Abstract
Adsorbent prepared from Annona squamosa (custard apple) fruit shell (CAS) was successfully used to remove lead (Pb2+) and cadmium (Cd2+) from aqueous solution in a batch process. Adsorption studies were conducted on a batch process, to study the effects of contact time, initial concentration of metal ions, adsorbent dose CAS, pH and temperature. The optimum contact time and pH for the removal of Pb2+ and Cd2+ was 30 min and pH 5, respectively. Kinetic parameters of adsorptions such as pseudo-first order, pseudo-second order were determined. The maximum loading capacity of Pb2+ and Cd2+ was found to be 90.93 and 71.0 mg g−1 of the adsorbent, respectively. The equilibrium data were analyzed by the Langmuir and Freundlich isotherms. Langmuir model gives a better fit than the Freundlich model. The pseudo-second-order kinetics was the best for the adsorption of Pb2+and Cd2+, by CAS (A. Squamosa) with good correlation. Thermodynamic parameters, such as standard free energy change (ΔG°), standard enthalpy change (ΔH°) and standard entropy change (ΔS°), were analyzed. The results suggest that the CAS can be used as an effective, low cost, and eco-friendly green adsorbent for the removal of selected metal ions from aqueous solution.
1. Introduction
Heavy metal contamination due to rapid industrialization has been a major global concern. It is well known that the industrial effluents loaded with heavy metals have severe adverse effect on human and aquatic life. Their toxic nature on environment has resulted in the enforcement of stringent laws for maximum allowable limits in the water bodies Citation[1]. Lead (Pb2+) and cadmium (Cd2+) among heavy metals are considered as most toxic and increase in disposal of these metals into water streams has been a continuous issue. Increased usage of cadmium and lead with increase in number of electroplating, pigments, metallurgy, battery industries as well as mining has set up severe environment contamination [Citation2–Citation4].
The potential health hazards of Pb2+ and Cd2+ contamination have been extensively studied and paved way to various treatment processes for the removal of Pb2+ and Cd2+ from wastewater. The treatment process included precipitation, ion exchange, filtration, membrane filtration, electrochemical treatment, and reverse osmosis Citation[5]. However, these conventional techniques have many disadvantages like less efficiency, high cost, long processing time, and high cost of disposal Citation[6]. Adsorption is a powerful and most efficient and cost effective technique for removal of heavy metals from waste water. Many agricultural wastes have been tried out as effective and low-cost adsorbents for the removal of heavy metals from waste water. Many of the fruit peels and seeds have also been used as effective sorbents for the removal of heavy metals which include banana peel Citation[7], mango peel Citation[8], orange peel, citrus peel Citation[9], jackfruit peel Citation[10], ponkan peel Citation[11], pomegranate peel Citation[12], watermelon rind Citation[13], gooseberry fruit Citation[14], and pine fruit coat. Heavy metals such as Pb2+, Cd2+, Zn2+, Ni2+, Cr6+, and Cu2+ are prior toxic pollutants in industrial wastewater, which become common groundwater contaminants and they tend to accumulate in organisms, causing numerous diseases and disorders Citation[15]. Thus, adequate treatment of metals containing effluents prior to discharge into receiving bodies of water is of great importance for human health and environmental quality. Custard apple shell (CAS) consists of pectin, citrulline, cellulose, proteins, and carateniods. These polymers have hydroxyl and carboxylic functional groups which can facilitate easy binding to metal ions Citation[16]. Processing of fruits produces two types of waste—a solid waste of peel/shell etc.––a liquid waste of juice and wash waters. (e.g. mango 30–50%, banana 20%, pineapple 40–50%, and custard apple 30–50%. Therefore, there is a serious waste disposal problem, which can lead to problem with flies and rats around the processing room, if not correctly dealt with. In that aspect, CAS is abundant and nonedible waste which is mainly composed of calcium carbonate and is found in many coastal areas; therefore, economically recycling and reusing this waste is desirable. In general, finding uses (especially on a large scale) for this abundant waste material would be profitable from both an environmental and economic point of view. Herein, we report the use of crushed CAS as an agent for the removal of Pb2+and Cd2+ from aqueous solution through adsorption. The conversion of this waste material, as contrasting to the use of commercially available materials, into materials that can potentially improve the environment would be advantageous in response to ecological constraints for the sustainable development of industries using heavy metals. In this work, the adsorption of Pb2+ and Cd2+ ions from aqueous solution was studies using CAS, to the best of our knowledge, no studies on the CAS removing heavy metals from aqueous solution have been reported so far. The influence of pH, temperature, adsorbent dose, contact time, and initial metal ion concentration on adsorption was investigated. Experimental sorption isotherms were fitted to the Langmuir and Freundlich isotherm models. The pseudo-first order and pseudo-second order were used to study the sorption kinetics and acid desorption tests were also performed.
2 Materials and methods
2.1 Preparation of adsorbent
A. squamosa fruits were collected from in and around Vellore (12°56′23″N, 79°14′23″E) Vellore district, Tamil Nadu, India. The taxonomic identification was made by Dr. Chelladurai, Professor in botany, Voorhees College. CAS was washed several times under tap water and then double distilled water. After thorough washing to remove all the surface impurities, CAS was cut into small pieces and dried under sun light for seven days to remove all moisture content present. Later, the dried CAS pieces were washed with 80°C hot water to remove any soluble matter present and dried in oven at 95°C for 48 h. The oven-dried CAS was powdered and sieved through 100 mesh and was stored in desiccators and used for all sorption experiments Citation[17].
2.2 Preparation of synthetic stock solutions
The stock solutions of Pb2+ and Cd2+ were prepared by dissolving appropriate nitrate salts in double distilled water. Adjustments of pH were carried out using 0.1 M HCl and 0.1 M NaOH solutions. All reagents used were of analytical grade. Each experiment was repeated twice and average values have been reported.
2.3 Batch mode adsorption studies
Sorption experiments were conducted at 303 k in a roto spin unit at 50 rpm using 50-mL tarsons tubes. Effect of parameters like adsorbent dosage, contact time, pH and initial metal concentration was studied. Sorption capacity of CAS was determined by contacting 30 mg with 20 mL of known concentration of metal ion solution (50–200 ppm). The solid phase was separated using 0.45-μm filter paper and the residual metal concentration present in the supernatant was determined by Atomic absorption spectrophotometer (AAS) (Varian, AA240). The effect of pH on the efficiency of adsorption of these heavy metals was verified between pH 2 and 9. The contact time was varied between 5 and 120 min to study the effect of time on sorption and adsorbent dosage was varied from 10 to 100 mg to get the ratio of adsorbates to adsorbent. The amount of metal adsorbed to CAS was determined using Eq. (1) and percentage removal of heavy metal was evaluated using Eq. (2).(1)
(2)
where qe is the metal uptake (mg/g) by CAS, C0 and C1 are initial and final metal concentrations (mg/L), V is the solution volume (L), and m is mass of the adsorbent (g).
2.4 Instrumentation
Flame AAS (AAS, Varian, AA-240) equipped with air/acetylene burner was used to determine the concentration of lead and cadmium. The detector response was taken at 283.3 nm for Pb2+ and 326.1 nm for Cd2+, respectively. Fourier transform infrared spectroscopy (FTIR) (Thermo Nicolet, AVATAR 330) data were utilized to know the presence of functional groups in CAS. FTIR spectra of CAS and metal loaded CAS was recorded in mid IR region in the range of 4,000–400 cm−1 by KBr pellet method. Scanning Electron Microscope (Phillips XL 30, Netherlands) pictures were taken to know the surface morphology of CAS before and after sorption of Pb2+ and Cd2+. Elemental analysis was done by electron dispersive X-ray analysis associated with scanning electron microscopy (SEM).
2.5 Data analysis
The applicability of Freundlich and Langmuir isotherms to the equilibrium sorption was studied using standard straight line equations and kinetic studies were done to understand the mechanism of adsorption.
2.6 Desorption studies
To investigate the possibilities of repeated use of the adsorbent, desorption and regeneration experiments were also conducted. Metal loaded CAS sorbent (0.1 g) was shaken with 20 mL of 0.1 M HCl as the desorbing agent in 50-mL tarson tubes at 50 rpm for 30 min at ambient temperature. The centrifuged supernatant was analyzed for metal ion concentration and metal ion desorbed CAS was used as a regenerated sorbent in three repeated cycles to determine the reusability potential of adsorbent.
3 Results and discussion
3.1 Effect of pH
The effect of pH on metal adsorption is considered as a very important parameter in adsorption process. The functional groups responsible for binding of metals ions in the adsorbent and the competition of metal ion that gets adsorbed to active sites of adsorbent are affected by pH. The pH optimization was done by varying the pH in the range of 2–9 for both the metal ions at 30 mg of adsorbent dosage, 30 min of spin time and 50 mg L−1 of initial metal ion concentration. It was found that adsorption increased by increasing pH, and at pH 5, the adsorption was maximum at 92.3% for Pb2+ and 87% for Cd2+ and practically constant till pH 7 represented in Fig. . The adsorption uptakes at equilibrium were lower under conditions that were highly acidic and became higher as the pH increased; the maximum adsorption efficiency was achieved at pH 5. This behavior can be described on the basis of zero point charge (pHpzc) of the CAS, which was determined to be at pH 4.41. The H+ ions effectively competed with the Pb2+ and Cd2+, which caused the uptake to be lower at the pHpzc and at pH less than the pHzpc. However, at pH higher than the pHzpc, the surface of the CAS became negatively charged and electrostatically adsorbed the positively charged metal ions. Similar type of observation has been reported for banana peel and mango peel [Citation7, Citation8]. The percentage removal of the adsorbent increased with increase in pH. The low level of metal ion uptake by the sorbent at lower pH values could be attributed to the increased concentration of hydrogen (H+) ions which compete along with Pb2+ and Cd2+ ions for binding sites on the adsorbent mass. As low pH, the overall surface charge on the CAS becomes positive, which will inhibit the approach of positively charged metal cations. At pH values above the isoelectric point, there is a net negative charge on the surface and the ionic point of ligand such as carboxyl, hydroxyl and amino groups are free so as to promote interaction with the metal cations. The metal uptake capacity of the adsorbent depends on its nature, its own pH, and the pHPZC of CAS is 4.41 Citation[17]. This would lead to electrostatic attractions between positively charged cations such as Pb2+ and Cd2+ and negatively charged binding sites.
3.2 Effect of adsorbent dosage
Adsorbent dosage is one of the important parameter studied while conducting batch adsorption studies. The effect of adsorbent dosage on removal of Pb2+ and Cd2 was studied by varying dosage from 10 to 60 mg g−1 as shown in Fig. .
Fig. 2 Effect of adsorbent dosage on adsorption of Pb2+ and Cd2+ by CAS, (pH 5.1, 50 mg L−1, 30 min).
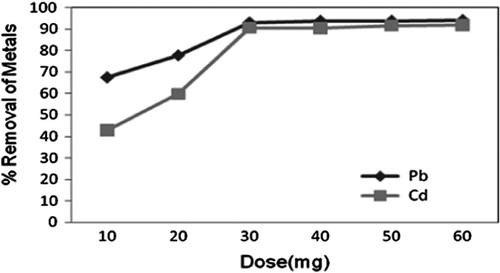
It was found that adsorption increased with increase in mass of adsorbent. Maximum removal efficiency of 93.08% for Pb2+ (from initial concentration of 50 mg L−1) and 71.0% for Cd2+ (from initial concentration of 50 mg L−1) was observed when 30 mg of CAS was used.
3.3 Effect of contact time
The adsorption capacity of CAS towards Pb2+ and Cd2+ as a function of time at different initial concentrations was studied. Increase in agitation time increased the uptake of metal ions and reached equilibrium. The results are shown in Fig. . Initial rapid phase may be due to the increased number of active sites, later the process becomes relatively slower and equilibrium condition reached within 30 min. At this point, the amount of metal ion adsorbed from the adsorbent is in a state of dynamic equilibrium with the amount of metal ion being adsorbed onto the adsorbent. The steps involved in the sorption, the first rapid and quantitatively predominant and the second slower and quantitatively insignificant, has been extensively reported in the literature [Citation18–Citation20]. The data obtained were further used to evaluate the kinetic parameters of the adsorption process.
3.4 Effect of initial metal ion concentration
It was found that adsorption decreased with increase in initial metal ion concentration. Maximum removal efficiency of 93.08% for Pb2+ (from initial concentration of 50 ppm) and 71.0% for Cd2+ (from initial concentration of 50 ppm) was observed when 30 mg of CAS was used. Generally, there are several adsorption steps involved in the transfer of a solute in adsorption dynamics: the movement of the adsorbate molecules from the bulk solution to the external surface of the adsorbent (film diffusion), the movement of the adsorbate molecules to the interior regions of the adsorbent (particle diffusion) and the sorption of the solute on the interior surfaces of the pores of the adsorbent. An increase in the uptake rate to the maximum adsorption capacities was observed at lower concentrations of metal ions, which demonstrated that the uptake of metal ions by CAS surface was very fast Fig. .
3.5 Kinetics of adsorption
The kinetics of the adsorption is determined by the rate of adsorbed metal to that of the adsorbate and the equilibrium for the adsorption process. The adsorption data were analyzed using pseudo-first- and pseudo-second-order kinetics model. The data derived from the kinetic models are given in Table . The rate constant of the adsorption was also derived using the pseudo-first- and pesudo-second-order equation.The pseudo first order reaction can be given as:(3)
Table 1 Pseudo-second-order kinetic for Pb2+ and Cd2+ parameters with experimental values obtained at 303 k
where qe and q are the amount of Pb2+ and Cd2+ adsorbed (mg g−1) at equilibrium and at time t (minutes), respectively, and k1 is the Lagergren equilibrium rate constant of pseudo-first-order equation (min−1). From the data, it was observed that it follow pseudo-second-order rate kinetics. The equation is as represented below(4)
where k2 is the equilibrium rate constant (g mg−1 min−1) of pseudo-second-order rate equation. The qe and k2 values were obtained from the slope and intercept of the linear plot of t/q vs. time. The theoretical values are given in Table the R2 values for pseudo-second-order rate equation were 0.99, and the calculated qe values are in agreement with the experimental values for both metals.
3.6 Adsorption equilibrium studies
In order to evaluate the maximum loading capacity of CAS, the sorbent was allowed in contact with varying concentration (50–200 mg L−1) of Pb2+ and Cd2+ metal solutions. Metal loading capacity of CAS was observed to increase with increasing metal ion concentration. It was found that maximum loading capacity of CAS was 90.93 and 71.0 mg g−1 for Pb2+ and Cd2+, respectively. Two well-known isotherm Langmuir and Freundlich isotherm models were used to examine the relationship between the concentration of metal ion at equilibrium (Ce) and metal loading capacity (qe). The nonlinear form of Langmuir equation after rearrangement is given as(5)
The Freundlich and Langmuir model constants for Pb2+ and Cd2+ after adsorption onto CAS are discussed in Table . Hence the adsorption of Pb2+ and Cd2+ on CAS powder followed second–order kinetic model. The Adsorption kinetics and their modeling at different initial concentrations are represented in Fig. .
Table 2 Freundlich and Langmuir model constants for Pb2+ and Cd2+ adsorbed onto CAS
Fig. 5 Adsorption kinetics and their modeling at different initial concentrations, (50, 100 mg L−1 pH 5.1, 30 mg, 30 min).
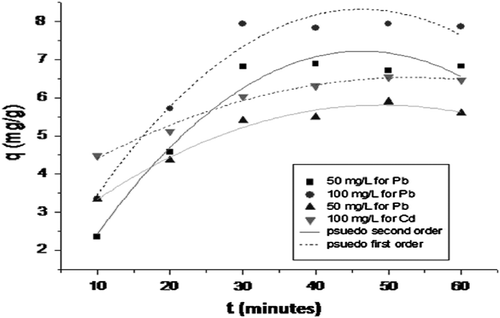
The nonlinear form of Freundlich adsorption isotherm is given as(6) where Ce is the metal ion concentration at equilibrium (mgL−1), qe is the adsorption capacity at equilibrium (mg g−1), qm is the maximum adsorption capacity (mg g−1), KL is the Langmuir constant (L mg−1), KF is the Freundlich adsorbent capacity, nF is the heterogeneity factor. The experimental equilibrium data obtained were fitted with Langmuir (Fig. ) and Freundlich (Fig. ) isotherm models, respectively. It was found that adsorption of Pb2+ and Cd2+ on CAS fits better with Langmuir model than the Freundlich. The better fit is further supported by respective correlation coefficients. The R2 values in respect to sorption of Pb2+ was noted to be 0.978 and 0.899, respectively, for Langmuir and Freundlich isotherms and values for Cd2+ were 0.991 and 0.844 correspondingly. The theoretical complete monolayer coverage of Pb2+ and Cd2+ on CAS was calculated as 91.74 and 73.29 mg g−1, respectively, against 90.93 and 71.0 mg g−1 found experimentally. The sorption capacity of CAS for Pb2+ and Cd2+ was significantly higher than many other sorption capacities of agricultural materials that have been reported in the literature (Table ).
Table 3 Comparison of maximum adsorption capacity of some biosorbents
3.7 FTIR analysis
The sorption of metals onto sorbent materials is attributable to the active groups and bonds present on them Citation[18]. In order to identify the major functional groups present in CAS preliminary quantitative analysis was done with FTIR spectroscopy. FTIR spectra Fig. of CAS displayed a number of peaks pertaining to different functional groups. The wide band observed at 3,623 cm−1 indicates that free and intermolecular bonded O–H groups were present, whereas the band at 2,924 cm−1 was assigned as the stretching vibration of the C–H groups that were present in the lignin structure Citation[19]. The band observed at approximately 1,656 and 1,529 cm−1 indicated that C=C bonds of aromatic rings were present. The band at 1,037 cm−1 was attributed to deformation of CH3 groups [Citation20, Citation21]. After adsorption of Pb2+, the weak interactions between the CAS surface and Pb2+ and Cd2+ was evidenced by a shift in the O–H and C=O bands from 3,623 and 1,656 cm−1 to 3,525 and 1,707 cm−1 for Pb2+ and 3,446 and 1,629 for Cd2+, respectively. These results provide strong evidence for the adsorption of Pb2+ and Cd2+ onto the CAS surface.
3.8 Energy dispersive X-ray analysis
Elemental analysis of CAS before and after sorption of Pb2+ and Cd2+ was done by energy dispersive X-ray analysis (EDX). Fig. , , and show the EDX pattern of CAS before and after sorption of Pb2+ and Cd2+. The EDX pattern of CAS Fig. before sorption shows the presence of calcium (Ca) and magnesium (Mg) signals and the absence of Pb2+ and Cd2+ signals Citation[22]. After sorption diminished peak of Mg and Ca and the presence of Pb2+ in Fig. and Cd2+ Fig. was observed. These findings on EDX analysis clearly indicate the mechanism involved is ion exchange for the removal of metals by CAS. The surface structure of CAS before and after Pb2+ and Cd2+ adsorption was analyzed using field-emission scanning electron microscopy (FE-SEM). As shown in Fig. , the surface of CAS was smooth before adsorption; however, the surface was moldy, crude and appeared to bulge after adsorption of Pb2+ in Fig. and Cd2+ in Fig. , which indicates that the CAS surface has a higher tendency to trap and adsorb the Pb2+ and Cd2+.
3.9 Effect of temperature
The adsorption of metal ions was studied at four different temperatures in the range 293–323 k using CAS as an adsorbent and at the metal ion concentration of 100 mg/L. The experimental result showed that the adsorption capacity increases with increase in the solution temperature. The enhanced sorption at higher temperature indicates endothermic adsorption. It is cleared from Table that ΔG° values were found to be negative which indicates that the adsorption efficiency of Cd2+and Pb2+ onto CAS increases with increase in temperature. The negative values of ΔG° confirm the feasibility of the process and the spontaneous nature of adsorption. The Gibbs free energy change of the adsorption process is related to the equilibrium constant by the classical Van’t Hoff equation(7) The Gibbs free energy change is also related to the entropy change and heat of adsorption at constant temperature according to the following equation
(8)
Table 4 Thermodynamic parameters of the adsorption of Pb2+ and Cd2+ ions by CAS
4 Desorption and regeneration of CAS
Desorption and regeneration of the biosorbent is of crucial importance in assessing its potential for commercial applications Citation[23]. In order to make the sorption process most economical, desorption and regeneration potential of CAS was investigated for four times using 0.1 M HCl as desorbing agent. For the study of desorption, 0.1 g of Pb2+ and Cd2+ loaded CAS was kept in contact with 20 mL of 0.1 M HCl for 30 min and desorbed acidic solution was subjected to Atomic Absorption spectrometer to determine the metal concentration. Desorption of Pb2+ and Cd2+ from the metal loaded CAS resulted in 97.6 and 98.7% metal recovery for first cycle, respectively. The efficiency remained almost unchanged during three repeated cycles for both the metal ions and then gradually reduced for the fourth and fifth cycles as shown in Fig. .
5 Conclusions
The present study shows CAS powder can be used as an inexpensive sorbent for the sorption of Pb2+ and Cd2+ from aqueous solution. Biosorption of Pb2+ and Cd2+ by CAS were fast, with equilibrium reached in 30 min and following pseudo-second-order kinetics. The maximum loading capacity (qe) of CAS was found to be 90.93 and 71.0 mg g−1 for Pb2+ and Cd2+ respectively, and perfect fit to Langmuir isotherm model with correlation coefficients R2 > 0.995. The thermodynamic studies revealed that the adsorption is spontaneous and endothermic for Cd2+ and Pb2+ ions onto CAS. The increase in sorptive uptake of the metal ions with an increase in temperature may be attributed to chemisorption. Complete desorption and regeneration of the sorbent for three cycles and partial regeneration in the fourth and fifth cycle showed that the material is an excellent sorbent and process involved in uptake of metals was ion exchange which also evidenced from EDX patterns of sorbent before and after sorption. From these observations, it can be concluded that CAS can be used as a nonhazardous material for removal of heavy metals from aqueous solution.
Acknowledgements
The authors wish to thank VIT University and Dr. G. Bhaskar Raju, Director in Charge NML Madras Centre, CSIR, for his technical support during the course of work.
References
- S. Chakravarty, M. Ashok, T. Nag Sudha, A.K. Upadhyay, J. Konar, J.K. Sircar, A. Madhukar, K.K. Gupta, Removal of Pb (II) ions from aqueous solution by adsorption using bael leaves (Aegle marmelos). J. Hazard. Mater. 173 (2010) 502–509.
- H. Benhima, M. Chiban, F. Sinan, P. Seta, M. Persin, Removal of lead and cadmium ions from aqueous solution by adsorption onto micro-particles of dry plants. Colloids Surf. B. 61 (2008) 10–16.
- S. Marina, R. Bogdanka, K. Zarko, K. Mile, Adsorption of heavy metals from electroplating wastewater by wood sawdust. Bioresource Technol. 98 (2007) 402–409.
- S. Dhiraj, M. Garima, M.P. Kaur, Agricultural waste material as potential adsorbent for sequestering heavy metal ions from aqueous solutions—a review. Bioresource Technol. 99 (2008) 6017–6027.
- F. Fenglian, Q. Wang, Removal of heavy metal ions from wastewaters: a review. J. Environ. Manage. 92 (2011) 407–418.
- M. Iqbal, A. Saeed, S.I. Zafar, FTIR spectrophotometry, kinetics and adsorption isotherms modeling, ion exchange, and EDX analysis for understanding the mechanism of Cd2+ and Pb2+ removal by mango peel waste. J. Hazard. Mater. 164 (2009) 161–171.
- S. Liang, X. Guo, N. Feng, Q. Tian, Isotherms, kinetics and thermodynamic studies of adsorption of Cu2+ from aqueous solutions by Mg2+/K+ type orange peel adsorbents. J. Hazard. Mater. 174 (2010) 756–762.
- B.S. Inbaraj, N. Sulochana, Carbonised jackfruit peel as an adsorbent for the removal of Cd (II) from aqueous solution. Bioresource Technol. 94 (2004) 49–52.
- A. Flavio Pavan, C. Mazzocato Ana, A. Rosangela Jacques, L.P. Silvio Dias, Ponkan peel a potential biosorbent for removal of Pb(II) ions from aqueous solution. Biochem. Eng. J. 40 (2008) 357–362.
- E.S.Z. El-Ashtoukhy, N.K. Amin, O. Abdelwahab, Removal of lead (II) and copper (II) from aqueous solution using pomegranate peel as a new adsorbent. Desalination 223 (2008) 162–173.
- R.A.K. Rao, S. Ikram, Sorption studies of Cu(II) on gooseberry fruit (emblica officinalis) and its removal from electroplating wastewater. Desalination 277 (2011) 390–398.
- J.C.P. Vaghetti, E.C. Lima, B. Royer, J.L. Brasil, B.M. da Cunha, N.M. Simon, N.F. Cardoso, C.P.Z. Noreña, Application of Brazilian-pine fruit coat as a biosorbent to removal of Cr (VI) from aqueous solution: kinetics and equilibrium study. Biochem. Eng. J. 42 (2008) 67–76.
- G.H. Sonawanea, V.S. Shrivastava, Orange-II removal from simulated wastewater by adsorption using Annona squamosa shell—a kinetic and equilibrium studies. Desalin. Water Treat. 36 (2011) 374–382.
- S. Asma, I. Muhammad, H.H. Wolfgang, Kinetics, equilibrium and mechanism of Cd2+ removal from aqueous solution by mungbean husk. J. Hazard. Mater. 168 (2009) 1467–1475.
- V.C. Srivastava, I.D. Mall, I.M. Mishra, Characterization of mesoporous rice husk ash (RHA) and adsorption kinetics of metal ions from aqueous solution onto RHA. J. Hazard. Mater. B 134 (2006) 257–267.
- R. Gnanasambandam, A. Protor, Determinations of pectin degree of esterification by diffuse reflectance Fourier transform infrared spectroscopy. Food Chem. 68 (2000) 327–332.
- A. Bhatnagar, A.K. Minocha, M. Sillanpaa, Adsorptive removal of cobalt from aqueous solution by utilizing lemon peel as biosorbent. Biochem. Eng. J. 48 (2010) 181–186.
- M.E. Argun, S. Dursun, M. Karatas, M. Gürü, Activation of pine cone using Fenton oxidation for Cd(II) and Pb(II) removal. Bioresource Technol. 99 (2008) 8691–8698.
- D. Zhang, X. Zeng, W. Lia, H. He, P. Ma, J. Falandysz, Selection of optimum formulation for biosorbing lead and cadmium from aquatic solution by using PVA-SA’s immobilizing Lentinus edodes residue. Desalin. Water Treat. 31 (2011) 107–114.