ABSTRACT
Contact electrification is a direct triboelectrification method for producing current through charge transfer when two different differentiated materials have brought into contact. For the first time, we developed a simple triboelectric nanogenerator (TENG) where the electronic charges were realized through contact separation mode between sand-polished Kapton (SPK) and Al surface. Here, we demonstrated the energetic interfacial contact between these surfaces and observed satisfactory output performance. The novel SPK-TENG produced 40 V and 2.8 µA, of open-circuit voltages (Voc) and short-circuit currents (Isc) at 4 Hz, respectively. Mainly, the SPK-TENG was dramatically increased up the performance of TENG, up to 40% of Voc and 42% of Isc, with respect to the Kapton film because the mobility of electrons is very high on the device surface compared to the other pristine Kapton film. The fabricated SPK-TENGs were good candidates for satisfying the need for alternative contact separation mode TENGs.
GRAPHICAL ABSTRACT
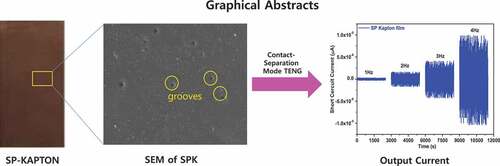
1. Introduction
With the growing hazard of energy calamity and ecological degradation by global roasting, scientist were seeking potential alternative energy technologies that can replace the conventional sources such as solar, wind, tidal energies, etc. [Citation1–Citation4]. Until now, various types of energy-harvesting systems were developed to collect energy from ambient resources, such as piezoelectric, photoelectric, thermoelectric, electrostatic, and electromagnetic strategies [Citation5,Citation6]. However, more robust, eco-friendly, cost-effective, and reliable energy-harvesting systems with modern technologies are desirable to satisfy the manufacturing demand. Mechanical energy is one of the natural renewable energy sources in the existing environment that can be taken care from human motion, including the body’s vibration, rotation, etc. [Citation7,Citation8]. Recently, triboelectric nanogenerators (TENGs) have received worldwide attention for the harvesting of sustainable green energy from ambient resources. TENGs are developed based on a combination of contact separation mode electrification and electrostatic induction to scavenge reduced mechanical energy using triboelectric materials [Citation9,Citation10]. The proper selection of triboelectric pair materials, and their rational design can upsurge the rate of energy harvesting and energy conversion efficiency [Citation11,Citation12]. At regular intervals of materials with oppositely charged surfaces, ions or electrons can be driven to flow through the external load, and generate a continuous voltage and currents [Citation13]. The proposed sand-polished Kapton (SPK)-Al TENG shows vital chemical solidity, and high strength to enhance the triboelectric current against to simple Kapton film and Al (K-Al) TENG [Citation14,Citation15]. The movement of electrons from the SPK-Al TENG is novel and simple system to generate the power from the contact separation mode process. The electric output was observed by contact separation mode TENG to generate maximum of Voc and Isc up to 75 V and 1 µA at 4 Hz, [Citation15,Citation16,Citation17].
2. Experimental section
2.1. Preparation of SPK film
A total of 2.0 cm × 2.0 cm = 4.0 cm2 of Kapton (K) commercial film was sand polished by free hand for 5 min. After completion of polish, the film was washed with acetone, DI water, and dried at 50°C until evaporation of DI water.
2.2 Characterization
The surface morphologies of Kapton before and after sand-polished samples were determined by FE-SEM using a Hitachi cold FE-SEM at 10 kV. The output signal of the all K-Al TENG and SPK-Al TENGs was performed by periodically pressed and released by means of an oscillator. The electrical outputs were measured by a Keithley Digital Multi Meter (KDMM). Next, we performed some experiments to determine the impact force using load cell YC33-5K (SETECH) at different frequencies of 1, 2, 3, and 4 Hz, respectively.
2.3. Fabrication of the contact separation K-AL TENG and SPK-AL TENGs
The fabrication, the working principle, and methodical understanding of the contact separation mode K-Al TENG and SPK-Al TENGs are examined [Citation17 Citation18, Citation19]. Here, the construction of the typical model is depicted in . First, the K-Al TENG and SPK-Al TENG were developed by attaching the Kapton and SPK films with the dimensions of 2.0 cm × 2.0 cm = 4.0 cm2 on an Al electrode. Then, the K-Al TENG and SPK-Al TENG electrodes were attached to commercial foam to reduce the reflecting force while contact and separation were progressed. Then, a load cell was connected to the top of the Al electrode.
Secondly, the Al electrode was placed on the on the foam with 2.0 cm × 2.0 cm. Meanwhile, a linear oscillator composed of a DC motor with an eccentric system consistently oscillated a linear slider. The maximum oscillation amplitude is 40 mm. The upper portion of the K-Al and SPK-Al films (load cell and Al) was then suspended by a cantilever-style beam that was connected to the linear slider. The careful setting of the overall system resulted in a slight contact between the K-Al and SPK-Al films polymeric surface and the Al surface while the slider oscillation was consistent.
3. Results and discussion
3.1. Set-up of SPK-AL TENG
showed the mechanical setup of the SPI-TENGs in full departure, and contact state, respectively, for generating high triboelectric voltage and currents. Harvesting of energy by contact electrification method by the contact and separation of SPK and Al under various frequencies of 1, 2, 3, and 4 Hz, respectively [Citation13].
The working mechanism of the developed SPK-TENGs is elucidated by the SPI polymeric surface and the aluminum (Al) electrodes were initially free of charge, as shown in ). However, after the oscillation was started by a DC motor, the triboelectrically negative Al material barely touched the surface of the SPK. The Al surface thus became negatively charged and the SPK surface became positively charged due to the contact electrification, ).
In the contact electrification procedure, the active charges were remain for a longer period of time due to the insulating properties of the material. Because of the superior hydrophobic properties of the Al surface (contact angle, CA ~ 120°), its dry nature was maintained and an effective charge separation occurs when it was detached from the SPI surface. In the course of this separation process, the charges were traveled through an external load and the current movement occurred, as shown in ). The current was remained stationary as two surfaces completely separated and new equilibrium state was reached, ). When the SPK and Al were both in a closing situation, the electrostatic induction became progressively stronger such that it broke the equilibrium, resulting in charge redistribution of Al electrodes through the acceptance, and release of electrons, as shown in ). As a consequence, the current flow in the opposite direction. Until the two layers have been completely sealed, the charge-transfer process vanished and there was no current at all [Citation11,Citation12].
To investigate the influence of the ion movement on the electric output of a SPK-Al TENG, we fabricated two types of devices for comparison: SPK-Al TENG and K-Al TENG with similar thickness (~110 µm). The two Al electrodes arranged at both sides for the electric measurements, including the open-circuit voltage (Voc) and short-circuit current (Isc) [Citation13].
3.2 FE-SEM
FE-SEM images are shown in , which disclose the surface morphologies and cross-sectional images before and after sand polishing of Kapton films. Mainly, plain surface was found without any abnormal changes on the surface, as shown in ), and the cross-sectional image was attributed longitudinal layers as layer-on-layer network due to several mono layers were packed by π-π stacking’s, as shown in ). Besides that several irregular submicron to micron-sized wholes, and channels were found on the surface which are coming from the sand polishing of the rough surface, ). Apart from, in the cross-sectional view, the sand-polished film contained inclined micro-patterned layers with uniform distance as shown in ). The distance of layers which were uniform and they can significantly contact over the surfaces when they press and released each other for generating of high output voltage and output currents while contact separation mode TENG process. Mainly, the loan pairs of electrons on the nitrogen atoms which carried by amidic links in the backbone of the oligomer chains of SPK polymer were contributed to generate power when they touch with the opposite charged surface. The loan pair of electrons were freely move with in/on the surface as source of negative charges. In addition, they can π-π stacking’s on the surface along with conjugated benzene sextets. The positive charges on Al metal surface that carry’s free positive charges while close interaction through contact separation mode phenomena. Obviously, the FE-SEM images suggested that the surface when it touches with oppositely charged Al metal surface, the power output was low. The power output was very important in the case of surface morphologies [Citation10, Citation14].
3.3. Results of SPI-TENGs
showed the confirmation of our idea, we first examined the electric output of the K-Al and SPK-Al TENGs through the contact separation mode protocol. ) shows that the open-circuit Voc of the K-Al TENG at 1 Hz surged from 1.3 to −1.2 V upon separated. By gradually increasing the speed of the linear motor from 1, 2, 3, and 4 Hz, the Voc values of K-Al TENG were increased from −1.2 to 1.3, −15.2 to 5.9, −7.6 to 8.7, and −10.8 to 12.3 V, respectively. And the output current (Isc) was also increased while increasing of the liner motor. Here, the Isc was attained from −0.03 to 0.026 µA at 1 Hz, −0.17 to 0.16 µA at 2 Hz, −0.42 to 0.47 µA at 3 Hz, and −0.9 to 0.9 µA at 4 Hz, as shown in ), respectively. On the other hand, the SPK-Al TENG was showed superior Voc and Isc at various applied speeds of contact separation TENG. Mainly, the Voc and Isc observed from SPK-Al TENG were from −2.1 to 2.6, −9.6 to 10.6, −12.7 to 14.5 V, as shown in ), and −18.5 to 21.2 V and from −0.086 to 0.086, −0.49 to 0.49, −0.72 to 0.72, and −1.4 to 1.4 µA, as shown in ), respectively. Also, the charge transferred between the Al electrodes promptly produced both Voc and Isc and the peak values were reached maximum from the TENG systems. The quantitative characterization of the output performance was showed due to induced surface charge density with respect to the Voc and Jsc of K-Al TENG is lower than that of SPK-Al TENG because the loan pair electrons were effectively participated during transferring of electrons. The tendency clearly shows that the triboelectric charge density in the SPK-Al TENG is strongly influenced by electrons moment when the contact separation process was occurred [Citation15].
Figure 4. (a) Open circuit voltages (Voc) and (b) short-circuit currents (Jsc) of K-Al TENGs; (c) open circuit voltages (Voc), and (d) short-circuit currents (Jsc) of SPK-Al TENGs at cyclic frequencies of 1, 2, 3, and 4.
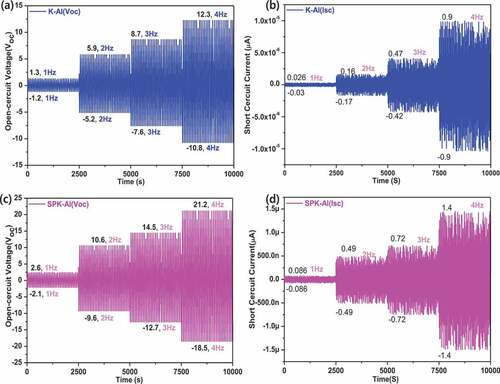
Next, we conducted some experiments to determine the impact force at various frequencies. The load cell YC33-5K (SETECH) was utilized to measure the contact force and the result is shown in ). Based on the experimental results, the change of impact force gradually increases with respect to the contact frequencies. The more impact force is applied, the more effective area is induced, which means the higher output current generated [Citation15,Citation16]. The examination of electric output of SPK-Al TENG upon connecting directly to an external load at different resistors. Based on the experimental results, the change in impact force was gradually increased with respect to the applied contact frequencies. When more impact force was applied, the more effective area was induced, which means that the higher output current generated according to the applied frequencies [Citation17,Citation19–Citation21].
The power and currents of K-Al TENGs are 0.625, 12.32, 26.56, and 53.3 μW; and 0.25, 1.1, 1.63, and 2.3 μA at 1, 2, 3, and 4 Hz, respectively [Citation22–Citation24]. Similarly, the power and currents of SPK-Al TENGs were produced 2.2 μW and 0.47 μA at 1 Hz, 44.9 μW and 2.1 μA at 2 Hz, 73.9 μW and 2.72 μA at 3 Hz, and 157.6 μW and 3.97 μA at 4 Hz, respectively. The power densities (PD) of K-Al TENGs are 0.312, 6.1, 13.13, and 26.68 μW/cm2 at 1, 2, 3, and 4 Hz due to smooth surface and the releasing electrons were poor. Where as in the case of SPK-Al TENGs, the of PD values were obtained from 1.10, 22.47, 36.9, 78.8 μW/cm2 at 1, 2, 3, and 4 Hz, respectively at 20 MΩ. These PD values of SPK-Al TENG were increased up to 71.6% at 1 Hz, 72.7% at 2 Hz, 64.4% at 3 Hz, and 66% at 4 Hz. Also, the mechanistic clarification strongly recommends that sand-polished surface was played a key role to generate more power output [Citation25].
4. Conclusion
We fabricated the commercial Kapton film for TENG application with the help of Al sheet as source of positive electrode. Next, we used sand-polished Kapton (SPK) film as negative electrode for the generation of the TENG applications. The contact separation modes SPK-Al TENG and K-Al TENG were developed which have been transferred electrons by electron transfer mechanism when they contacted each other. The K-Al and SPK-Al TENGs shown the Voc and Isc are 23.1 V and 1.8 µA, 39.7 V and 2.8 µA at 4 Hz, respectively. The Voc and Isc of SPK-Al TENG shown 40% and 42% with respect to K-Al TENG because the mobility of electrons is very high on the device surface. Consequently, the maximum instantaneous power, current and power density of SPK-Al TENG were reached up to 157.6 μW, 3.97 μA, 78.8 μW/cm2 (0.788 W/m2) at 4 Hz and 20 MΩ, respectively. These values are superior compared to the bare K-Al TENG.
Acknowledgments
This work was supported by the National Research Foundation of Korea (NRF) grant funded by the Korean government (MSIP No. 2017R1A2B2011730, No. 2018R1A6A1A03024509 and No. 2019R1A2C1011113).
Disclosure statement
No potential conflict of interest was reported by the authors.
Additional information
Funding
References
- Wiles JA, Grzybowski BA, Winkleman A, et al. A tool for studying contact electrification in systems comprising metals and insulating polymers. Anal Chem. 2003;75:4859–4867.
- Diaz AF, Guay J. Contact charging of organic materials: ion vs. electron transfer. IBM J Res Dev. 1993;37:249–260.
- Horn RG, Smith DT, Grabbe A. Contact electrification induced by monolayer modification of a surface and relation to acid–base interactions. Nature. 1993;366:442–443.
- Horn RG, Smith DT. Contact electrification and adhesion between dissimilar materials. Science. 1992;256:362–364.
- Fan FR, Tian ZQ, Wang ZL. Flexible triboelectric generator. Nano Energy. 2012;1:328–334.
- Fan FR, Lin L, Zhu G, et al. Transparent triboelectric nanogenerators and self-powered pressure sensors based on micropatterned plastic films. Nano Lett. 2012;12:3109–3114.
- Zhu G, Pan C, Guo W, et al. Triboelectric-generator-driven pulse electrodeposition for micropatterning. Nano Lett. 2012;12:4960–4965.
- Wang S, Lin L, Wang ZL. Nanoscale triboelectric-effect-enabled energy conversion for sustainably powering portable electronics. Nano Lett. 2012;12:6339–6346.
- Zhu G, Lin ZH, Jing Q, et al. Toward large-scale energy harvesting by a nanoparticle-enhanced triboelectric nanogenerator. Nano Lett. 2013;13:847–853.
- Lowell J, Rose-Innes AC. Contact electrification. Adv Phys. 1980;29:947–1023.
- Zhao D, Duan LT, Xue MQ, et al. Patterning of electrostatic charge on electrets using hot microcontact printing. Angew Chem Int Ed. 2009;48:6699–6703.
- Bandodkar AJ, Jeerapan I, Wang J. Wearable chemical sensors: present challenges and future prospects. ACS Sens. 2016;1:464–482.
- Soh S, Kwok SW, Liu H, et al. Contact de-electrification of electrostatically charged polymers. J Am Chem Soc. 2012;134:20151–20159.
- Diaz AF, Alexander DF. An ion transfer model for contact charging. Langmuir. 1993;9(993):1009–1015.
- Cheedrala RK, Rachna S, Palakodety. PK. Lipase mediated kinetic resolution of benzimidazolyl ethanols. Tetrahedron: Asymmetry. 2008;19:901–905.
- Cheedrala RK, Vijaya S, Park JW. Facile synthesis of second-generation dendrons with an orthogonal functional group at the focal point. Synth Commun. 2009;39:1966–1980.
- Cheedrala RK, Kim GH, Cho S, et al. Ladder-type heteroacenepolymers bearing carbazole and thiophene ring units and their use in field-effect transistors and photovoltaic cells. J Mater Chem. 2011;21:843–850.
- Diaz AF. adder-type heteroacene polymers bearing carbazole and thiophene ring units and their use in field-effect transistors and photovoltaic cells. J Adhes. 67(998): 11–22.
- Cheedarala RK., Park EJ, Park YB, et al. Physica Status Solidi (a), 2015;212:1756–1766
- Cheedarala RK, Park EJ, Kong K, et al. Experimental study on critical heat flux of highly efficient soft hydrophilic CuO–chitosan nanofluid templates. Int J Heat Mass Trasfer. 2016;100:396–406.
- Cheedarala RK, Duy LC, Ahn KK. Double characteristic BNO-SPI-TENGs for robust contact electrification by vertical contact separation mode through ion and electron charge transfer. Nano Energy. 2018;44:430–437.
- Cheedarala RK, Parvez AN, Ahn KK. Electric impulse spring-assisted contact separation mode triboelectric nanogenerator fabricated from polyaniline emeraldine salt and woven carbon fibers. Nano Energy. 2018;53:362–372.
- Cheedarala RK, Shahriar M, Ahn JH, et al. Harvesting liquid stream energy from unsteady peristaltic flow induced pulsatile flow-TENG (PF-TENG) using slipping polymeric surface inside elastomeric tubing. Nano Energy. 2019;65:104017.
- Cheedarala RK, Song JI. In situ generated hydrophobic micro ripples via π–π stacked pop-up reduced graphene oxide nanoflakes for extended critical heat flux and thermal conductivities. RSC Adv. 2019;9:31735–31746.
- Jeon JJ, Cheedarala RK, Kee CD, et al. Dry-type artificial muscles based on pendent sulfonated chitosan and functionalized graphene oxide for greatly enhanced ionic interactions and mechanical stiffness. Adv Funct Mater. 2013;23:6007–6018.