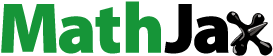
ABSTRACT
Recently, the triboelectric nanogenerator (TENG) has been widely studied for the development of flexible and wearable electronics. Among the various methods for TENG modification, melting near-field direct writing is a new method to fabricate solid-liquid TENG. Here, the electrospun PCL was introduced with traditional polymers to fabricate the compound solid triboelectric layer, and water, dimethicone and GaIn were chosen as the liquid triboelectric layers. In this paper, the solid substrate effect, temperature gradient effect, and the liquid substrate effect were compared in terms of TENGs. A free-standing model of TENG is employed in this paper, and the charge generated by the PCL-PI composite solid triboelectrical solid layer is more than 10 times higher than the pristine one, showing the high charge generating ability to melt near-field direct written microfibers. Furthermore, detailed investigation will be discussed how we can get a high the out-circuit voltage and short-circuit current.
GRAPHICAL ABSTRACT
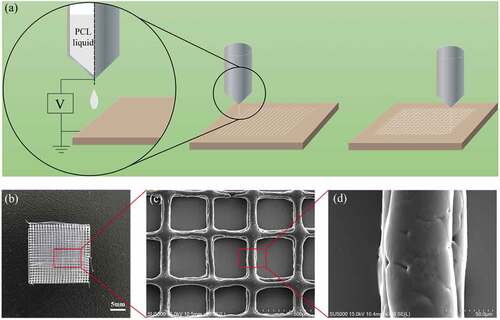
1. Introduction
Friction is a common phenomenon in our daily life, and it is used in flexible and wearable electronics since Dr Wang Zhonglin proposed the triboelectric nanogenerator (TENG) which can harvest mechanical energy in nature [Citation1,Citation2]. However, the traditional TENG is usually limited, because the solid–solid friction produces heat, limiting the efficiency of TENG [Citation3–10]. Compared with the solid–solid interface, the solid–liquid interface has a larger contact area and lower friction coefficient, leading to higher energy conversion efficiency and better reliability [Citation11–13]. Recently, novel device designs including bubble-based TENG and transistor-like design TENG have been proposed to boost the liquid–solid electricity generation [Citation14–16].
Polymers are commonly employed as a main solid triboelectric layer in the traditional TENGs and multiple TENG-based devices [Citation17–19] due to high dielectric constant and high thermal resistance. However, polymers usually have low surface roughness, causing a small contact area with liquid triboelectric layers. Hence, nanostructure materials/techniques are necessary to be introduced into the solid phase to improve the efficiencies [Citation20–26]. Xi et al. [Citation27] proposed a numerical model for calculating output performance of TENG with microstructures on the surface and obtained up to more than 100% higher output voltage compared to the original one. Huang [Citation28] constituted the microstructure on PDMS by femtosecond laser direct writing method and achieved 21 times higher power density.
In this study, we proposed a cylindrical structure of solid–liquid contact TENG and aimed to improve its electrical performance by combining the electrospinning structure with the common polymer. Various kinds of polymers with different thicknesses are compared to get the optimistic solid triboelectric layer. In this paper, the solid substrate effect, temperature gradient effect, and the liquid substrate effect were compared in terms of TENGs. A free-standing model of TENG is employed in this paper, and the charge generated by the PCL-PI composite solid triboelectrical solid layer is more than 10 times higher than the pristine one, showing the high charge generating ability to melt near-field direct written microfibers. Furthermore, detailed investigation will be discussed how we can get a high the out-circuit voltage and short-circuit current.
2. Materials and experiments
The solid triboelectric layer PI Kapton tape (50 μm, from the market) was posted on the copper (3.5 × 4 cm) electrode directly, also the tape can avoid leakage charge between the copper sheet and the liquid phase. The polycaprolactone (PCL) microstructure is introduced by electrospinning technology, using the M08-001 electrospinning machine from Foshan Qingzi Precision Measurement and Control Technology Co., Ltd. PCL can be used to make microstructures by melting near-field directing technology, this micro-net structure further increasing the contact area and improving the electrical performance of the TENG. PI-copper sheet act as the substrate for melting near-field direct writing to obtain good contact between the electrospinning structure and the PI surface. The PP cylinder (diameter, d = 3 cm; height, h = 5 cm) is subsequently placed on the solid triboelectric layer for packaging to prevent the liquid phase from leakage. The denture powder, which is used as an encapsulant material, is sprinkled on the interface between PP and PI. 13.4 ml deionized water (or equal volume liquid triboelectric materials) is injected into the container consisting of a solid triboelectric layer and PP sidewall. Also, polytetrafluoroethylene (PTFE) and polyethylene terephthalate (PET) are compared to show the effect of different materials on the output performance of TENG. The open-circuit voltage, short circuit current, and charge were performed using Electrometer Kethley 6517 for measurements at 20°C.
3. Results and discussions
3.1 Electrospinning methods
In this situation, high voltage (about 5.5 kV) is employed in producing electrical field force to pull out the PCL spinning fibers from the sprinkler continuously. The fabrication process and SEM images of PCL can be found in . The microfibers have been introduced into TENG [Citation29–32]. The charge is accumulated on the surface of the PCL liquid drop, making the drop stretch and finally change into the liquid cone. Besides, the gas press during the printing process is important when the press is low. The motive power that drives the PCL fibers is too small to achieve smooth extrusion; when the press is high, the liquid drops fall at high speed and result in oversize or non-homogeneous fiber diameter. The gap distance of every fiber also plays a core role in the print process, and the microstructure cannot be formed without the critical gap distance value while the adhesion phenomenon may appear when the gap is too narrow. To manufacture the PCL-based TENG, the first step is to print the PCL microstructure using electrospinning technology. After adjustment, the optimistic process parameters are listed in .
Figure 1. The fabrication process and SEM images of PCL. (a) The manufacturing of PCL by electrospinning. (b) The photo of the PCL microstructure. (c), (d) The SEM image of PCL.
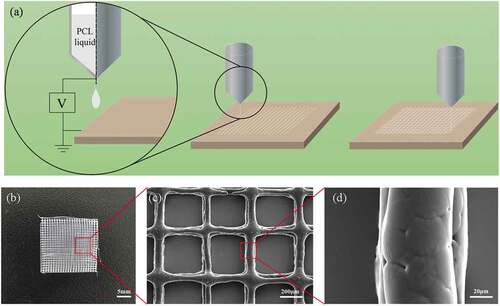
Table 1. Optimistic process parameters of electrospinning PCL.
When high voltage and gas pressure are applied, the fiber will be charged and comes out from the sprinkler and forms a microstructure as the sprinkler moves in two orthogonal directions, as demonstrated in . shows the photo and SEM images of the final microstructure, respectively. The microstructure is composed of continuous and uniform filaments with homogeneous separation distance, which is close to set parameters, and the diameter of electrospinning fiber PCL is about 60 μm. The surface microstructure is slightly rough without obvious printing defects, as shown in .
The fabrication process and output performance of PCL-PI-Deionized water TENG is reported in . When the TENG is driven through the external mechanical energy, the liquid phase triboelectric layer will leave the original interface and finally contact with the opposite solid-phase triboelectric layer instead, the charge in the deionized water moves as the deionized water moves and generates the current. The results of their output abilities (open-circuit voltage, short circuit current, and charge transportation) are demonstrated and compared in . The red lines represent the results of bare PI without PCL on or under it; blue lines represent the results of PCL on PI, and the yellow lines represent the results of PCL under PI. For the open-circuit voltage, the bare PI shows a much higher value (15.2 V) than the other two solid triboelectrics with PCL. Currently, there is no obvious difference between the three kinds. For charge transportation, PCL on PI gains a charge of about 28 nC, which is 7 times that of the original PI and 10 times t of PCL under PI. The difference between the charge results of the three electrical layers shows that the contact between the PCL microstructure and the liquid triboelectric layer can significantly increase the charge in the system due to the pre-charged PCL microstructure, and achieve the success of pre-set charge on the solid triboelectric layer by electrospinning.
Figure 2. The fabrication process and output performance of PCL-PI-Deionized water TENG. (a) The manufacturing process of TENG (PCL on PI). (b) Three diverse types of composited solid triboelectric layer. (c) The output performance (open-circuit voltage, short-circuit current, and charge, respectively) of TENG is based on three solid triboelectric layers (PI, PCL on PI, PCL under PI).
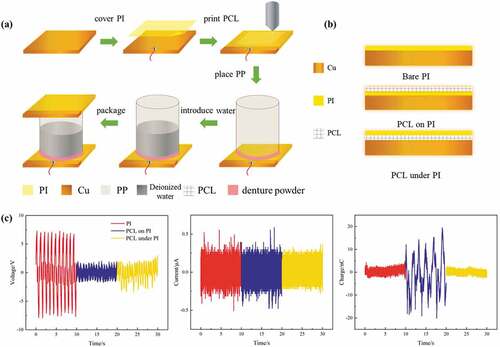
At the same time, the existence of the PCL layer is also isolated from the liquid triboelectric layer and PI layer, so that the charge generated by the interface of solid–liquid is stuck on the surface of the PCL microstructure and cannot effectively cause the change of the potential on the surface above the PI layer. In conclusion, though the amount of charge has increased, the introduction of the PCL microstructure cannot improve the output performance of the TENG.
3.2 Solid substrate effects
For the solid triboelectic layer substrate, the commonly used polymers in TENG – PI, PET, and PTFE with 50 μm thickness – have been employed as the base of solid triboelectric layer respectively due to their good triboelectric properties in gaining(PI, PTFE)/losing(PET) the electrons after the contact with other materials. The images of these three polymers under light microscope and SEM are shown in , respectively. It can be observed that the surfaces of the three polymers are clean and tidy in total with little pits. The plasma cleaner is employed to clean the three polymers of PI, PTFE, and PET in an oxygen plasma atmosphere for 5 min to tune the surface properties. We drop 0.1 ml of deionized water on the three polymers of PI, PTFE, and PET to test the wetting angle between polymers and deionized water before and after cleaning at room temperature in . In , the wetting angle of the polymer after cleaning due to the surface of each polymer becoming smoother and cleaner after cleaning. Under the cleaning action of oxygen plasma, the surface of the polymer may also be oxidized and modified.
Figure 3. The enhanced performance of TENGs using oxygen plasma-enhanced solid surfaces. (a) The optical microscope image of PI, (b) The optical microscope image of PET, (c) The optical microscope image of PTFE, (d) The SEM image of PI, (e) The SEM image of PET, (f) The SEM image of PTFE, (g) Contact angles between different polymers with deionized water before and after oxygen plasma cleaning, (h) The open-circuit voltage of TENGs, (i) The short-circuit current of TENGs, (j) The generated charge of TENGs.
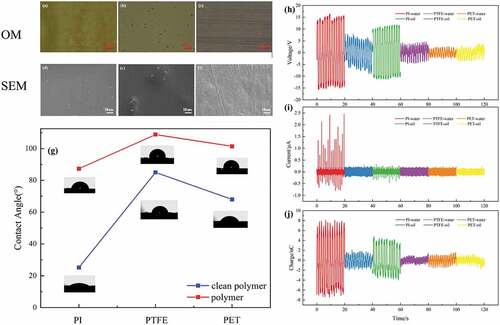
demonstrates the output performance of polymers before and after modification, respectively. In fact, plasma modification has a certain effect on the dielectric properties of the polymers [Citation33,Citation34]. After the oxygen plasma surface treatment of the polymer, the long chains of the polymer will be entangled with each other under the oxygen plasma due to the entanglement effect, and it is difficult to perform effective displacement during the polarization. When the polarization of the polymer is suppressed, it will be more difficult for the charges between different materials to exchange, the amount of charge stored after triboelectrification decreases, and the overall output performance of TENG also decreases.
Therefore, from the perspective of energy conversion efficiency and electrical performance stability, for the solid triboelectric layer composed of pure polymer, electronic polarization, and atomic polarization should dominate the polarization of the polymer, to achieve a more effective mechanical energy harvesting. Among the materials used in this topic, PET has the highest dielectric constant and should also exhibit higher output performance, but the actual result is the opposite: The output performance of the PET-based triboelectric nanogenerator is the worst among the three polymers.
This is precisely due to PET being a polar polymer, and the probability of energy loss during its polar polarization process of PET, based on the rotation and displacement of the molecules, the polarization speed is slow and the energy consumption is too high to provide an effective and stable output [Citation35].
Hence, in the research of this subject, PI with good dielectric properties and high output performance is mainly selected as the solid triboelectric layer.
3.3 Temperature gradient effects
The temperature gradient effect is proposed to change the property between the two sides. After annealing, the surface properties of polymer will change even when cooled down to room temperature, resulting in improved triboelectric performance. ) shows the difference between the hot end and the cold side of electrification performance in TENG. shows the surface of PI type before and after heat treatment, the color of PI type becomes dark after 30 min of heat treatment of 120°C. The electrical properties of the triboelectric nanogenerator composed of PI types and deionized water at room temperature (293 K) after heat treatment for 30 min at different temperatures are demonstrated in (e) and (f). When the temperature difference between the two plates increases to 150°C, the electrical performance is improved, but when the temperature difference continues to increase, the electrical performance drops again. When the temperature rises, the kinetic energy of electrons increases, making many electrons escape from the surface of the material. The hot terminal where thermionic escape occurs is more likely to lose electrons and become positive, while the cold terminal without thermionic escape is more likely to become negative than the hot one. These results give a sight into improving the TENG performance by pre-heating the solid triboelectric layer and demonstrate the potential of TENG in self-power temperature sensor.
Figure 4. TENG performance under temperature gradient. (a)The temperature gradient between the two sides, (b) the surface of PI type, (c) the surface of a PI type after 30 min of heat treatment of 225°C, (d) The charge density of TENG with the temperature difference between the terminals.
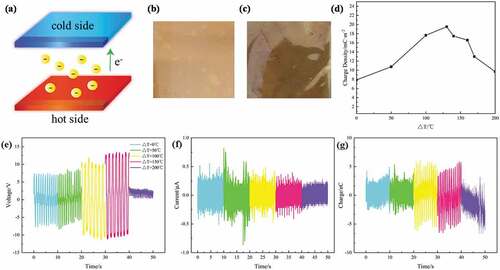
The electrical properties (e) open-circuit voltage, (f) short circuit current, (g) charge of the triboelectric nanogenerator is composed of PI types and deionized water at room temperature after heat treatment for 30 min at different temperatures.
For the ideal state, when there is a temperature difference between the two terminals of the plate, the charge transfer density σ of the friction layer has a linear relationship with the temperature of the hot terminal Th [Citation36]:
However, in real-life situations, the two terminals are not completely insulated. The liquid triboelectric layer constantly contacts and separates from the two terminals, resulting in the continuous heat exchange between the two terminals. In the process of TENG power generation, it is impossible to use a heat source to keep the hot terminals continuously insulated, making the relationship under ideal conditions fail to work. In addition, when the temperature rises, the polymer surface charge density increases, and these accumulated electrons will form a space charge zone in the polymer layer, which will inhibit the continuous escape of polymer electrons. According to the literature, in actual situations, due to the heat exchange phenomenon and space charge effect, the charge transfer density of the triboelectric layer should conform to the following optimization equation [Citation36]:
where S is defined in the following equation:
That is to say, as the temperature difference increases, the charge density on the polymer surface should follow the rule of increasing first and then decreasing. The charge density of the temperature difference PI-deionized water TENG was measured and calculated under actual conditions. The results are shown in ), a trend of first increasing and then decreasing is demonstrated, which conforms to the above law. The impact of charge density is reflected in the overall output performance of TENG.
It is worth noting that when the temperature difference continues to increase, when the hot terminal is heated to a higher temperature, the overall electrical performance of TENG decreases significantly. When the temperature difference is 200°C, the voltage (5 V) is only one-fifth of that at 150°C (25 V). This is because when the hot end is heated above 200°C, the polymer reaches the glass transition temperature, the morphology of the polymer is transformed, and the microstructure of the surface is likely to be destroyed. After testing the electrical properties, it was observed that the surface color of the hot terminal polymer after high-temperature treatment was changed.
In order to further prove the influence of temperature difference on charge density, the PTFE-deionized water friction nanogenerator and PET-deionized water triboelectric nanogenerator were also subjected to heat treatment at different temperatures for 30 min, as shown in . demonstrates the improved performance when the temperature gradient is increased at first as the blue and yellow curve shows. However, when the temperature gradient reaches 150°C, the output performance decreases due to the changes in polymer morphology. For PTFE, at a temperature difference of 50°C, the voltage is 3.5 times higher, the current is 1.8 times higher, and the charge is 1.2 times higher compared with zero temperature difference. It can be seen that an appropriate temperature difference between the two terminals can indeed improve the output performance of TENG. For PET, at a temperature difference of 50°C, the voltage is 2.8 times higher, the current is 1.1 times higher, and the amount of charge is 1.8 times higher compared with zero temperature difference. We can find that the overall trend of the influence of the temperature difference on the output performance also follows the same law: as the temperature difference increases, the charge density on the polymer surface should follow the rule of increasing first and then decreasing. The results prove that the temperature difference between the two solid triboelectric layers can improve the output electrical performance of TENG.
Figure 5. The electrical properties of the triboelectric nanogenerator composed of PTFE and PET types and deionized water at room temperature after heat treatment for 30 min at different temperatures. (a) Open-circuit voltage of PTFE. (b) Short-circuit current of PTFE. (c) Charge of PTFE. (d) Open-circuit voltage of PET. (e) Short-circuit current of PET. (f) Charge of PET.
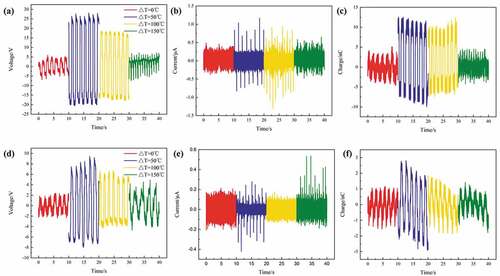
3.4 Liquid substrate effect
As the deionized water is employed as the liquid triboelectric layer, which is an important part of the solid–liquid TENG, the different liquid triboelectric layers will also cause the improvement or degradation of the performance. In this step, we choose two other different liquid triboelectric layers (dimethicone and GaIn liquid metal) to produce the TENG and further improve the performance of the TENG.
First, the dimethicone is employed, after the contact with three different polymers solid electrical layers, compared with the deionized water, the output performance is shown in (a)–(c), it is obvious that the performance of polymer-dimethicone TENG is much worse than that of polymer-deionized water TENG, that is because the dimethicone has the similar chemical property with the polymers and the difference of their dielectric property is negligible. Besides, since the contact angles between dimethicone and polymers are quite low as shown in (g), after a single triboelectric cycle, the dimethicone will remain on the surface of the polymer, resulting in degradation in the output performance of the next triboelectric cycle.
Figure 6. The application and work process of TENG. (a) the open-circuit voltage of TENGs, (b) the short-circuit current of TENGs, (c) the generated charge of TENGs. (d) The mechanism of TENG in the free-standing model. (e) The configuration of PCL based solid–liquid TENG. (f) The application of TENG for wearable energy harvesting device. (g) The working process of TENG.
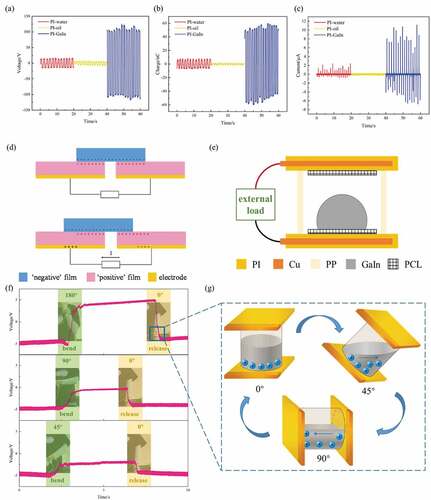
Then, the liquid metal GaIn is employed, which has superior electrical properties, as the electronic conductance, the GaIn can provide much more charges to support the effective triboelectric process when in contact with the polymers. However, the GaIn easily becomes oxidation in the open air; hence, the production and packaging processes here were operated in the grove box. The performance of the PI-based TENG with a different liquid triboelectric layer including deionized water, dimethicone, and GaIn liquid metal is demonstrated in ) where it can be clearly observed that when the liquid GaIn is used as the liquid triboelectric layer, the output performance is much better than that of deionized water and dimethicone, the voltage generated by GaIn alloy-PI is about 223 V, which is 8.6 times than that of deionized water-PI (26 V) and 18.6 times than that of simethicone-PI (12 V); in terms of current, the peak current generated by GaIn alloy-PI is about 17.8 μA, which is 6.8 times than that of deionized water-PI (2.6 μA) and 59.3 times than that of simethicone-PI (0.3 μA); in terms of charge quantity, the charge quantity generated by GaIn alloy-PI is about 112nC, which is 8.4 times than that of Deionized water-PI (13.4nC), 35 times than that of dimethicone-PI (3.2nC).
A free-standing model [Citation36–39] of TENG is employed in this paper. Its working mechanism is demonstrated in ) and the configuration of the TENG is shown in ). When the two materials with different dielectric constant or different electron distribution state contact and separate, the negative charge will be generated in the ‘negative’ film surface which has a lower and stable energy level, and the positive charge will be generated in the ‘positive’ film which has a higher energy level, when the ‘negative film’ move from one side to another side, the numbers of generated charge of two ‘positive’ film will change and the current will flow from the lower charge part to the higher charge part. The periodic change of the position of the triboelectric layer can drive the charge flow in the external load to balance local potential distribution. The triboelectric layer can directly contact one of the metal electrodes and does not need to be connected with the electrode at all times. Here, a solid–liquid contact TENG structure is proposed, it is constituted of composing solid-phase triboelectric layer including dielectric layer polymer and PCL microstructure, liquid triboelectric layer deionized water/GaIn liquid metal/dimethicone, copper electrodes, and package materials PP. In the process of movement, the liquid triboelectric layer, as an independent layer, contacts and separates the upper and lower solid triboelectric layers to generate current.
) shows the application and working process of TENG: when the TENG is fixed on the wrist, the open-circuit voltage will increase as the angle of elbow rotation which means the TENG can harvest the elbow motion energy in people’s daily life and serve as the self-powering device.
4. Conclusion
In this paper, an enhanced output performance of solid–liquid contact TENG using a melting near-field direct writing PCL nano-fiber structure has been proposed. When the liquid GaIn is used as the liquid triboelectric layer, the output performance is much better than that of deionized water and dimethicone, the voltage generated by GaIn alloy-PI is about 223 V, which is 8.6 times that of deionized water-PI (26 V) and 18.6 times than that of simethicone-PI (12 V). To test GaIn-PI-based solid–liquid dual-phase TENG, by shaking the TENG with a ‘HIT’ patterned LEDs, the LEDs light up when the two phases rub together and maintain a stable output after several cycles. In the future, the electric potential inside the solid–liquid dual-phase TENG can be changed by the simple waving of both hands, as a self-powered wearable electronic system can be obtained. Furthermore, the charge generated by the PCL-PI composite solid triboelectrical solid layer is more than 10 times higher than the pristine one, showing the high charge generating ability to melt near-field direct writing structure PCL. Further investigation should be done to find out the reason why we can get a high charge and then improve the out-circuit voltage and short-circuit current.
Acknowledgments
This work was financially supported by the opening fund of Key Laboratory of Science and Technology on Silicon Devices, Chinese Academy of Sciences (KLSDTJJ2022-5), the National Key Research and Development Program of China (2020YFE0205304), Natural Science Foundation of Chongqing, China (cstc2021jcyj-msxmX1002), the Fundamental Research Funds for the Central Universities (AUGA5710051221), and Heilongjiang Provincial Natural Science Foundation of China (YQ2022E024).
Disclosure statement
No potential conflict of interest was reported by the author(s).
Additional information
Funding
References
- Frfa B, Zqt B, Zhong L. Flexible triboelectric generator[J]. Nano Energy. 2012;1(2):328–334.
- Lináwang Z. Triboelectric nanogenerators as new energy technology and self-powered sensors–Principles, problems and perspectives[J]. Faraday Discuss. 2014;176:447–458.
- Liu Y, Zheng Y, Wu Z, et al. Conductive elastic sponge-based triboelectric nanogenerator (TENG) for effective random mechanical energy harvesting and ammonia sensing[J]. Nano Energy. 2021;79:105422.
- Ravi Kumar CM. Shahriar, Jee Hwan Ahn, et al. Nano Energy. 2019;65:2211–2855.
- Yfa B, Lza C, Yza B, et al. Leaves based triboelectric nanogenerator (TENG)and TENG tree for wind energy harvesting[J]. Nano Energy. 2019;55:260–268.
- Zhang D, Shi J, Si Y, et al. Multi-grating triboelectric nanogenerator for harvesting low-frequency ocean wave energy[J]. Nano Energy. 2019;61:132–140.
- Nguyen V, Zhu R, Yang R. Environmental effects on nanogenerators[J]. Nano Energy. 2015;14:49–61.
- Nguyen V, Yang R. Effect of humidity and pressure on the triboelectric nanogenerator[J]. Nano Energy. 2013; 2(5):604–608.
- Nguyen V, Zhu R, Yang R. Rusen Yang,Environmental effects on nanogenerators[J]. Nano Energy. 2015;14(49–61):2211–2855.
- Lin S, Xu L, Xu C, et al. Electron transfer in nanoscale contact electrification: effect of temperature in the metal–dielectric case[J]. Adv Mater. 2019;31(17):1808197.
- Yuxiang W, Yusheng L, Zou Y, et al. A multi-mode triboelectric nanogenerator for energy harvesting and biomedical monitoring, [J]. Nano Energy. 2022;92(106715):2211–2855.
- Wang C, Liang J, Zhao Y, et al. All-solid-state lithium batteries enabled by sulfide electrolytes: from fundamental research to practical engineering design. Energy Environmental Science. 2021;14(5):2577–2619.
- Zou Y, Tan P, Shi B, et al. A bionic stretchable nanogenerator for underwater sensing and energy harvesting. Nature Communications. n.d.;10(1). DOI:10.1038/s41467-019-10433-4
- Yan X, Xu W, Deng Y, et al. Bubble energy generator. Sci Adv. 2022;8(25):7698.
- Xu W, Wang Z. Fusion of slippery interfaces and transistor-inspired architecture for water kinetic energy harvesting. Joule. 2020;4(12):2527–2531.
- Xu W, Zheng H, Liu Y, et al. A droplet-based electricity generator with high instantaneous power density. Nature. 2020;578(7795):392–396.
- Zhu P, Zhang B, Wang H, et al. 3D printed triboelectric nanogenerator as self-powered human-machine interactive sensor for breathing-based language expression. 2022;15(8):7460–7467.
- Xiang S, Liu D, Jiang C, et al. Liquid-metal-based dynamic thermoregulating and self-powered electronic skin. Adv Funct Mater. 2021;31(26):2100940.
- Tang Y, Zhou H, Sun X, et al. Triboelectric touch-free screen sensor for noncontact gesture recognizing. Adv Funct Mater. 2020;30(5):1907893.
- Ev A, Mt B, Sr C. Boosted output performance of nanocellulose-based triboelectric nanogenerators via device engineering and surface functionalization[J]. Carbohydr Polym. 2021;266:118120.
- Kim SM. Effects of surface density of states N_S(E) on the number of effective triboelectric phonons in triboelectric nanogenerators (TENGs): an analytical and numerical study[J]. Mat Sci Eng. 2019;246(JUL.):76–79.
- Nie S, Fu Q, Lin X, et al. Enhanced performance of a cellulose nanofibrils-based triboelectric nanogenerator by tuning the surface polarizability and hydrophobicity[J]. Chem Eng J. 2021;404:126512.
- Qian TA, Xp A, Qz A, et al. A strategy to promote efficiency and durability for sliding energy harvesting by designing alternating magnetic stripe arrays in triboelectric nanogenerator[J]. Nano Energy. 2019;66:104087.
- Muthu M, Pandey R, Wang X, et al. Enhancement of triboelectric nanogenerator output performance by laser 3D-Surface pattern method for energy harvesting application[J]. Nano Energy. 2020;78:105205.
- Yu Y, Wang X. Chemical modification of polymer surfaces for advanced triboelectric nanogenerator development[J]. Extreme Mech Lett. 2016;9:514–530.
- Patnam H, Dudem B, Graham SA, et al. High-performance and robust triboelectric nanogenerators based on optimal microstructured poly(vinyl alcohol) and poly(vinylidene fluoride) polymers for self-powered electronic applications[J]. Energy. 2021;223:120031.
- Xi Y, Zhang F, Shi Y. Effects of surface micro-structures on capacitances of the dielectric layer in triboelectric nanogenerator: a numerical simulation study[J]. Nano Energy. 2021;79:105432.
- Huang J, Xianpeng F, Liu G, et al. Micro/nano-structures-enhanced triboelectric nanogenerators by femtosecond laser direct writing. Nano Energy. 2019;62:638–644.
- Tang Q, Pu X, Zeng Q, et al. A strategy to promote efficiency and durability for sliding energy harvesting by designing alternating magnetic stripe arrays in triboelectric nanogenerator[J]. Nano Energy. 2019;66:104087.
- Muthu M, Pandey R, Wang X, et al. Enhancement of triboelectric nanogenerator output performance by laser 3D-Surface pattern method for energy harvesting application[J]. Nano Energy. 2020;78:105205.
- Yu Y, Wang X. Chemical modification of polymer surfaces for advanced triboelectric nanogenerator development[J]. Extreme Mech Lett. 2016;9:514–530.
- Patnam H, Dudem B, Graham SA, et al. High-performance and robust triboelectric nanogenerators based on optimal microstructured poly (vinyl alcohol) and poly (vinylidene fluoride) polymers for self-powered electronic applications[J]. Energy. 2021;223:120031.
- Vandencasteele N, Reniers F. Plasma-modified polymer surfaces: characterization using XPS[J]. J Electron Spectros Relat Phenomena. 2010;178:394–408.
- Sundriyal P, Sahu M, Prakash O, et al. Long-term surface modification of PEEK polymer using plasma and PEG silane treatment[J]. Surf Interfaces. 2021;25:101253.
- Sourisseau C. Polarization measurements in macro- and micro-Raman spectroscopies: molecular orientations in thin films and azo-dye containing polymer systems. Chem Rev. 2004;104(9):3851–3891.
- Lin S, Xu L, Xu, C, et al. Electron transfer in nanoscale contact electrification: effect of temperature in the metal-dielectric case.[J]. Adv Mater. 2019; 31(17): 1808197.
- Han Q, Ding Z, Sun W; Han Q, Ding Z, Sun W, et al. Hybrid triboelectric-electromagnetic generator for self-powered wind speed and direction detection[J]. Sustainable Energy Technol Assess. 2020;39:100717.
- Sohn A, Lee JH, Yoon H-J, et al. Self-boosted power generation of triboelectric nanogenerator with glass transition by friction heat[J]. Nano Energy. 2020;74:2211–2855.
- Wang S, Xie Y, Niu S, et al. Freestanding triboelectric‐layer‐based nanogenerators for harvesting energy from a moving object or human motion in contact and non‐contact modes[J]. Adv Mater. 2014;26(18):2818–2824.