Abstract
Durum wheat cultivars with varying abilities to accumulate cadmium were grown and treated in the field with a glyphosate-containing herbicide at different stages of maturity to produce grain with higher and lower concentrations of cadmium (0.066–0.214 mg/kg) and glyphosate (0.474–0.874 mg/kg). The grain was milled, and fractions were analysed for cadmium and glyphosate. The highest concentrations for both cadmium and glyphosate were associated with bran and shorts, although the percentage of total cadmium mass in bran (23–25%) was less than glyphosate (38%). The preparation of dried pasta from semolina and flour milling fractions reduced concentrations by a factor of 1.8 for glyphosate and 1.4 for cadmium. Dried pasta was cooked and analysed along with the cooking water for cadmium and glyphosate at seven-time points from 0 to 15 min. Concentrations of glyphosate in cooked pasta decreased significantly with cooking time; no decrease was observed for cadmium concentrations. Analysis of cooking water demonstrated that glyphosate migrated from pasta to the cooking water. After 15 min of cooking, approximately 73% of the total glyphosate mass had transferred from pasta to cooking water. Over the same time period, only 5% of the total cadmium mass had transferred from pasta to cooking water.
Introduction
The heavy metal cadmium and the pesticide glyphosate are relevant for durum wheat (Triticum durum) from both global trade and food safety perspectives. Cadmium has been observed in durum wheat and durum products (Conti et al. Citation2000; Cubadda et al. Citation2003; Vergine et al. Citation2017; Cammerata et al. Citation2021; Tittlemier and Richter Citation2022); when compared to common wheat (T. aestivum) and other cereal grains, durum can accumulate cadmium to a greater extent (Meyers et al. Citation1982). Residues of glyphosate, the active ingredient in widely used commercial herbicides, have also been quantified in durum wheat products (Zoller et al. Citation2018; Kolakowski et al. Citation2020). Maximum levels have been set in various jurisdictions for cadmium in durum wheat and range from 0.1 to 0.2 mg/kg (Codex Alimentarius Commission Citation2019; European Commission Citation2021; United States Department of Agriculture Citation2023). Maximum residue limits also exist for residues of glyphosate in wheat, including durum, and range from 5.0 to 30 mg/kg (Codex Alimentarius Commission Citation2006; Health Canada Citation2021).
Data on the fate of these potential contaminants in durum are needed to ensure that dietary exposure and risk assessments are relevant. Past research on glyphosate has demonstrated that processing common wheat through milling and bread baking can significantly reduce glyphosate content in foods, as compared to the raw grain. Therefore, dietary exposure and risk assessments performed using concentrations in raw grain could lead to different risk management outcomes than if concentrations in food “as consumed” were used. Researchers reported that glyphosate content was greatly reduced (up to 81%) when removing bran and associated milling fractions in the production of white flour (Granby et al. Citation2003; Tittlemier et al. Citation2021; Kadžienė et al. Citation2023). The fermentation stage was also reported to degrade 21% of glyphosate in dough in one study (Low et al. Citation2005) but not in another (Gélinas et al. Citation2018). Baking did not affect glyphosate content (Gélinas et al. Citation2018; Tittlemier et al. Citation2021). There are no published peer-reviewed studies on the fate of glyphosate during the production or cooking of pasta.
There are a small number of publications on cadmium in durum milling fractions and pasta. Cheli et al. (Citation2010) reported a 10–20% reduction of cadmium in whole grain during milling and production of semolina. Cubadda et al. (Citation2005) reported a 29–37% reduction of cadmium in whole grain after production of semolina in an industrial setting.
In addition, almost all data reported are for durum or durum products as sold and were not prepared for consumption; therefore, the data do not fully reflect what may be consumed, as any changes that occur during preparation and cooking are not captured. One publication describes the fate of cadmium in cooked pasta. Cubadda et al. (Citation2003) milled durum, prepared, and cooked pasta to investigate the effects of processing and cooking on a number of heavy metals, including cadmium. They reported an average 48% reduction in cadmium content of pasta after cooking. The resulting cooking water was not analysed for cadmium, so it is not known if the apparent loss from cooked pasta was due to migration into cooking water or an artefact of the experimental design.
The aim of our study was to examine cadmium and glyphosate residues throughout the process of milling durum, producing, and cooking pasta. To achieve this, Canada Western Amber Durum was grown under different treatment regimens to produce grain with a range of concentrations of cadmium and glyphosate. This provided test material with incurred residues at a range of relevant concentrations, which facilitates accurate dietary exposure assessments.
Materials and methods
Durum test material
Transcend, a wide variety in the Canada Western Amber Durum wheat class, was grown near Swift Current, Saskatchewan, Canada in 2019. Transcend is a low accumulator of cadmium, registered for production in Canada, in 2011 (Singh et al. Citation2012). The durum cultivars AC Navigator and Transcend were selected to produce test material with a range of cadmium content.
Plots of Transcend were treated with a commercially available herbicide containing glyphosate as the active ingredient (540 g acid equivalent/L). Two replicate areas, with each replication consisting of 30 plots, were used to obtain durum with incurred glyphosate levels that could be obtained with realistic use of the product. The first area was sprayed when the durum stage was at >30% kernel moisture at the recommended rate of 0.66 L product/acre (360 g acid equivalent/acre) to control weeds at the small growth stage (4–6 leaf stage). The second replicate area was sprayed at the maximum rate of 1.31 L/acre (720 g acid equivalent/acre) used to control larger weeds beyond the 4–6 leaf stage or problematic weeds that are difficult to control. The treatment on this replicate area occurred when the durum stage was at <30% kernel moisture. The different application rates were used to produce durum with higher (test material C) and lower (test material B) glyphosate content.
Treatment at higher moisture content has been reported to result in higher glyphosate residues (McNaughton et al. Citation2015; Subedi et al. Citation2017). Materials harvested from the two plots of higher or lower glyphosate content were combined for milling and pasta processing.
AC Navigator was registered for production in Canada in 2002 (Clarke et al. Citation2000), prior to the requirement of low cadmium accumulation (maximum 0.1 mg/kg cadmium) that began in 2004 in order for new cultivars to be registered for production in Canada. A sample of AC Navigator (test material A) was prepared by blending the residue materials from the 2018 and 2019 new durum variety registration trials, where it was grown as one of the check varieties. All candidates and checks in the registration trials were not treated with herbicide. A sample of AC Navigator was used as a test material of high cadmium content and also served as a control for the glyphosate portion of the study.
Milling
Durum wheat samples were milled on a four-stand Allis-Chalmers laboratory mill coupled with a small-scale semolina purifier previously described by Dexter et al. (Citation1990). The milling process consisted of four corrugated break roll passages, five corrugated sizing roll passages, and 10 purification steps. The mill room was controlled at 21 °C and 60% relative humidity. Roll gaps on the first three breaks were kept relatively wide (1.29, 0.41, and 0.20 mm) to produce semolina with coarse granulation. After the fourth break passage, particles retained on 425 µm screen were collected as bran. The coarser material retained on a 600-µm screen from purifiers 1–6 was passed through the sizing rolls and sifted on a box sifter. After sizing passages, streams with fine particles passing through the 180 µm sieve were collected as flour, whereas particles retained on a 700-µm sieve were collected as shorts. The remaining streams were passed through the purifier. Semolina streams were collected after each purification step. Streams with particles passing through the 571 µm screens but retained on the 183 µm screens were collected as semolina. Particles retained on the 630 µm screens on purifiers 7–8, 600 µm screen on purifier 9, and 425 µm screen on purifier 10 were collected as feeds. The durum milling flow diagram is available online (https://www.grainscanada.gc.ca/en/grain-research/scientific-reports/milling-evaluation/_images/figure3-en.jpg).
Pasta production and cooking
Spaghetti was prepared from the produced semolina using a customized micro-extruder as described in Fu et al. (Citation2013). Semolina and flour milling fractions (mass ratio of 6.2:1.2:1.0 for the milling fractions semolina 1, semolina 2, and flour, respectively) were mixed with water in a high-speed asymmetric centrifugal mixer (DAC 400 FVZ SpeedMixer, FlackTec, Landum, SC, USA) at constant water absorption of 31.5% to prepare dough. Dough crumbs were extruded through a four-hole Teflon-coated spaghetti die (1.8 mm) at 70 rpm and 45 °C; the fresh pasta was subsequently dried in a pilot pasta dryer with a 325-min drying cycle and maximum temperature of 85 °C (Bühler, Uzwil, Switzerland).
A number of individual trials with varying cooking times were used to avoid the complexity of removing pasta and water during the cooking process. For each of the three test materials, triplicate trials were performed for each cooking time (3, 5, 7, 9, 11,13, and 15 min). For each trial, dried pasta (35 g, accurately weighed to the nearest hundredth of a gram) was added to boiling water (350 g) in a titanium pot (0.75 L pasta pot, Evernew, Japan) heated on a hot plate. The pasta was stirred frequently using a wooden stir stick during the entire cooking time.
After the cooking time had elapsed, the pasta was removed and drained for 1 min. The cooking water was collected and weighed in a glass beaker. Water was cooled to room temperature and stored in PTFE bottles at 4 °C for approximately 10 days until analysis. A portion of cooked pasta (10 g after cooking, accurately weighed to the nearest hundredth of a gram) was used for moisture content determination, as described in Tittlemier et al. (Citation2019). The remaining cooked pasta was dried at 85 °C for 17 h in preparation for further analysis. A sub-sample of dried, cooked pasta (4 g) was ground and used for gravimetric moisture content analysis.
Sample grinding and dividing for cadmium and glyphosate analysis
Bran and shorts milling fractions were further ground on a Retsch ZM200 rotor mill fitted with the 0.75 mm sieve; all other milling fractions did not undergo further processing prior to analysis. Feeds, flour, and semolina were sub-sampled for further analysis by manual scooping of multiple increments using a scoopula and pooling to form a composite. Dried cooked pasta was ground on a Retsch ZM200 rotor mill fitted with the 0.75 mm sieve.
Cadmium analysis
All samples of grain, milling fractions, and pasta were analysed for cadmium using closed vessel microwave digestion followed by inductively coupled mass spectrometry (ICP-MS) as described in Tittlemier and Richter (Citation2022). Cooking water samples were diluted and acidified prior to ICP-MS analysis. Test portions of 1 g ground grain and grain products were digested in 5 mL of distilled concentrated nitric acid and 3 mL of ultrapure water single reaction chamber microwave digestion system using a multistage microwave programme, (UltraWAVE single reaction chamber microwave digestion system, Milestone, Shelton, CT). Digested samples were mixed with 0.25 mL of hydrochloric acid and manually taken to a volume of 50 mL using deionised water after they had reached room temperature. Aliquots of cooking water samples (25 mL) were weighed in a 50-mL digestion tube, combined with 1 mL concentrated nitric acid and 0.25 mL concentrated hydrochloric acid, and brought up to volume with ultrapure water.
Digested and diluted samples were analysed for cadmium using an Agilent 7800 ICP-MS and an automated inline system (ESI prepFAST, Elemental Scientific, Omaha, NE, USA) for dilution of standards and samples. The ICP-MS was fitted with a nickel-plated sampler cone, a nickel skimmer cone, and a 2.5-mm quartz torch. The ion at m/z 111 was acquired in spectrum scan mode for cadmium, using a dwell and integration time of 2000 ms and 103Rh as the internal standard. Interferences from MoO+ were minimized using a collision cell in He gas mode. Quantitation was performed directly using one blank and a series of calibration standards. The limit of quantitation of 0.01 mg/kg was determined as 10× the standard deviation of solution blank results, using seven digested solution blanks analysed over 5 d. The working range of the method was 0.01 to 36 mg/kg.
Quality control samples were included in each sample batch and were taken through sample preparation, microwave digestion, and ICP-MS analysis to monitor method performance. Quality control samples included reagent blanks that contained no matrix but were otherwise treated the same as samples; in-house grain-based reference materials and commercially available certified reference materials used to monitor method accuracy and precision; duplicate test portions of one sample per batch used to monitor method precision and triplicate test portions of the same sample fortified with cadmium at 0.2 mg/kg prior to microwave digestion to monitor analyte recovery.
Glyphosate analysis
All samples of grain, milling fractions, pasta, and cooking water were analysed for glyphosate and its main metabolite, aminomethylphosphonic acid (AMPA), using a comprehensive instrumental method involving extraction with water and dichloromethane, derivatization, and high-resolution mass spectrometric analysis. AMPA was included in the analysis as it is part of the Canadian residue definition (Health Canada Citation2021).
Most test portions of milling fractions and pasta were 30 g. For instances when only smaller amounts of sample were available, the volume of 2:1 (v/v) water/dichloromethane extraction solvent used maintained the 1:8 ratio of sample mass to extraction solvent volume. An aliquot of the aqueous extract was diluted with a borate buffer and mixed with stable isotope labelled internal standards (13C2-glyphosate and 13C,15N,2H2-AMPA) prior to derivatization with 9-fluorenylmethyl chloroformate. The derivatized extract solutions were cleaned using a reversed-phase solid-phase extraction sorbent, dried under vacuum, and reconstituted in water as described in Tittlemier et al. (Citation2017).
Cooking water (10 mL) test portions were fortified with the stable isotope labelled internal standards and 100 µL hydrochloric acid (6 M). The samples were shaken for 1 min and then rested for 1 min. A 115 µL aliquot of potassium hydroxide (6 M) was then added, along with borate buffer (300 µL, 0.45 M, pH = 9) and 9-fluorenylmethyl chloroformate derivatizing agent (600 µL, 28 mg/mL in acetonitrile). The derivatization proceeded for 60 min, after which water (5 mL, pH = 3) was added to stop the reaction. The samples were then centrifuged at room temperature for 10 min at 9700 × g and then further processed as described in the previous paragraph.
Liquid chromatography and high-resolution mass spectrometric analysis was performed on a Dionex Ultimate 3000 UHPLC fitted with a Waters Acquity UHPLC BEH C18 column (1.7 µm particle size, 2.1 × 50 mm) and coupled to an ion trap mass analyser (Q-Exactive Orbitrap, ThermoFisher Scientific). Separation occurred using the same program as described in Tittlemier et al. (Citation2017). Mass spectrometric identification and quantitation were performed using a full scan (100-500 m/z; resolution 70,000) with data dependant MS2 (resolution 17,500) in negative electrospray ionization mode. Mass spectrometer parameters were as follows: auxiliary gas heater temperature 413 °C, capillary temperature 300 °C, spray voltage 2.50 kV, and S-lens RF level 60 V. Gas flow rates were as follows, in arbitrary units reported by instrument: sheath gas 48, auxiliary gas 11, and sweep gas 2. Stepped collision energies of 10, 20, and 40 eV were used during collisional dissociation. For each analyte and internal standard, quantifier and qualifier ions were identified: glyphosate (m/z 390.0748, 168.0068, respectively), 13C2-glyphosate (m/z 392.0752, 170.0075), AMPA (m/z 332.0693, 110.0018), and 13C,15N,2H2-AMPA (m/z 336.0823, 114.0145).
Analytes were quantitated against a seven-point calibration curve prepared in solvent. Analyte peak areas were normalized to peak areas of analogous stable isotope labelled internal standards prior to performing quantitation using 1/× weighting of the calibration curve. Analytes were determined to be positively identified if retention time was within ± 0.1 min of average retention time in calibration standards, ≥12 points were collected across the peak, mass accuracy was ≤5 ppm when comparing experimental to theoretical masses of quantifier ions, and ratios of quantifier to qualifier ions were within ± 30% of the average ratio observed in calibration standards. Limits of quantitation were 0.1 mg/kg glyphosate and AMPA in milling fractions, 0.05 mg/kg glyphosate and 0.2 mg/kg AMPA in cooked and dried pasta, and 0.003 mg/kg for glyphosate in water, determined according to Wenzl et al. (Citation2016). The working ranges of the method for the various matrices analysed were from the limits of quantitation up to 4 mg/kg glyphosate for milling fractions and pasta and up to 0.05 mg/kg glyphosate in water.
Quality control samples were included in each sample batch and were taken through sample preparation and analysis to monitor method performance. Quality control samples included ground pasta, water, and ground durum known to contain no quantifiable glyphosate; in-house durum reference material containing 1.3 mg/kg glyphosate used to monitor method accuracy and precision; duplicate test portions of one sample per batch used to monitor method precision; and fortified durum, pasta, and water to monitor analyte recovery. Results from the quality control analyses are available in the Supplementary Information .
Results and discussion
Distribution of glyphosate and cadmium in milling fractions
Concentrations of the target analytes in harvested whole grain and various milling fractions produced are listed in and ; summaries of quality control data demonstrating good performance of the analytical methods are provided in Supplementary Information and . As expected, no glyphosate was detected in grain and milling products from test material A, which was from untreated durum (). The reported impact of grain maturity on glyphosate residues in grain was exploited to produce test materials with low and high levels of incurred glyphosate. Glyphosate concentrations in grain and milling fractions from test material C, prepared from durum treated when the kernel moisture was >30%, were 1.9-fold higher than in grain and milling fractions from test material B. This difference in glyphosate residue concentrations due to application at various stages of maturity (and, therefore, grain moisture content) has been reported for beans (McNaughton et al. Citation2015) and red lentils (Subedi et al. Citation2017). The glyphosate transformation product aminomethylphosphonic acid (AMPA) was not detected in any grain or subsequent products; this absence is consistent with other studies of glyphosate residues in wheat (Gélinas et al. Citation2018; Tittlemier et al. Citation2021).
Table 1. Mean ± standard deviation (n = 3) glyphosate (mg/kg) in whole grain and durum milling fractions from the test materials produced from durum treated with glyphosate-containing herbicide product during the growing season [A: no application of product; B: application at <30% grain moisture content (MC); C: application at >30% MC].
Table 2. Mean ± standard deviation (n = 3) cadmium (mg/kg) in whole grain and durum milling fractions from the test materials representing a high cadmium accumulating variety (A) and a low cadmium accumulating variety (B, C).
The use of two durum cultivars also ensured the test materials used in this study spanned a range of cadmium concentrations. The high cadmium accumulating AC Navigator produced whole grain at 0.214 mg/kg (), which is similar to concentrations of 0.243 mg/kg reported for this cultivar in a previous study (Tittlemier and Richter Citation2022). Test materials B and C, prepared from the low accumulating cultivar Transcend, also contained cadmium at similar concentrations in whole grain (0.066–0.0714 mg/kg) to those reported in other low accumulating cultivars in Co-operative Registration Trials over the past decade (0.058–0.079 mg/kg) (Tittlemier and Richter Citation2022). The ratio of cadmium concentrations in the high accumulating as compared to the low accumulating cultivar was 3.1, which was consistent for all milling products prepared from the three test materials as well.
The distribution of glyphosate residues among milling fractions was consistent between the low (B) and high (C) test materials (). Approximately 38% of glyphosate was associated with bran and 13% with shorts. In a similar fashion to glyphosate, cadmium distribution among milling fractions was consistent among the low (B, C) and high (A) cadmium accumulating cultivars (); however, it was less sequestered in bran than glyphosate residues. Concentrations were lowest in the milling fractions associated with the endosperm, consistent with the mapped distribution in kernels (Yan et al. Citation2020). The reduction in the concentration of cadmium from whole grain to semolina observed (29%) was comparable to the reductions of 29–37% reported by Cubadda et al. (Citation2003, Citation2005) and somewhat higher than the reductions of 10–20% reported by Cheli et al. (Citation2010).
Figure 1. Distribution of total cadmium (Cd), glyphosate (GLY), and sample mass in test material (A, B, and C) milling fractions. Percentages represent the mean of three replicate test portions.
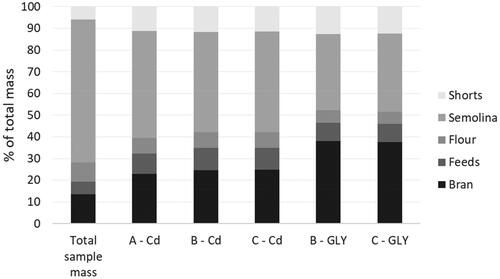
The fractionation of glyphosate and cadmium among milling products resulted in a decrease in glyphosate and cadmium content when pasta was prepared. The preparation of dried pasta from semolina and flour milling fractions reduced concentrations by a factor of 1.8 for glyphosate and 1.4 for cadmium.
Fate of glyphosate and cadmium during pasta cooking
Concentrations of glyphosate and cadmium in cooked pasta and cooking water are provided in and . Glyphosate concentrations in pasta decreased during cooking (). Over 15 min of cooking time, concentrations decreased by a factor of 3 for both test materials. There was a concomitant increase in glyphosate concentrations in the cooking water over the cooking time. All mean pasta and cooking water glyphosate concentrations were significantly different at each time point for test material C (one-way ANOVA, p < 0.001). For test material B with the lower glyphosate concentrations, all mean pasta glyphosate concentrations were significantly different at each time point, but the cooking water glyphosate concentrations were significantly different only between the early (0 and 3 min) and end (15 min) time points.
Table 3. Mean ± standard deviation (n = 3) glyphosate in pasta (dry weight) and cooking water sampled at various time points during cooking. Pasta was prepared from test materials B [application of glyphosate-containing herbicide product at <30% grain moisture content (MC)] and C (application at >30% MC). Unique superscripts indicate means were significantly different (one-way ANOVA, p < 0.001).
Table 4. Mean ± standard deviation (n = 3) cadmium in pasta (dry weight) and cooking water sampled at various time points during cooking. Pasta was prepared from test materials representing a high cadmium accumulating variety (A) and a low cadmium accumulating variety (B, C). Unique superscripts indicate means were significantly different (one-way ANOVA, p < 0.001).
In contrast to glyphosate, cadmium concentrations in pasta did not decrease significantly with an increase in cooking time (). However, cadmium concentrations in cooking water did increase. Mean cadmium water concentration increases from test material A were statistically significant from time point to subsequent time point (one-way ANOVA, p < 0.001). For cooking water from test materials B and C, only median cadmium concentrations from the early (0 and 3 min) and end (9 and 15 min) time points were significantly different (Kruskal–Wallis one-way ANOA on ranks, p = 0.011). The source of cadmium in the cooking water was confirmed to be the pasta. A small amount of pasta, depending on cooking time, can be disintegrated during cooking and leached into cooking water. Analysis of water from blank trials where pasta was not added to the cooking water did not contain any cadmium above the limit of quantitation of 0.1 pg/µL at any of the time points.
To investigate the fate of glyphosate and cadmium in pasta during cooking, the mass of each analyte in pasta at various cooking times was determined. This removes the impact of water absorption by pasta as well as pasta loss because of solubilisation in cooking water (Malcolmson and Matsuo Citation1993).
Over 15 min of cooking, the glyphosate content of pasta decreased by 73.5 ± 0.7% and 73.0 ± 0.7% for the pasta containing lower and higher amounts of glyphosate, respectively. The loss of glyphosate at each individual time point was consistent between the pasta from the two test materials (). The decrease in glyphosate content was more rapid at the beginning of the cooking process, with a mean loss of almost half of the glyphosate (49 ± 1%; n = 6) to cooking water after 3 min of cooking. After a more realistic cooking time of 9 min, the loss of glyphosate from pasta prepared from test materials B and C averaged 65.1 ± 0.6% (n = 6).
Figure 2. Change in glyphosate content of pasta and water during cooking. Bars represent the mean from the analysis of triplicate test portions.
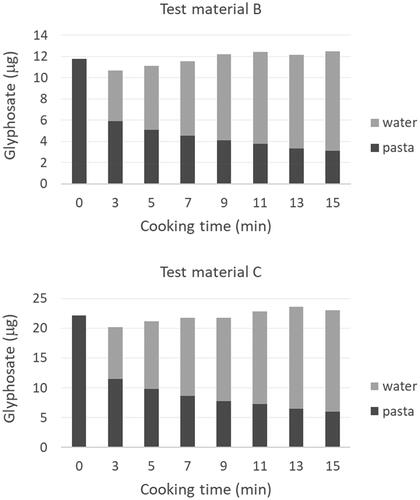
Consideration of the mass of pasta transferred to water during cooking suggests that the decrease in pasta glyphosate content is mainly due to the migration of glyphosate to water and not via solubilisation of glyphosate-starch or glyphosate-protein complexes. An average and standard deviation of only 7.76 ± 0.03% (n = 9) of the starting pasta mass was lost during 15 min of cooking. As described earlier, approximately 73% of the mass of glyphosate was lost from pasta during 15 min of cooking.
There was no notable loss of glyphosate from the cooking “system” after assessing the total mass of glyphosate over the entire cooking process, starting from dried spaghetti through to cooked pasta and including cooking water. The mean change in glyphosate content of pasta plus cooking water from start (0 min) to finish (15 min) of cooking was –0.2% and ranged from –6.0% to 9.0% for each of the seven sampling time points. This indicates that glyphosate “lost” from pasta remains in the cooking water.
As with glyphosate, there was no notable loss of cadmium from the cooking system. The mean change in cadmium content of pasta plus cooking water from start (0 min) to finish (15 min) of cooking was –1.1%.
As compared to glyphosate, however, there was no evidence of substantial cadmium loss from pasta during cooking (). After 15 min of cooking, only approximately 5% of the total cadmium mass in the pasta was present in the cooking water from pasta prepared from all three test materials. Data from the pasta and cooking water obtained from test materials B and C were combined for analysis because of the lack of difference in cadmium concentration between the two test materials.
Figure 3. Change in cadmium content of pasta and water during cooking. Bars represent the mean from the analysis of replicate test portions (n = 3 for A and n = 6 for B, C).
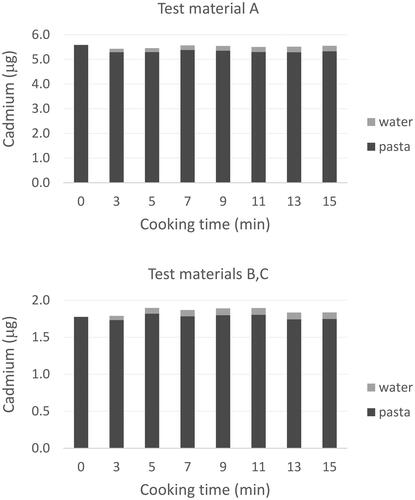
The absence of change during cooking is different than what was noted by Cubadda et al. (Citation2003), where cadmium concentrations were reported to decrease from dry to cooked spaghetti by a mean of 48% on a dry weight basis. Unfortunately, the resulting cooking water from this study was not analysed for cadmium, so it is not known if the apparent loss from cooked pasta was due to the migration of cadmium into the cooking water. The ratio of pasta to water nor the cooking time were provided; therefore, the discrepancy in cadmium fate during pasta cooking observed by Cubadda et al. (Citation2003) and in our study cannot be explained.
The marked difference in the fate of glyphosate and cadmium during pasta cooking observed in our study is likely governed by various factors involved in their bioaccumulation, in planta transport, and sequestration within the pasta matrix. There is some published information on these factors for cadmium in durum plants, but less so for durum grain (Sterckeman and Thomine Citation2020). For example, cadmium uptake in durum involves complexing with counter ions in aqueous solution, including citrate (Sterckeman Citation2023). Within various plant tissues, cadmium can also bind to cysteine-rich metallothionein proteins. These proteins appear to be involved in metal homeostasis and oxidative stress responses in a number of organisms, including plants. Metallothionein has been identified in durum wheat with a binding capacity of 4 ± 1 mol cadmium/mol protein (Bilecen et al. Citation2005). Cadmium binding to metallothioneins is consistent with the co-occurrence of cadmium and sulphur in durum kernels (Yan et al. Citation2020). There is minimal published information on the factors that could be impacting the fate of glyphosate during cooking. Glyphosate is noted as phloem-transported (Arnaud et al. Citation1994), likely facilitated by aqueous solubility of glyphosate 15.7 g/L (Skark et al. Citation1998). However, it is unclear how processing durum grain, including producing and cooking pasta, affects bound or otherwise sequestered cadmium and glyphosate within the grain.
The transfer from pasta to cooking water has implications for consumers’ exposure, particularly to glyphosate. Cooking pasta would greatly reduce dietary exposure to glyphosate but have minimal impact on cadmium dietary exposure. Glyphosate processing factors for cooking pasta, estimated as the ratio of concentration (dry weight basis) in cooked to uncooked pasta, are 0.5, 0.4, and 0.3 for 7 (for al dente texture), 9 (fully cooked), and 15 min (overly cooked) cooking times, respectively. Incorporating these processing factors into dietary exposure and risk assessments would provide a more realistic picture and be better aligned with ideal practices that use occurrence data from food “as consumed” rather than “as grown” or “as purchased” (Food and Agriculture Organization World Health Organization 2009).
Credit authorship contribution statement
Sheryl A. Tittlemier: conceptualization, project administration, methodology, supervision (chemical analyses), formal analysis, writing – original draft. Lianna Bestvater: investigation, writing – review & editing. Jason Chan: investigation. Valentina Timofeiev: investigation. Anja Richter: supervision, validation, writing – review & editing. Kun Wang: investigation, methodology, writing – review & editing. Marta Izydorczyk: supervision (milling). Yuefeng Ruan – resources (durum production), supervision (durum production), methodology (field trialling with herbicide application), writing – review & editing. Bin Xiao Fu: conceptualization, project administration, supervision (pasta production, cooking), methodology, writing – review & editing.
Acknowledgment
Dave Turnock performed the milling, and Michael Bestvater assisted with the preparation of samples for analysis.
References
- Arnaud L, Nurit F, Ravanel P, Tissut M. 1994. Distribution of glyphosate and of its target enzyme inside wheat plants. Pestic Sci. 40(3):217–223. doi: 10.1002/ps.2780400308.
- Bilecen K, Ozturk UH, Duru AD, Sutlu T, Petoukhov MV, Svergun DI, Koch MHJ, Sezerman UO, Cakmak I, Sayers Z. 2005. Triticum durum metallothionein: isolation of the gene and structural characterization of the protein using solution scattering and molecular modeling. J Biol Chem. 280(14):13701–13711. doi: 10.1074/jbc.M412984200.
- Cammerata A, Marabottini R, Allevato E, Aureli G, Stazi SR. 2021. Content of minerals and deoxynivalenol in the air-classified fractions of durum wheat. Cereal Chem. 98(5):1101–1111. doi: 10.1002/cche.10458.
- Cheli F, Campagnoli A, Ventura V, Brera C, Berdini C, Palmaccio E, Dell’Orto V. 2010. Effects of industrial processing on the distributions of deoxynivalenol, cadmium and lead in durum wheat milling fractions. LWT-Food Sci Technol. 43(7):1050–1057. doi: 10.1016/j.lwt.2010.01.024.
- Clarke JM, McLeod JG, DePauw RM, Marchylo BA, McCaig TN, Knox RE, Fernandez MR, Ames N. 2000. AC Navigator durum wheat. Can J Plant Sci. 80(2):343–345. doi: 10.4141/P99-108.
- Codex Alimentarius Commission. 2006. Pesticides database search 158-Glyphosate. [accessed 2023 April 26]. https://www.fao.org/fao-who-codexalimentarius/codex-texts/dbs/pestres/pesticide-detail/en/?p_id=158.
- Codex Alimentarius Commission. 2019. Codex general standard for contaminants and toxins in food and feed. 193–1995. [accessed 2023 September 29]. https://www.fao.org/fao-who-codexalimentarius/sh-proxy/en/?lnk=1&url=https%253A%252F%252Fworkspace.fao.org%252Fsites%252Fcodex%252FStandards%252FCXS%2B193-1995%252FCXS_193e.pdf.
- Conti ME, Cubadda F, Carcea M. 2000. Trace metals in soft and durum wheat from Italy. Food Addit Contam. 17(1):45–53. doi: 10.1080/026520300283577.
- Cubadda F, Raggi A, Marconi E. 2005. Effects of processing on five selected metals in the durum wheat food chain. Microchem J. 79(1-2):97–102. doi: 10.1016/j.microc.2004.06.011.
- Cubadda F, Raggi A, Zanasi F, Carcea M. 2003. From durum wheat to pasta: effect of technological processing on the levels of arsenic, cadmium, lead and nickel—a pilot study. Food Addit Contam. 20(4):353–360. doi: 10.1080/0265203031000121996.
- Dexter JE, Matsuo RR, Kruger J. 1990. The spaghetti-making quality of commercial durum wheat samples with variable a-amylase activity. Cereal Chem. 67:405–412.
- European Commission, 2021. Commission regulation (EU)2021/1323 of 10 August 2021 amending regulation (EC)No 1881/2006 as regards maximum levels of cadmium incertain foodstuffs. Off J Eur Commun. L288:13–18.
- Food and Agriculture Organization, World Health Organization. 2009. Chapter 6: Dietary exposure assessment of chemicals in food. In: Environmental health criteria 240 on principles and methods for the risk assessment of chemicals in food. Geneva (Switzerland): World Health Organization. pp. 6-1-6-95.
- Fu BX, Schlichting L, Pozniak CJ, Singh AK. 2013. Pigment loss from semolina to dough: rapid measurement and relationship with pasta colour. J Cereal Sci. 57(3):560–566. doi: 10.1016/j.jcs.2013.03.007.
- Gélinas P, Gagnon F, McKinnon C. 2018. Wheat preharvest herbicide application, whole-grain flour properties, yeast activity and the degradation of glyphosate in bread. Int J Food Sci Tech. 53(7):1597–1602. doi: 10.1111/ijfs.13741.
- Granby K, Johannesen S, Vahl M. 2003. Analysis of glyphosate residues in cereals using liquid chromatography-mass spectrometry (LC-MS/MS). Food Addit Contam. 20(8):692–698. doi: 10.1080/0265203031000109477.
- Health Canada. 2021. Maximum residue limits search. [accessed 2023 April 26]. https://pest-control.canada.ca/pesticide-registry/en/mrl-search.html.
- Health Canada. 2021. Residue definitions for chemicals with maximum residue limits regulated under the Pest Control Products Act. [accessed 2023 August 31]. https://www.canada.ca/en/health-canada/services/consumer-product-safety/pesticides-pest-management/public/protecting-your-health-environment/pesticides-food/residue-definitions-chemicals-maximum-residue-limits-regulated-under-pest-control-products-act.html.
- Kadžienė G, Pranaitienė S, Auškalnienė O, Veršulienė A, Supronienė S, Žvirdauskienė R, Gecaitė V, Cesevičienė J, Semaškienė R. 2023. Oilseed rape, wheat, and barley grain contamination as affected by different glyphosate usage. Plants. 12(6):1335. doi: 10.3390/plants12061335.
- Kolakowski BM, Miller L, Murray A, Leclair A, Bietlot H, van de Riet JM. 2020. Analysis of glyphosate residues in foods from the Canadian retail markets between 2015 and 2017. J Agric Food Chem. 68(18):5201–5211. doi: 10.1021/acs.jafc.9b07819.
- Low FL, Shaw IC, Gerrard JA. 2005. The effect of Saccharomyces cerevisiae on the stability of the herbicide glyphosate during bread leavening. Lett Appl Microbiol. 40(2):133–137. doi: 10.1111/j.1472-765X.2004.01633.x.
- Malcolmson L, Matsuo RR. 1993. Effects of cooking water composition on stickiness and cooking loss of spaghetti. Cereal Chem. 70:272–275.
- McNaughton KE, Blackshaw RE, Waddell KA, Gulden RH, Sikkema PH, Gillard CL. 2015. Effect of application timing of glyphosate and saflufenacil as desiccants in dry edible bean (Phaseolus vulgaris L. Can J Plant Sci. 95(2):369–375. doi: 10.4141/cjps-2014-157.
- Meyers MW, Fricke FL, Holmgren GGS, Kubota J, Chaney RL. 1982. Cadmium and lead in wheat grain and associated surface soils of major wheat production areas in the United States. Agrononomy Abstracts. 34.
- Singh AK, Clarke JM, Knox RE, DePauw RM, McCaig TN, Fernandez MR, Clarke FR. 2012. Transcend Durum wheat. Can J Plant Sci. 92(4):809–813. doi: 10.4141/cjps2011-255.
- Skark C, Zullei-Seibert N, Schöttler U, Schlett C. 1998. The occurrence of glyphosate in surface water. Int J Environ Anal Chem. 70(1-4):93–104. doi: 10.1080/03067319808032607.
- Sterckeman T. 2023. On the contribution of cadmium-citrate complexes to cadmium uptake by durum wheat. Plant Soil. 487(1-2):455–465. doi: 10.1007/s11104-023-05943-6.
- Sterckeman T, Thomine S. 2020. Mechanisms of cadmium accumulation in plants. Crit Rev Plant Sci. 39(4):322–359. doi: 10.1080/07352689.2020.1792179.
- Subedi M, Willenborg CJ, Vandenberg A. 2017. Influence of harvest aid herbicides on seed germination, seedling vigor and milling quality traits of red lentil (Lens culinaris L.). Front Plant Sci. 8:311. doi: 10.3389/fpls.2017.00311.
- Tittlemier SA, Bestvater L, Carlson J, Kletke J, Izydorczyk M, Fu BX. 2021. Fate of glyphosate in wheat during milling and bread production. Cereal Chem. 98(1):100–108. doi: 10.1002/cche.10369.
- Tittlemier SA, Drul D, Lake B, Zirdum T, Hammond E, Sobering D, Lin WJ, Tran M, Roscoe M. 2017. Evaluation of a commercially available enzyme-linked immunosorbent assay and a liquid chromatography–tandem mass spectrometric method for the analysis of glyphosate in wheat, oats, barley, malt, and lentils. Cereal Chem. 94(6):1028–1036. doi: 10.1094/CCHEM-01-17-0021-R.
- Tittlemier SA, Drul D, Roscoe M, Turnock D, Taylor D, Fu BX. 2019. Fate of ergot alkaloids during laboratory scale durum processing and pasta production. Toxins (Basel). 11(4):195. doi: 10.3390/toxins11040195.
- Tittlemier SA, Richter A. 2022. Cadmium concentrations in Canadian durum exports decreased with the adoption of low accumulating cultivars. Food Addit Contam Part A: Chem Anal Control Expo Risk Assess. 39(12):1953–1962. doi: 10.1080/19440049.2022.2130441.
- United States Department of Agriculture. 2023. Foreign agricultural service global agricultural information net-work. China releases the standard for maximum levels ofcontaminants in foods (National Food Safety Standardfor Maximum Levels of Contaminants in Foods). Report number CH18025. Attaché Report (GAIN). GB 2762–2022.
- Vergine M, Aprile A, Sabella E, Genga A, Siciliano M, Rampino P, Lenucci MS, Luvisi A, Bellis LD. 2017. Cadmium concentration in grains of durum wheat (Triticum turgidum L. subsp. durum). J Agric Food Chem. 65(30):6240–6246. doi: 10.1021/acs.jafc.7b01946.
- Wenzl T, Johannes H, Schaechtele A, Robouch P, Stroka J. 2016. Guidance document on the estimation of LOD and LOQ for measurements in the field of contaminants in feed and food. EUR 28099 EN, OPOCE LA-NA-28099-EN-N.
- Yan B, Isaure M-P, Mounicou S, Castillo-Michel H, De Nolf W, Nguyen C, Cornu J-Y. 2020. Cadmium distribution in mature durum wheat grains using dissection, laser ablation-ICP-MS and synchrotron techniques. Environ Pollut. 260:113987. doi: 10.1016/j.envpol.2020.113987.
- Zoller O, Rhyn P, Rupp H, Zarn JA, Geiser C. 2018. Glyphosate residues in Swiss market foods: monitoring and risk evaluation. Food Addit Contam Part B Surveill. 11(2):83–91. doi: 10.1080/19393210.2017.1419509.