ABSTRACT
The frequency dependence of scattering parameters of interdigital surface acoustic wave transducers placed on ferroelectric barium titanate (BaTiO3) epitaxial film in c-phase coated over magnesium oxide has been studied using the finite-element method (FEM) approach along with the perfectly matched layer (PML) technique. The interdigital transducer which has a comb-like structure with aluminum electrodes excites the mechanical wave. The distance between the fingers allows tuning the frequency properties of the wave propagation. The magnesium oxide is taken as the substrate. The two-dimensional model of two-port surface acoustic wave filter is created to calculate scattering parameters and to show how to design the fixture in COMSOLTM. Some practical computational challenges of finite element modeling of SAW devices in COMSOLTM are shown. The effect of lattice misfit strain on acoustic properties of heterostructures of BaTiO3 epitaxial film in c-phase at room temperature is discussed in present article for two low-frequency surface acoustic resonances.
1. Introduction
The devices on surface acoustic waves (SAW), which use interdigital electrodes, have a wide range of applications [Citation1] in today’s telecommunication systems for real-time analog signals processing in the frequency range from about 10MHz to 10GHz and higher. They are extensively used as filters, delay lines, convolvers, resonators, wireless identification systems (ID tags), etc.
The communication channels evolve towards increasing the data rate many times over, therefore the need for considerably higher operating frequencies. It is very attractive purpose of modern acoustoelectronics. To achieve desirable properties of developing acoustic devices, it is required applying the sound-conducting substrates with a higher sound velocity and decreasing the geometrical sizes of transmitting and receiving interdigital transducers. However, these ways have limitations [Citation2]. The sound velocity in substrate usually is fixed and it is very complicated to make a gap width between electrodes of interdigital transducer (IDT), converting electrical energy into acoustic energy and vice versa, less than by the lithographic method.
The heterostructures of thin ferroelectric films having a higher electromechanical coupling coefficient may be regarded as an alternative way to develop SAW devices operating at significantly higher frequencies compared to high-speed acoustic devices produced by now.
Recently, thin barium titanate (BT) epitaxial films have been studied extensively due to their potential applications in micro- and nanoelectronic devices [Citation2,Citation3]. The high dielectric constant of BT, the strong dependence of the permittivity on the electric field and low loss suitable for use over a wide frequency and temperature range allow developing compact frequency-tunable microwave devices. However, there is a number of fundamental issues, such as microstructural and compositional inhomogeneities, internal stresses and defects. It limits the potential applications of thin BaTiO3 epitaxial films compared to single crystals or bulk ceramics.
Internal stresses occur in epitaxial films due to the lattice mismatch between film and the substrate. As the mismatch gets larger, the film material may strain to accommodate the lattice structure of the substrate. This is the case during the early stages of film formation. High mechanical stresses at the film-substrate interface also arising from the difference in their thermal expansion coefficients, as well as the occurrence of spontaneous deformation at the phase transition, if the film is deposited at temperatures above the phase transition temperature. The presence of thermal stress can lead to a shift in the temperature of the ferroelectric phase transition in the film, and to change the structure of the low-symmetry phases, which must be considered when designing acoustoelectronic devices.
Large strains in nonlinear materials, such as ferroelectrics, significantly change their properties up to the formation of new ferroelectric phase states. The nonlinear properties of barium titanate lead to changes in the material constants of the linear equations for the piezoelectric effect in thin films. Epitaxy-induced stresses have a noticeable effect on the dielectric responses of BT films. The dependence of lattice misfit strain in BT films on the dielectric and material constants was studied in [Citation4,Citation5]. It is derived based on Landau-Devonshire phenomenological theory.
When SAW is incident on an electrically loaded IDT, the total input wave energy divides into transmitting forward, reflecting and absorbing parts. Therefore IDT can be presented as a scatterer of SAW. A knowledge of the scattering parameters (S-parameters) is obviously important in the developing of electroacoustic devices.
There are a number of papers [Citation6–Citation8] devoted to the scattering parameters and numerical methods used to obtain high-precision results or estimates. The methods, however, need laborious calculations, numerical algorithms programming skills and do not give any physical insight into the issue. Sometimes it would be convenient to create certain piezoelectric models in a specialized simulation software. The popular finite element analysis program comprising various physics and coupled phenomena is cross-platform package COMSOLTM . It is being actively developed and today’s version is 5.2a. The modeling S-parameters issues in the frequency domain are presented insufficiently on the official site ‘comsol.com’ – neither the application gallery nor technical papers. However, there are a number of papers and models that allow understanding how to design the SAW filter to obtain S-parameters in frequency domain, such as ‘Two-port Piezoelectric SAW Device’[Citation9], ‘Piezoacoustic Transducer’[Citation10], ‘Acoustic-Structure Interaction with a Perfectly Matched Layer (PML)’[Citation11], ‘Extraction of Electrical Equivalent Circuit of One Port SAW Resonator using FEM based Simulation’[Citation12], etc.
In this paper, a physical modeling of SAW filter in COMSOLTM is presented. The model is used to obtain numerical results for S-parameters in the frequency domain of heterostructures of BaTiO3 epitaxial film in c-phase at room temperature for two low-frequency surface acoustic resonances. The effect of lattice misfit strain on S-parameters is discussed.
2. Statement of problem
In this paper, we have performed FEM simulation of layered SAW device using the commercial COMSOL[Citation13]. It is mathematically equivalent to the resolution of partial differential equations (PDE) of piezoelasticity in the frequency domain with a given excitation by FEM. The numerical finite-element formulation of the direct FEM used for the full-scale analysis of electromechanical phenomena involved in SAW devices is discussed in [Citation14].
2.1. Geometry
The shows two-dimensional geometry of a typical layered SAW filter considered in the simulation. The filter consists of BT film (areas and
) coated over magnesium oxide substrate (
,
) and IDTs with a finite number of finger pairs fabricated over the film. The film and substrate have the thickness
and
, respectively.
Transducers are a comb-shaped structure of aluminum electrodes (), alternately connected to two bus bars, placed at the two ends of the film surface separated by a space at the length
. One IDT (IDT1, Port1) is called as a transmitter and is used for generation of SAW. The IDT2 (Port2) at the other end for reception of the waves is called as a receiver. The
electrodes, constituting IDT, with thickness
and size
are spaced apart from each other at the distance
. The transducer on the left side has an applied through Port1 voltage
. It produces dynamic strains in the structure and initiates elastic waves that travel along the surface and receive ones by IDT2 to Port2 .
The layered structure is finite and contains absorbing areas. They are denoted by index containing letter ‘a’ and are implemented by the perfectly matched layer (PML) technique [Citation15,Citation16]. The PML allows simulating a model with open boundaries through which electromagnetic and mechanical waves will pass without any reflection.
A boundary in is denoted by the letter “‘‘ with a numeric index for all four sides in clockwise order. Suppose the first boundary is the left one.
The displacements along ,
,
directions are designated as
,
,
. Surface waves propagate in both directions along the coordinate
. The coordinate
is the direction of wave attenuation. The displacement does not depend on
directed from the observer in . In general, there are three components of mechanical displacement due to the anisotropy properties of the ferroelectric material. Let’s assume that the electric potential is denoted by
and is used to describe the electric field
. The vector
allows to define all the mechanical and electrical parameters in the quasi-static approximation. The significant difference between solving in COMSOLTM of the issue and general one implemented in many FEM software is available bias
, because oscillations are not considered as sagittal polarized compared to the classical Rayleigh waves (
).
2.2. Finite-element modeling
The finite-element modeling of piezoelectric material is well explained in [Citation17–Citation19]. The relation between the stress, strain, electric field, and electric displacement field in a stress-charge of a piezoelectric crystal is given by the piezoelectric linear constructive equations. They are governed by the continuum equation of motion, Maxwells equations under the quasi-static assumption, the strain-mechanical displacement relations and proper boundary conditions. In a homogeneous piezoelectric BT film, the stress component at each point depends on the applied electric field. The piezoelectric equations [Citation20] in the time domain can be written in the following form:
where, are components of the stiffness tensor for constant electric field,
are components of the strain tensor,
are components of the piezoelectric tensor,
are components of the stress tensor,
are components of the displacement,
is the mass density,
are components of the electric displacement,
are components of the electric field, and
are components of the permittivity tensor for constant strain.
The equations of motion, in the absence of internal body forces, are:
The components of the strain are defined by
The Equation. 1 may be defined in the weak form or be implemented in COMSOLTM by ‘Solid Mechanics (solid)’, ‘Electrostatics (es)’ and ‘Piezoelectric Effect (pze)’ physics interfaces. The multiphysics couplings interface ‘Piezoelectric Effect’ is active on the ferroelectric domains only and couple the ‘Solid Mechanics’ and ‘Electrostatics’ interface equations solved in these domains via the linear constitutive equations that model the piezoelectric effect by coupling stresses and strains with the electric field and displacement.
In this article, we show the modeling method, which used ready-made physics interfaces. This way is easier to implement and it is more prevalent. When the model geometry was prepared and the materials were assigned to it, the physics interfaces should be configured.
The ‘Solid Mechanics’ interface may be configured at first. It contains the predefined node ‘Linear Elastic Material (lemm)’ which we associated with domains of the substrate () and IDT’s electrodes (
). It adds the equations for a linear elastic solid and an interface for setting the elastic material properties. The node ‘Piezoelectric Material (pzm)’ linked with the film domain (
) defines the piezoelectric material properties in the stress-charge form using the elasticity matrix and the coupling matrix. It is used together with a ‘Piezoelectric Effect (pze)’ multiphysics coupling node and a corresponding node ‘Charge Conservation, Piezoelectric (ccnp)’ in the ‘Electrostatics’ interface.
The vertical boundary surfaces and the bottom edge of the substrate are assigned to the low-reflecting conditions (which implemented by the node ‘Low-Reflecting Boundary (lrb)’) for pressure and shear waves in order to avoid interferences between nonscattered and scattered waves:
where is the stress tensor, the mechanical impedance
is a diagonal matrix, n is the unit normal vector at the boundary, and
and
are the velocities of pressure and shear waves in the material, respectively.
The damping condition also provides the PML associated with areas and
. It applies a complex coordinate scaling to domains, which absorbs all outgoing wave energy in frequency-domain problems, without any impedance mismatch.
Next, we set up the physics interface ‘Electrostatics’. The predefined node ‘Charge Conservation’ associates with areas and
. It adds the equations for charge conservation according to Gauss law for the electric displacement field:
where ,
are the permittivity of free space and the relative permittivity, respectively, P is the polarization vector, which is non-zero only in the film area (
).
The odd input electrodes of IDT1 are the node ‘Terminal (term)’. They have the type ‘Terminated’ with the terminal name ‘1’. The terminal power is W, The initial value for voltage is
V. The even output electrodes of IDT2 have same parameters with the terminal name ‘2’. The remaining electrodes are addressed with ground potential (
V).
The response of a two-transducer SAW device is often expressed in terms of the scattering matrix (
,
,
) or, as usual called, S-parameters, in which
represents transmission from source to load, and vice versa. The parameters
and
are electrical reflection coefficients.
To obtain scattering parameters we used the input and output IDTs, which changing places. It is distinguished by selecting the ‘Activate manual terminal sweep’ in ‘Electrostatics’ physics interface to switch on the sweep and invoke a parametric sweep over the terminals. We enter a sweep parameter name ‘PortName’ to assign a specific name to the variable that controls the terminal number ‘1’ or ‘2’ solved for during the sweep. It should be noted that the sweep parameter name must also be declared as a model parameter. The parameter is usually swept in the study node ‘Frequency Domain’. It adds to ‘Auxiliary sweep’ as specified combination ‘PortName = [1 2]’.
3. Results and discussion
The two-dimensional model of the surface-wave bandpass filter shown in is used to calculate the scattering coefficients. The geometrical parameters are presented in the . They are chosen close to the prototypes that can be manufactured and measured in our department.
Table 1. The parameters of SAW filter model.
The material constants of BaTiO3 are given by [Citation5]. The material properties of aluminum () and magnesium oxide () are taken from the standard material library of COMSOLTM, which at the moment has no bibliography references to the original sources.
Table 2. The material properties of aluminum.
Table 3. The material properties of magnesium oxide.
shows two low-frequency responses of the filter with various lattice misfit strain of the thin film
. The center frequency and bandwidth are almost unchanged for the first resonance ()) because most of the power of the acoustic wave is concentrated in the substrate and the film makes a weak contribution in the filter properties. However, there is the effect of the misfit strain on reflection coefficient
. Upon approaching the boundary of phase transition (the solid curve
, ), the
parameter decreases significantly, which degrades the filter characteristics. The film already begins to affect the filter properties near the lower cutoff frequency of second resonance. As shown in ), the properties change significantly near the phase boundary.
Figure 2. The dependence of scattering parameters (a, c) and
(b, d) on the lattice misfit strain of BaTiO3 film with thickness
for first (a, b) and second (c, d) low-frequency surface acoustic resonances.
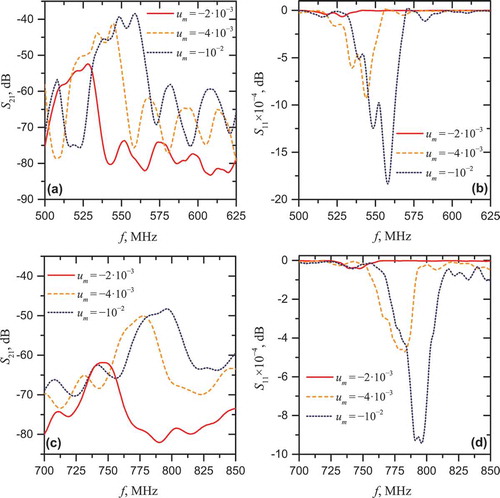
As shown in , the film affects the filter properties if the film is thick enough () to concentrate greater portion of the acoustic wave energy. In this case, when approaching the phase boundary, the central frequency of the resonance shifts to the low-frequency region, the frequency band decreases and the filter attenuation increases.
Figure 3. The dependence of scattering parameters (a, c) and
(b, d) on the lattice misfit strain of BaTiO3 film with thickness
for first (a, b) and second (c, d) low-frequency surface acoustic resonances.
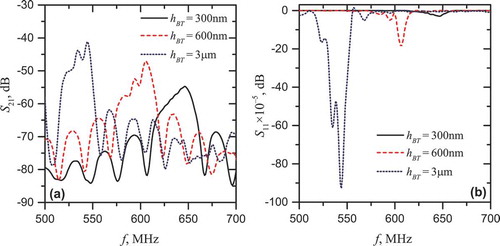
shows the influence of the film thickness on the filter characteristics. If it grows up, the center frequency shifts up, the frequency band and the attenuation also increase. At the same time, the reflection coefficient decreases significantly.
4. Conclusion
We have created a finite-element model in COMSOLTM for full-scale analysis of SAW devices. This two-dimensional model of two-port surface acoustic wave filter allows calculating the frequency dependence of scattering parameters for interdigital surface acoustic wave transducers, which have a comb-like structure, with aluminum electrodes placed on ferroelectric barium titanate film coated over magnesium oxide substrate.
We have used the developed model to study the effect of lattice misfit strain on acoustic properties of heterostructures of BaTiO3 epitaxial film in c-phase at room temperature for two low-frequency surface acoustic resonances. The two low-frequency responses of the filter with various lattice misfit strain of the thin film are presented and the influence of the phase transition boundary on the SAW filter properties is discussed.
The center frequency and bandwidth are almost unchanged for the first resonance because most of the power of the acoustic wave is concentrated in the substrate and the film makes a weak contribution in the filter properties. The film already begins to affect the filter properties near the lower cutoff frequency of second resonance. However, upon approaching the boundary of phase transition, the scattering parameters change significantly even at the first resonance.
Acknowledgments
The authors would like to thank the referees for their valuable comments. Also, they are thankful to the Russian Science Foundation for supporting this work by Grant No. 14-19-01676.
Disclosure statement
No potential conflict of interest was reported by the authors.
Additional information
Funding
References
- D. Morgan, Surface Acoustic Wave Filters with Applications to Electronic Communications and Signal Processing, 2nd, Elsevier Ltd, Northampton, UK, 2007, Available at http://store.elsevier.com/Surface-Acoustic-Wave-Filters/David-Morgan/isbn-9780123725370/
- V.M. Mukhortov, S.V. Biryukov, Y.I. Golovko, G.Y. Karapet’yan, S.I. Masychev, and V.M. Mukhortov, Surface acoustic waves in thin films of barium strontium titanate on magnesium oxide substrates, Tech. Phys. Lett. 37 (2011) pp. 207–209. Available at doi:10.1134/S1063785011030084
- N.A. Pertsev, A. Petraru, and H. Kohlstedt, Nanometer-Sized Ferroelectric Capacitors, in Handbook of Nanophysics: Nanoelectronics and Nanophotonics, K.D. Sattler, ed., chap. 6, CRC Press, NY, 2010, pp. 1–19, Available at https://www.crcpress.com/Handbook-of-Nanophysics/book-series/CRCHANOFNANO
- V.B. Shirokov, V.V. Kalinchuk, R.A. Shakhovoy, and Y.I. Yuzyuk, Anomalies of piezoelectric coefficients in barium titanate thin films, EPL (Europhysics Letters) 108 (2014) pp. 47008. Available at doi:10.1209/0295-5075/108/47008
- V.B. Shirokov, V.V. Kalinchuk, R.A. Shakhovoi, and Y.I. Yuzyuk, Material constants of barium titanate thin films, Phys. Solid State 57 (2015) pp. 1535–1540. doi:10.1134/S1063783415080302
- H. Chambon, P. Nicolay, G. Bruckner, and A. Benjeddou, Analysis of the sensitivity to pressure and temperature of a membrane based SAW sensor, International, J. Smart Nano Mater. 8 (2017), pp. 95–109. doi:10.1080/19475411.2017.1335658
- T. Hoang, SAW parameters analysis and equivalent circuit of SAW device. In: M.G. Beghi (ed.), Acoustic Waves — From Microdevices to Helioseismology, InTech, 2011. doi:10.5772/19910
- A. Reddy and S. Lahiri, Scattering parameters of interdigital and group type unidirectional saw transducers, IETEJ Res. 31 (1985) pp. 46–51. Available at doi:10.1080/03772063.1985.11436485
- Two-port piezoelectric SAW device (Application ID: 19155) (2015). Available at https://www.comsol.com/model/two-port-piezoelectric-saw-device-19155.
- Piezoacoustic transducer (Application ID: 1477) (2014). Available at https://www.comsol.com/model/piezoacoustic-transducer-1477.
- Acoustic-structure interaction with a perfectly matched layer (PML) (Application ID: 23521) (2014). Available at https://www.comsol.com/model/acoustic-structure-interaction-with-a-perfectly-matched-layer-pml-23521.
- A.K. Namdeo and H. Nemade, Extraction of COM parameters and quality factor of one port SAW resonator using FEM based simulation (2015). Available at https://www.comsol.com/paper/extraction-of-electrical-equivalent-circuit-of-one-port-saw-resonator-using-fem-30321.
- R.W. Pryor, Multiphysics modeling using COMSOL 5 and MATLAB (Engineering), Mercury Learning and Information, Virginia, USA, 2015, Available at https://www.comsol.com/books.
- Direct finite element analysis of SAW filter frequency response, Vol. 3984, jun. 2000, pp. 12–21, Available at http://proceedings.spiedigitallibrary.org/proceeding.aspx?articleid=923963.
- Y. Li, O.B. Matar, V. Preobrazhensky, and P. Pernod, Convolution-perfectly matched layer (C-PML) absorbing boundary condition for wave propagation in piezoelectric solid, in 2008 IEEE Ultrasonics Symposium, nov. IEEE, 2008, pp. 1568–1571, Available at http://ieeexplore.ieee.org/lpdocs/epic03/wrapper.htm?arnumber=4803643
- M. Mayer, S. Zaglmayr, K. Wagner, and J. Schoberl, 8E-4 perfectly matched layer finite element simulation of parasitic acoustic wave radiation in microacoustic devices, in 2007 IEEE Ultrasonics Symposium Proceedings, oct. IEEE, 2007, pp. 702–706, Available at http://ieeexplore.ieee.org/lpdocs/epic03/wrapper.htm?arnumber=4409754
- F. Ihlenburg (ed.), Finite Element Analysis of Acoustic Scattering, Applied Mathematical Sciences Vol. 132, Springer-Verlag, NY, USA, 1998
- N. Ramakrishnan, A.K. Namdeo, H.B. Nemade, and R.P. Palathinkal, Simplified model for FEM simulation of SAW Delay Line Sensor, Procedia Eng. 41 (2012), pp. 1022–1027. doi:10.1016/j.proeng.2012.07.278
- M.M. Elsherbini, M.F. Elkordy, and A.M. Gomaa, Finite element method simulation for SAW resonator-based sensors, Int. Electrical Eng. J. (IEEJ). 7 (2016), Available at http://www.ieejournal.com/2016/finite-element-method-simulation-for-saw-resonator-based-sensors/.
- A. Arnau, D. Soares, and A.A. Vives, Piezoelectric Transducers and Applications, 2nd ed., Springer-Verlag, Berlin Heidelberg, 2008.