Abstract
The phenomenon of rutting is one of the most serious problems on asphalt pavements. Decreasing the surface temperature of the asphalt pavement is an effective method to solve the rutting problem on asphalt pavements. In this study, a nano-sized-particles-filled polymer composite was developed as an overlay to reflect solar energy and decrease the surface temperature of asphalt pavements. The overlay was composed of acrylic or epoxy resin filled with nano TiO2 or nano TiNO2. The solar reflection of the nanoparticle-filled polymers was tested, and the results showed that solar reflection effectiveness of the TiO2/acrylic composite reached the highest value. The results of outdoor temperature tests indicate that the solar-reflective overlay could decrease the surface temperature of asphalt pavements by about 10°C when the pavement temperature is about 60°C.
1. Introduction
In urban areas, rutting is one of the most serious damages in asphalt pavements, especially in recent years, and with many overloaded vehicles and increasing temperatures in summer, this problem is becoming more obvious in the transportation field [Citation1–3 Citation Citation3]. Of all the reasons, high-surface temperature is considered to be the main reason for pavement rutting. Asphalt binder will become soft if the temperature is higher than its softening point [Citation4], which will lead to a decrease in the bearing property of asphalt concrete. In previous studies, it was found that rutting can be largely controlled in several millimeters level if the surface pavement temperature is set lower than 55°C, whereas it will be sharply increased if the surface pavement temperature is increased from 55°C to 65°C [Citation5]. Therefore, how to decrease the surface temperature has become a key issue to improve the anti-rutting properties of asphalt pavements.
Nanotechnology is the creation of new materials, devices, and systems at the molecular level so as to induce desirable improvements in macroscopic material properties [Citation6]. Despite potential cost and health concerns over the use of nano-sized particles, recent years have seen increased research and development in the use of nanotechnology to remarkably enhance the properties of construction materials (e.g., coatings, concretes, asphalts, and steels) [Citation7]. Most applications of nanotechnology for asphalt pavements were carried out by adding nano-sized particles to polymer-modified asphalt or to asphalt directly [Citation8–10 Citation Citation10], while nano-sized particles were rarely added in the coating as the overlay for asphalt pavement. Moreover, nano-modified asphalt improved its thermal performance by increasing its anti-high-temperature capacity, namely, the high temperature was passively accepted and the inner temperature field of asphalt pavements was not changed. However, solar-reflective coating serves to decrease the temperature of asphalt pavement, that is, the working and inner temperatures of asphalt pavements were decreased. Therefore, solar-reflective coating is a new and promising method to decrease the temperature of asphalt pavement actively.
Pavements absorb solar radiation, store this energy in the pavement subsurface, manifested as the increase of temperature, and release it as infrared radiation and through convection to their surrounding area during the evening and nighttime [Citation11]. Therefore, decreasing the absorption of solar radiation of pavement can lead to a decrease of pavement temperature. Solar-reflective coating is an active technique that helps lower surface temperatures and reduces the amount of heat absorbed into the pavement. Cool materials are characterized by solar reflectance or albedo (the percentage of solar energy reflected by a surface), because it is the main determinant of a material's maximum surface temperature [Citation12–14 Citation Citation14]. Solar reflectance is largely governed by its color, and it was found that a white color coating has a better thermal performance than other color coatings [Citation15–17 Citation Citation17]. Uemoto [Citation15] pointed out that the reflectance decreases with the color changing from light to dark. Solar reflectance is also governed by its surface roughness [Citation17]; it was found that rough surfaces tend to absorb more solar radiation than smooth and flat surfaces. Although increasing solar reflectance of pavements can decrease the surface temperatures of pavements, it leads to potential safety problems for drivers by introducing glare problems. Recently, Kinouchi [Citation18] had developed a new type of pavement coating that can satisfy both high solar reflectance and low glaring problem. This pavement coating is characterized by low solar reflectance in visible spectrum (23%) and high solar reflectance in near-infrared spectrum (86%). Akbari [Citation19] reported that increasing pavement's solar reflectance by 0.25 will significantly decrease the asphalt pavement temperature by 10°C.
In this study, solar heat reflective coatings were developed to lower the surface temperature of asphalt pavements. These coatings are able to highly reflect the visible light, thus decreasing the solar energy absorption and leading to a decrease in the surface pavement temperatures.
2. Experimental
Epoxy and acrylic polymers were supplied by Shanghai Juxing Polymer Co. (Shanghai, China) as the coating matrix materials. Briefly, TiO2 nanoparticles were prepared by oxidation of titanium precursor at high temperature in the reaction system. TiNO2 nanoparticles were prepared by a similar process by blowing N2 gas into the reaction system.
Scanning electron microscope (SEM) images were obtained with a FEI-Quanta 200F electron microscope, which was used for investigating the feature and size of nanoparticles. The SEM morphology of nano-TiO2 and nano-TiNO2 particles is shown in As can be seen in this figure, the TiO2 and TiNO2 particles are both spherical shaped with diameters of about 75 and 60 nm, respectively. The self-dispersion of the nano-sized TiNO2 is better than the nano-sized TiO2, which is agglomerate with an average diameter of about 300 nm to 1 μm.
The acrylic/nanoparticle compound was prepared by mixing both polymer and filler with weight fractions of 0%, 0.1%, 0.3%, 0.5%, 0.7%, and 0.9%. During the process, the compound was ultrasonic-dispersed for 30 minutes at room temperature, followed by magnetic stirring for 5 minutes at room temperature. After the process, a curing agent was added for another 3-minute magnetic stirring. Finally, the mixture was poured into a mold and then cured for about 12 hours.
The epoxy/nanoparticle compound was prepared by mixing both polymer and filler with the filler's weight fractions of 0%, 0.1%, 0.3%, 0.5%, 0.7%, and 0.9%. Due to the high viscosity of epoxy resin at room temperature, the compound was ultrasonic-dispersed for 30 minutes, followed by magnetic stirring for 5 minutes at 80°C rather than at room temperature. After the process, a curing agent was added for another 3-minute magnetic stirring. Finally, the mixture was poured into a mold and then cured for about 12 hours.
shows the SEM image of the fracture surfaces of the composites. As can be seen in this figure, there is no obvious agglomeration of the TiO2 and TiNO2 particles, which represents a well dispersion of the nanoparticles.
Asphalt concrete specimens with size of 300 × 300 × 50 mm3 were used as substrates for temperature tests and skid-resistance tests. The coatings were prepared by the above method and brushed on the surface of the asphalt concrete specimen. The final thickness of the coated films was about 1 mm for temperature test. For the skid-resistance test, the coatings were added with quartz sands, which were of diameters from 1 mm to 3 mm. The samples taken for the UV/VI/IR reflection spectra tests were of dimensions φ30 mm × 15 mm, which have no substrates.
Reflection spectra tests were tested on Lambda 900 (PerkinElmer) with the range from 200 to 2500 nm. In order to study the solar reflectance of the coatings, the surface temperatures of the samples were measured for 24 hours. In the temperature tests, the light source was natural sunlight and the environmental conditions were clear sky and low wind speed (<4 m/s). The average ambient temperature was about 27°C in the daytime and 16°C in the nighttime. The temperature sensors were placed under 25 mm of the surface at the center of the specimen. In view of traffic safety, the British Pendulum Number (BPN) and the sand patch method were adopted to evaluate the skid resistance of the samples.
3. Results and discussion
The wavelength of solar wave with most of the heat is mainly in the range of 250–2500 nm. As reported by the Berkeley Lab Heat Island Group, the solar energy mainly comes from ultraviolet, visible lights, and IR with ratios of 5:43:52 [Citation20].
shows the spectral reflectance of asphalt. Due to the black color, the average solar reflectance of asphalt is very low, which is approximately 5%, that is, nearly 95% solar energy was absorbed by asphalt and results in an increase in the temperature.
For the solar-reflective coating, the total reflectance is composed of the reflectance of the matrix and the fillers, respectively. For the matrix polymer materials, lower absorption of visible lights and near-infrared lights should be used; therefore, functional groups such as C-O-C, C=O, and -OH should be avoided [Citation21]. Meanwhile, solar reflectance can be influenced by the material type, weight fraction, and particle size of the fillers.
shows the solar reflectance of pure epoxy and acrylic resins. It was found that the solar reflectance of epoxy resin was higher in the visible lights spectrum, while acrylic was higher in the near-infrared spectrum. The maximum solar reflectance of pure acrylic and epoxy was 30.9% and 14.9%, respectively. The reflected radiation of pure acrylic resin and epoxy resin was 10.5% and 7.4%, respectively.
shows the reflectance as a function of wavelength of epoxy resin filled with different weight fractions of nano-TiO2 and TiNO2, respectively. As shown in this figure, the reflectance increases with increasing weight fraction of nanoparticle fillers. shows the reflectance as a function of wavelength of TiO2 and TiNO2 with weight fractions of 0.1, 0.3, 0.5, 0.7, and 0.9. It can be found that the maximum reflectance of TiO2/epoxy was 76.2% and the average reflectance was 33.0% with 0.9 wt. % of nano-TiO2, while the maximum solar reflectance of TiNO2/epoxy was 69.3% and the average reflectance was 27.4% with 0.9 wt. % nano-TiNO2.
Figure 5. Spectral reflectance of epoxy resin matrix with different weight fractions of (a) TiO2 and (b) TiNO2.
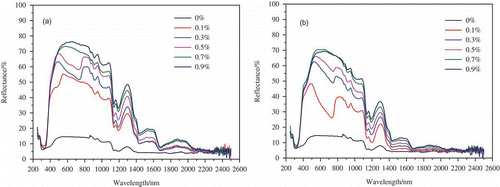
Figure 6. Reflectance of epoxy resin matrix with weight fraction of TiO2 and TiNO2 (a) 0.1%, (b) 0.3%, (c) 0.5%, (d) 0.7%, and (e) 0.9%.
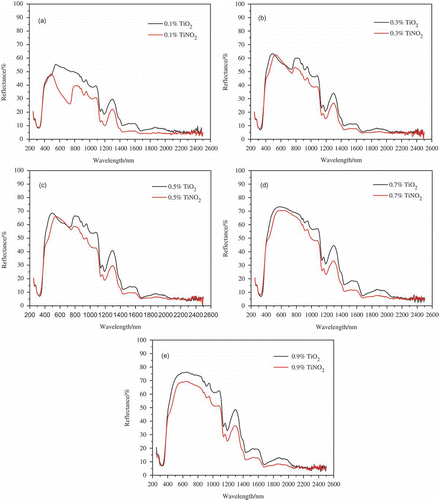
shows the reflectance as a function of wavelength of acrylic resin filled with different weight fractions of nano-TiO2. As shown in this figure, the reflectance increases with increasing weight fraction of nanoparticle fillers. It can be found that the maximum reflectance of TiO2/acrylic was 79.8% and the average reflectance was 36.0% with 0.9 wt. % of nano-TiO2.
shows the reflectance of epoxy and acrylic matrices with 0.7% nano-TiO2.
The result shows that the solar reflectance of epoxy resin is higher in the visible lights than acrylic resin, while acrylic is higher in near-infrared than the epoxy resin. From an economic perspective, 0.7 wt. % was chosen as the final weight fraction of the coatings.
shows the temperature variation in a 24-hour cycle. The results show that the temperatures of the samples are epoxy>asphalt concrete>TiO2/epoxy>TiNO2/epoxy. It was also shown that the temperature of the epoxy resin with nano-TiNO2 particles was lower than that with nano-TiO2 particles, which might result from high solar reflectance or good dispersion of nano-TiNO2 particles. Besides, it was also found that the temperature of pure epoxy was higher than that of asphalt concrete (no coatings). It resulted from the transparent color and molecule structure of epoxy; therefore, the asphalt concrete sample coated with pure epoxy could be seen as a black coating which was sensitive to temperature, and also the sample with the coating might be endowed with low thermal emittance; hence, it was hard for the sample to radiate heat and keep the temperature high. Among these materials, the maximum temperature of TiNO2/epoxy was 12.9°C lower than that of asphalt concrete. In this study, the average surface temperatures were tested as well; the temperatures were about 5°C lower than those tested at the point under 25 mm of the surface.
shows the related skid-resistance parameters of conventional asphalt concrete, asphalt concrete coated with epoxy resin and quartz sands, asphalt concrete coated acrylic resin and quartz sands. The value of BPN, which should be greater than 45 when the samples are under wet condition, reflected the approximate size of pavement microtexture; the mean macro-texture depth (MTD) was measured to assess the macrotexture of pavement through the sand patch method. Numerous studies have revealed that the wet pavement accident rate decreased with increased BPN values, and a pavement surface is considered rough if the average depth of the macrotexture is more than 1.0 mm [Citation22]. According to the data shown in , the skid assistance of asphalt concrete coated with epoxy resin and acrylic resin was qualified and was even better than that of conventional asphalt concrete.
Table 1. Average values of BPN and SN for tested samples
4. Conclusions
High temperature has been considered as the main reason for rutting of asphalt pavements; therefore, solar-reflective coatings were developed to decrease the surface temperatures by increasing heat reflection from the pavement. In this study, four types of reflective coatings, epoxy or acrylic filled with TiO2 or TiNO2 nanoparticles, were investigated. Among these, the temperature drop range of TiO2/acrylic was 12.9°C, which might prevent pavement rutting significantly. The reflectance spectrum shows that TiO2/acrylic reaches the maximum solar reflectance of up to 79.8%. From the reflected solar energy perspective, TiO2/acrylic is also the best choice, whose reflected solar energy ratio was 36.0%. From the statistical analysis perspective, it was found that additional TiO2 nanoparticles to substrates significantly lead to an improvement of solar reflectance of coating in visible light spectrum. The addition of TiNO2 nanoparticles effectively lowered the surface temperature of asphalt pavement, likely due to better dispersion of the nanoparticles. In conclusion, TiO2/acrylic is suggested as a potential reflective coating candidate in this study.
Acknowledgements
This work was financially supported by the project (HIT. NSRIF. 2009100) of Natural Scientific Research Innovation Foundation in Harbin Institute of Technology and Project (HIT.KLOF.2009105) of Key Laboratory Opening Funding of Special Materials Lab on Transportation Safety and project of China Postdoctoral Science Foundation (no. 20110491065).
References
- Balghunaim , F. , Al-Dhubaib , I. , Khan , S. , Fatani , M. , Al-Abdulwahhab , H. and Babshait , A. Pavement rutting in the Kingdom of Saudi Arabia: a diagnostic approach to the problem . Proceedings of 3rd IRF Middle East Regional Meeting Vol. , 6, Ministry of Communications, Saudi Arabia, 1988 pp. 210 – 232 .
- Abdulshafi , A. 1988 . Rutting-review of existing models and some application to Saudi Arabia . Proceedings of 3rd IRF Middle East Regional Meeting Vol. , 6 : pp. 244 – 256 . Ministry of Communications, Saudi Arabia,
- Mahrez , A. 2011 . A review on fatigue and rutting performance of asphalt mixes . Sci. Res. Essays , 6 : 670 – 682 . M. Taher Baghaee, K. Mohamed Rehan, and
- Zare-Shahabadi , A. , Shokuhfar , A. and Ebrahimi-Nejad , S. 2010 . Preparation and rheological characterization of asphalt binders reinforced with layered silicate nanoparticles . Constr. Build. Mater. , 24 : 1239 – 1244 .
- Sirin , O. , Kim , H.-J. and Tia , M. 2008 . Bouzid Choubane. Comparison of rutting resistance of unmodified and SBS-modified Superpave mixtures by accelerated pavement testing . Constr. Build. Marter. , 22 : 286 – 294 .
- K.P. , Chong , ed. Nanotechnology and information technology in civil engineering Conference Proceeding – Towards a Vision for Information Technology in Civil Engineering. In 4th Joint International Symposium on Information Technology in Civil Engineering, 15–16 November 2003, Nashville, TN, Ian Flood, ed., 2004, ASCE, Nashville, TN, pp. 1–9
- Shi , X. , Nguyen , T.A. , Suo , Z. , Liu , Y. and Avci , R. 2009 . Effect of nanoparticles on the anticorrosion and mechanical properties of Epoxy Coating . Surf. Coat. Tech. , 204 : 237 – 245 .
- You , Z. , Mills-Beale , J. , Foley , J.M. , Roy , S. , Odegard , G.M. , Dai , Q. and Goh , S.W. 2011 . Nanoclay- modified asphalt materials: preparation and characterization . Constr. Build. Mater. , 25 : 1072 – 1078 .
- Amirkhanian , A.N. and Amirkhanian , S.N. 2011 . Influence of Carbon Nanoparticles on the rheological characteristics of short-term aged asphalt binders . Am. Soc. Civil Eng. , F. Xiaodoi:10.1061/(ASCE)MT.1943–5533.0000184
- Shi , X. , Goh , S.W. , Akin , M. , Stevens , S. and You , Z. 2011 . Exploring the interactions of chloride solution with nano/micro-modified asphalt mixtures using artificial neural networks . J. Mater. Civil Eng. , doi:10.1061/(ASCE)MT.1943–5533.0000452
- Santamouris , M. , Synnefa , A. and Karlessi , T. 2011 . Using advanced cool materials in the urban built environment to mitigate heat islands and improve thermal comfort conditions . Solar Energy , 85 : 3085 – 3102 .
- What Factors Influence Elevated Pavement Temperatures Most During Day and Night? National Center of Excellence on SMART Innovations at Arizona State University, Case Study 1 (2007), p. 1
- Asaeda , T. and Ca , V.T. 1996 . Heat storage of pavement and its effect on the lower atmosphere . Atmos. Environ. , 30 : 413 – 427 .
- Synnefa , A. , Santamouris , M. and Livada , I. 2006 . A study of the thermal performance of reflective coatings for the urban environment . Solar Energy , 80 : 968 – 981 .
- Doulos , L. , Santamouris , M. and Liviada , I. 2004 . Passive cooling of outdoor urban spaces. The role of materials . Solar Energy , 77 : 231 – 249 .
- Sunnefa , A. , Santamouris , M. and Livada , I. 2006 . A study of the thermal performance of reflective coatings for the urban environment . Solar Energy , 80 : 968 – 981 .
- Uemoto , K.L. , Neide , M.N.S. and Hohn , V.M. 2010 . Estimating thermal performance of cool colored paints . Energy. Buildings , 42 : 17 – 22 .
- Kinouchi , T. , Yoshinaka , T. , Noriyuki , F. and Kanada , M. Development of cool pavement with dark colored high albedo coatings and In Fifth Conference for the Urban Environment, Vancouver, Canada, 2004, p. 38
- Akbari , H. , Pomerantz , M. and Taha , H. 2001 . Cool surfaces and share trees to reduce energy use and improve air quality in urban areas . Solar Energy , 70 : 295 – 310 .
- Reducing Urban Heat Islands: Compendium of Strategies Cool Pavements. Available at http://www.epa.gov/heatisld/resources/pdf/GreenRoofsCompendium.pdf (http://www.epa.gov/heatisld/resources/pdf/GreenRoofsCompendium.pdf)
- Su , B. , Liu , X. , Peng , X. , Xiao , T. and Su , Z. 2003 . Preparation and characterization of the TiO2/polymer complex nanomaterial . Mater. Sci. Eng. A. , 349 : 59 – 62 .
- Kokkalis , A.G. and Panagouli , O.K. Fractal evaluation of pavement skid resistance cariations. I: Surface wetting . Chaos Soliton. Fract. , 9 ( 1998 ) 1875 – 1890 .