closas N, Segura J, De La Torre R. 2003. Stability studies of selected doping agents in urine: caffeine. J Chromatogr B. 795:167–177. doi:10.1016/S1570-0232(03)00557-9.
- [WADA] World Anti-Doping Agency. 2019. The 2019 Prohibited List International Standard. https://www.wada-ama.org/sites/default/files/wada_2019_english_prohibited_list.pdf