Abstract
Spent brewery grains (BSG) are the main by-product of beer production and are incorporated in rations of food-delivering animals, mainly dairy cows. Like other agricultural commodities, BSG can be contaminated by a broad spectrum of natural and synthetic undesirable substances, which can be hazardous to animal and human health as well as to the environment. The co-occurrence of mycotoxins, phytoestrogens, other fungal and plant secondary metabolites, along with pesticides, was investigated in 21 BSG samples collected in dairy farms in Austria. For this purpose, a validated multi-metabolite liquid chromatography/electrospray ionisation tandem mass spectrometry (LC/ESI–MS/MS) was employed. Metabolites derived from Fusarium, Aspergillus, Alternaria and pesticide residues, were ubiquitous in the samples. Zearalenone (ZEN), T-2 and HT-2 toxins were the only regulated mycotoxin detected, albeit at concentrations below the European guidance values for animal feeds. Ergot alkaloids, Penicillium-derived metabolites, and phytoestrogens had occurrence rates of 90, 48 and 29%, respectively. Penicillium metabolites presented the highest levels among the fungal compounds, indicating contamination during storage. Aflatoxins (AFs), ochratoxins and deoxynivalenol (DON) were not detected. Out of the 16 detected pesticides, two fungicides, ametoctradin (9.5%) and mandipropamid (14.3%) revealed concentrations exceeding their respective maximum residue level (MRL) (0.01 mg kg−1) for barley in two samples. Although based on European guidance and MRL values the levels of the detected compounds probably do not pose acute risks for cattle, the impact of the long-time exposure to such mixtures of natural and synthetic toxicants on animal health and food safety are unknown and must be elucidated.
Introduction
The use of agro-industrial by-products as valuable feeds in dairy farming is a strategy that can reduce the direct dependence on whole cereals grains and oilseeds, which are essential in human nutrition. Due to the low cost of by-products, their incorporation into livestock rations improves the economics, also contributing to increasing the edible feed conversion ratio (Bocquier and González-García Citation2010; Ertl et al. Citation2015). Beer is the most consumed alcoholic beverage in Europe and worldwide (Violino et al. Citation2020; Ambra et al. Citation2021). Spent brewery grains (BSG) are the main by-product of beer production, accounting for around 85% of its agricultural waste and allowing the availability of this by-product throughout the year (Mussatto Citation2014; Petit et al. Citation2020). Most of the generated BSG are utilised in animal nutrition; however, this product has also been used for biogas production or, in a minor proportion, simply disposed of in landfill (Bianco et al. Citation2020). The vast potential of this by-product as a valuable feed/foodstuff in animal and human nutrition is because of a high content of digestible fibre (usually fluctuating from 30 to 50% on a dry matter (DM) basis), good quality protein (varying from 19 to 30% on DM basis), lipids and several minerals (José et al. Citation2013; Mussatto Citation2014; Lynch et al. Citation2016). Additionally, the BSG contain arabinoxylans and β-glucans, which can be used as prebiotics, promoting the activity of beneficial bacteria, such as Bifidobacterium, Enterococcus, and Lactobacillus species in monogastric livestock (Lao et al. Citation2020). In the feeding of ruminants, wet BSG are ideal for mixing with forage rations, offering high-quality protein feed, which is inexpensive and can reduce the dependency on commercial concentrate feeds (Gonzalez Pereyra et al. Citation2011). Although BSG have been fed to beef cattle, horses, pigs, sheep and poultry, the primary market for wet BSG is as a dairy cattle feedstuff (Westendorf and Wohlt Citation2002; Mussatto Citation2014; Kamboh Citation2017; Pack et al. Citation2021).
Like other agricultural products, the presence of contaminants and residues in BSG is associated with feed safety issues. Potential hazards of feedstuffs can be of natural (e.g. mycotoxins and plant toxins) as well as synthetic origin (like pesticides) (FAO and WHO Citation2019). Safety of wet BSG can be jeopardised by hundreds of fungal toxic compounds, which can be produced pre- and postharvest (especially during storage on the farms). However, most studies have investigated only a limited number of them in feedstuffs (including BSG) and other agricultural commodities. Specifically, research on mycotoxin contamination has focused mostly on a limited range of mycotoxins such as aflatoxins (AFs), fumonisins (FBs), trichothecenes, ochratoxin A (OTA) and zearalenone (ZEN) (Lynch et al. Citation2016; Cinar and Onbaşı Citation2019; Battilani et al. Citation2020; Pack et al. Citation2021), which are regulated by European legislation (EC Citation2002, 2006, 2012, 2013). Barley is the main cereal utilised for beer production (Palmer Citation2018). Like other cereal grains, barley is susceptible to mould infection with subsequent mycotoxin contamination and other secondary metabolites during the complete feed-production chain, pre-and postharvest (Pascari et al. Citation2018). Thus, research concerning other fungal toxins such as emerging and modified mycotoxins from Fusarium, Alternaria, Aspergillus and Penicillium is still limited but often advocated (Battilani et al. Citation2020; Lao et al. Citation2020). Additionally, other compounds of natural origin like phytoestrogens can occur in feeds, affecting animal farming. For instance, these plant secondary metabolites are known endocrine disruptors that, at certain levels, can impair reproductive functions and reducing the productive efficiency of dairy herds (Wocławek-Potocka et al. Citation2008, Citation2013).
The pre- and post-harvest use of pesticides under conventional farming systems protects crops, like barley, from insects, pests, weeds and plant pathogens, improving the production yields. Residues of such pesticides can be accumulated in crops and the environment, with potentially toxic effects on human and animal (including wildlife) health as well as on the soil microorganisms (Igbedioh Citation1991; Damalas and Eleftherohorinos Citation2011; Cozma et al. Citation2017). Additionally, pesticide applications can generate the presence of residues, which are usually at lower levels than the mycotoxins and phytoestrogens, but which should be analysed in order to address the exposure to entire mixtures of toxicants and endocrine-disrupting chemicals (EDCs), which can have public health and environmental implications (Igbedioh Citation1991; Connolly Citation2009; Rivera-Becerril et al. Citation2017; Guo et al. Citation2020; Geissen et al. Citation2021; Pires et al. Citation2021). Like some mycotoxins, pesticides are also regulated by European Union legislation (specifically by Regulation (EC) No 396/2005) (EC Citation2005). Thus, this study aimed to analyse a broad-spectrum profile of mycotoxins, phytoestrogens, other secondary metabolites, and pesticide residues in wet BSG intended to feed Austrian dairy cattle. This was achieved using a validated multi-analyte method based on liquid chromatography-electrospray ionisation tandem mass spectrometry (LC-ESI-MS/MS).
Materials and methods
Sample collection and preparation
Representative samples of wet BSG intended for dairy cattle feeding were collected from batches acquired by farmers from regional breweries (). The sampling was conducted between June and September 2020 from 21 conventional dairy farms located in Lower Austria (n = 9), Upper Austria (n = 8) and Styria (n = 4) (). Upon sample collection, BSG batches had been stored for less than two weeks at the respective farms. Each representative sample was collected manually, consisting of 20–30 incremental subsamples (randomly selected handfuls) using nitrile gloves, collected superficially (not deeper than 20 cm) from the next-to-be-fed section of the wet BSG (). The incremental samples were composited, giving a final sample amount of 1.5 kg, vacuum-packed in plastic bags and stored at −20 °C in the dark until sample preparation. Subsequently, the samples were thawed for 12 h, freeze-dried in a SCANVAC CoolSafe™ freeze (Labogene, Lillerød, Denmark) for 24 h, and milled through a 0.5 mm sieve using a cutting mill (SM 300, Retsch GmbH, Haan, Germany) at 1500 rpm for approximately 1 min. Five grams (± 0.01 g) of the homogenised samples were added to 50 ml polypropylene conical tubes (Sarstedt, Nümbrecht, Germany) and stored at −20 °C until analysis. The DM content of the sampled wet BSG was, on average, 25.3%, fluctuating from 20.9 to 30.6%. The farms were in altitude ranges between 266 and 814 m. The temperature during the months of sampling of participating farms fluctuated from 14.8 to 21.5 °C, being on average 18.4 °C (According to the Agency of Meteorology and Geodynamics, Zentralanstalt für Meteorologie und Geodynamik (ZAMG), https://www.zamg.ac.at/cms/de/klima/klimauebersichten/jahrbuch).
Figure 1. Representative sampling of wet barley brewery’s spent grains (BSG) intended for feed dairy cattle in Austria. A) The samples were collected from piles or silo bags, which were stored for a maximum of 2 weeks (Picture gently provided by Mr. Alexander Kopper©). B) The farms (n = 21) were in Lower Austria (n = 9), Upper Austria (n = 8) and Styria (n = 4). C) Subsamples were collected manually (around 20 − 30 handfuls) from the next-to-be-fed section of the BSG charge of each farm, for a final sample of 1 − 1.5 kg.
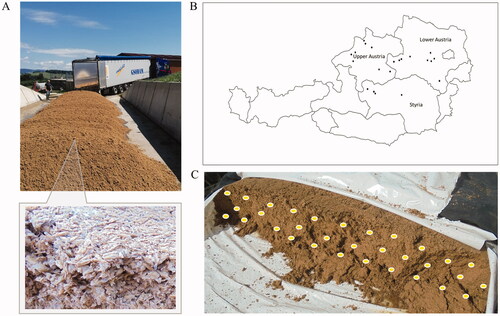
Analysis multiple of metabolites and pesticides
The previously dried and milled sample (5 ± 0.01 g) was placed into a 250 ml Erlenmeyer flask with 20 ml of extraction solvent according to the protocol described by Steiner et al. (Citation2020). After agitation with a GFL 3017 rotary shaker (GFL, Burgwedel, Germany) for 90 min, the solvent solution-sample mixture was centrifuged for 2 min at 2012 × g on a GS-6 centrifuge (Beckman Coulter Inc., Brea, CA). The extract was diluted 1:1 with dilution solvent. The injection volume of both diluted extracts of the samples and the standard analyte solutions was 5 µl. Identification and quantification of each analyte were performed in the mode of multiple reaction monitoring with positive and negative polarity in two separate chromatographic runs using a QTrap 5500 LC-MS/MS system (Applied Biosystems, Foster City, CA) equipped with a TurboV electrospray ionisation (ESI) source coupled to a 1290 series UHPLC system (Agilent Technologies, Waldbronn, Germany). Quantitative analysis of all the analytes was performed using a validated method based on LC-ESI-MS/MS described by Sulyok et al. (Citation2020). Quantification was based on external calibration using a serial dilution of a multi-analyte stock solution. Results were corrected for apparent recoveries determined during method validation according to Steiner et al. (Citation2020). The values of the method performance (apparent recovery, limit of detection [LOD] and limit of quantification [LOQ]) of each analyte are presented in . The apparent recovery for each pesticide was calculated using the equation proposed by Awapak et al. (Citation2021). The method’s accuracy is verified on a routine basis by participating in a proficiency testing scheme organised by BIPEA (Genneviliers, France) with current z-scores between −2 and 2 indicating > 95% confidence. All values submitted for a sample of wheat chaff were within this satisfactory range.
Table 1. Performance values of LC–MS/MS analysis targeting fungal and other contaminants as well as pesticide residues detected in wet brewery’s spent grains intended for dairy cattle nutrition.
Data analysis
Concentrations of all detected contaminants, residues, and other non-regulated metabolites were presented on a DM basis in μg kg−1. Descriptive statistics, i.e. frequencies, mean, median and ranges of the concentration of analytes, were calculated considering only the positive results (x ≥ limit of LOD). Results below the LOQ were computed as LOQ/2. All statistical evaluations, tables, and graphs were performed using Microsoft Excel® and GraphPad Prism® version 9.1 (GraphPad Software, San Diego, CA).
Results and discussion
General overview of detected groups of analytes
Analytes of natural origin were categorised into groups: Alternaria, Aspergillus, ergot alkaloids, Fusarium and Penicillium mycotoxins, other fungal species, phytoestrogens and unspecific metabolites, as in previous reports (Szulc et al. Citation2019; Hajnal et al. Citation2020; Penagos-Tabares Citation2021). shows the occurrences and respective average, median, and range concentrations (μg kg−1 DM) of observed secondary metabolites. A total of 107 out of more than 1400 targeted secondary metabolites and pesticides were detected: 78 fungal compounds, three phytoestrogens, 16 pesticides and 10 unspecific metabolites (). The categories of fungal metabolites with the highest number of detected metabolites in this exploratory study were Fusarium spp. (26 metabolites), Penicillium (18), Aspergillus (11), ergot alkaloids (10), Alternaria (9), with fewer metabolites from other fungal genera (4). All the samples contained metabolites derived from Aspergillus, Alternaria, Fusarium and other fungal species, while ergot alkaloids and Penicillium-derived metabolites occurred in 90 and 48% of BSG, respectively. Phytoestrogens were found in 29% of the analysed BSG, and the detection of pesticide residues was ubiquitous (100%). In this study, Fusarium metabolites showed the highest level of diversity, which has been previously observed in other naturally-contaminated samples from Austria and Europe (Reisinger et al. Citation2019; Penagos-Tabares et al. Citation2021, Citation2022). This again suggests the status of Fusarium as the most widespread fungal genus in cereal growing areas and as a significant contributor to mycotoxin contamination in animal feeds (Nesic et al. Citation2014). Additionally, it also corroborates the widespread occurrence of other mycotoxigenic genera (Aspergillus, Alternaria and Penicillium) (Grenier and Oswald Citation2011). Regarding the detected levels, the group of unspecific metabolites showed the highest average concentration (7010 μg kg−1), followed by fungal metabolites (5140 μg kg−1), phytoestrogens (957 μg kg−1) and pesticides (208 μg kg−1). Specifically for the fungal metabolites, the highest average levels were for the category of Penicillium (5600 μg kg−1), followed by Fusarium (2200 μg kg−1), Alternaria (107 μg kg−1), ergot alkaloids (69.5 μg kg−1), Aspergillus (79.6 μg kg−1) and metabolites from other fungi (15.5 μg kg−1) (, ).
Figure 2. (A) Distribution of concentrations and (B) co-contamination grade (detected analytes per sample) of major categories of analytes detected in wet brewery’s spent grains intended for the nutrition of dairy cattle in Austria.
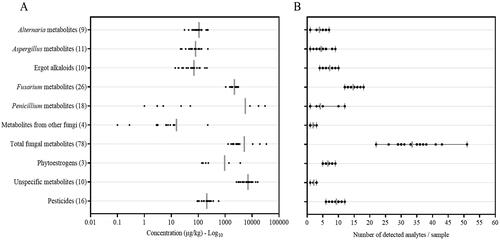
Table 2. Occurrences and levels of fungal and other natural contaminants detected in wet brewery’s spent grains intended for dairy cattle nutrition.
Regulated mycotoxins and related forms
Among the mycotoxins included in the European legislation were detected ZEN, T-2 toxin, and HT-2 toxin, which have recommended GVs (EC Citation2006, 2013). ZEN was the regulated mycotoxins that occurred the most, detected in 57% of the samples with a maximum concentration of 32.3 μg kg−1, whereas T-2 and HT-2 toxins were detected in only one sample (5%), showing concentrations of 3.8 and 4.25 μg kg−1, respectively. The observed concentrations were still under the GVs of the European Union, which are 500 μg kg−1 for ZEN and 250 μg kg−1 for the sum of T-2 toxin and HT-2 toxin relative to a feed with moisture of 12% (EC Citation2002, 2006, 2013). The findings align with a previous report, which detected ZEN contamination in beer (Bauer et al. Citation2016). Other regulated mycotoxins such as AFB1, deoxynivalenol (DON), OTA and FB1 and FB2, which are regulated in European legislation for dairy cattle, were not detected. Regulated mycotoxins are known to cause adverse health effects. For example, ZEN is linked with hyper-estrogenism, reduced milk production, early abortion and other reproductive abnormalities (Hussein and Brasel Citation2001; Marczuk et al. Citation2012). Trichothecenes, like T-2 toxin and HT-2 toxin, are known for inducing inhibition of DNA and RNA synthesis, which can be a secondary effect of the inhibition of protein synthesis or due to apoptosis (Cope Citation2018). Fusarium head blight is a common disease in barley associated with ZEN, type A trichothecenes and other mycotoxins. This disease is a significant threat to the brewing industry, with Fusarium graminearum considered the predominant causal species worldwide (Bai and Shaner Citation2004; Starkey et al. Citation2007; Schwarz Citation2017). Other species like Fusarium culmorum, Fusarium poae and Fusarium avenaceum have also been described as some of the most widely occurring in Europe (Becher et al. Citation2013). F. graminearum and F. culmorum are important producers of ZEN, DON and nivalenol (Bottalico and Perrone Citation2002). F. langsethiae and F. sporotrichioides are producers of HT-2 and T-2 toxins (Thrane et al. Citation2004). It has been suggested that low levels of fusarial mycotoxins like ZEN and type-B trichothecenes (like DON, 15-acetyldeoxynivalenol and 3-acetyldeoxynivalenol) are retained in BSG (Pack et al. Citation2021). Previous studies found that the residual concentrations of several fungal toxins including OTA, AFB2, FB2, AFG1, AFB1, ZEN and patulin decreased to less than 20% during the brewing process (Inoue et al. Citation2011; Piacentini et al. Citation2019). Although T-2 toxin has received special attention in the malting barley chain, due to its occurrence in this cereal crop and its toxic potency, the contamination levels of this toxin generally decrease during the brewing process (Edwards et al. Citation2009; Malachova et al. Citation2010). A study of mycotoxins in BSG in Argentina evidenced FB1 (100%; range: 104–145 μg kg−1) and AFB1 (18%; 19–44.5 μg kg−1), whereas AFB2, AFG1, AFG2 and ZEN were not detected (Pereyra et al. Citation2011).
Regarding ergot alkaloids, which were detected in high occurrence (90%), the European Union recommends the monitoring of these metabolites (Recommendation 2012/154/EC) (EC Citation2012). There is no specific guidance value for ergot alkaloids in animal feeds. Still, the limit of 1000 mg kg−1 rye ergot (Claviceps purpurea) represents a maximum value relative to a feedstuff with a moisture of 12% described in the directive 2002/32/EC (EC Citation2012). Stricter regulation is in place for foodstuffs. Since January 2022, the Commission Regulation (EU) 2021/1399 established a maximum level of ergot alkaloids in certain foodstuffs. For instance, for milling barley products intended for human consumption (with an ash content lower than 9000 mg kg−1), a maximum level of 100 μg kg−1 (50 μg kg−1) will be implemented from 1.7.2024). For milling barley products (with an ash content equal to or higher than 9000 mg kg−1) or barley grains placed on the market for the final consumer, the maximum level set is 150 μg kg−1 (EU Citation2021).
Moreover, our results show that members of the ergopeptine class alone, including ergocryptine, ergosin and ergocristine, presented with occurrences of over 85%. The average concentrations of individual ergot alkaloids were under 30 µg kg−1, and the maximal accumulated concentration of total ergot alkaloids was 210 µg kg−1, which should not be ignored. Feed contaminated with 250 µg kg−1 of ergot alkaloids should not be fed to pregnant or lactating animals due to a higher risk of abortion and agalactia syndrome; even low concentrations of alkaloids in the diet (<100 kg−1 total) can reduce the growth efficiency of livestock (Coufal-Majewski et al. Citation2016). Some members of the ergot alkaloids such as ergotamine, ergocristine, ergosine, ergocornine, ergocryptine and ergovaline are responsible for the majority of nervous or gangrenous syndromes in humans and animals, which consume grains, grain products or grasses contaminated with the sclerotia of the fungus (Gupta et al. Citation2018). Ingestion of this kind of alkaloids by livestock can trigger a range of impacts from decreased performance and reduced fertility to acute clinical signs of ergotism, including nervous or gangrenous syndromes, hyperthermia, convulsions, necrosis of the extremities and death (Evans Citation2011). According to the scientific opinion of EFSA, ergotism in ruminants is usually a chronic disease and the result of continued ingestion of minor quantities of the fungus on grass (EFSA Citation2012). A large proportion of the original peptide alkaloids can be removed during brewing which is believed to result from thermal degradation (Schwarz et al. Citation2007). However, our data confirm that the reduction is not absolute.
Other fungal toxins and metabolites
Emerging mycotoxins are the focus of high scientific interest. They are defined as commonly occurring in feed and foods (agricultural commodities) and are legislatively unregulated and non-regularly tested (Vaclavikova et al. Citation2013). We showed that several emerging and non-regulated mycotoxins and metabolites were detected in samples of BSG intended for cattle feeding on Austrian farms. Several Fusarium-derived emerging mycotoxins include culmorin, siccanol, aurofusarin, beauvericin, bikaverin and enniatins (A, A1, B, B1 and B2), were detected in all the samples. The enniatins B and B1 presented the highest concentrations among the fusarial mycotoxins, with averages exceeding 170 μg kg−1. Enniatins and beauvericin have haematotoxic, immunotoxic and antibiotic activities (Sy-Cordero et al. Citation2012; EFSA Citation2014; Juan et al. Citation2019; Křížová et al. Citation2021). Research on the impact of such fungal antimicrobial compounds on rumen ecology and functionality is essential (Fink-Gremmels Citation2005, Citation2008; Reisinger et al. Citation2019). Siccanol (also called terpestacin) (Chan and Jamison Citation2003) was the fusarial compound with the highest concentration (average: 966 μg kg−1) in BSG. Another fusarial metabolite, fusaric acid occurred with a frequency of 19%. This compound can increase the toxicity of other Fusarium mycotoxins such as moniliformin, trichothecenes and FBs (Bacon et al. Citation1996; D’Mello et al. Citation1999).
Emerging Alternaria mycotoxins, such as alternariol (81%), alternariol methyl ether (86%) and tenuazonic acid (48%) were detected in relatively low concentrations. It is known that these compounds have estrogenic activity and genotoxic effects (Escrivá et al. Citation2017; Aichinger et al. Citation2019, Citation2021). The genus Alternaria is widely distributed in the environment and is one of the leading causes of disease in cereal crops like wheat, barley and sorghum (Deshpande Citation2002). However, information is still missing regarding Alternaria mycotoxins in the feeds and their toxicological repercussions on animal health (EFSA Citation2011). Infectopyrone was found in all the BSG samples and the compound with the highest average and maximum concentration among the Alternaria-derived metabolites. Is a potential mycotoxin whose biological activities are unknown and should be further explored (Andersen et al. Citation2002; Larsen et al. Citation2003). Alternaria-derived compounds like altersetin, altertoxin-I, pyrenophorol and tentoxin were also found. Although at this time, there are no global regulations establishing limits for these toxins in food and feed, the European Food Safety Authority (EFSA) has raised concern about Alternaria mycotoxins in relation to public health (EFSA Citation2011; Escrivá et al. Citation2017). Aspergillus-derived compounds were omnipresent in Austrian BSG evaluated in this study. The most frequently-occurring metabolites from Aspergillus were deoxytryptoquivaline A, tryptoquialanine derivate, flavoglaucin and quinadoline A, detected in frequencies above 70%. Viriditoxin and deoxytryptoquivaline A presented the highest average, median and maximum concentration of Aspergillus-derived metabolites. Other molecules produced by this genus including deoxynortryptoquivalin (33%), fumiquinazolin D (10%), kotanin A (33%), pinselin (43%), pseurotin (5%) and tryptoquivaline A (14%) were also found in BGS (). Viriditoxin, fumiquinazolin D, quinadoline A exhibit antibacterial properties (Qian et al. Citation2019; Urquhart et al. Citation2019; Almeida et al. Citation2021). Tryptoquialanines belong to the group of tremorgenic mycotoxins that can be produced by species of Aspergillus and Penicillium (Clardy et al. Citation1975; Ariza et al. Citation2002).
Strongly linked to postharvest contamination, Penicillium-derived metabolites presented an occurrence of 48%. This category of fungal compounds showed the highest average and maximal concentration (average: 5604 µg kg−1; max: 30,300 µg kg−1). The metabolites verrucofortine (29%) phenopyrrozin (24%) were the more recurrent compounds from penicillia, but their mean concentrations were very low (<10 µg kg−1). The Penicillium-derived metabolites detected in highest concentrations were andrastin C (average: 6,400 µg kg−1, occurrence: 10%), andrastin B (5990 µg kg−1, 10%) and andrastin A (2910 µg kg−1, 19%). These compounds, among other Penicillium-derived substances, are commonly found in silage, with higher concentrations in mouldy hot spots (Reisinger et al. Citation2019; Gallo et al. Citation2021; Penagos-Tabares et al. Citation2022; Manni et al. 2022). The complete spectrum of the biological activities and toxicological effects of the andrastins has not been elucidated. It is known that the andrastins are protein farnesyltransferase inhibitors, which can inhibit the efflux of anticancer drugs from multidrug-resistant cancer cells and are devoid of antimicrobial activity. They are commonly found in European blue (mould) cheeses (Uchida et al. Citation1996). Other compounds detected in this study were mycophenolic acid, and roquefortines, the most investigated Penicillium metabolites in silages (Gallo et al. Citation2015). A common feature of many detected metabolites like mycophenolic acid, roquefortines and patulin, is their immunotoxic properties (Oh et al. Citation2012; Brennan et al. Citation2017), which could interfere with the activity of innate and adaptive immune responses, predisposing to secondary infectious diseases (Oh et al. Citation2015). Phenopyrrozin, along with marcfortines A and C, were also detected in the analysed BSG samples. Several researchers have proposed that Penicillium toxins can induce unspecific clinical signs like appetite reduction, affecting nutrient efficiency and increasing the incidence of abomasal ulcers, laminitis, gastroenteritis, abortion and paralysis (Dzidic et al. Citation2006; Nielsen et al. Citation2006; Fink-Gremmels Citation2008; Alonso et al. Citation2013; Gallo et al. Citation2015). Additionally, further less-known metabolites produced mainly by other fungal species were detected in this study. Rubellin D and sporidesmolide occurred at a frequency of 90%, whereas cyclosporin A and monocerin were detected in only one sample (5%). The quantified levels of these metabolites were below 15 μg kg−1, except for cyclosporin A (222 μg kg−1) (). Rubellin D is an anthraquinone derived from Ramularia collo-cygni with antibacterial activity (Walters et al. Citation2008; Miethbauer et al. Citation2009). Cyclosporin A has a potent immunotoxic activity, which has even been used commercially in human and veterinary medicine as an immunosuppressant (Laupacis et al. Citation1982; Shevach Citation1985; Stähelin Citation1996).
Postharvest infestations with moulds proliferate under aerobic conditions, producing potent toxins, disruptive endocrine substances and antimicrobial compounds. Moreover, fungal growth leads to spoilage, thereby reducing the nutritional value, DM content, intake and palatability (O'Brien et al. Citation2006). The high proportion of moisture in wet BSG makes this product especially susceptible to microbial growth and spoilage in a short period (7–10 d) (Lilly et al. Citation1980; Stojceska and Ainsworth Citation2008; Chanie and FieVez Citation2017). Strategies suggested for preserving wet BSG include drying with solar radiation and ensiling. Drying by solar radiation is found to be challenging due to costs (Conrad and Rogers Citation1977). Alternatively, the ensiling of wet brewery grain alone or mixed with dry fodders is the proposed practice for dairy farmers, especially in developing countries (Kindbom Citation2012; Souza et al. Citation2012). The preservation of wet BSG by lowering the water activity of the material using beet molasses (30%) and further stabilising the mixture by incorporating an anti-mycotic agent (0.3% of potassium sorbate) has been achieved at both laboratory-scale and pilot-scale. For practical preservation, the stabilised grains should be stored under anaerobic conditions in plastic bags, squeezing the air out and sealing tightly (Lilly et al. Citation1980). However, more applied research on preservation strategies for BGS is still required.
Contamination of phytoestrogens and other secondary metabolites
In the present research, three isoflavones were detected in medium-low frequencies: Daidzein (24%), genistein (29%) and glycitein (10%) (). The predominant daidzein and genistein presented average concentrations above 500 μg kg−1 and maximum above 1600 μg kg−1. These metabolites are found primarily in Leguminosae plants, such as soy (Glycine max) but also occur in clovers (Trifolium spp.) and alfalfa/lucerne (Medicago sativa) (Reed Citation2016). Glycitein presented an average concentration of 93.6 μg kg−1, ranging from 52.5 to 135 μg kg−1. Liggins et al. (Citation2002) reported daidzein and genistein in cereal-derived products for human consumption. In pearl barley, only genistein was detected with an average concentration of 86 μg kg−1. The concentration of the two isoflavones in the remaining foods ranged from 33 to 11,873 µg kg−1 (Liggins et al. Citation2002). Coumetrans like coumestrol, which were not found. seem to have a more potent estrogenic activity than the detected isoflavones here (Romero et al. Citation1997). The detected concentration of phytoestrogens (isoflavones) found in our study apparently does not represent a potential risk for cattle (Grgic et al. Citation2021).
In addition, several unspecific secondary metabolites were detected. These analytes can be produced by different and unrelated living systems belonging to diverse kingdoms (Plantae, Fungi, Animalia and/or Eubacteria). Several of the unspecific secondary metabolites detected in our study are biologically active molecules. These compounds could influence the toxicological complexity of the complete cocktails of secondary metabolites evidenced in this investigation. They included, for example, emodin (antibacterial and immunosuppressive) (Kiyoshi et al. Citation1984; Dong et al. Citation2016) as well as the diketopiperazines cyclo-(L-Pro-L-Tyr) (synonym: maculosin) and cyclo-(L-Pro-L-Val) (antibacterial) (Park et al. Citation1993; Rahman et al. Citation2020; Zin et al. Citation2020; Paudel et al. Citation2021). Other detected unspecific metabolites were neoechinulin A, physcion, rugulusovine and tryptophol. The most predominant unspecific metabolites (detected in all the samples) were tryptophol, cyclo-(L-Pro-L-Tyr) and cyclo-(L-Pro-L-Val), which also showed the highest average concentrations of this group (>1900 µg kg−1) ().
Pesticide residues
All the samples presented residues of pesticides, varying from six to twelve different biocides per sample (). No illegal compounds (EU-MRL-Database Citation2022) were detected. In total, 16 pesticides were found: 14 fungicides, one insecticide (pirimiphos-methyl) and an insecticide synergist (piperonyl butoxide) as classified in previous studies () (Huang and Subramanyam Citation2005; Opalski et al. Citation2006; Lamberth et al. Citation2008; Sooväli and Koppel Citation2010; Harp et al. Citation2011; Lazzari et al. Citation2012; Rodrigues et al. Citation2013; Kanungo and Joshi Citation2014; Rumbos et al. Citation2016; EFSA Citation2017, 2018; McLean and Hollaway Citation2019; Xu et al. Citation2020; Basak et al. Citation2021; Yao et al. Citation2021; EU-MRL-Database Citation2022; Rathod et al. Citation2022). Occurrences along with the corresponding average, median and range concentrations (expressed in μg kg−1 DM) of the detected pesticide residues, their respective uses, the maximum residue levels (MRLs) in barley, and the proportion of samples above the respective MRL are presented in . The most frequently detected pesticides in the analysed BSG samples were fluopyram, piperonyl butoxide, fluxapyroxad, bixafen, mandipropamid and tebocunazol, which were seen in ≥85% of the samples. The fungicides azoxystrobin, benzovindiflupyr and boscalid as well as the insecticide pirimiphos-methyl showed occurrences between 43 and 62%. Residues of ametoctradin, isopyrazam, pyraclostrobin and trifloxystrobin were found in less than 40% of the evaluated BGS samples. The pesticides with the highest average levels of residues were piperonyl butoxide (116µg kg−1), metrafenone (30.4 µg kg−1) and fluopyram (24.7 µg kg−1) (). Notably, 9.5% and 14.3% of the samples exceed the respective current MRLs (0.01 mg kg−1) of ametoctradin and mandipropamid for barley. The other pesticides were detected in amounts lower than the MRLs (EU-MRL-Database Citation2022). Piperonyl butoxide occurred frequently and presented the highest levels among the groups of pesticides. Piperonyl butoxide enhances the potency of certain pesticides such as carbamates, pyrethrins and pyrethroids but has no pesticide activity of its own (Basak et al. Citation2021). It has been demonstrated that this insecticide synergist can induce the formation of liver tumours in mice via the constitutive androstane receptor, which is qualitatively not plausible for humans due to the lack of effect on replicative DNA synthesis in human hepatocytes (Lake et al. Citation2020). This insecticide synergist is not a cholinesterase inhibitor and has low toxicity; it is also employed for other purposes than crop protection. It may also be used in conjunction with flea or tick dips, collars and oral medications in farm animals (Keane Citation1999). A widespread use of fluopyram, a pyridinyl ethylbenzamide, applied as a broad-spectrum fungicide with nematicide activity (Becker et al. Citation2020; Rathod et al. Citation2022) was recorded in this study, because it was detected in all the samples. The literature suggests that the high persistence of fluopyram in the environment (soil and water/sediment) can present risks for human, animal and soil health. The fate of this fungicide in diverse soil environments is still to be studied (Rathod et al. Citation2022). Fluopyram is authorised for use on crops that might be fed to livestock (EFSA Citation2020). A feeding study (Schoening and Wolters 2008 Ref: MR-07/367 (unpublished) cited by Lunn (Citation2010) investigated the residue depuration of fluopyram in lactating dairy cattle, finding fluopyram and its metabolites in milk and different tissues. Barley has frequently been found contaminated with traces of fungicides (Palladino et al. Citation2021). In terms of risk, according to the WHO, five of the detected biocides here (benzovindiflupyr, prochloraz [confirmed by its metabolite of BTS 44595], isopyrazam, pirimiphos-methyl and tebuconazole) were classified as moderately hazardous (II) (WHO Citation2020). The last three are included in the list of highly hazardous pesticides (HHP) of the Pesticide Action Network (PAN Citation2021). Isopyrazam was added to the HHP list in 2011, it is considered likely carcinogenic for humans, very persistent in water, soil or sediment and very toxic for aquatic organisms (<50 ng/L) (US EPA Citation2017; Yao et al. Citation2018). Pirimiphos methyl was added to the HHP list in 2009, which is considered highly toxic for bees (Barnett et al. Citation2007; PAN Citation2021). Also, in the HHP list, tebuconazole can induce acute toxicity and long-term effects (PAN 2021) and can have ecotoxicological effects on an aquatic decomposer-detritivore system (Zubrod et al. Citation2011). Other detected pesticides like ametoctradin, fluopyram and fluxapyroxad are classified as slightly hazardous (III). In contrast, azoxystrobin, boscalid, mandipropamid, metrafenone, piperonyl butoxide and trifloxystrobin are grouped as unlikely to present acute hazards (WHO Citation2020). It has been suggested that reduction in pesticide levels during the malting and brewing processes reduced considerably the risks of contaminating beer with pesticides and only a few pesticides remained without being removed or resolved (Navarro et al. Citation2007; Kong et al. Citation2016). Several pesticides found during beer production are adsorbed onto the spent grain after mashing. Moreover, some pesticides are degraded or transformed during boiling and fermentation, indicating that such reduction was caused primarily by adsorption, pyrolysis, and hydrolysis (Inoue et al. Citation2011; Xi et al. Citation2014). In the European Union, pesticide residue levels in particular plant and animal-derived foods and feeds, have been set by the Commission (EC) No 396/2005 (EC Citation2005). Information concerning MRLs and toxicity is available in the EU Pesticide database (EU-MRL-Database Citation2022).
Table 3. Occurrences and levels of pesticide residues detected in wet brewery’s spent grains intended for dairy cattle nutrition.
Co-occurrence of fungal toxins, phytoestrogens and pesticide residues
Bioaccumulation rates and effects of long-term exposure to contaminant mixtures are unpredictable and should be investigated through the feed and food production chain. The co-contamination of several natural and synthetic contaminants was found (). All the samples presented co-contamination with several fungal secondary metabolites, fluctuating from 22 to 51 fungal metabolites per sample; 34 on average. Similarly, a broad spectrum of co-contamination with fungal and other metabolites has been observed in different complex matrices of feedstuffs such as silage, pastures, concentrate feed, and total mix rations (Shimshoni et al. Citation2013; Nichea et al. Citation2015; Kemboi et al. Citation2020; Awapak et al. Citation2021). Interestingly, we generated data concerning pesticide residues, which occurred with an average of nine compounds per sample, ranging from six to twelve (). Additionally, illustrates co-occurrence matrices of mycotoxins (with recurrence over 25%) and phytoestrogens () as well as pesticides (). All the samples evidenced that three fusarial emerging mycotoxins, aurofusarin, beauvericin and enniatins, were detected and co-occurred with ZEN in 57% of the samples. More than 50% of the BSG presented co-contamination with several ergot alkaloids. Combinations between mycoestrogens derived from Alternaria AOH, AME, and TeA with ZEN were 43%, 52% and 33%, respectively. Co-occurrences between the mentioned mycoestrogens and the detected phytoestrogens (daidzein, genistein and glycitein) were lower than 30% (). Concerning pesticide residues, over 60% of the samples presented mixtures of fluopyram, fluxapyroxad, mandipropamid, metrafenone, piperonyl butoxide and tebuconazole. All the samples containing the insecticide for storage pirimiphos-methyl (48%) contained the semisynthetic synergist piperonyl butoxide: both compounds has been recently found in cereal samples from Croatia (Kovač et al. Citation2021).
Figure 3. Heatmaps of the co-occurrence (%) of (A) fungal contaminants (with occurrence rate > 25%) and phytoestrogens as well as (B) of pesticide residues detected in wet brewery’s spent grains intended for the nutrition of dairy cattle in Austria.
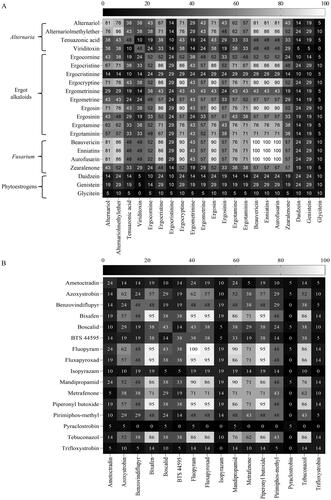
These outcomes evidenced the ubiquitous presence of mixtures of multiple natural and synthetic chemicals in this by-product, linked to the feed and food supply chain. Although the occurrence of several contaminants was high, the concentrations found were low and under the legal limits (GVs and MRLs). The individual concentrations indeed do not represent an acute or critical risk for farm animals and human consumers. However, it is known that the combined effect of several co-occurring toxins and endocrine disruptors may be additive, synergistic or antagonistic, varying by type of compound or/and concentration (Guo et al. Citation2020). Such biological effects of toxin mixtures on animal and human health have been growing notably in recent years, but related knowledge is still overall scarce (Battilani et al. Citation2020; Gil-Serna et al. Citation2014; Smith et al. Citation2016; Weaver et al. Citation2020). Toxicological interactions have been described among mycotoxins, phytoestrogens and pesticides (Hessenberger et al. Citation2017; Vejdovszky, Hahn, et al. Citation2017a, Vejdovszky, Schmidt, et al. Citation2017a,Citationb; Eze et al. Citation2019). For example, it is known that the interaction of diverse kinds of natural and synthetic xenobiotics, such mycotoxins, plant metabolites and chemical biocides, can also shape microbiota composition, which influences the health and metabolic status of the host (Lindell et al. Citation2022). The relevance of the co-occurrence (in real-world situations) of natural and synthetic chemicals has to be addressed by toxicologists (Warne and Hawker Citation1995; Groten et al. Citation2001; Mattsson Citation2007). Nowadays, advances in analytic methods allow for evaluating hundreds of natural and synthetic pollutants, achieving high performances (LOD, LOQ and recovery) (Steiner et al. Citation2020; Sulyok et al. Citation2020; Steiner et al. Citation2021). Multi-toxin and multi-metabolites analysis has been used during the last decade to bring more insights into the complex field of mixture toxicology (Groten et al. Citation2001; Battilani et al. Citation2020; Martin et al. Citation2021). The evidenced ubiquitous presence of mixtures of pesticides suggests an extended application of this kind of substances in barley intended for beer production. This could also indicate that multiple biocides are being incorporated constantly at low levels in the feed and food chain, which can result in negative ecological and toxicological consequences (Mishra et al. Citation2014; Rivera-Becerril et al. Citation2017; Vanbergen Citation2021; Panico et al. Citation2022). Pesticide interactions lead mainly to synergic effects. Mixture effects differ depending on the dose and/or physiological target. Thus, more research and data for this important and exciting field are still required (Rizzati et al. Citation2016).
Conclusion
This study provides insights into the widespread occurrence of cocktails of mycotoxins, phytoestrogens and pesticides in wet BSG. Mycotoxins/metabolites produced by the genera Fusarium, Aspergillus, and Alternaria were detected in all the samples. Ergot alkaloids were also frequently found (90%). Penicillium secondary metabolites, associated primarily with storage contamination, were present in 48% of the samples and showed the highest average concentration among the groups of fungal compounds. The storage-associated contamination leads to the necessity to improve strategies for preserving wet BSG in the farms. Additionally, we demonstrate the ubiquitous co-occurrence of several pesticide residues (at least six per sample, primarily fungicides). Two of them (ametoctradin and mandipropamid) exceeded the EU MRLs. Some pesticides (azoxystrobin, bixafen, fluopyram, fluxapyroxad, mandipropamid, metrafenone, piperonyl butoxide and tebuconazole) showed high occurrences (>60%), which could suggest a common and extended use on food/feed crops of the mentioned pesticides and incorporation of these biocides into the feed/food chain and into the agroecosystems. Although the vast majority (88%) of the detected pesticides presented low concentrations, the potential combined effects of such biocide mixtures and natural toxins are unpredictable and should be subject to future studies. Further investigations with a larger number of samples and evaluation of BSG together with other feeds/foods is highly advocated.
Abbreviations | ||
BSG | = | Spent brewery grains |
ZEN | = | Zearalenone |
DM | = | Dry matter |
OTA | = | Ochratoxin A |
AFs | = | Aflatoxins |
DON | = | Deoxynivalenol |
EDCs | = | Endocrine-disrupting chemicals |
ZAMG | = | Agency of Meteorology and Geodynamics, Zentralanstalt für Meteorologie und Geodynamik |
LOD | = | Limit of detection |
LOQ | = | Limit of quantification |
Supplemental Material
Download TIFF Image (19.1 MB)Acknowledgments
The authors appreciate enormously the excellent technical support and cooperation provided by Dr. Manfred Hollmann, Anita Dockner, Sabine Leiner (Institute of Animal Nutrition and Functional Compounds, Vetmeduni, Vienna) for the excellent technical assistance during the sample preparation.
Disclosure statement
N.V. and J.F. are employed by BIOMIN Holding GmbH, part of DSM Animal Nutrition and Health, which operates the BIOMIN Research Center and produces animal feed additives. This, however, did not influence sampling, analyses or interpretation of data.
Additional information
Funding
References
- Aichinger G, Del Favero G, Warth B, Marko D. 2021. Alternaria toxins—Still emerging? Compr Rev Food Sci Food Saf. 20(5):4390–4406. doi:10.1111/1541-4337.12803
- Aichinger G, Krüger F, Puntscher H, Preindl K, Warth B, Marko D. 2019. Naturally occurring mixtures of Alternaria toxins: anti-estrogenic and genotoxic effects in vitro. Arch Toxicol. 93(10):3021–3031. doi:10.1007/s00204-019-02545-z
- Almeida MC, Resende DI, da Costa PM, Pinto M, Sousa E. 2021. Fumiquinazoline related alkaloids: synthesis and evaluation of their antibacterial activities. Proceedings of the 7th International Electronic Conference on Medicinal Chemistry; 2021 November 1–30; Basel, Switzerland: MDPI. doi:10.3390/ECMC2021-11474
- Alonso VA, Pereyra CM, Keller LAM, Dalcero AM, Rosa CAR, Chiacchiera SM, Cavaglieri LR. 2013. Fungi and mycotoxins in silage: an overview. J Appl Microbiol. 115(3):637–643. doi:10.1111/jam.12178
- Ambra R, Pastore G, Lucchetti S. 2021. The role of bioactive phenolic compounds on the impact of beer on health. Molecules. 26(2):486. doi:10.3390/molecules26020486
- Andersen B, Krøger E, Roberts RG. 2002. Chemical and morphological segregation of Alternaria arborescens, A. infectoria and A. tenuissima species-groups. Mycol Res. 106(2):170–182. doi:10.1017/S0953756201005263
- Ariza MR, Larsen TO, Peterson BO, Duus JO, Barrero AF. 2002. Penicillium digitatum metabolites on synthetic media and citrus fruits. J Agric Food Chem. 50(22):6361–6365. doi:10.1021/jf020398d
- Awapak D, Petchkongkaew A, Sulyok M, Krska R. 2021. Co-occurrence and toxicological relevance of secondary metabolites in dairy cow feed from Thailand. Food Addit Contam Part A Chem Anal Control Expo Risk Assess. 38(6):1013–1027. doi:10.1080/19440049.2021.1905186
- Bacon C, Porter J, Norred W, Leslie J. 1996. Production of fusaric acid by Fusarium species. Appl Environ Microbiol. 62(11):4039–4043. doi:10.1128/aem.62.11.4039-4043.1996
- Bai G, Shaner G. 2004. Management and resistance in wheat and barley to Fusarium head blight. Annu Rev Phytopathol. 42:135–161. doi:10.1146/annurev.phyto.42.040803.140340
- Barnett EA, Charlton AJ, Fletcher MR. 2007. Incidents of bee poisoning with pesticides in the United Kingdom, 1994–2003. Pest Manag Sci. 63(11):1051–1057. doi:10.1002/ps.1444
- Basak M, Ahmed Choudhury R, Goswami P, Kumar Dey B, Ahmed Laskar M. 2021. A review on non-target toxicity of deltamethrin and piperonyl butoxide: aynergist. J Pharm Res Int. 33(51B):85–89.
- Battilani P, Palumbo R, Giorni P, Dall’Asta C, Dellafiora L, Gkrillas A, Toscano P, Crisci A, Brera C, De Santis B. 2020. Mycotoxin mixtures in food and feed: holistic, innovative, flexible risk assessment modelling approach: MYCHIF. EFSA Support. 17:1757E.
- Bauer JI, Gross M, Gottschalk C, Usleber E. 2016. Investigations on the occurrence of mycotoxins in beer. Food Control. 63:135–139. doi:10.1016/j.foodcont.2015.11.040
- Becher R, Miedaner T, Wirsel SGR. 2013. Biology, diversity and management of FHB-causing Fusarium species in small-grain cereals. In: Seiten, Kempken F, editor. The mycota. XI. Agricultural applications. 2nd ed. Berlin, Germany: Springer-Verlag; p. 199–241.
- Becker JO, Loffredo A, Edwards S, Becker Smith J, Ploeg A. 2020. Seed-delivered fluopyram mitigates root-knot nematode damage in fresh market carrot field trials. Poster session presented at: virtual SON 2020. [accessed 2022 June 11]. doi:10.6084/m9.figshare.13356596.v1.
- Bianco A, Budroni M, Zara S, Mannazzu I, Fancello F, Zara G. 2020. The role of microorganisms on biotransformation of brewers’ spent grain. Appl Microbiol Biotechnol. 104(20):8661–8678. doi:10.1007/s00253-020-10843-1
- Bocquier F, González-García E. 2010. Sustainability of ruminant agriculture in the new context: feeding strategies and features of animal adaptability into the necessary holistic approach. Animal. 4(7):1258–1273. doi:10.1017/S1751731110001023
- Bottalico A, Perrone G. 2002. Toxigenic Fusarium species and mycotoxins associated with head blight in small-grain cereals in Europe. Eur J Plant Pathol. 108(7):611–624. doi:10.1023/A:1020635214971
- Brennan KM, Oh SY, Yiannikouris A, Graugnard DE, Karrow NA. 2017. Differential gene expression analysis of bovine macrophages after exposure to the Penicillium mycotoxins citrinin and/or ochratoxin A. Toxins. 9(11):366. doi:10.3390/toxins9110366
- Chan J, Jamison TF. 2003. Synthesis of (−)-terpestacin via catalytic, stereoselective fragment coupling: Siccanol is terpestacin, not 11-epi-terpestacin. J Am Chem Soc. 125(38):11514–11515. doi:10.1021/ja0373925
- Chanie D, FieVez V. 2017. Review on preservation and utilization of wet brewery spent grain as concentrate replacement feed for lactating dairy cows. J Anim Health Prod. 5(1):10–13.
- Cinar A, Onbaşı E. 2019. Mycotoxins: the hidden danger in foods. In: Sabuncuoğlu S, editor. Mycotoxins and food safety. London: IntechOpen; p. 2020.
- Clardy J, Springer JP, Buchi G, Matsuo K, Wightman R. 1975. Tryptoquivaline and tryptoquivalone, two tremorgenic metabolites of Aspergillus clavatus. J Am Chem Soc. 97(3):663–665. doi:10.1021/ja00836a045
- Connolly L. 2009. Endocrine-disrupting chemicals: origins, fates and transmission into the food chain. In: Shaw I, editor. Endocrine disrupting chemicals in food. Cambridge: Woodhead Publishing Limited; p. 103–125.
- Conrad HR, Rogers JA. 1977. Comparative value of brewers’ wet and brewers’ dried grains for dairy cattle. St. Louis (MO): US Brewers Association Feed Conference; p. 26–33.
- Cope RB. 2018. Trichothecenes. In: Gupta RC, editor. Veterinary toxicology. 3rd ed. Cambridge (MA): Academic Press; p. 1043–1053.
- Coufal-Majewski S, Stanford K, McAllister T, Blakley B, McKinnon J, Chaves AV, Wang Y. 2016. Impacts of cereal ergot in food animal production. Front Vet Sci. 25(3):15.
- Cozma P, Apostol LC, Hlihor RM, Simion IM, Gavrilescu M. 2017. Overview of human health hazards posed by pesticides in plant products. Proceedings of 2017 E-Health and Bioengineering Conference (EHB). Sinaia, Romania; June 22–24; Piscataway (NJ): IEEE. doi:10.1109/EHB.2017.7995419
- D’Mello JPF, Placinta CM, Macdonald AMC. 1999. Fusarium mycotoxins: a review of global implications for animal health, welfare, and productivity. Anim Feed Sci Technol. 80(3–4):183–205. doi:10.1016/S0377-8401(99)00059-0
- Damalas CA, Eleftherohorinos IG. 2011. Pesticide exposure, safety issues, and risk assessment indicators. Int J Environ Res. 8(5):1402–1419.
- Deshpande S. 2002. Handbook of food toxicology. NY, USA: CRC Press.
- Dong X, Fu J, Yin X, Cao S, Li X, Lin L, Ni J. 2016. Emodin: a review of its pharmacology, toxicity and pharmacokinetics. Phytother Res. 30(8):1207–1218. doi:10.1002/ptr.5631
- Dreinert A, Wolf A, Mentzel T, Meunier B, Fehr M. 2018. The cytochrome bc1 complex inhibitor Ametoctradin has an unusual binding mode. Biochim Biophys Acta Bioenerg. 1859(8):567–576. doi:10.1016/j.bbabio.2018.04.008. 29704498
- Dzidic A, Prgomet C, Mohr A, Meyer K, Bauer J, Meyer HHD, Pfaffl MW. 2006. Effects of mycophenolic acid on inosine monophosphate dehydrogenase I and II mRNA expression in white blood cells and various tissues in sheep. J Vet Med Series A. 53(4):163–169. doi:10.1111/j.1439-0442.2006.00809.x
- [EC] European Commission. 2002. Directive 2002/32/EC of the European Parliament and of the Council of 7 May 2002 on undesirable substances in animal feed. O J Eu L. 140:10–21.
- [EC] European Commission. 2005. Commission regulation (EC). 2005. No 396/2005 of the European parliament and of the council, maximum residue levels of pesticides in or on food and feed of plant and animal origin. O J Eu L. 70:1–16.
- [EC] European Commission. 2006. Commission recommendation of 17 August 2006 on the presence of deoxynivalenol, zearalenone, ochratoxin A, T-2 and HT-2 and fumonisins in products intended for animal feeding (2006/576/EC). O J Eu L. 229:7–9.
- [EC] European Commission. 2012. Commission recommendation 2012/154/EU of 15 March 2012 on the monitoring of the presence of ergot alkaloids in feed and food. O J Eu L. 77:20–21.
- [EC] European Commission. 2013. Commission recommendation of 27 March 2013 on the presence of T-2 and HT-2 toxin in cereals and cereal products (2013/165/EU). O J Eu L. 91:12–15.
- Edwards S, Barrier-Guillot B, Clasen PE, Hietaniemi V, Pettersson H. 2009. Emerging issues of HT-2 and T-2 toxins in European cereal production. WMJ. 2(2):173–179. doi:10.3920/WMJ2008.1126
- [EFSA] European Food Safety Authority, Anastassiadou M, Bernasconi G, Brancato A, Carrasco Cabrera L, Greco L, Jarrah S, Kazocina A, Leuschner R, Magrans JO. 2020. Review of the existing maximum residue levels for fluopyram according to Article 12 of Regulation (EC) No 396/2005. EFSA J. 18(4):6059. [accessed 2022 June 11]. doi:10.2903/j.efsa.2020.6059.
- [EFSA] European Food Safety Authority, Arena M, Auteri D, Barmaz S, Bellisai G, Brancato A, Brocca D, Bura L, Byers H, Chiusolo A. 2017. Peer review of the pesticide risk assessment of the active substance trifloxystrobin. EFSA J. 15(10):4989.
- [EFSA] European Food Safety Authority, Brancato A, Brocca DLC, Cabrera C, De Lentdecker L, Ferreira L, Greco J, Janossy S, Jarrah D, Kardassi R, Leuschner C. 2018. Modification of the existing maximum residue levels for prochloraz in various commodities. EFSA J. 16(4):5241.
- [EFSA] European Food Safety Authority. 2011. Scientific opinion on the risks for animal and public health related to the presence of Alternaria toxins in feed and food. EFSA J. 9(10):1–97.
- [EFSA] European Food Safety Authority. 2012. Scientific Opinion on Ergot alkaloids in food and feed. EFSA J. 10(7):2798.
- [EFSA] European Food Safety Authority. 2014. Scientific opinion on the risks to human and animal health related to the presence of beauvericin and enniatins in food and feed. EFSA J. 12(8):1–174.
- Ertl P, Zebeli Q, Zollitsch W, Knaus W. 2015. Feeding of by-products completely replaced cereals and pulses in dairy cows and enhanced edible feed conversion ratio. J Dairy Sci. 98(2):1225–1233. doi:10.3168/jds.2014-8810
- Escrivá L, Oueslati S, Font G, Manyes L. 2017. Alternaria mycotoxins in food and feed: An overview. J. Food Qual. 2017:1–20. doi:10.1155/2017/1569748
- [EU] European Union. 2021. Commission Regulation (EU) 2021/1399 of 24 August 2021 amending Regulation (EC) No 1881/2006 as regards maximum levels of ergot sclerotia and ergot alkaloids in certain foodstuffs. Off J European Union. 301:1–5.
- [EU-MRL-Database]. 2022. European union maximum residue limit; [accessed 2022 June 11]. https://ec.europa.eu/food/plants/pesticides/eu-pesticides-database_en.
- Evans TJ. 2011. Diminished reproductive performance and selected toxicants in forages and grains. Vet Clin North Am Food Anim Pract. 27(2):345–371. doi:10.1016/j.cvfa.2011.03.001
- Eze UA, Huntriss J, Routledge MN, Gong YY, Connolly L. 2019. The effect of individual and mixtures of mycotoxins and persistent organochloride pesticides on oestrogen receptor transcriptional activation using in vitro reporter gene assays. Food Chem Toxicol. 130:68–78. doi:10.1016/j.fct.2019.05.014
- [FAO and WHO] Food and Agriculture Organization of the United Nations and World Health Organization. 2019. Hazards Associated with Animal Feed: Joint FAO/WHO Expert Meeting FAO Headquarters; Rome, Italy; 12–15 May 2015. FAO animal production and health/report No. 14. Geneva, Switzerland: World Health Organization. [accessed 2022 June 11]. https://www.fao.org/3/ca6825en/CA6825EN.pdf.
- Fink-Gremmels J. 2005. Mycotoxins in forages. In: Diaz DE, editor. The mycotoxin blue book. Nottingham: Nottingham University Press; p. 249–268.
- Fink-Gremmels J. 2008. The role of mycotoxins in the health and performance of dairy cows. Vet J. 176(1):84–92. doi:10.1016/j.tvjl.2007.12.034
- Gallo A, Ghilardelli F, Atzori AS, Zara S, Novak B, Faas J, Fancello F. 2021. Co-occurrence of regulated and emerging mycotoxins in corn silage: relationships with fermentation quality and bacterial communities. Toxins. 13(3):232. doi:10.3390/toxins13030232
- Gallo A, Giuberti G, Frisvad JC, Bertuzzi T, Nielsen KF. 2015. Review on mycotoxin issues in ruminants: occurrence in forages, effects of mycotoxin ingestion on health status and animal performance and practical strategies to counteract their negative effects. Toxins. 7(8):3057–3111. doi:10.3390/toxins7083057
- Geissen V, Silva V, Lwanga EH, Beriot N, Oostindie K, Bin Z, Pyne E, Busink S, Zomer P, Mol H, et al. 2021. Cocktails of pesticide residues in conventional and organic farming systems in Europe–Legacy of the past and turning point for the future. Environ Pollut. 278:116827. doi:10.1016/j.envpol.2021.116827
- Gil-Serna J, Vázquez C, Gonzaléz-Jaén MT, Patiño B. 2014. Mycotoxins: Toxicology. In: Batt C, Tortorello ML, editors. Encyclopedia of Food Microbiology. 2nd ed. St. Louis, MO, USA: Elsevier. p. 887–892.
- Gonzalez Pereyra M, Rosa C, Dalcero A, Cavaglieri L. 2011. Mycobiota and mycotoxins in malted barley and brewer’s spent grain from Argentinean breweries. Lett Appl Microbiol. 53(6):649–655. doi:10.1111/j.1472-765X.2011.03157.x
- Grenier B, Oswald I. 2011. Mycotoxin co-contamination of food and feed: meta-analysis of publications describing toxicological interactions. World Mycotoxin J. 4(3):285–313. doi:10.3920/WMJ2011.1281
- Grgic D, Varga E, Novak B, Müller A, Marko D. 2021. Isoflavones in animals: metabolism and effects in livestock and occurrence in feed. Toxins. 13(12):836. doi:10.3390/toxins13120836
- Groten JP, Feron VJ, Sühnel J. 2001. Toxicology of simple and complex mixtures. Trends Pharmacol Sci. 22(6):316–322. doi:10.1016/s0165-6147(00)01720-x
- Guo H, Ji J, Wang J-S, Sun X. 2020. Co-contamination and interaction of fungal toxins and other environmental toxins. Trends Food Sci Technol. 103:162–178. doi:10.1016/j.tifs.2020.06.021
- Gupta RC, Evans TJ, Nicholson SS. 2018. Ergot and fescue toxicoses. In: Gupta RC, editor. Veterinary toxicology. Amsterdam, The Netherlands: Elsevier; p. 995–1001.
- Hajnal EJ, Kos J, Malachová A, Steiner D, Stranska M, Krska R, Sulyok M. 2020. Mycotoxins in maize harvested in Serbia in the period 2012–2015. Part 2: non-regulated mycotoxins and other fungal metabolites. Food Chem. 317:126409. doi:10.1016/j.foodchem.2020.126409
- Harp T, Godwin J, Scalliet G, Walter H, Stalker A, Bartlett D, Ranner D. 2011. Isopyrazam, a new generation cereal fungicide. Asp Appl Biol. 106:113–120.
- Hessenberger S, Botzi K, Degrassi C, Kovalsky P, Schwab C, Schatzmayr D, Schatzmayr G, Fink-Gremmels J. 2017. Interactions between plant-derived oestrogenic substances and the mycoestrogen zearalenone in a bioassay with MCF-7 cells. Pol J Vet Sci. 20(3):513–520. doi:10.1515/pjvs-2017-0062
- Huang F, Subramanyam B. 2005. Management of five stored-product insects in wheat with pirimiphos-methyl and pirimiphos-methyl plus synergized pyrethrins. Pest Manag Sci. 61(4):356–362. doi:10.1002/ps.968
- Hussein HS, Brasel JM. 2001. Toxicity, metabolism, and impact of mycotoxins on humans and animals. Toxicology. 167(2):101–134. doi:10.1016/s0300-483x(01)00471-1
- Igbedioh S. 1991. Effects of agricultural pesticides on humans, animals, and higher plants in developing countries. Arch Environ Health. 46(4):218–224. doi:10.1080/00039896.1991.9937452
- Inoue T, Nagatomi Y, Suga K, Uyama A, Mochizuki N. 2011. Fate of pesticides during beer brewing. J Agric Food Chem. 59(8):3857–3868. doi:10.1021/jf104421q
- José C, Prinsen P, Gutiérrez A. 2013. Chemical composition of lipids in brewer’s spent grain: a promising source of valuable phytochemicals. J Cereal Sci. 58(2):248–254. doi:10.1016/j.jcs.2013.07.001
- Juan C, Oueslati S, Manes J, Berrada H. 2019. Multimycotoxin determination in tunisian farm animal feed. J Food Sci. 84(12):3885–3893. doi:10.1111/1750-3841.14948
- Kamboh AA. 2017. Review on preservation and utilization of wet brewery spent grain as concentrate replacement feed for lactating dairy cows. J Anim Health Prod. 5(1):10–13.
- Kanungo M, Joshi J. 2014. Impact of pyraclostrobin (F-500) on crop plants. Plant Sci Today. 1(3):174–178. doi:10.14719/pst.2014.1.3.60
- Keane P. 1999. The use of piperonyl butoxide in formulations for the control of pests of humans, domestic pets, and food animals. In: Jones DG, editor. Piperonyl butoxide. The insect synergist. London: Academic Press; p. 289–300.
- Kemboi DC, Ochieng PE, Antonissen G, Croubels S, Scippo M-L, Okoth S, Kangethe EK, Faas J, Doupovec B, Lindahl JF, et al. 2020. Multi-mycotoxin occurrence in dairy cattle and poultry feeds and feed ingredients from Machakos Town, Kenya. Toxins. 12(12):762. doi:10.3390/toxins12120762
- Kindbom S. 2012. Ensiling wet brewer’s waste in peri-urban areas of Kampala. Uganda: Degree project/Swedish University of Agricultural Sciences, Department of Animal Nutrition and Management.
- Kiyoshi K, Taketoshi K, Hideki M, Jiro K, Yoshinori N. 1984. A comparative study on cytotoxicities and biochemical properties of anthraquinone mycotoxins emodin and skyrin from Penicillium islandicum Sopp. Toxicol Lett. 20(2):155–160. doi:10.1016/0378-4274(84)90141-3
- Kong Z, Li M, Chen J, Gui Y, Bao Y, Fan B, Jian Q, Francis F, Dai X. 2016. Behavior of field-applied triadimefon, malathion, dichlorvos, and their main metabolites during barley storage and beer processing. Food Chem. 211:679–686. doi:10.1016/j.foodchem.2016.05.058
- Kovač M, Bulaić M, Jakovljević J, Nevistić A, Rot T, Kovač T, Dodlek Šarkanj I, Šarkanj B. 2021. Mycotoxins, pesticide residues, and heavy metals analysis of Croatian cereals. Microorganisms. 9(2):216. doi:10.3390/microorganisms9020216
- Křížová L, Dadáková K, Dvořáčková M, Kašparovský T. 2021. Feedborne mycotoxins beauvericin and enniatins and livestock animals. Toxins. 13(1):32. doi:10.3390/toxins13010032
- Lake BG, Price RJ, Scott MP, Chatham LR, Vardy A, Osimitz TG. 2020. Piperonyl butoxide: mode of action analysis for mouse liver tumour formation and human relevance. Toxicology. 439:152465. doi:10.1016/j.tox.2020.152465
- Lamberth C, Jeanguenat A, Cederbaum F, De Mesmaeker A, Zeller M, Kempf H-J, Zeun R. 2008. Multicomponent reactions in fungicide research: the discovery of mandipropamid. Bioorg Med Chem. 16(3):1531–1545. doi:10.1016/j.bmc.2007.10.019
- Lao EJ, Dimoso N, Raymond J, Mbega ER. 2020. The prebiotic potential of brewers’ spent grain on livestock’s health: a review. Trop Anim Health Prod. 52(2):461–472. doi:10.1007/s11250-019-02120-9
- Larsen TO, Perry NB, Andersen B. 2003. Infectopyrone, a potential mycotoxin from Alternaria infectoria. Tetrahedron Lett. 44(24):4511–4513. doi:10.1016/S0040-4039(03)01018-9
- Laupacis A, Keown P, Ulan R, McKenzie N, Stiller C. 1982. Cyclosporin A: a powerful immunosuppressant. Can Med Assoc J. 126(9):1041–1046.
- Lazzari V, Arcangeli G, Boebel A, Gualco A, Lazzati S, Risi C, Cantoni A. 2012. Bixafen: a new fungicide active substance effective against foliar diseases of wheat and barley. Proceedings in Giornate Fitopatologiche 2012Milano; Marittima (RA); Marz 13–16; Bologna, Italy: Università di Bologna; p. 213–218.
- Liggins J, Mulligan A, Runswick S, Bingham S. 2002. Daidzein and genistein content of cereals. Eur J Clin Nutr. 56(10):961–966. doi:10.1038/sj.ejcn.1601419
- Lilly V, Birch M, Garscadden B. 1980. The preservation of spent brewers’ grains by the application of intermediate moisture food technology. J Sci Food Agric. 31(10):1059–1065. doi:10.1002/jsfa.2740311014
- Lindell AE, Zimmermann-Kogadeeva M, Patil KR. 2022. Multimodal interactions of drugs, natural compounds, and pollutants with the gut microbiota. Nat Rev Microbiol. 20(7):431–443. doi:10.1038/s41579-022-00681-5
- Lunn D. 2010. Fluopyram (243) the first draft was prepared by Mr David Lunn. Wellington, New Zealand: New Zealand Food Safety Authority; p. 1415. https://www.fao.org/fileadmin/templates/agphome/documents/Pests_Pesticides/JMPR/Evaluation12/Fluopyram.pdf.
- Lynch KM, Steffen EJ, Arendt EK. 2016. Brewers’ spent grain: a review with an emphasis on food and health. J Inst Brew. 122(4):553–568. doi:10.1002/jib.363
- Malachova A, Cerkal R, Ehrenbergerova J, Dzuman Z, Vaculova K, Hajslova J. 2010. Fusarium mycotoxins in various barley cultivars and their transfer into malt. J Sci Food Agric. 90(14):2495–2505. doi:10.1002/jsfa.4112
- Manni K, Rämö S, Franco M, Rinne M, Huuskonen A. 2022. Occurrence of mycotoxins in grass and whole-crop cereal silages—a farm survey. Agriculture. 12(3):398. doi:10.3390/agriculture12030398
- Marczuk J, Obremski K, Lutnicki K, Gajecka M, Gajecki M. 2012. Zearalenone and deoxynivalenol mycotoxicosis in dairy cattle herds. Pol J Vet Sci. 15(2):365–372. doi:10.2478/v10181-012-0055-x
- Martin O, Scholze M, Ermler S, McPhie J, Bopp SK, Kienzler A, Parissis N, Kortenkamp A. 2021. Ten years of research on synergisms and antagonisms in chemical mixtures: a systematic review and quantitative reappraisal of mixture studies. Environ Int. 146:106206. doi:10.1016/j.envint.2020.106206
- Mattsson JL. 2007. Mixtures in the real world: the importance of plant self-defense toxicants, mycotoxins, and the human diet. Toxicol Appl Pharmacol. 223(2):125–132. doi:10.1016/j.taap.2006.12.024
- McLean MS, Hollaway GJ. 2019. Control of net form of net blotch in barley from seed-and foliar-applied fungicides. Crop Pasture Sci. 70(1):55–60. doi:10.1071/CP18142
- Miethbauer S, Gaube F, Möllmann U, Dahse HM, Schmidtke M, Gareis M, Pickhardt M, Liebermann B. 2009. Antimicrobial, antiproliferative, cytotoxic, and tau inhibitory activity of rubellins and caeruleoramularin produced by the phytopathogenic fungus Ramularia collo-cygni. Planta Med. 75(14):1523–1525. doi:10.1055/s-0029-1185835
- Mishra P, Sharma A, Sharma D. 2014. A study on harmful effects of pesticide residue in vegetables. Int J Recent Res Rev. 7(1):45–48.
- Mussatto SI. 2014. Brewer’s spent grain: a valuable feedstock for industrial applications. J Sci Food Agric. 94(7):1264–1275. doi:10.1002/jsfa.6486
- Navarro S, Perez G, Navarro G, Vela N. 2007. Decline of pesticide residues from barley to malt. Food Addit Contam. 24(8):851–859. doi:10.1080/02652030701245189
- Nesic K, Ivanovic S, Nesic V. 2014. Fusarial toxins: secondary metabolites of Fusarium fungi. Rev Environ Contam Toxicol. 228:101–120. doi:10.1007/978-3-319-01619-1_5
- Nichea MJ, Palacios SA, Chiacchiera SM, Sulyok M, Krska R, Chulze SN, Torres AM, Ramirez ML. 2015. Presence of multiple mycotoxins and other fungal metabolites in native grasses from a wetland ecosystem in Argentina intended for grazing cattle. Toxins 7(8):3309–3329. doi:10.3390/toxins7083309
- Nielsen KF, Sumarah MW, Frisvad JC, Miller JD. 2006. Production of metabolites from the Penicillium roqueforti complex. J Agric Food Chem. 54(10):3756–3763. doi:10.1021/jf060114f
- O’Brien M, Nielsen KF, O’Kiely P, Forristal PD, Fuller HT, Frisvad JC. 2006. Mycotoxins and other secondary metabolites produced in vitro by Penicillium paneum Frisvad and Penicillium roqueforti Thom isolated from baled grass silage in Ireland. J Agric Food Chem. 54(24):9268–9276. doi:10.1021/jf0621018
- Oh SY, Fisher RE, Swamy HVLN, Boermans HJ, Yiannikouris A, Karrow NA. 2015. Silage Penicillium mycotoxins: hidden modulators of the immune system. In: Rios C, editor. Mycotoxins: occurrence, toxicology and management strategies. New York (NY): Nova Science Publishers Inc.; p. 1–40.
- Oh SY, Boermans HJ, Swamy HVLN, Sharma BS, Karrow NA. 2012. Immunotoxicity of Penicillium mycotoxins on viability and proliferation of bovine macrophage cell line (BOMACs). TOMYCJ. 6(1):11–16. doi:10.2174/1874437001206010011
- Opalski KS, Tresch S, Kogel KH, Grossmann K, Köhle H, Hückelhoven R. 2006. Metrafenone: studies on the mode of action of a novel cereal powdery mildew fungicide. Pest Manag Sci. 62(5):393–401. doi:10.1002/ps.1176
- Pack ED, Meyerhoff K, Schmale DG. III 2021. Tracking zearalenone and type-b trichothecene mycotoxins in the commercial production of beer and brewers’ spent grains. J Am Soc Brew Chem. 80(2):180–189. doi:10.1080/03610470.2021.1938489
- Palladino C, Puigvert F, Muela A, Taborda B, Pérez CA, Pérez-Parada A, Pareja L. 2021. Evaluation of Fusarium mycotoxins and fungicide residues in barley grain produced in Uruguay. J Agric Food Res. 3:100092. doi:10.1016/j.jafr.2020.100092
- Palmer GH. 2018. Barley and malt. In: Stewart GG, Anstruther A, Russell I, editors. Handbook of brewing. 3rd ed. Boca Raton (FL): CRC Press; p. 107–128.
- [PAN] Pesticide Action Network. 2021. PAN international list of highly hazardous pesticides (PAN List of HHPs). [accessed 2022 June 11]. https://pan-international.org/wp-content/uploads/PAN_HHP_List.pdf.
- Panico SC, van Gestel CA, Verweij RA, Rault M, Bertrand C, Barriga CAM, Coeurdassier M, Fritsch C, Gimbert F, Pelosi C. 2022. Field mixtures of currently used pesticides in agricultural soil pose a risk to soil invertebrates. Environ Pollut. 305:119290. doi:10.1016/j.envpol.2022.119290
- Park SH, Stierle A, Strobel GA. 1993. Metabolism of maculosin, a host-specific phytotoxin produced by Alternaria alternata on spotted knapweed (Centaurea maculosa). Phytochemistry. 35(1):101–106. doi:10.1016/S0031-9422(00)90516-8
- Pascari X, Ramos AJ, Marín S, Sanchís V. 2018. Mycotoxins and beer. Impact of beer production process on mycotoxin contamination. A review. Food Res Int. 103:121–129. doi:10.1016/j.foodres.2017.07.038
- Paudel B, Maharjan R, Rajbhandari P, Aryal N, Aziz S, Bhattarai K, Baral B, Malla R, Bhattarai HD. 2021. Maculosin, a non-toxic antioxidant compound isolated from Streptomyces sp. KTM18. Pharm Biol. 59(1):933–936.
- Penagos-Tabares F, Khiaosa-Ard R, Schmidt M, Pacífico C, Faas J, Jenkins T, Nagl V, Sulyok M, Labuda R, Zebeli Q. 2022. Fungal species and mycotoxins in mouldy spots of grass and maize silages in Austria. Mycotoxin Res. 38(2):117–136. doi:10.1007/s12550-022-00453-3
- Penagos-Tabares FK, Khiaosa-Ard R, Nagl V, Faas J, Jenkins T, Sulyok M, Zebeli Q. 2021. Mycotoxins, phytoestrogens, and other secondary metabolites in Austrian pastures: occurrences, contamination levels, and implications of geo-climatic factors. Toxins. 13(7):460. doi:10.3390/toxins13070460
- Pereyra ML, Rosa CAR, Dalcero AM, Cavaglieri LR. 2011. Mycobiota and mycotoxins in malted barley and brewer’s spent grain from Argentinean breweries. Lett Appl Microbiol. 53(6):649–655. doi:10.1111/j.1472-765X.2011.03157.x
- Petit G, Korbel E, Jury V, Aider M, Rousselière S, Audebrand LK, Turgeon SL, Mikhaylin S. 2020. Environmental evaluation of new brewer’s spent grain preservation pathways for further valorization in human nutrition. ACS Sustainable Chem Eng. 8(47):17335–17344. doi:10.1021/acssuschemeng.0c04236
- Piacentini KC, Běláková S, Benešová K, Pernica M, Savi GD, Rocha LO, Hartman I, Čáslavský J, Corrêa B. 2019. Fusarium mycotoxins stability during the malting and brewing processes. Toxins. 11(5):257. doi:10.3390/toxins11050257
- Pires NA, Gonçalves De Oliveira ML, Gonçalves JA, Faria AF. 2021. Multiclass analytical method for pesticide and mycotoxin analysis in malt, brewers’ spent grain, and beer: development, validation, and application. J Agric Food Chem. 69(15):4533–4541. doi:10.1021/acs.jafc.0c07004
- Qian SY, Yang CL, Khan A, Chen RX, Wu MS, Tuo L, Wang Q, Liu JG, Cheng GG. 2019. New pyrazinoquinazoline alkaloids Isolated from a culture of Stenotrophomonas maltophilia QB-77. Nat Prod Res. 33(9):1387–1391. doi:10.1080/14786419.2018.1475381
- Rahman A, Siddiqui SA, Rahman MO, Kang SC. 2020. Cyclo (L-Pro-L-Tyr) from Streptomyces sp. 150: exploiting in vitro potential in controlling foodborne pathogens and phytopathogens. Anti-Infect Agents. 18(2):169–177. doi:10.2174/2211352517666190716155147
- Rathod PH, Shah PG, Parmar KD, Kalasariya RL. 2022. The fate of fluopyram in the soil–water–plant ecosystem: a review. Rev Environ Contam Toxicol. 260(1):1–19.
- Reed KFM. 2016. Fertility of herbivores consuming phytoestrogen-containing Medicago and Trifolium species. Agriculture. 6(3):35. doi:10.3390/agriculture6030035
- Reisinger N, Schurer-Waldheim S, Mayer E, Debevere S, Antonissen G, Sulyok M, Nagl V. 2019. Mycotoxin occurrence in maize silage-a neglected risk for bovine gut health? Toxins. 11(10):577. doi:10.3390/toxins11100577
- Rivera-Becerril F, van Tuinen D, Chatagnier O, Rouard N, Béguet J, Kuszala C, Soulas G, Gianinazzi-Pearson V, Martin-Laurent F. 2017. Impact of a pesticide cocktail (fenhexamid, folpel, deltamethrin) on the abundance of Glomeromycota in two agricultural soils. Sci Total Environ. 577:84–93. doi:10.1016/j.scitotenv.2016.10.098
- Rizzati V, Briand O, Guillou H, Gamet-Payrastre L. 2016. Effects of pesticide mixtures in human and animal models: an update of the recent literature. Chem Biol Interact. 254:231–246. doi:10.1016/j.cbi.2016.06.003
- Rodrigues ET, Lopes I, Pardal MÂ. 2013. Occurrence, fate and effects of azoxystrobin in aquatic ecosystems: a review. Environ Int. 53:18–28. doi:10.1016/j.envint.2012.12.005
- Romero CM, Castellanos MRT, Mendoza RM, Reyes RA, García AR. 1997. Oestrogenic syndrome in dairy cows by alfalfa consumption with large amount of coumestrol. Vet Mex. 28(1):25–30.
- Rumbos CI, Dutton AC, Athanassiou CG. 2016. Insecticidal efficacy of two pirimiphos-methyl formulations for the control of three stored-product beetle species: effect of commodity. Crop Prot. 80:94–100. doi:10.1016/j.cropro.2015.10.002
- Schwarz PB, Hill NS, Rottinghaus GE. 2007. Fate of ergot (Claviceps purpurea) alkaloids during malting and brewing. J Am Soc Brew Chem. 65(1):1–8. doi:10.1094/ASBCJ-2007-0116-01
- Schwarz PB. 2017. Fusarium head blight and deoxynivalenol in malting and brewing: successes and future challenges. Trop Plant Pathol. 42(3):153–164. doi:10.1007/s40858-017-0146-4
- Shevach EM. 1985. The effects of cyclosporin A on the immune system. Annu Rev Immunol. 3:397–423. doi:10.1146/annurev.iy.03.040185.002145
- Shimshoni JA, Cuneah O, Sulyok M, Krska R, Galon N, Sharir B, Shlosberg A. 2013. Mycotoxins in corn and wheat silage in Israel. Food Addit Contam Part A Chem Anal Control Expo Risk Assess. 30(9):1614–1625. doi:10.1080/19440049.2013.802840
- Smith MC, Madec S, Coton E, Hymery N. 2016. Natural co-occurrence of mycotoxins in foods and feeds and their in vitro combined toxicological effects. Toxins 8(4):94. doi:10.3390/toxins8040094.
- Sooväli P, Koppel M. 2010. Efficacy of fungicide tebuconazole in barley varieties with different resistance level. Agric Food Sci. 19(1):34–42. doi:10.2137/145960610791015069
- Souza LC, Zambom MA, Pozza MSdS, Neres MA, Radis AC, Borsatti L, Castagnara DD, Gundt S. 2012. Development of microorganisms during storage of wet brewery waste under aerobic and anaerobic conditions. R Bras Zootec. 41(1):188–193. doi:10.1590/S1516-35982012000100027
- Stähelin H. 1996. The history of cyclosporin A (Sandimmune®) revisited: another point of view. Experientia. 52(1):5–13. doi:10.1007/BF01922409
- Starkey DE, Ward TJ, Aoki T, Gale LR, Kistler HC, Geiser DM, Suga H, Tóth B, Varga J, O’Donnell K. 2007. Global molecular surveillance reveals novel Fusarium head blight species and trichothecene toxin diversity. Fungal Genet Biol. 44(11):1191–1204. doi:10.1016/j.fgb.2007.03.001
- Steiner D, Malachová A, Sulyok M, Krska R. 2021. Challenges and future directions in LC-MS-based multiclass method development for the quantification of food contaminants. Anal Bioanal Chem. 413(1):25–34. doi:10.1007/s00216-020-03015-7
- Steiner D, Sulyok M, Malachová A, Mueller A, Krska R. 2020. Realizing the simultaneous liquid chromatography-tandem mass spectrometry based quantification of >1200 biotoxins, pesticides and veterinary drugs in complex feed. J Chromatogr A. 1629:461502. doi:10.1016/j.chroma.2020.461502
- Stojceska V, Ainsworth P. 2008. The effect of different enzymes on the quality of high-fibre enriched brewer’s spent grain breads. Food Chem. 110(4):865–872. doi:10.1016/j.foodchem.2008.02.074
- Sulyok M, Stadler D, Steiner D, Krska R. 2020. Validation of an LC-MS/MS-based dilute-and-shoot approach for the quantification of> 500 mycotoxins and other secondary metabolites in food crops: challenges and solutions. Anal Bioanal Chem. 412(11):2607–2620. doi:10.1007/s00216-020-02489-9
- Sy-Cordero AA, Pearce CJ, Oberlies NH. 2012. Revisiting the enniatins: a review of their isolation, biosynthesis, structure determination and biological activities. J Antibiot. 65(11):541–549. doi:10.1038/ja.2012.71
- Szulc J, Okrasa M, Dybka-Stępień K, Sulyok M, Nowak A, Otlewska A, Szponar B, Majchrzycka K. 2019. Assessment of microbiological indoor air quality in cattle breeding farms. Aerosol Air Qual Res. 20(5):1–10.
- Thrane U, Adler A, Clasen P-E, Galvano F, Langseth W, Lew H, Logrieco A, Nielsen KF, Ritieni A. 2004. Diversity in metabolite production by Fusarium langsethiae, Fusarium poae, and Fusarium sporotrichioides. Int J Food Microbiol. 95(3):257–266. doi:10.1016/j.ijfoodmicro.2003.12.005
- Uchida R, Shiomi K, Inokoshi J, Sunazuka T, Tanaka H, Iwai Y, Takayanagi H, Omura S. 1996. Andrastins AC, new protein farnesyltransferase inhibitors produced by Penicillium sp. FO-3929 II. structure elucidation and biosynthesis. J Antibiot. 49(5):418–424. doi:10.7164/antibiotics.49.418
- Urquhart AS, Hu J, Chooi YH, Idnurm A. 2019. The fungal gene cluster for biosynthesis of the antibacterial agent viriditoxin. Fungal Biol Biotechnol. 6:2. doi:10.1186/s40694-019-0072-y
- [US EPA]. United States Environmental Protection Agency. 2017. Isopyrazam fact sheet. [accessed 2022 June 11]. https://www3.epa.gov/pesticides/chem_search/reg_actions/pending/fs_PC-129222_05-Oct-11.pdf.
- Vaclavikova M, Malachova A, Veprikova Z, Dzuman Z, Zachariasova M, Hajslova J. 2013. Emerging’mycotoxins in cereals processing chains: changes of enniatins during beer and bread making. Food Chem. 136(2):750–757. doi:10.1016/j.foodchem.2012.08.031
- Vanbergen AJ. 2021. A cocktail of pesticides, parasites and hunger leaves bees down and out. Nature. 596(7872):351–352. doi:10.1038/d41586-021-02079-4
- Vejdovszky K, Hahn K, Braun D, Warth B, Marko D. 2017a. Synergistic estrogenic effects of Fusarium and Alternaria mycotoxins in vitro. Arch Toxicol. 91(3):1447–1460. doi:10.1007/s00204-016-1795-7
- Vejdovszky K, Schmidt V, Warth B, Marko D. 2017b. Combinatory estrogenic effects between the isoflavone genistein and the mycotoxins zearalenone and alternariol in vitro. Mol Nutr Food Res. 61(3):1600526. doi:10.1002/mnfr.201600526
- Violino S, Figorilli S, Costa C, Pallottino F. 2020. Internet of beer: a review on smart technologies from mash to pint. Foods. 9(7):950. doi:10.3390/foods9070950
- Walters DR, Havis ND, Oxley SJ. 2008. Ramularia collo-cygni: the biology of an emerging pathogen of barley. FEMS Microbiol Lett. 279(1):1–7. doi:10.1111/j.1574-6968.2007.00986.x
- Warne MSJ, Hawker DW. 1995. The number of components in a mixture determines whether synergistic and antagonistic or additive toxicity predominate: the funnel hypothesis. Ecotoxicol Environ Saf. 31(1):23–28. doi:10.1006/eesa.1995.1039
- Weaver AC, Adams N, Yiannikouris A. 2020. Invited Review: Use of technology to assess and monitor multimycotoxin and emerging mycotoxin challenges in feedstuffs. Appl Anim Sci. 36(1):19–25. doi:10.15232/aas.2019-01898.
- Westendorf ML, Wohlt JE. 2002. Brewing by-products: their use as animal feeds. Vet Clin North Am Food Anim Pract. 18(2):233–252. doi:10.1016/s0749-0720(02)00016-6
- [WHO] World Health Organization. 2020. The WHO recommended classification of pesticides by hazard and guidelines to classification 2019. Geneva, Switzerland: World Health Organization. [accessed 2022 June 11]. https://apps.who.int/iris/bitstream/handle/10665/332193/9789240005662-eng.pdf?ua=1.
- Wocławek-Potocka I, Korzekwa A, Skarzyński DJ. 2008. Czy nieodżywcze składniki pasz – fitoestrogeny stanowią zagrożenie w rozrodzie krów? [Can phytoestrogens pose a danger in the reproduction of cows?]. Med Weter. 64(4 B):515–519.
- Wocławek-Potocka I, Mannelli C, Boruszewska D, Kowalczyk-Zieba I, Waśniewski T, Skarżyński DJ. 2013. Diverse effects of phytoestrogens on the reproductive performance: cow as a model. Int J Endocrinol. 2013:650984. doi:10.1155/2013/650984
- Xi X, Yan J, Quan G, Cui L. 2014. Removal of the pesticide pymetrozine from aqueous solution by biochar produced from brewer’s spent grain at different pyrolytic temperatures. BioRes. 9(4):7696–7709.
- Xu Q, Zhang K, Fu Y, Ma H, Zhu F. 2020. Toxic action and baseline sensitivity of boscalid against Penicillium digitatum. Crop Prot. 137:105272. doi:10.1016/j.cropro.2020.105272
- Yao H, Xu X, Zhou Y, Xu C. 2018. Impacts of isopyrazam exposure on the development of early-life zebrafish (Danio rerio). Environ Sci Pollut Res Int. 25(24):23799–23808. doi:10.1007/s11356-018-2449-5
- Yao X, Qiao Z, Zhang F, Liu X, Du Q, Zhang J, Li X, Jiang X. 2021. Effects of a novel fungicide benzovindiflupyr in Eisenia fetida: evaluation through different levels of biological organization. Environ Pollut. 271:116336. doi:10.1016/j.envpol.2020.116336
- Zin NM, Al-Shaibani MM, Jalil J, Sukri A, Al-Maleki AR, Sidik NM. 2020. Profiling of gene expression in methicillin-resistant Staphylococcus aureus in response to cyclo-(l-Val-l-Pro) and chloramphenicol isolated from Streptomyces sp., SUK 25 reveals gene downregulation in multiple biological targets. Arch Microbiol. 202(8):2083–2092. doi:10.1007/s00203-020-01896-x
- Zubrod JP, Bundschuh M, Feckler A, Englert D, Schulz R. 2011. Ecotoxicological impact of the fungicide tebuconazole on an aquatic decomposer-detritivore system. Environ Toxicol Chem. 30(12):2718–2724. doi:10.1002/etc.679