ABSTRACT
Self-powered technology is a novel power supply technology. In recent years, self-powered intelligent products have attracted much interests. Triboelectric nanogenerators (TENGs) can convert mechanical energy into electrical energy by contact and relative motion, thus providing the possibility of self-powering for electronic equipments. However, TENG-based self-powered technologies are limited by low power output and poor conversion efficiency. In this review, we present the development of TENG-based self-powered systems, with the emphasis on the output power of TENG and how to improve it. Based on the above applications, we propose the idea of TENG-driven self-powered remote robots, providing promising scenarios of small remote robots for land rescue or underwater detection. Due to the limited power output of the current TENGs, there are still some difficulties in driving the robot. Aiming at the problems of low power supply and poor conversion efficiency, we introduce the current attempts to improve the power generation efficiency from the perspectives of mechanical structure, electrode materials and auxiliary tools. We also outline the applications of TENGs as power supply systems in various fields such as sensing, wearable devices, and collecting Marine energy. Finally, we forecast the development prospect of TENG.
Graphical abstract
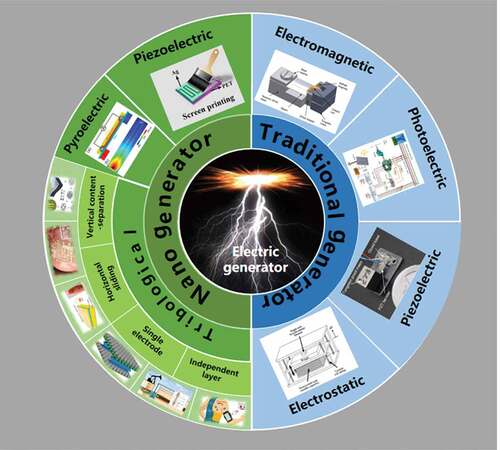
Introduction
As a basic secondary energy, electricity plays an indispensable role in human life. Power generation that converts other forms of energy into electricity, can be divided into electromagnetic [Citation1], photoelectric [Citation2], piezoelectric [Citation3] or electrostatic [Citation4] types (). The world’s smallest electric generator, first developed in 2006, was based on zinc oxide that converted mechanical energy into electricity at nanoscale [Citation5]. In general, the nanogenerators include piezoelectric nanogenerators [Citation6], tribological nanogenerators (TENG) [Citation7–14] and pyroelectric nanogenerators [Citation15]. By the combination of triboelectric effect and electrostatic induction, TENGs can collect microscale mechanical energy and convert it into electric energy [Citation16]. Its high conversion efficiency for low-frequency frictions is unmatched by any other similar technology. Generally, TENGs consist of vertical content-separation mode, horizontal sliding mode, single electrode mode and independent layer mode [Citation17,Citation18]. Through these four simple structure modes, mechanical energy can be effectively converted to achieve the power supply. Due to their advantages of high sensitivity, high integration, diverse forms, small size and low cost, TENGs can provide power in various fields such as sensing, wearable devices and collecting Marine energy [Citation19,Citation20]. In addition, TENGs are available for nanoscale integration to build self-powered systems to drive simple movements [Citation21].
Figure 1. Traditional types of electric power generation (right) and new types of electric power generation by nanogenerators (left) [Citation1–4,Citation6–12,Citation15]
![Figure 1. Traditional types of electric power generation (right) and new types of electric power generation by nanogenerators (left) [Citation1–4,Citation6–12,Citation15]](/cms/asset/abcc14a1-bc01-4317-b5d5-859eea61b86e/tsnm_a_1973143_f0001_c.jpg)
With the continuous economic and social development, electric energy plays a key role in industrial production and daily life [Citation22]. Due to the limited power generation efficiency and the increasing consumption of nonrenewable energy sources, the world is facing energy and environmental crisis [Citation23]. The voice of energy conservation and environmental protection is rising day by day. The demand for energy saving is getting higher and higher, and enters the next stage from saving electricity to not using it. In order to solve the problems of power generation and energy shortage, some researchers put forward the self-powered technology [Citation24]. The self-power supply technology is a novel energy technology and can save the power equivalent to the annual generation of the Yangtze Three Gorges power station each year, showing an inestimable market prospect [Citation25]. The application of self-powered technology in small electronic devices might bring about an unprecedented energy revolution and create great changes to people’s production and life [Citation26–29].
In this review, we provide an overview of the TENG-based self-powered technology developments in recent years, and review the applications of TENG in powering sensors, wearable devices, and collecting Marine energy. To address the problems of low power output and poor conversion efficiency of TENG, methods to improve system performance including improved mechanical structures, auxiliary equipment and electrode material modification are outlined, thus broadening the possibility of further applications of TENG in many fields. This article also covers the applications of TENG in sustainable development, providing insight into the synergies and capabilities of existing self-powered devices. This paper proposes the idea of TENG-powered robot with self-powered system. In order to enable TENG to drive a passive power supply system robot, TENG’s power supply ability should be greatly improved. Finally, this review lists the relevant shortcomings of TENGs that needed to be solved in future studies.
Existing research on TENG self-powered system
Owing to the scientific advancement and social development, human being has never stopped their pursuit to comfort life. As a result, electronic devices are gradually developing toward miniaturization, mobility and multifunction [Citation30,Citation31]. Battery-powered electronic equipments are facing the problems of frequent battery replacement, difficult recovery and serious environmental pollution [Citation32]. The demand for self-powered clean and sustainable energy is increasing. TENG, an energy conversion technology inspired by frictional electrification, shows excellent properties of high voltage, low current, small volume, diverse material structures, flexibility and good biocompatibility [Citation33]. The first flexible triboelectric nanogenerator output a voltage of about 3.3 V which applied polyethylene terephthalate (PET)-Kapton as the anode and cathode material and gold film as the external electrode () [Citation28]. In recent years, the self-powered supply systems based on TENG developed rapidly. Advances in research on biomimetic, stretchable, wear resistant materials and sealing technology, as well as the improvement of the overall electrical performance, provide opportunities for TENG self-powered systems [Citation34,Citation35].
Figure 2. Working mechanism of the first flexible triboelectric nanogenerator [Citation28]
![Figure 2. Working mechanism of the first flexible triboelectric nanogenerator [Citation28]](/cms/asset/42e6827c-3c94-480e-8cb5-5a8d71b2924d/tsnm_a_1973143_f0002_c.jpg)
This section will provide a comprehensive overview of self-powered systems driven by TENG. It mainly introduces devices that detect physiological signals, monitor environmental, collect marine energy. The TENG implanted in the body and composed of environmentally friendly materials are also mentioned. Using TENG to power robot is one of the research hotspots. This review proposes an idea of a TENG-based self-powered robot and lists the current challenges, which could serve as a reference for future research. Sensors, small electronic devices and robots can be powered by TENGs by collecting energy from the environment, such as wind energy, acoustic energy, mechanical energy of human motion and wave energy in the ocean [Citation36,Citation37]. After a period of energy accumulation, the system can even supply power to devices with higher power consumption, which realizes the vision of passive electrical equipments.
Wearable devices that detect physiological signals
With the continuous development of modern technology, sensors have shown excellent performance in daily life, biomedicine, chemistry, environment, food safety and many other fields. However, active sensors cannot always play a role in practical applications [Citation38]. TENG-based self-powered sensors have shown excellent performance in physiological signal monitoring in vitro, implant devices in vivo and environmental monitoring.Some basic information and capabilities of the TENG are listed in .
Table 1. Application of triboelectric nanogenerators in in vitro detection and wearable devices
In vitro detection of human physiological parameters is one of the hotspots in recent years. The monitoring of heart rate, pulse, blood pressure, body temperature, blood oxygen, sweat and other key indicators reflecting the physiological state of the human body at any time and anywhere is one of the key directions of scientific research achievements transformation in large companies [Citation39]. A single-electrode TENG consisting of Au-electrode and polydimethylsiloxane (PDMS) was integrated with blood oxygen monitoring biosensors, with output voltage up to 75.3 V () [Citation7]. This TENG is a square with sides of 1 cm. The unique crumpled structure supplies strong flexibility for TENG. The output performance was stable in about 700 cycles. This system realized the self-powered oxygen saturation detection system installed on the fingernail, and provided a new approach for passive continuous monitoring of oxygen saturation.
Figure 3. First demonstrated flexible self-powered blood oxygen detect system based on triboelectric nanogenerator [Citation7]
![Figure 3. First demonstrated flexible self-powered blood oxygen detect system based on triboelectric nanogenerator [Citation7]](/cms/asset/56857643-6388-4f2a-8d26-7f1dc522f3a4/tsnm_a_1973143_f0003_c.jpg)
Triboelectric nanogenerators could also be placed in shoes to collect energy from walking. The Georgia Institute of Technology team, led by Zhonglin Wang, used silicon rubber to construct a fully flexible nanogenerator array [Citation40]. This device placed in a smart sole could convert physical activity such as walking or jogging into electricity to drive electronic devices such as LED arrays, electronic watches or thermohygrometers (). This wearable power supply from TENG had a charge density of 250 C/cm2. TENG in shoes using fluorinated ethylene propylene (FEP) as the negative electrode, Al as the positive electrode and Cu as the conducting electrode, could produce about 1.6 Hz of electrical output during human walking, and provide a continuous and stable power source for commercial electrocardiogram (ECG) monitors, temperature sensors and pedometers. The tubular TENG of symmetrical structure guaranteed stable performance when it accessed to energy from mechanical motion triggered from various directions. Apart from physiological signals such as heart rate and pulse, the distribution of plantar pressure is also important. Therefore, a research group further developed the real-time foot pressure monitoring insole based on the nanogenerator, which used the self-driven nanogenerator at the sensing position to greatly reduce the device power consumption () [Citation41]. The device used the nanogenerator as the energy source to provide power for the data acquisition, data processing and Bluetooth transmission of the sensor system and realized real-time data acquisition and imaging including dozens of key points of force, which could be viewed in the mobile phone APP at any time. The nanometer energy research team demonstrated a lab model of a self-propelled smart shoe [Citation42]. This mini-sized hybrid nanogenerator could be implanted in shoes as energy source. By hybridizing a triboelectric nanogenerator and an electromagnetic generator (TENG/EMG), the effective combination of tribological initiation and electromagnetic induction resulted in the charging of a 1,000 F capacitor to 5.09 V after 100 vibrations for a hybrid nanogenerator with a radius of 2 cm and height of 1.2 cm (). This kind of small hybrid nanogenerator was embedded into the shoe as an energy battery. During human walking, the kinetic energy was collected by the nanogenerator and converted into electric energy. It could power a variety of electronic devices fitted to shoes including pedometers, LED lights, temperature detectors and GPS positioning devices.
Figure 4. TENG used in smart shoes. (a) A completely flexible array of nanogenerators used to collect energy generated during walking [Citation40]. (b) A real-time foot pressure monitoring insole based on the nanogenerator [Citation41].(c) A laboratory model of self-powered intelligent footwear using a TENG/EMG hybrid strategy [Citation42]
![Figure 4. TENG used in smart shoes. (a) A completely flexible array of nanogenerators used to collect energy generated during walking [Citation40]. (b) A real-time foot pressure monitoring insole based on the nanogenerator [Citation41].(c) A laboratory model of self-powered intelligent footwear using a TENG/EMG hybrid strategy [Citation42]](/cms/asset/38efce1d-a8dc-473a-a510-944114bd6c8b/tsnm_a_1973143_f0004_c.jpg)
Wei Gao of the California Institute of Technology and others proposed a highly durable, mass-productive, battery-free sweat detection system () [Citation8]. This paper showed a battery-free triboelectrically driven system that could power multiplexed sweat biosensors. This wearable device could efficiently harvest energy from human motion through flexible printed circuit board (FPCB)-based free-standing triboelectric nanogenerators (FTENG). Well-designed FTENG showed high power output of approximately 416 mW/m2. The device has better sensitivity, the voltage would rise sharply when the resistance increases beyond 1 MΩ. Jao et al. proposed a self-powered skin sensor to detect the composition of sweat and monitor human status [Citation43]. This system used the contact between the sweat and PDMS to generate electricity in the single-electrode mode, and diagnosed the composition of body fluid according to the electrical signals caused by the changes of NaCl concentration in sweat. In order to meet the requirements of biocompatibility, comfort and sensitivity, the outer package was constituted of chitosan and glycerin membrane.
Figure 5. A self-powered sweat monitoring device. (a) Schematic illustration of the FTENG-powered wearable sweat sensor system. (b–c) Optical images of the wearable system. The scale bars are 4 cm. (d) The structural diagram of FTENG. (e) Schematic diagram of the sweat sensor patch contacted with the flexible circuitry. (f) Working logic diagram of the system [Citation8]
![Figure 5. A self-powered sweat monitoring device. (a) Schematic illustration of the FTENG-powered wearable sweat sensor system. (b–c) Optical images of the wearable system. The scale bars are 4 cm. (d) The structural diagram of FTENG. (e) Schematic diagram of the sweat sensor patch contacted with the flexible circuitry. (f) Working logic diagram of the system [Citation8]](/cms/asset/6e527c7b-5a68-4f71-b409-f7f06fbeeb8e/tsnm_a_1973143_f0005_c.jpg)
TENG can also be used to detect pulse, voice, and eye movement signals. Chen et al. embedded Ag nanowires into a specially treated PDMS film as a friction electrode and the film was attached to the side of the upper and lower eyelids, respectively, to detect the blinking of eyes () [Citation44,Citation45]. This paper innovatively proposed a stretchable nanocomposite nanogenerator with good transparency, which could fit well to the human body to gain biomechanical energy. By placing it close to the vocal cords, different sounds could be preliminarily distinguished from the differences of the output waveforms. By placing it on the wrist, the radial pulse could be monitored in real time with high sensitivity, and the wrist movement could also be detected. Dong et al. embedded a continuous ‘chain-like’ fence-like interlaced conductive network in the silicone rubber elastomer to construct a stretchable and washable self-powered electronic skin () [Citation45]. This device generated voltage through contact and separation between human skin and TENG. The highly flexible nanogenerator was able to detect lateral stretching deformation up to 60% strain under piezoelectric mechanisms. This system could sense arterial pulse and sound vibration and could automatically count steps, measure speed, or work as a flexible keyboard. All of the above signal measurements did not require external voltage to complete signal transmission.
Figure 6. Detection devices for tensile vibration signal based on TENG. (a) A self-powered stretchable transparent nanogenerator to monitor the signals of eye movements or sound vibrations [Citation44]. (b) A multi-function stretchable, yarn-embedded electronic skin[Citation45]
![Figure 6. Detection devices for tensile vibration signal based on TENG. (a) A self-powered stretchable transparent nanogenerator to monitor the signals of eye movements or sound vibrations [Citation44]. (b) A multi-function stretchable, yarn-embedded electronic skin[Citation45]](/cms/asset/821a9683-27e2-4363-87f6-842f45bb9918/tsnm_a_1973143_f0006_c.jpg)
Wearable device is a portable hardware device that can be directly worn on the body or carried around. The existing products are mainly concentrated in the applications of watches [Citation46], smart shoes [Citation47] and glasses [Citation48]. However, existing wearable devices face problems such as unacceptable price and limited battery life. Designing and developing a cost-effective self-powered wearable electronic device is the key to this problem. He et al. proposed a self-powered respiration monitoring system based on Ag nanowires which showed the ability to remove impurities such as PM2.5 in the inhaled air and exhibited excellent antibacterial properties () [Citation9]. The CF-TENG system was able to remove PM2.5 with high efficiency of 98.83%. It could also monitor breathing status on its own power. Its output voltage could reach 21.9 V, and the output current was 21.9 µA. Wang et al. proposed an airflow-driven TENG for breathing detection () [Citation49]. This device was based on the flexible nanostructured polytetrafluoroethylene (PTFE) film in the acrylic tube driven and vibrated by the airflow to generate electricity, and it could sense different forms of breathing in real time.
Figure 7. Applications of TENG in air purification and respiratory surveillance. (a) A self-powered respiration monitoring system based on Ag nanowires [Citation9]. (b) A TENG used for respiratory detection which collected energy from airflow disturbances [Citation49]
![Figure 7. Applications of TENG in air purification and respiratory surveillance. (a) A self-powered respiration monitoring system based on Ag nanowires [Citation9]. (b) A TENG used for respiratory detection which collected energy from airflow disturbances [Citation49]](/cms/asset/f8aaa1e0-33a8-4403-afc8-c48f017714c3/tsnm_a_1973143_f0007_c.jpg)
With the widespread of COVID-19 all over the world, there is a growing demand on marks. Controlling the spread of the virus has become a key means to prevent the further spread of the epidemic. Ghatak et al. proposed a mask that could filter and inactivate viruses to a certain degree [Citation50]. The mask was composed of an internal three-layer triboelectric nano power generation device and an external three-layer click-through layer, which could collect the energy generated during speaking or breathing. Different combinations were tested including polyvinyl chloride (PVC)-nylon, polypropylene (PP)-polyurethane (PU), latex-PU, polyimide (PI)-nylon and other materials. It was found that the latex rubber-PU combination was the most suitable one for the mask in terms of material cost and actual effect. Its output power could reach μW level, which could inactivate the external protein of the virus. By constructing multiscale nanostructures and using the unique power generation mode of triboelectric nanogenerators, cellulose fiber wikin-friction nanogenerators (CF-TENG) were prepared with the functions of PM2.5 removal, antibacterial activity and self-powered monitoring of human respiration [Citation9]. Its excellent performance in improving air quality and respiratory monitoring indicated its great potential in medical self-powered wearable electronic devices.
With the rapid development of relative technologies and the consecutive improvement of the quality of people’s life, wearable devices not only need to meet the requirements of flexibility and portability, but also require their sustainability and electrical energy storage performance. Wang et al. proposed a flexible triboelectric nanogenerator integrated with a flexible battery to construct a wearable self-charging power pack [Citation51]. This system applied nickel-coated fabric as the current collector, which was worn on the body in the form of a belt to collect the mechanical energy generated from human motion. The wearable TENG could charge the battery when collecting the energy from the human body. The generated electric energy could charge the LiFPO4/Li4Ti5O12 battery after passing through the rectifier circuit, and then provided power supply for mobile phones or other electronic devices.
Device implanted in the body
Due to the limited battery life and poor biocompatibility, the implanted electronic devices have caused great troubles to users in practical applications. Replacing the battery not only requires considerable costs, but also causes damage to users’ body. Therefore, developing a set of small devices that can power implanted electronic devices is crucial to solve this problem [Citation52]. The pacemaker is an important device to maintain regular heartbeat at the critical moment. However, there are still many limitations for pacemakers since they are made of rigid materials and unable to provide continuous power supply. K Sim et al. proposed a flexible and biocompatible all-rubber self-powered cardiac pacemaker [Citation53]. It reported an epicardial bioelectronic patch made from stuffs which were similar to heart tissue in mechanical softness. This system included a rubber substrate, rubber conductors and semiconductors, and an elastic grid isolation layer formed by ion gel. The material stiffness should be similar to that of the native epicardium to allow for improved interfacing. The flexible structure made up of PDMS reduced the physical damage during deformation. The transistors were stable for a few weeks in biofluid due to the use of PDMS for encapsulation. It employed the contact and separation between Ag nanowires and PDMS to collect energy. It used patches to monitor the cardiac electrophysiological characteristics, physical characteristics and pathophysiological information in an all-round way. This rubber epicardial patch could perform electrocardiogram positioning, strain and temperature sensing, electrical pacing, thermal ablation and energy harvesting functions, and demonstrated its functions on the pig heart. In addition, TENG was implanted into the lung, bladder, stomach, and joint surfaces to monitor the surface contraction and expansion process and mechanical properties (). Packaged by soft silicone elastomer, the device showed a super low Young’s modulus to fit to the surface of organ. Abnormal changes in various organs could be monitored and electrical signals could also be sent to intervent the organs to a certain extent [Citation10,Citation54–57].
Figure 8. TENG-powered implantable devices in the body. TENG used to detect changes in lung (a), bladder (b, c), stomach (d), and joint surface [Citation54,Citation55,Citation56,Citation10]
![Figure 8. TENG-powered implantable devices in the body. TENG used to detect changes in lung (a), bladder (b, c), stomach (d), and joint surface [Citation54,Citation55,Citation56,Citation10]](/cms/asset/1f3d60d8-c464-4788-b118-12e0431643e8/tsnm_a_1973143_f0008_c.jpg)
Environmental monitoring
With the social and economic development, air pollution, such as haze, is increasingly serious and severely harmful to human health [Citation58]. Accurate perception of the surrounding environment is of great significance to human health, life safety and disaster warning. The self-powered environment sensor based on TENG can monitor the environment under passive conditions. The Hg2+ monitoring sensor was integrated with the TENG consisting of Au nanoparticles and PDMS () [Citation59]. This device provided a detection limit at an S/N of 3 at 30 nm for Hg2+ ions. It could output up to 105 V and could also detect mercury in the environment with sensitivity and specificity. If the reaction time was over 12 h, there was a non-single Au NP adsorption layer, which could lead to unstable electrical output. A multi-layered, high-speed rotating TENG was reported for air purification [Citation60]. Ag-polyimide (PI) nanofiber filters in combination with TENG resulted in the removal of air pollutants less than 0.54 μm in diameter. The addition of Ag nanoparticles could make the filter more antibacterial. Fan et al. proposed a sensor to monitor the moving speed of bubbles in coalbed methane () [Citation11]. This sensor applied Cu and low-density polyethylene (LDPE) as positive and negative electrodes. The accuracy of the sensor could reach 100% when the bubble emission interval was greater than 0.3 s. The sensor showed excellent signal-to-noise ratio and output stability at different temperatures even under the interference of coal dust. An ultra-low triboelectric nanogenerator mixed with an electromagnetic generator was reported to detect wind speed in the surrounding environment and supply power to temperature and humidity sensors () [Citation61]. This sensor provided electrical energy through the rotating sliding mode between the copper electrode and the composite material (PTFE, FEP and Kapton) film. It could monitor the wind speed even at a wind speed of 3.5 m/s. As the wind speed increased to 5.7 m/s, the power generation function still worked and the maximum load voltage per unit mass reached 65 V. A self-powered fire alarm was proposed with self-extinguishing performance () [Citation12]. In this case, TENG was composed of flame-retardant Ag-plated cotton fabric, PTFE-coated cotton fabric and a partition. It had strong flame retardancy and could sense temperature changes from 0 to 250°C and react to voltage changes. The FT-TENG could maintain 49.2% of the original output even after being burned at 17 different parts. It also maintained 34.48% of the electrical output at 220°C. It could light up warning signs, fire-fighting uniforms, LED lights, and so on, and could also supply power to the fire location alarm system. It showed excellent application prospects in fire early warning, firefighter protection as well as rapid and accurate fire extinguishing. Besides, a micro-cable power fabric combined with solar cells was proposed to convert solar energy, mechanical energy or wind energy in the environment into electrical energy. It could charge the capacitor by 2 V within 1 min. In practical applications, it could continuously supply power to electronic watches or mobile phones. On aspect of experiments, it could promote the hydrolysis reaction.
Figure 9. TENGs used for environmental monitoring. (a) A Hg2+ monitoring sensor [Citation59,Citation60]. (b) A sensor monitoring the moving speed of bubbles [Citation11]. (c) A triboelectric nanogenerator and electromagnetic generator mixed device to detect wind speed [Citation61]. (d) Flame-retardant textile-based triboelectric nanogenerators for fire protection applications [Citation12]
![Figure 9. TENGs used for environmental monitoring. (a) A Hg2+ monitoring sensor [Citation59,Citation60]. (b) A sensor monitoring the moving speed of bubbles [Citation11]. (c) A triboelectric nanogenerator and electromagnetic generator mixed device to detect wind speed [Citation61]. (d) Flame-retardant textile-based triboelectric nanogenerators for fire protection applications [Citation12]](/cms/asset/e7622d67-d5e1-486a-a650-93a47e8abfb2/tsnm_a_1973143_f0009_c.jpg)
Marine energy collection
With the expansion of economic output and the increase in population, the number of energy demand consumed in production and living continues to increase. Facing the environmental pollution and climate change caused by the consumption of fossil fuels, the development and utilization of green and renewable energy has received more and more attention. Marine energy reserves are abundant and widely distributed but not affected by environmental factors such as time, weathers or seasons. It is a kind of renewable clean energy with promising prospects [Citation62]. However, most current devices for collecting ocean energy mainly relied on electromagnetic generators [Citation63]. They owed the disadvantages of complex structure, unacceptable cost, high weight, and corrosion by seawater. Most importantly, electromagnetic power generation is not suitable for low frequency (<3 Hz) wave energy collection. The triboelectric nanogenerator provides a new route to convert mechanical energy into electrical energy. Compared with traditional electromagnetic generators, triboelectric nanogenerators exhibit the advantages of light weight, reasonable price, simple preparation, and high energy conversion efficiency at low frequency [Citation64]. Since humidity significantly affects the power output, the device well sealed by the tile cement or other materials can have only slight decreases in the electric outputs measurement after several months.
In the past few years, researchers have designed various TENGs for ocean energy harvesting. Among them, the spherical shell structure is widely used in the development of nanogenerator structures for seawater wave energy collection due to its simple structure, easy manufacture, light weight, floatability and low resistance. Xu et al. used PDMS and a smooth rubber ball to generate electricity by friction, and applied Ag-Cu electrodes to drive it () [Citation65]. Multiple rubber balls were connected and applied low-frequency sinusoidal excitation. The results showed that the charge output of the flexible connection network could be over 10 times that of the unconnected network.
Figure 10. TENGs used for the collection of Marine energy. (a) A smooth rubber ball connected to several rubber balls to increase output efficiency[Citation65]. (b) A sealed ball with spring multilayer structure to enhance the output performance by amplifying the wave vibration [Citation64]. (c) A spring-assisted TENG with a silicone rubber segmented electrode structure [Citation65]. (d) TENG inspired from bionic jellyfish. (e) A high-sensitivity wave sensor based on a liquid-solid interface triboelectric nanogenerator. (f) A triboelectric nanogenerator for collecting underwater ultrasonic energy [Citation67–69]
![Figure 10. TENGs used for the collection of Marine energy. (a) A smooth rubber ball connected to several rubber balls to increase output efficiency[Citation65]. (b) A sealed ball with spring multilayer structure to enhance the output performance by amplifying the wave vibration [Citation64]. (c) A spring-assisted TENG with a silicone rubber segmented electrode structure [Citation65]. (d) TENG inspired from bionic jellyfish. (e) A high-sensitivity wave sensor based on a liquid-solid interface triboelectric nanogenerator. (f) A triboelectric nanogenerator for collecting underwater ultrasonic energy [Citation67–69]](/cms/asset/9655b32c-87d5-4823-a664-4928812f0518/tsnm_a_1973143_f0010_c.jpg)
On this basis, Wang et al. proposed a spring multilayer structure () [Citation64]. The contact and separation between Al electrode and FEP were used to generate electrical energy. This device applied Kapton as the substrate to convert low-frequency wave energy into high-frequency vibration through a spring. The use of multi-layer structure also improved space utilization, and finally obtained an output two times higher than that of a traditional spherical generator. A spring-assisted TENG with a silicone rubber segmented electrode structure was proposed to convert low-frequency water wave motion into high-frequency vibration () [Citation66]. The output performance was further improved by optimizing electrode materials and increasing the friction surface area. This device used flexible silicone rubber/carbon black electrodes. Using the contact or separation with PTFE, it could output 22.3 μA, 630.7 V electric energy, providing electric energy support for marine lighting and marine monitoring. TENG inspired from bionic jellyfish used the contact between PTFE and copper-aluminum electrodes to separate and generate electricity () [Citation67]. The hermetic package PDMS cover was molded by injection technology according to the unique structure of bionic design, and the connection was stuck through an elastic adhesive polymer. This system applied soft PDMS for elastic packaging, and realized the monitoring of water surface temperature and fluctuations via TENG underwater power generation and self-driving. This TENG showed well resilient ability, which could detect water level and fluctuation with high sensitivity, including from press or slight deformation. The stability of undersea TENG device was detected for over 2500 cycles without decrease in its current response. Wang et al. proposed an application of a high-sensitivity wave sensor based on a liquid-solid interface triboelectric nanogenerator () [Citation68]. This sensor was composed of Cu electrodes and microstructured PTFE, which could sensitively detect wave height changes in the millimeter level. In addition to water wave energy, a triboelectric nanogenerator was proposed to collect underwater ultrasonic energy () [Citation69]. This device added free-moving balls in the holes of an acrylic plate. The up and down movements of the small balls when they vibrate under the influence of ultrasonic energy were used to collect high and low-frequency vibration energy at the same time.
Environmentally friendly TENG
With the emergence of energy crisis and environmental pollution, the demand for renewable and environmentally friendly materials is also expanding. Adopting new materials and cutting-edge technology leads to higher costs and usually requires complicated multi-step manufacturing processes. These problems largely limit the possible applications of TENGs in self-powered devices. An et al. used an extensible solution blow molding technique to blow soy protein and lignin solution into a continuous integral nanofiber (NF) with a diameter of several hundred nanometers () [Citation70]. It could produce a large fluffy and porous NF pad in a few minutes, thus achieving high voltage output. The system was highly biocompatible and could be used in implantable electronic devices and medical tapes. The good stretchability also provided the possibility as a wearable device. This system was the most efficient of its kind and the first reported TENG made from nanoscale plant-derived biopolymers.
Figure 11. Environmentally friendly triboelectric nanogenerator. (a) Natural biopolymer-based triboelectric nanogenerators [Citation70]. (b) TENG using leaves as rubbing materials to capture wind energy [Citation71]
![Figure 11. Environmentally friendly triboelectric nanogenerator. (a) Natural biopolymer-based triboelectric nanogenerators [Citation70]. (b) TENG using leaves as rubbing materials to capture wind energy [Citation71]](/cms/asset/26703440-7c8b-47bf-993a-dc4a3301a10d/tsnm_a_1973143_f0011_c.jpg)
In addition, the use of cheap biodegradable materials to collect natural energy is also a solution to the problem of sustainable development. Feng et al. applied cheap and environmentally degradable fresh leaves, dried leaves and leaf powder as friction materials to produce a new type of leaf-based TENG to collect mechanical energy and wind energy in nature () [Citation71]. In order to reuse plant leaf resources, fresh and dried leaves were used as raw materials for TENG. The energy collected in a green manner at a wind speed of 7 m/s could power an electronic watch and a set of LEDs. TENG using fresh leaves as a friction material could output 15 μA and 430 V of electrical energy. After surface modification with poly-L-lysine, TENG could reach a high voltage output of 1000 V, which could easily be used for commercial electronic watches and 868 LED power supply. In order to simulate the process of collecting wind energy in the leaf-shaped TENG, an artificial tree was created to collect natural wind energy. This showed the potential application prospects of leaf-based TENGs in environmental energy harvesting and self-powered sensors. It could be used to power the ‘EXIT’ LED lights of the exit passage in windy weather. The tree is designed based on live leaves and artificial leaves. They have potential applications in remote areas such as mountains or islands and can be used for warning and indicator lights.
Other
Wang et al. proposed a TENG-driven transparent micro-robot for water environment detection [Citation72]. This device could generate capillary waves by changing the surface of the EWOD brake. The reaction force of capillary waves was used to drive the robot to move on the water surface. This robot could carry about 3 times its own weight and move in the sea at a speed of 1 cm/s. On this basis, this paper proposes an idea of a TENG-based self-powered robot. The spring structure is embedded in the robot which consists of nanoscale electrode materials with strong positive and negative electrical properties to increase the output power of TENG to provide electrical support for small unbounded robots used in land rescue and underwater detection. However, due to the output power of TENG and other problems, it is still very challenging to realize it.
Performance improvement
At present, triboelectric nanogenerators show good performance in the applications of low-power self-powered equipments [Citation73,Citation74]. However, due to their limited output voltage, low output current and power, and too long electric energy accumulation time, it has not yet been realized for TENGs to provide continuous, efficient and long-term power supply for high-power electronic devices. It was demonstrated that the main limiting factor affecting the maximum effective energy output of the triboelectric nanogenerator was the air breakdown effect [Citation75]. In this case, Wang et al. used a high vacuum environment to minimize the impact of air breakdown and further increased the frictional charge density which refreshed the historical record of ultra-high frictional charge density at that time [Citation76].
In this section, we will focus on this problem and propose a summary of ideas from aspects including improving the mechanical structure, additional auxiliary equipment, material selection and modification, etc. This section provides details of the various methods used to improve the efficiency of electricity generation. Based on the existing research, this paper provides a reference for improving the output efficiency of TENG.
Improvement of mechanical structure
The triboelectric nanogenerator came out in 2012 [Citation28]. The first flexible TENG used PET and Kapton as positive and negative materials. The outer surface of the material was plated with a gold film to conduct electricity, and the internal contact surface of the two materials was made irregular. To achieve the power generation by amplifying the change in the distance between the positive and negative electrodes when bending up and down, the output voltage could reach about 5 V in a single bending operation. Many studies based on the above experiment added springs or structures similar to springs inside the devices to amplify the mechanical energy collected by TENGs. Yang et al. proposed a disc type TENG that could obtain three-dimensional energy [Citation77]. This device used horizontal sliding mode to generate electricity. Springs were added in three directions at a distance of 120° from the horizontal plane, which had a very wide working bandwidth and could collect lower frequency vibrations. Its output power was 140 V, 25 μA and 3.5 mW. This device could be used to collect the vibration energy generated by wind or raindrops in nature, as well as the rotational energy when the wheels rotated. A new flexible triboelectric nanogenerator was proposed which was composed of a silicone rubber spring spiral structure and an elastic electrode based on nanocomposites [Citation78]. This device consisted of two layers of conductive elastomer and two non-conductive elastomer (silicone rubber) layers. The elastic structure could collect vibration in both horizontal and vertical directions. It showed great potential in collecting arbitrary vibration energy generated from walking or the rotating of vehicles.
In addition to the spring structure, a three-dimensional super-flexible TENG built via 3D printing technology could collect mechanical energy in a wider frequency range [Citation79]. This device consisted of two different resins as the electric layer, and polyacrylamide (PAAm)-LiCl ion hydrogel as the conductive electrode. It could continuously generate 10.98 Hz/m2 electrical energy output under low-frequency stimulation of about 1.3 Hz. It was able to provide power supply for self-powered devices such as distress signal systems, smart lighting shoes, watches, and temperature sensors. This 3D structure of TENG provided the possibility to further collect ultra-low frequency human vibration energy.
Addition of auxiliary equipment
The power density of triboelectric nanogenerators depends on many factors. The most critical factor is the surface charge density of the friction electrode material. It is also a key to improve the output efficiency of TENG. Xu et al. proposed a TENG integrated with a charge pump [Citation80]. This device used a charge pump to migrate charge into the floating layer to achieve charge accumulation. The amount of accumulated surface charge was increased by about 3 times compared with before (1020 μC/m2). The simple structure and stable working condition of the device is promising for applications of self-powered equipments.
The output voltage of the triboelectric nanogenerator is high generally, but the output current is rather small. This is also one of the key reasons for its low output power. Therefore, a switch was proposed to reduce the duration of charge and discharge [Citation81]. The contact between the two friction electrodes generated electric charges while the switch was triggered to open once they were separated. At this time, no induced charges were generated in the electrodes. When the trigger switch was closed, the induced charge immediately charged the electrodes. When the two friction surfaces contacted again, the trigger switch was still open. The induced charge still stayed on the electrodes until the trigger switch was closed, and the induced charge would be discharged in an instant. The peak output current was close to 1325 A/m2, more than 2500 times the existing output current. This switch greatly increased the value of the instantaneous output pulse current.
Selection and modification of material
The choice of material is also one of the most critical factors affecting the output performance of the triboelectric nanogenerator. Generally, the conductive material is fixed as Au or Cu with good conductivity to transport charges. The choice of positive and negative electrode friction materials is very important. The friction electrode needs to choose two materials with a large difference in electron affinity which need to be counter-charged and likely to lose positive or negative electrons during the friction process. For TENG, the most important principle in selecting material is the ability to lose and gain the electrons. The material requirements of TENG in different application scenarios are not the same. For wearables, the flexibility and stretchability of devices are the key points, which need to be paid attention to. For device implanted in the body, the biocompatibility is extremely important. For the Marine energy collecting device, It is critical to build appropriate structures that amplify water waves. For environmentally friendly TENG, it is critical to find environmentally friendly materials for generating electricity. Starting from the PET-Kapton combination proposed by Wang et al., combinations of conventional materials such as Cu-FEP [Citation82], Al-FEP [Citation64], Al-Kapton [Citation80], etc., appeared one after another. Recently, Xiong et al. proposed a gas-solid interaction power generation TENG with viscosity, air tightness and self-healing properties [Citation83]. This device used supramolecular polysiloxane-dimethyl succinonyl polyurethane (PDPU) porous elastomer to generate electricity from friction with air. The surface electrostatic potential of PDPU was about ten times that of conventional anode materials.
Modification based on conventional materials is also one of the important methods to improve output efficiency. A nanogenerator based on copper and PTFE films used a fragmented structure to further increase the friction surface charge density to 120 µC/m2. Wang et al. obtained large-scale energy through nanoparticle-enhanced triboelectric nanogenerators [Citation84]. This device applied conventional PDMS as the negative electrode, PMMA as the substrate, and Au as the positive electrode with Au nanoparticles coated on the surface to increase the surface charge generated by friction. Its instantaneous output power could reach 1.2 W, while the open circuit voltage was as high as 1200 V. The energy conversion efficiency reached 14.9%, and it could instantly light up more than 600 multi-color commercial LED lights. The way the electrode material made into nanowires can also effectively improve the output efficiency. Deng et al. combined the glass elastomer and the conductive network of Ag nanowires to form a self-healing, flexible and deformable mechanical energy conversion device [Citation80]. This device could be scaled up and converted from a 2D structure to a 3D structure. The glass elastomer with an area of 3 cm×3 cm could produce a voltage output of 25 V. Besides, there are other attempts to make the self-powered systems more efficient, such as reducing mechanical wear during friction [Citation85], surface modification of friction electrode materials [Citation86,Citation87], multilayer structure for energy amplification [Citation88] and so on.
Conclusion and perspectives
This paper systematically summarizes the research progress of triboelectric nanogenerator in terms of self-powered devices, including powering sensors, powering wearable devices, collecting ocean energy, and other aspects. A new idea of TENG-driven robot is put forward. Subsequently, several methods to improve the power supply performance of TENG are introduced, including improving the mechanical structure, adding auxiliary equipment, selecting materials and modification, and so on. Structurally, TENG can use flexible materials to generate electricity to adapt to various complex environments, including the internal and external environments of the human body. Its high cost-effectiveness, light weight, good wear resistance, comfort, and biocompatibility provide the necessary conditions for the practical applications of wearable devices. Using biodegradable materials can also contribute to environmental protection and sustainable development.
With the trend of energy saving and environmental protection, more and more attention has been paid to the related researches of TENG. TENG takes advantage of the friction phenomenon that widely exists in daily life and converts mechanical energy into electrical energy efficiently by designing the friction surface with micro/nano structure. It has a broad application prospect in wearable intelligent devices, Internet of things, self-driven devices and so on. In the future, TENG will be developed toward higher output power and wider application scenarios. Developing new sources of power, absorbing a wider range of fluctuations, and finding more application scenarios will be crucial to the development of TENG. In addition, using environmentally friendly materials in the fabrication of TENG is of great significance to sustainable development. As an emerging technology from 2012, triboelectric nano power generation still has much room for improvement and many problems to be solved. Further explorations and researches are needed to apply TENGs to high-power electrical equipments.
Acknowledgments
This work was supported by the National Natural Science Foundation of China (Grant No. 21874082) and the Fundamental Research Program of Shenzhen (Grant No. JCYJ20170413104646428 and JCYJ20170307153548350).
Disclosure statement
No potential conflict of interest was reported by the author(s).
Additional information
Funding
References
- Beeby SP, Torah RN, Tudor MJ, et al. A micro electromagnetic generator for vibration energy harvesting. J Micromech Microeng. 2007;17(7):1257–1265.
- Kendzi M, Aissaoui A, Abid M, et al. Control of the photoelectric generator for used in feeding of the independent wind turbine system. IJPEDS. 2019;10(3):1613.
- Roundy S, Wright PK. A piezoelectric vibration based generator for wireless electronics. Smart Mater Struct. 2004;13(5):1131–1142.
- Tashiro R, Kabei N, Katayama K, et al. Development of an electrostatic generator that harnesses the motion of a living body: Use of a resonant phenomenon. JSME Int. J. Ser. C. 2000;43(4):916–922.
- Wang X, Zhou J, Song J, et al. Piezoelectric field effect transistor and nanoforce sensor based on a single ZnO nanowire. Nano Lett. 2006;6(12):2768–2772.
- Wang X, Yang B, Liu J, et al. A transparent and biocompatible single-friction-surface triboelectric and piezoelectric generator and body movement sensor. J Mater Chem A. 2017;5(3):1176–1183.
- Chen H, Xu Y, Zhang J, et al. Self-powered flexible blood oxygen monitoring system based on a triboelectric nanogenerator. Nanomaterials. 2019;9(5):778.
- Song Y, Min J, Yu Y, et al. Wireless battery-free wearable sweat sensor powered by human motion. Sci Adv. 2020;6(40):eaay9842.
- He X, Zou H, Geng Z, et al. A hierarchically nanostructured cellulose fiber-based triboelectric nanogenerator for self-powered healthcare products. Adv Funct Mater. 2018;28(45):1805540.
- Yao G, Kang L, Li J, et al. Effective weight control via an implanted self-powered vagus nerve stimulation device. Nat Commun. 2018;9(1):5349.
- Fan C, Wu C, Wen G, et al. Development of self-powered bubble velocity sensor for gas–liquid two-phase flow based on triboelectric nanogenerator. Nanotechnology. 2020;32(8):085503.
- Cheng R, Dong K, Liu L, et al. Flame-retardant textile-based triboelectric nanogenerators for fire protection applications. ACS Nano. 2020;14(11):15853–15863.
- Wang ZL. Triboelectric nanogenerators as new energy technology for self-powered systems and as active mechanical and chemical sensors. ACS Nano. 2013;7(11):9533–9557.
- Chen C, Zhu Y, Tian M, et al. Sustainable self-powered electro-Fenton degradation using N, S co-doped porous carbon catalyst fabricated with adsorption-pyrolysis-doping strategy. Nano Energy. 2021;81:105623.
- Yang Y, Pradel KC, Jing Q, et al. Thermoelectric nanogenerators based on single Sb-doped ZnO micro/nanobelts. ACS Nano. 2012;6(8):6984–6989.
- Dudem B, Ko YH, Leem JW, et al. Highly transparent and flexible triboelectric nanogenerators with subwavelength-architectured polydimethylsiloxane by a nanoporous anodic aluminum oxide template. ACS Appl Mater Interfaces. 2015;7(37):20520–20529.
- Lin Z, Chen J, Yang J. Recent progress in triboelectric nanogenerators as a renewable and sustainable power source. J Nanomater. 2016;(2016):5651613.
- Rathore S, Sharma S, Swain B. A critical review on triboelectric nanogenerator. IOP Conf Ser Mater Sci Eng. 2018;377:012186.
- Wang Z, Lin L, Niu S, et al. Triboelectric nanogenerators. Cham: Springer International Publishing; 2016.
- Sun Q-J, Lei Y, Zhao X-H, et al. Scalable fabrication of hierarchically structured graphite/polydimethylsiloxane composite films for large-area triboelectric nanogenerators and self-powered tactile sensing. Nano Energy. 2021;80:105521.
- Wu X, Zhu J, Evans JW, et al. A single-mode, self-adapting, and self-powered mechanoreceptor based on a potentiometric–triboelectric hybridized sensing mechanism for resolving complex stimuli. Adv Mater. 2020;32(50):2005970.
- Al Asbahi AAMH, Fang Z, Chandio ZA, et al. Assessing barriers and solutions for Yemen energy crisis to adopt green and sustainable practices: a fuzzy multi-criteria analysis. Environ Sci Pollut Res. 2020;27(29):36765–36781.
- Phillips DF. The energy crisis. Hospitals. 1973;47:56–59.
- Zhang C, Peng Z, Huang C, et al. High-energy all-in-one stretchable micro-supercapacitor arrays based on 3D laser-induced graphene foams decorated with mesoporous ZnP nanosheets for self-powered stretchable systems. Nano Energy. 2021;81:105609.
- Lim T, Tang T, Bowen WM. The impact of intergovernmental grants on innovation in clean energy and energy conservation: evidence from the American recovery and reinvestment act. Energy Policy. 2021;148:111923.
- Qiao H, Huang Z, Ren X, et al. Self-powered photodetectors based on 2D materials. Adv Opt Mater. 2020;8(1):1900765.
- Zhu M, Yi Z, Yang B, et al. Making use of nanoenergy from human – nanogenerator and self-powered sensor enabled sustainable wireless IoT sensory systems. Nano Today. 2021;36:101016.
- Fan F-R, Tian Z-Q, Lin Wang Z. Flexible triboelectric generator. Nano Energy. 2012;1(2):328–334.
- Sripadmanabhan Indira S, Aravind Vaithilingam C, Oruganti KSP, et al. Nanogenerators as a sustainable power source: state of art, applications, and challenges. Nanomaterials. 2019;9(5):773.
- Goldoni R, Ozkan-Aydin Y, Kim Y-S, et al. Stretchable Nanocomposite sensors, nanomembrane interconnectors, and wireless electronics toward feedback–loop control of a soft earthworm robot. ACS Appl Mater Interfaces. 2020;12(39):43388–43397.
- Xu F, Li X, Shi Y, et al. Recent developments for flexible pressure sensors: a review. Micromachines. 2018;9(11):580.
- Sommerville R, Zhu P, Rajaeifar MA, et al. A qualitative assessment of lithium ion battery recycling processes. Resour. Conserv. Recy. 2021;165:105219.
- Pan M, Yuan C, Liang X, et al. Triboelectric and piezoelectric nanogenerators for future soft robots and machines. iScience. 2020;23(11):101682.
- Wu H, Chen Z, Xu G, et al. Fully biodegradable water droplet energy harvester based on leaves of living plants. ACS Appl Mater Interfaces. 2020;12(50):56060–56067.
- Cheng X, Miao L, Su Z, et al. Controlled fabrication of nanoscale wrinkle structure by fluorocarbon plasma for highly transparent triboelectric nanogenerator. Microsyst. Nanoeng. 2017;3(1):16074.
- Wu Z, Cheng T, Wang ZL. Self-powered sensors and systems based on nanogenerators. Sensors. 2020;20(10):2925.
- Pang Y, Chen S, Chu Y, et al. Matryoshka-inspired hierarchically structured triboelectric nanogenerators for wave energy harvesting. Nano Energy. 2019;66:104131.
- Fu X, Mo X. Review: The application of wearable sensors on the diagnosis and monitoring of parkinson’s disease. 2018 3rd International Conference on Automation, Mechanical and Electrical Engineering (AMEE, 2018), 2018; pp. 249-254.
- Jegan R, Anusuya KV. Biosensor-based feature extraction and physiological parameters measurement for biomedical applications. IJBET. 2018;28(1):67–80.
- Wang J, Li S, Yi F, et al. Sustainably powering wearable electronics solely by biomechanical energy. Nat Commun. 2016;7(1):12744.
- Deng C, Tang W, Liu L, et al. Self -powered insole plantar pressure mapping system. Adv Funct Mater. 2018;28(29):1801606.
- Liu L, Tang W, Deng C, et al. Self-powered versatile shoes based on hybrid nanogenerators. Nano Res. 2018;11(8):3972–3978.
- Jao Y-T, Yang P-K, Chiu C-M, et al. A textile-based triboelectric nanogenerator with humidity-resistant output characteristic and its applications in self-powered healthcare sensors. Nano Energy. 2018;50:513–520.
- Chen X, Parida K, Wang J, et al. A stretchable and transparent nanocomposite nanogenerator for self-powered physiological monitoring. ACS Appl Mater Interfaces. 2017;9(48):42200–42209.
- Dong K, Wu Z, Deng J, et al. A stretchable yarn embedded triboelectric nanogenerator as electronic skin for biomechanical energy harvesting and multifunctional pressure sensing. Adv Mater. 2018;30(43):1804944.
- Chen J, Huang Y, Zhang N, et al. Micro-cable structured textile for simultaneously harvesting solar and mechanical energy. Nat. Energy. 2016;1(10):16138.
- Moulaei K, Malek M, Sheikhtaheri A. A smart wearable device for monitoring and self-management of diabetic foot: a proof of concept study. Int. J. Med. Inf. 2021;146:104343.
- Munusamy T, Karuppiah R, Bahuri NFA, et al. Telemedicine via smart glasses in critical care of the neurosurgical patient—COVID-19 pandemic preparedness and response in neurosurgery. World Neurosurg. 2021;145:e53–e60.
- Wang S, Tai H, Liu B, et al. A facile respiration-driven triboelectric nanogenerator for multifunctional respiratory monitoring. Nano Energy. 2019;58:312–321.
- Ghatak B, Banerjee S, Ali SB, et al. Design of a self-powered triboelectric face mask. Nano Energy. 2021;79:105387.
- Li S, Meng X, Yi Q, et al. Structural and electrochemical properties of LiMn0.6Fe0.4PO4 as a cathode material for flexible lithium-ion batteries and self-charging power pack. Nano Energy. 2018;52:510–516.
- Bocchetta P, Frattini D, Ghosh S, et al. Soft materials for wearable/flexible electrochemical energy conversion, storage, and biosensor devices. Materials. 2020;13(12):2733.
- Sim K, Ershad F, Zhang Y, et al. An epicardial bioelectronic patch made from soft rubbery materials and capable of spatiotemporal mapping of electrophysiological activity. Nat. Electron. 2020;3(12):775–784.
- Li J, Kang L, Long Y, et al. Implanted battery-free direct-current micro-power supply from in vivo breath energy harvesting. ACS Appl Mater Interfaces. 2018;10(49):42030–42038.
- Arab Hassani F, Mogan RP, Gammad GGL, et al. Toward self-control systems for neurogenic underactive bladder: a triboelectric nanogenerator sensor integrated with a bistable micro-actuator. ACS Nano. 2018;12(4):3487–3501.
- Lee S, Wang H, Xian Peh WY, et al. Mechano-neuromodulation of autonomic pelvic nerve for underactive bladder: a triboelectric neurostimulator integrated with flexible neural clip interface. Nano Energy. 2019;60:449–456.
- Ibrahim A, Jain M, Salman E, et al. A smart knee implant using triboelectric energy harvesters. Smart Mater Struct. 2019;28(2):025040.
- Liu J, Jiang T, Li X, et al. Triboelectric filtering for air purification. Nanotechnology. 2019;30(29):292001.
- Lin Z-H, Zhu G, Zhou YS, et al. A self-powered triboelectric nanosensor for mercury ion detection. Angew Chem Int Ed. 2013;52(19):5065–5069.
- Gu GQ, Han CB, Tian JJ, et al. Triboelectric nanogenerator enhanced multilayered antibacterial nanofiber air filters for efficient removal of ultrafine particulate matter. Nano Res. 2018;11(8):4090–4101.
- Wang P, Pan L, Wang J, et al. An ultra-low-friction triboelectric–electromagnetic hybrid nanogenerator for rotation energy harvesting and self-powered wind speed sensor. ACS Nano. 2018;12(9):9433–9440.
- Huang Y. Research on hydrodynamic characteristics of wave energy power plant. J Phys Conf Ser. 2020;1634:012144.
- Uchiyama T, Takamure K, Okuno Y, et al. Development of a self-powered wireless sensor node to measure the water flowrate by using a turbine flowmeter. Internet Things. 2021;13:100327.
- Xiao TX, Liang X, Jiang T, et al. Spherical triboelectric nanogenerators based on spring-assisted multilayered structure for efficient water wave energy harvesting. Adv Funct Mater. 2018;28(35):1802634.
- Xu L, Jiang T, Lin P, et al. Coupled triboelectric nanogenerator networks for efficient water wave energy harvesting. ACS Nano. 2018;12(2):1849–1858.
- Xiao TX, Jiang T, Zhu JX, et al. Silicone-based triboelectric nanogenerator for water wave energy harvesting. ACS Appl Mater Interfaces. 2018;10(4):3616–3623.
- Chen BD, Tang W, He C, et al. Water wave energy harvesting and self-powered liquid-surface fluctuation sensing based on bionic-jellyfish triboelectric nanogenerator. Mater Today. 2018;21(1):88–97.
- Xu M, Wang S, Zhang SL, et al. A highly-sensitive wave sensor based on liquid-solid interfacing triboelectric nanogenerator for smart marine equipment. Nano Energy. 2019;57:574–580.
- Xi Y, Wang J, Zi Y, et al. High efficient harvesting of underwater ultrasonic wave energy by triboelectric nanogenerator. Nano Energy. 2017;38:101–108.
- An S, Sankaran A, Yarin AL. Natural biopolymer-based triboelectric nanogenerators via fast, facile, scalable solution blowing. ACS Appl Mater Interfaces. 2018;10(43):37749–37759.
- Feng Y, Zhang L, Zheng Y, et al. Leaves based triboelectric nanogenerator (TENG) and TENG tree for wind energy harvesting. Nano Energy. 2019;55:260–268.
- Su Q, Quan Q, Deng J, et al. A quadruped micro-robot based on piezoelectric driving. Sensors. 2018;18(3):810.
- Zhong T, Li H, Zhao T, et al. Self-powered/self-cleaned atmosphere monitoring system from combining hydrovoltaic, gas sensing and photocatalytic effects of TiO2 nanoparticles. J Mater Sci Technol. 2021;76:33–40.
- Zhou YS, Zhu G, Niu S, et al. Nanometer resolution self-powered static and dynamic motion sensor based on micro-grated triboelectrification. Adv Mater. 2014;26(11):1719–1724.
- Zi Y, Wu C, Ding W, et al. Maximized effective energy output of contact-separation-triggered triboelectric nanogenerators as limited by air breakdown. Adv Funct Mater. 2017;27(24):1700049.
- Wang J, Wu C, Dai Y, et al. Achieving ultrahigh triboelectric charge density for efficient energy harvesting. Nat Commun. 2017;8(1):88.
- Yang J, Chen J, Yang Y, et al. Broadband vibrational energy harvesting based on a triboelectric nanogenerator. Adv Energy Mater. 2014;4(6):1301322.
- Xu M, Wang P, Wang Y-C, et al. A soft and robust spring based triboelectric nanogenerator for harvesting arbitrary directional vibration energy and self-powered vibration sensing. Adv Energy Mater. 2018;8(9):1702432.
- Chen B, Tang W, Jiang T, et al. Three-dimensional ultraflexible triboelectric nanogenerator made by 3D printing. Nano Energy. 2018;45:380–389.
- Deng J, Kuang X, Liu R, et al. Vitrimer elastomer-based jigsaw puzzle-like healable triboelectric nanogenerator for self-powered wearable electronics. Adv Mater. 2018;30(14):1705918.
- Cheng G, Lin Z-H, Lin L, et al. Pulsed nanogenerator with huge instantaneous output power density. ACS Nano. 2013;7(8):7383–7391.
- Zhao J, Zhen G, Liu G, et al. Remarkable merits of triboelectric nanogenerator than electromagnetic generator for harvesting small-amplitude mechanical energy. Nano Energy. 2019;61:111–118.
- Xiong J, Thangavel G, Wang J, et al. Self-healable sticky porous elastomer for gas-solid interacted power generation. Sci Adv. 2020;6(29):eabb4246.
- Zhu G, Lin Z-H, Jing Q, et al. Toward large-scale energy harvesting by a nanoparticle-enhanced triboelectric nanogenerator. Nano Lett. 2013;13(2):847–853.
- Zhou Y, Deng W, Xu J, et al. Engineering materials at the nanoscale for triboelectric nanogenerators. Cell Rep. Phys. Sci. 2020;1(8):100142.
- Tang W, Jiang T, Fan FR, et al. Liquid-metal electrode for high-performance triboelectric nanogenerator at an instantaneous energy conversion efficiency of 70.6%. Adv Funct Mater. 2015;25(24):3718–3725.
- Ahmed A, Hassan I, Pourrahimi AM, et al. Toward high-performance triboelectric nanogenerators by engineering interfaces at the nanoscale: looking into the future research roadmap. Adv Mater Technol. 2020;5(11):2000520.
- Han Y, Han Y, Zhang X, et al. Fish gelatin based triboelectric nanogenerator for harvesting biomechanical energy and self-powered sensing of human physiological signals. ACS Appl Mater Interfaces. 2020;12(14):16442–16450.