Abstract
The total electron content (TEC) derived from the global positioning system (GPS) at Varanasi (latitude 25.3° N, longitude 82.99° E), India has been measured during 2007 and 2010. We have chosen nine earthquakes (M > 5) that occurred within 2000 km of Varanasi during 2007 and 2010 to study the changes in TEC associated with these earthquakes. A monthly median (MM) of the TEC and associated inter quartile range (IQR), upper bound (UB) and lower bound (LB) are utilized as a reference for identifying abnormal signals during all nine earthquakes. The results show anomalous reductions and enhancements in the TEC. These pre-earthquake ionospheric anomalies appear within 5 days prior to earthquakes. A possible mechanism responsible for ionospheric anomalies associated with earthquakes is discussed.
1. Introduction
Earthquakes are one of the most important, frightening and destructive phenomena of nature and have terrible after effects. In the course of history, earthquakes have killed many people and have destroyed many communities. During the last 100 years there has been an average of about 18 large earthquakes (magnitude 7.0–7.9) in each year (http://earthquake.usgs.gov). Recent studies using multisensor data have shown that pronounced changes occur on the Earth's surface, meteorological and atmospheric, and ionospheric parameters associated with earthquakes which occurred in China, Russia, Japan, Taiwan and Europe (Liu et al. Citation2000, Citation2001, Matsuda and Ikeya Citation2001, Cervone et al. Citation2004, Singh et al. Citation2006, Citation2010 a,b,c, Walia et al. Citation2006, Yasouka et al. 2006, Cioni et al. Citation2007, Dutta et al. Citation2007, Ghosh et al. Citation2007, Omori et al. Citation2007, Sharma et al. Citation2007, Inan et al. Citation2008). These changes can be utilized to obtain early information about an impending earthquake. Numerous efforts have also been made to use parameters to predict earthquakes using many phenomena such as seismicity pattern (Wang et al. Citation2010), electromagnetic fields (seismo-electromagnetic) (Parrot Citation1994), ground movement (Hunt and Latter Citation1982), weather conditions and unusual clouds (Matsuda and Ikeya Citation2001), radon or hydrogen gas (Planini et al. Citation2004) and space based observations of energetic electron precipitation (Kudela et al. Citation1992). Many pseudo scientific theories and predictions are made, which scientific practitioners find problematic. Thus, reliable, repeatable earthquake forecasting is a subject surrounded by controversy and scepticism. Substantial progress has been made on the development of earthquake methods for earthquake-hazard analysis on a time scale of a few decades (Jason et al. Citation2003). However, the forecast of specific earthquakes on time scales of a few years to a few days is a highly complex problem. Recent studies have proposed that satellites and ground-based facilities may detect earthquake precursors in the ionosphere a few hours or days prior to the main earthquake (Pulinets Citation1998, Liu et al. Citation2004).
After the pioneering work of Gokhberg et al. (Citation1982), active earthquake prediction studies started by monitoring the electromagnetic waves associated with earthquakes. The primary mechanisms for the generation of electric and magnetic fields during crustal deformation and earthquakes include the piezoelectric effect, the triobo-electric effect and the electro-kinetic effect (Dobrovolsky et al. Citation1989, Sornette and Sornette Citation1990). The variations of near-Earth plasma observed over seismically active areas several days/hours before strong seismic shocks have been explained by Pulinets and Boyarchuk (Citation2004). They have demonstrated how seismo-ionospheric coupling is part of the global electric circuit and have shown that the anomalous electric field appearing in active seismic areas is the main carrier of information from the Earth into the ionosphere.
The discussion of physical mechanisms is based on experimental data. The results can be regarded as the basis for future applications such as short-term earthquake prediction. It proceeds to describe existing complex systems of spaceborne and ground-based monitoring for electromagnetic and ionospheric precursors of earthquakes, as well as those still under construction. Although so many hypotheses have been proposed for the seismo-ionospheric coupling still many points remain unsolved, especially for the equatorial and low latitude ionosphere, due to its highly dynamic behaviour. Previously, many workers reported precursory seismo-ionospheric effects on the basis of ground and satellite based observations. An increase in the critical frequency of the F-layer (f 0 F 2) two days before and a decrease in the critical frequency one day after the main shock was observed by Fatkullin et al. (Citation1989). Zelenova and Legen'ka (Citation1989) have observed large electron density variations along with spread-F phenomena occupying the area over future epicentres with a diameter more than 1000 km. Many experimental facts about variations in ionospheric E- and F-layer parameters associated with earthquakes have been discussed by Liperovsky et al. (Citation1990). Anomalies in the subionospheric propagation of very low frequency (VLF) transmitter signals associated with earthquakes have been reported by Morgounov et al. (Citation1994) and Hayakawa et al. (Citation1996a).
Reutov and Marenko (Citation1995) reported optical emissions (night airglow) approximately two days before the earthquake and also suggested that their optical emissions are connected with increasing plasma density anomalies at the F 2 layer or processes at the E-layer. A brief account of effects of seismic activities on the E- and F-regions of the ionosphere can be found in the paper by Pulinets et al. (Citation1994). Liu et al. (Citation2001) and Chuo et al. (Citation2002) have discussed the f 0 F 2 variations related to Taiwan earthquakes and found precursors (afternoon reductions) 1–6 days prior to the main shocks. Dutta et al. (Citation2007), also using a ground based ionosonde system, found strong ionospheric perturbations over Delhi a few days prior to the deadly 26 December 2004 Sumtara earthquake. Ionosondes have been the most popular instrument probing the ionospheric electron density for more than seven decades (Hunsucker Citation1991). Currently there are more than 200 ionosondes available worldwide. However, only a fraction of them are routinely operational. Therefore, the spatial and temporal coverage of the ionosonde observations are rather limited and difficult to use to correlate with seismic activities systematically. Meanwhile, due to the use of median and high frequencies (MF and HF), 1–20 MHz, ionosondes often suffer from shortwave fadeout and result in data gaps (Davies Citation1990). By contrast, today there are thousands of ground-based receivers of the global positioning system (GPS) deployed to monitor the Earth's surface deformation rates (Calais and Amarjargal Citation2000 and references therein). Owing to the use of two ultra high frequency (UHF, F1 = 1575.42 MHz and F2 = 1227.60 MHz) waves, the GPS studies are generally free from shortwave fadeout. Therefore, while performing the Earth's surface deformation observations, the same network of GPS receivers can also be used simultaneously and continuously to monitor the ionospheric TEC (Sardon et al. Citation1994, Leick Citation1995, Liu et al. Citation1996).
Recently, an apparent reduction in GPS TEC was observed a few days prior to some strong earthquakes (Calais and Minster Citation1995, Liu et al. Citation2001, Citation2002, Citation2004, Zhou et al. Citation2009, Xia et al. Citation2010). Devi et al. (Citation2008) reported that the manifestation of seismo-ionospheric effects should be strongly dependent (among others) on regional features of the ionosphere and such an increase in TEC is seen when the epicentre lies away from the observing stations (latitude, longitude or both). Karia and Pathak (Citation2010) used GPS based TEC from their observing station (Surat, India) at times close to some earthquake events (M > 5) during the year 2009 in India and its neighbouring regions. They observed anomalous variations and their relation to the seismic effect due to isolation from any known solar or magnetic activities. They argued that ionospheric behaviour changes a few days prior to a major earthquake or during an earthquake preparation zone. Liu et al. (Citation2004) identified pre-earthquake ionospheric anomalies derived from GPS TEC 15 days before and after each of the 20 (M > 6.0) earthquakes in Taiwan from September 1999 to December 2002.
The main phenomenological features of ionospheric precursors of strong earthquakes are summarized by Pulinets et al. (Citation2003). Various experimental and mathematical models clearly indicate lithosphere-ionosphere coupling within or far from the area of the earthquake preparation zone (Karia and Pathak Citation2010). This is due to the seismogenic electric field or the acoustic- gravity wave generation inside the radius of the earthquake preparatory zone. The concept of the earthquake preparation zone was introduced by Dobrovolsky et al. (Citation1979). The size of the earthquake preparation zone depends on the magnitude of the earthquake. The anomalous state of the ionosphere during seismic activities, as observed above, reveals a coupling between the lithosphere and the ionosphere. In order to explain this coupling, several possible sources have been suggested: (i) variation in the Earth's gravity (Molchanov et al. Citation2004); (ii) variation in the geomagnetic field (Iyemori et al. Citation1996); (iii) a quasi-stationary electric field (Gokhberg et al. Citation1995); (iv) penetration of seismo-electromagnetic emissions in the ionosphere (Molchanov et al. Citation1995); (v) internal gravity waves (Pokhotelov Citation1994); (vi) gas emanation (King Citation1986); and (vii) turbulent diffusion (Singh et al. Citation2004). It is worthwhile noting here that none of the above-mentioned sources alone fully explains the ionospheric anomalies.
In this paper, dual frequency GPS signals at low latitude ground station Varanasi are used to study the ionospheric perturbations in TEC associated with nine earthquakes (M > 5.0) occurring within a radius of ∼2000 km around Varanasi, India. Using the method of Liu et al. (Citation2004), a monthly median of the TEC and associated inter quartile range (IQR), upper bound (UB) and lower bound (LB) are utilized as a reference for identifying abnormal signals during all these nine earthquakes. The present results show anomalous reductions and enhancements in the TEC a few days prior to the earthquakes.
2 Observation and method of analysis
Employing a GPS receiver, we have continuously monitored the ionospheric TEC at Varanasi (geographic latitude 25.3° N, longitude 82.99° E), India since January 2007. In this study, we analysed nine earthquakes (M > 5), occurring during a quiet period of geomagnetic activity, whose epicentre distance from Varanasi, India is less than 2000 km to examine perturbations associated with earthquakes using the method of Liu et al. (Citation2004). The location of observation station Varanasi (marked by a green star) and the location of the epicentre (marked by a red plus sign) of all the nine analysed earthquakes are shown in .
Figure 1. Location of the Varanasi observation station (marked by a green star) and the locations of the epicentres (marked by red crosses) of the nine earthquakes analysed. Available in colour online.
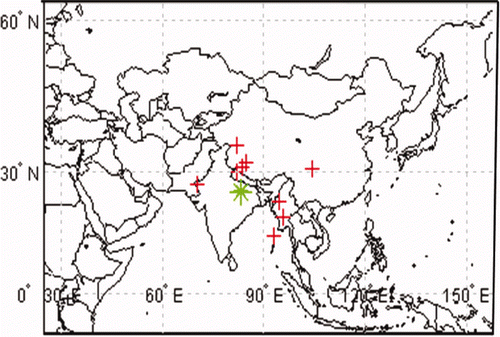
The ionosphere has a variable refractive index at radio frequencies, which are different from unity and can affect GPS signals in a number of ways as they pass from the satellite to the ground receiver. One of the significant effects is that the GPS signals traversing the ionosphere undergo an additional delay proportional to the TEC. The slant total electron content (STEC) along the ray path between a GPS satellite S and a ground-based receiver R can be written as:
where N is the electron density; 1 TEC unit = 1016 electrons m−2. In the present study, the STEC derived from the GPS data recorded at our low latitude station Varanasi is converted into vertical total electron content (VTEC) according to (Rama Rao et al. Citation2006)
where, bR and bS are the receiver and satellite biases, respectively, El is the elevation angle of the satellite in degrees, S(El ) is the obliquity factor with zenith angle χ () at the ionospheric pierce point (IPP) and VTEC is the vertical TEC at the IPP. The obliquity factor S (El ) (or mapping function) is defined as (Fedrizzi et al. Citation2002)
Figure 2. Schematic diagrams showing the geometry of the configuration in the conversion of slant total electron content (STEC) to vertical total electron content (VTEC) from the ionospheric pierce point (IPP) at altitude h.
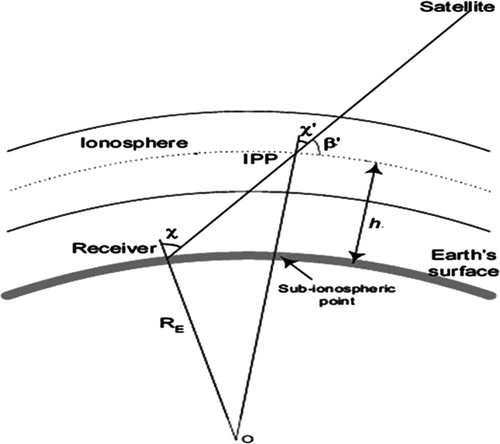
where RE is the mean Earth's radius in km, h is the ionospheric effective height above the Earth's surface, χ is the zenith angle and El is the elevation angle of the satellite in degrees. The upper and lower bounds of the TEC, utilized as a reference for identifying abnormal signals during an earthquake are calculated using the following relations (Liu et al. Citation2004):
where σ is the standard deviation and TEC (MM) is the monthly median value of the TEC.
The present study reports the ionospheric perturbations, if any, observed over Varanasi, prior to the occurrences of nine earthquakes (M > 5) that occurred from May 2007 to April 2010. We have also plotted the Dst, AE and ap indices for the same period of days so that one can be assured that there was no geomagnetic storm on the day of the earthquake and the ionospheric perturbations observed are fully due to the earthquakes.
3 Results
We are dealing here with the earthquake precursors only, so we are explaining the anomalies we have observed before the main shock, which are defined as the earthquake precursors. Details about the place, day, latitude, longitude and time of the main shock of all nine earthquakes studied are given in . The days of ionospheric precursors observed before the main shock as well as effects on the ionospheric TEC (i.e. increase or decrease in TEC) are also shown for all analysed earthquakes. Three typical examples of the earthquakes with their ionospheric perturbations are shown in –.
Table 1. Catalogue of the nine earthquakes analysed.
Figure 3. Variation in (a) total electron content (TEC), (b) Dst-index, (c) AE-index and (d) ap-index (15–25 March 2008) during the China earthquake (20 March 2008).
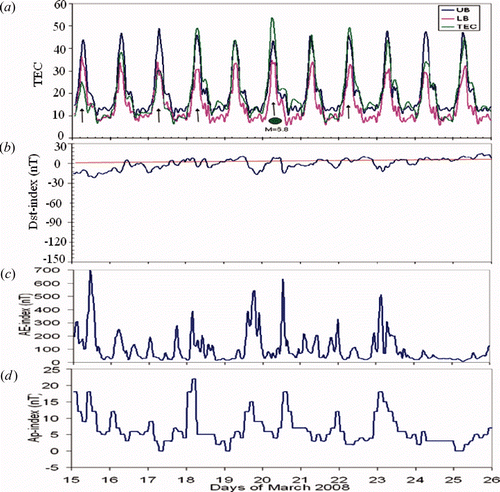
Figure 4. Variation in (a) total electron content (TEC), (b) Dst-index, (c) AE-index and (d) ap-index (3–15 April 2009) during the Jaisalmer Rajasthan earthquake (9 April 2009).
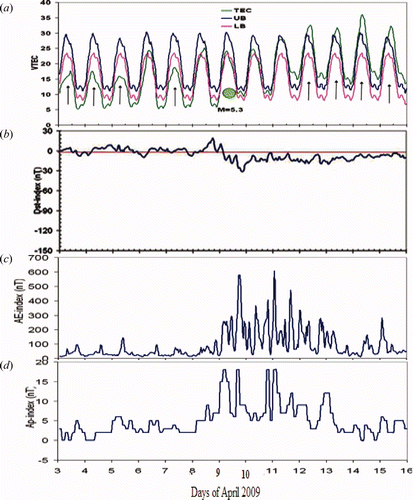
Figure 5. Variation in (a) total electron content (TEC), (b) Dst-index, (c) AE-index and (d) ap-index (24 Jul–5 August 2007) during the Myanmar earthquake (30 July 2007).
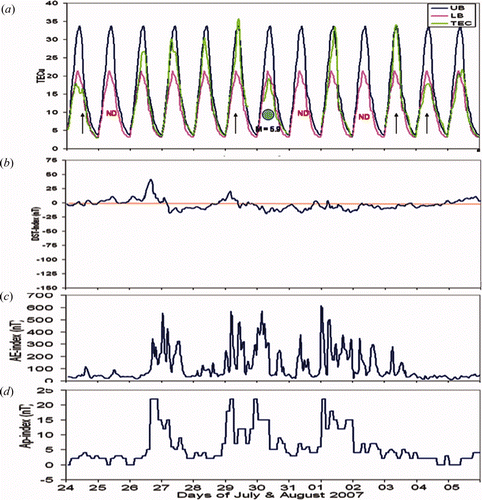
An example is the earthquake that occurred on 20 March 2008 of magnitude M = 6.8 () at Xinjiang-Xizang, a border region in China (latitude 35.8° N, longitude 81.8° E). Here we observed the changes in the ionospheric TEC within 5 days before the earthquake, which is marked by arrows (). In this particular case, we observed an increase in ionospheric TEC two days before the commencement of the main shock while we observed a decrease in the ionospheric TEC 3–5 days prior to the earthquake. The variations in the Dst index, ap index and AE-index during these periods are also shown (whose module is always less than −20 nT), the value of the ap index is less than 25 and the AE-index is less than 800 nT, which clearly shows a geomagnetic quiet period. We observed that the module of the Dst index is always less than −20 nT. Another typical example of the perturbations in the ionosphere observed over Varanasi due to the Jaisalmer, Rajasthan earthquake (M = 5.3 on the Richter scale) (latitude 27.1° N) occurred on 9 April 2009 (). Here we observed a reduction in the ionospheric TEC at 2, 4, 5 and 6 days prior to the earthquake. The Dst index, AE index and ap index, clearly show a geomagnetic quiet period. shows the third typical example of the Myanmar earthquake (M = 5.9 on the Richter scale) (latitude 19.3° N, longitude 95.6° E), which occurred on 30 July 2007. In this case, we observed a reduction in the ionospheric TEC 6 days prior to the earthquake, while an increase in the ionospheric TEC was observed one day prior to the main seismic shock. The plotted Dst index shows clear quiet day variations.
Furthermore, we also studied two more China earthquakes occurring on 9 January 2008 and 12 May 2008, the Tibet earthquake occurring on 25 August 2008 and the Nepal earthquake occurring on 8 December 2008. Two recent earthquakes occurred in year 2010: one at Andaman with the main shock occurring on 25 February 2010 and another Myanmar earthquake occurring on 12 March 2010. The details of all these earthquakes and the ionospheric peturbations are shown in .
To ensure the effect on TEC is fully due to the earthquake the level of geomagnetic activity is also plotted in each case. In all of these nine cases the module of the Dst index was smaller than −30 nT, the AE index is less than 800 nT and the ap index is less than 25, which clearly indicates that there was no geomagnetic storm 10 days before and after the main shock of the earthquake (for geomagnetic storm, Dst peaks ≤30 nT, (Osella et al. Citation1997), ap > 30, AE > 1000 nT (http://www.astrosurf.com/luxorion/qsl-perturbation5.html i.e. geomagnetic quiet days)). Thus, all the presented ionospheric perturbations are associated with the earthquakes. However, it is known that magnetospheric variations may trigger earthquakes (Sobolev et al. Citation2002, Anagnostopoulos et al. Citation2010a) and that earthquakes influence the magnetic field (Hayakawa et al. Citation1996b). This can be seen when we make some close correspondence between the various parameters shown in –. We cannot ignore the evident information presented in our figures but, in all the cases the magnetospheric variations are very weak and discussion of this point is beyond the scope of the present paper.
4 Discussion
In this paper, we have made efforts to study the main characteristic features of the seismo-ionospheric variations derived from GPS data measured at Varanasi. Here we are discussing the seismo-ionospheric mechanism and trying to demonstrate the physics of how the ionosphere starts to feel an earthquake.
Most existing models of seismo-ionospheric coupling acknowledge the important role of atmospheric electricity. Geo-electric fluctuations are the end product of several geophysical phenomenona. In particular geo-electric signals measured in seismically active areas can be attributed to stress and strain changes associated with earthquakes (Telesca et al. Citation2004). It is proposed that, due to anisotropy of atmospheric conductivity at heights greater than 60 km, the large scale high intensity (ca 1000 V m−1) vertical electric field appearing at seismically active regions a few days before strong earthquakes can penetrate into the ionosphere and create specific irregularities of electron concentration in this region (Pulinets et al. Citation1998). This anomalous quasi-static electric field generated on the ground in a seismo-active zone is detected through the seismogenic variations in the near-Earth plasma due to the high conductivity along the geomagnetic field lines. This is manifested in many physical parameters of space plasma that could be measured onboard satellites.
Theoretical calculation (Pulinets et al. Citation1998) shows that the vertical ground electric field starts to penetrate into the ionosphere and create irregularities of electron concentration when the size of the area on the ground surface occupied by the anomalous field exceeds 200 km in diameter. The Dobrovolsky et al. (Citation1979) formula shows that the radius of the earthquake preparatory zone is ρ = 100.43M km, where M is the magnitude of the earthquake on the Richter scale.
Another additional factor for estimation is simple scaling. The first most conductive layer of the ionosphere, the E-layer, is at an altitude ∼100 km, and the electric field starts to penetrate into the ionosphere when its spatial scale reaches the size of the height of the ionospheric layer. A quantity between 100 and 200 km is a transition size for the electric fields and the corresponding ionospheric reaction (Chen et al. Citation1999).
Impedance of the lower atmosphere decreases, which leads to the change in the electric field in the ionosphere (e.g. Dobrovolsky et al. Citation1979, Pulinets et al. Citation1998). The seeds of the electric field originally start from the troposphere, where temperature rises for two reasons. One is latent heat flux due to water cluster ions, which are originally produced by radon emanation, and finally form a cloud (Planini et al. Citation2004). Another heat source might be the ejection of extremely hot material from the ground. An increase in atmospheric temperature caused by the above channels might cause the internal gravity wave in the troposphere to propagate to a height of 100 km, which we call the dynamo electric field, which is generated by neutral wind under the existence of the Earth's electric field (e.g. Chen et al. Citation1999). The electric field in the dynamo region is modified by the waves propagating from the troposphere. Once the effect reaches the dynamo region the electric field, which is modified, propagates to the topside ionosphere. This electric field interacts with the ionosphere and may cause ionospheric perturbations in TEC. Since this electric field is anomalous (hence the way it interacts with the ionosphere), it results in a reduction or enhancement of the ionospheric TEC.
Recently, anomalous changes to the ground, atmosphere and ionosphere, observed by both Earth- and space-based instrumentation, were found to be associated with several earthquakes that occurred in China, Taiwan, Europe and Japan (Walia et al. Citation2006, Yasuoka et al. Citation2006, Cioni et al. Citation2007, Omori et al. Citation2007, Sharma et al. Citation2008, Anagnostopoulos et al. Citation2010b, Hayakawa and Hobara Citation2010, Singh et al. Citation2010a,b,c). These recent reported pronounced changes in the various surface and atmospheric parameters a few days prior to various earthquakes provide a strong evidence of lithosphere-atmosphere-ionosphere coupling.
Sorokin et al. (Citation2001) and Sorokin and Chmyrev (Citation2002) have formulated the electrodynamic model for atmosphere-ionosphere coupling. Sorokin et al. (Citation2005) have constructed the theoretical model of the conductivity-current distribution in the atmosphere and ionosphere, initiated by external electric current. According to this model the external current arises as a result of emanation of charged aerosols transported in the atmosphere by soil gases and the subsequent process of upward transfer, gravitational sedimentation and charge relaxation. Further development of the electrodynamic model, including the method for calculating the electron-density altitude distribution in the ionosphere caused by an external current occurring in the lower atmosphere, was presented by Sorokin et al. (Citation2006). Based on the electrodynamic model and discussions, we can explain that the injection of active substances such as radon gas and products of its decay over the earthquake preparation zone modifies the altitude profile of the electrical conductivity of the atmosphere. Also convective transport of charged aerosols in the lower atmosphere at different stages of earthquake development leads to the formation of an external electric current. Its inclusion in the atmosphere-ionosphere electric circuit is accompanied by amplification of the conductivity current that flows into the ionosphere to perturb it during the earthquake preparation. The above basic mechanism of seismo-ionospheric coupling is summarized in . It shows the processes begin from tectonic plates and the stress of the tectonic plates causes an enormous release of energy, which is the primary stage for the earthquake preparation and explains how the emanation of radon and other gases causes the generation of the acoustic gravity waves. If the amount of these emanating gases is quite large they directly cause the generation of an anomalous electric field. The way this anomalous electric field interacts with the ionosphere causes large scale change (both enhancement and depletion) in the electron density.
To understand fully the mechanism of lithosphere-atmosphere-ionosphere coupling, a rigorous study using various measurements at different heights from the lithosphere to the upper atmosphere using ground based as well as satellite based measurements at various locations of the earthquake preparatory zone is further required.
5 Conclusion
Based on the present investigations, the main features of the study are listed below:
1. | By analysing the data of nine earthquakes (M > 5) from January 2007 to 2010 in the light of magnetic storms and earthquakes, we observed that the anomalous variations in TEC are very well correlated with the occurrences of high magnitude (M > 5.0) earthquakes that occurred within 2000 km of the Varanasi observing station. | ||||
2. | We observed that out of all nine cases of high magnitude earthquakes (M > 5.0) there are three cases in which both depletions and enhancements occurred, in five cases depletions only were observed while in one case enhancement only occurred. | ||||
3. | In all of these nine cases the module of the Dst index was less than −30 nT, the AE index is less than 800 nT and the ap index is less than 25, which clearly indicates that there was no geomagnetic storm 10 days before and after the main shock of the earthquake (i.e. geomagnetic quiet days). Thus, we can conclude that all these ionospheric anomalies were fully due to the earthquakes. | ||||
4. | A possible mechanism based on the seismogenic electric field in the preparatory zone of the earthquake has been discussed. To study the complete mechanism of lithosphere-ionosphere coupling, multi-station measurement using different instruments at different height levels of the atmosphere is required. |
Acknowledgments
The work is partly supported by the Department of Science and Technology (DST), New Delhi under the Science and Engineering Research Council (SERC) projects and partly by the Ministry of Earth Sciences (MOES), New Delhi. We are also thankful to the World Data Center for Geomagnetism at Kyoto University Japan for providing geomagnetic data (http://swdcwww.kugi.kyto-u.ac.jp). We are thankful to all three reviewers for their valuable comments and suggestions to improve the quality of the manuscript.
References
- Anagnostopoulos , G. , Papandreou , A. and Antoniou , P. 2010a . Solar wind triggering of geomagnetic disturbances and strong (M > 6.8) earthquakes during the November–December 2004 period . arXiv: 1012.3585v1 ,
- Anagnostopoulos , G. , Rigas , V. , Athanasiou , M. , Iliopulos , A. , Vassiliadis , E. and Iossifidis , N. 2010b . Temporal evolution of energetic electron precipitation as a promising tool for earthquake prediction . Astronomical Society of the Pacific Conference Series , 424 : 67 – 74 .
- Calais , E. and Minster , J. B. 1995 . GPS detection of ionospheric perturbations following the January 17, 1994, Northridge earthquake . Geophysical Research Letters , 22 : 1045 – 1048 .
- Calais , E. and Amarjargal , S. 2000 . New constraints on current deformation in Asia from continuous GPS measurements at Ulan Baatar, Mongolia . Geophysical Research Letters , 27 : 1527 – 1530 .
- Cervone , G. , Kafatos , M. , Napoletani , D. and Singh , R. P. 2004 . Wavelet maxima curves of surface latent heat flux associated with two recent Greek earthquakes . Natural Hazards and Earth Systems Sciences , 4 : 1 – 16 .
- Chen , Y. I. , Chuo , J. Y. , Liu , J. Y. and Pulinets , S. A. 1999 . “ A statistical study of ionospheric precursors of strong earthquake at Taiwan area ” . In XXIVth General Assembly of International Union of Radio Science (URSI), Toronto, 13–21 August 745
- Chuo , Y. J. , Liu , J. Y. , Pulinets , S. A. and Chen , Y. I. 2002 . The ionospheric perturbations prior to the Chi-Chi and Chia-Yi earthquakes . Journal of Geodynamics , 33 : 509 – 517 .
- Cioni , R. , Guidi , M. , Pierotti , L. and Scozzari , A. 2007 . An automatic monitoring network installed in Tuscany (Italy) for studying possible geochemical precursory phenomena . Natural Hazards and Earth System Sciences , 7 : 405 – 416 .
- Davies , K. 1990 . Ionospheric Radio , London : Peter Peregrinus .
- Devi , M. , Barbara , A. K. , Depueva , A. and Depuev , V. 2008 . Preliminary results of TEC measurement in Guwahati, India . Advances in Space Research , 42 : 753 – 756 .
- Dobrovolsky , I. P. , Zubkov , S. I. and Miachkin , V. I. 1979 . Estimation of the size of earthquake preparation zones . Pure and Applied Geophysics , 117 : 1025 – 1044 .
- Dobrovolsky , I. P. , Gershenzon , N. I. and Gokhberg , M. B. 1989 . Theory of electrokinetic effects occurring at the final stage in the preparation of a tectonic earthquake . Physics of the Earth and Planetary Interiors , 57 : 144 – 156 .
- Dutta , H. N. , Dabas , R. S. , Das , R. M. , Sharma , K. and Singh , B. 2007 . Ionospheric perturbations over Delhi caused by the 26 December 2004 Sumatra Earthquake . International Journal of Remote Sensing , 28 : 3141 – 3151 .
- Fatkullin , M. N. , Zelenova , T. I. and Legenka , A. D. 1989 . On the ionospheric effects of asthenospheric earthquakes . Physics of the Earth and Planetary Interiors , 57 : 82 – 85 .
- Fedrizzi , M. , Langley , R. B. , Komjathy , A. , Santos , M. C. , de Paula , E. R. and Kantor , I. J. 2002 . The low-latitude ionosphere: monitoring its behavior with GPS . GPS World , 13 : 41 – 47 .
- Ghosh , D. , Deb , A. , Sengupta , R. , Patra , K. K. and Bera , S. 2007 . Pronounced soil-radon anomaly – precursor of recent earthquakes in India . Radiation Measurements , 42 : 466 – 471 .
- Gokhberg , M. B. , Morgounov , V. A. , Yoshino , T. and Tomizawa , I. 1982 . Experimental measurement of electromagnetic emissions possibly related to earthquakes in Japan . Journal of Geophysical Research , 87 : 7824 – 7828 .
- Gokhberg , M. B. , Pokhotelov , O. A. and Morgounov , V. A. 1995 . “ Earthquake prediction: Seismo–electromagnetic phenomenon ” . Reading–Philadelphia : Gordon and Breach, Science Publishers .
- Hayakawa , M. and Hobara , Y. 2010 . Current status of seismo-electromagnetics for short-term earthquake prediction . Geomatics, Natural Hazards and Risk , 1 : 115 – 155 .
- Hayakawa , M. , Molchanov , O. A. , Ondoh , T. and Kawai , E. 1996a . Anomalies in the subionospheric VLF signals for the 1995 Hyogo-ken Nanbu earthquake . Journal of Physics of the Earth , 44 : 413 – 418 .
- Hayakawa , M. , Kawate , R. and Molchanov , O. A. 1996b . Results of ultra-low-frequency magnetic field measurements during the Guam earthquake of 8 August 1993 . Geophysical Research Letters , 23 : 241 – 244 .
- Hunsucker , R. D. 1991 . Radio Techniques for Probing the Terrestrial Ionosphere , Berlin, Heidelberg, New York : Springer .
- Hunt , T. M. and Latter , J. H. 1982 . A survey of seismic activity near Wairakei geothermal field, New Zealand . Journal of Volcanology and Geothermal Research , 14 : 319 – 334 .
- Inan , S. , Akgul , T. , Seyis , C. , Saatcilar , R. , Baykut , S. , Ergintav , S. and Bas , M. 2008 . Geochemical monitoring in the Marmara region (NW Turkey): a search for precursors of seismic activity . Journal of Geophysical Research , 113 DOI: 10.1029/2007JB005206
- Iyemori , T. , Kamei , T. , Tanaka , Y. , Takeda , M. , Hashimoto , T. , Araki , T. , Okamoto , T. , Watanabe , K. , Sumitomo , N. and Oshiman , N. 1996 . Co-seismic geomagnetic variation observed at the 1995 Hygoken-Nanbu earthquake . Journal of Geomagnetism Geoelectricity , 48 : 1059 – 1070 .
- Jason , S. J. , Pulinets , S. , Curiel , A. D.S. and Liddle , D. 2003 . Earthquake science research with microsatellite . Philosophical Transactions of the Royal Society A , 361 : 169 – 173 .
- Karia , S. P. and Pathak , K. N. 2010 . Change in refractivity of the atmosphere and large variation in TEC associated with some earthquakes, observed from GPS receiver . Advances in Space Research , DOI:10.1016/j.asr.2010.09.019
- King , C.-Y. 1986 . Gas geochemistry applied to earthquake prediction: an overview . Journal of Geophysical Research , 91 : 12269 – 12281 .
- Kudela , K. , Matisin , J. , Shuiskaya , F. K. , Akentieva , O. S. , Romantsova , T. V. and Venkatesan , D. 1992 . Inner zone electron peaks observed by the “Active” satellite . Journal of Geophysical Research , 97 : 8681 – 8683 .
- Leick , A. 1995 . GPS Satellite Surveying , New York : John Wiley .
- Liperovsky , V. A. , Alimov , O. A. , Shalimov , S. L. , Gokhberg , M. B. , Liperovskaya , R. K.H. and Saidshoyev , A. 1990 . Research on ionospheric F-region before earthquakes . Izvestiya of USSR Academy of Sciences: Physics of the Solid Earth , 26 : 77 – 86 .
- Liu , J. Y. , Tsai , H. F. and Jung , T. K. 1996 . Total electron content obtained by using the global positioning system . Terrestrial, Atmospheric and Oceanic Sciences , 7 : 107 – 117 .
- Liu , J. Y. , Chen , Y. I. , Pulinets , S. A. , Tasi , Y. B. and Chuo , Y. J. 2000 . Seismo-ionospheric signatures prior to M ≥ 6.0 Taiwan earthquakes . Geophysical Research Letters , 27 : 3113 – 3116 .
- Liu , J. Y. , Chen , Y. I. , Chuo , Y. J. and Tsai , H. F. 2001 . Variations of ionospheric total electron content during the Chi-Chi earthquake . Geophysical Research Letters , 28 : 1383 – 1386 .
- Liu , J. Y. , Chuo , Y. J. , Pulinets , S. A. , Tsai , H. F. and Zeng , X. 2002 . “ A study on the TEC perturbations prior to the Rei-Li, Chi-Chi and Chia-Yi earthquakes ” . In Seismo Electromagnetics: Lithospheric-Atmospheric-Ionospheric Coupling , Edited by: Hayakawa , M. and Molchanov , O. A. 297 – 301 . Tokyo : Terra Scientific .
- Liu , J. Y. , Chuo , Y. J. , Shan , S. J. , Tsai , Y. B. , Chen , Y. I. , Pulinets , S. A. and Yu , S. B. 2004 . Pre-earthquake ionospheric anomalies registered by continuous GPS TEC . Annales Geophysicae , 22 : 1585 – 1593 .
- Matsuda , T. and Ikeya , M. 2001 . Variation of nitric oxide concentration before the Kobe earthquake, Japan . Atmospheric Environment , 35 : 3097 – 3102 .
- Molchanov , O. A. , Hayakawa , M. and Rafalsky , V. A. 1995 . Penetration characteristics of electromagnetic emissions from an underground seismic source into the atmosphere, ionosphere and magnetosphere . Journal of Geophysical Research , 100 : 1691 – 1712 .
- Molchanov , O. , Fedorov , E. , Schekotov , A. , Gordeev , E. , Chebrov , V. , Surkov , V. , Rozhnoi , A. , Andreevsky , S. , Iudin , D. , Yunga , S. , Lutikov , A. , Hayakawa , M. and Biagi , P. F. 2004 . Lithosphere-atmosphere-ionosphere coupling as governing mechanism for preseismo short-term events in atmosphere and ionosphere . Natural Hazards and Earth System Sciences , 4 : 757 – 767 .
- Morgounov , V. A. , Ondoh , T. and Nagai , S. 1994 . “ Anomalous variation of VLF signals associated with strong earthquakes (M>7) ” . In Electromagnetic Phenomena Related to Earthquake Prediction , Edited by: Hayakawa , M. and Fujinawa , Y. 409 – 428 . Tokyo : Terra Scientific .
- Omori , Y. , Yasuoka , Y. , Nagahama , H. , Kawada , Y. , IshikawA , T. , Tokonami , S. and Shinogi , M. 2007 . Anomalous radon emanation linked to preseismic electromagnetic phenomena . Natural Hazards and Earth System Sciences , 7 : 629 – 635 .
- Osella , A. , Favetto , A. and Silbergleit , V. 1997 . A fractal temporal analysis of moderate and intense magnetic storms . Journal of Atmospheric and Solar–Terrestrial Physics , 59 : 445 – 451 .
- Parrot , M. 1994 . Statistical study of ELF/VLF emissions recorded by a low-altitude satellite during seismic events . Journal of Geophysical Research , 99 : 23339 – 23347 .
- Planini , J. , Radolič , V. and Vukovič , B. 2004 . Radon as an earthquake precursor . Nuclear Instruments and Methods in Physics Research, Section A , 530 : 568 – 574 .
- Pokhotelov , O. A. 1994 . “ Seismo-ionospheric interactions ” . In Electromagnetic Phenomena Related to Earthquake Prediction , Edited by: Hayakawa , M. and Fujinawa , Y. 627 – 629 . Tokyo : Terra Scientific .
- Pulinets , S. A. 1998 . Seismic activity as a source of the ionospheric variability . Advances in Space Research , 22 : 903 – 906 .
- Pulinets , S. and Boyarchuk , K. 2004 . Ionospheric Precursor of Earthquakes , Berlin : Springer .
- Pulinets , S. A. , Legen'ka , A. D. and Alekseev , V. A. 1994 . “ Pre-earthquake ionospheric effects and their possible mechanisms ” . In Dusty and Dirty Plasmas, Noise and Chaos in Space and in the Laboratory , Edited by: Kikuchi , H. 545 – 557 . NewYork : Plenum Press .
- Pulinets , S. A. , Legen'ka , A. D. and Zelenova , T. I. 1998 . Dependence of the seismoionospheric variations in the F-layer maximum on the local time . Geomagnetism and Aeronomy , 38 : 400 – 402 .
- Pulinets , S. A. , Legen'ka , A. D. , Gaivoronskaya , T. V. and Depuev , V. KH. 2003 . Main phenomenological features of ionospheric precursors of strong earthquakes . Journal of Atmospheric and Solar–Terrestrial Physics , 65 : 1337 – 1347 .
- Rama Rao , P. V.S. , Gopi Krishna , S. , Niranjan , K. and Prasad , D. S.V.V.D. 2006 . Temporal and spatial variation in TEC using simultaneous measurements from the Indian GPS Network of receivers during the low solar activity period of 2004–2005 . Annales Geophysicae , 24 : 3279 – 3292 .
- Reutov , A. P. and Marenko , V. F. 1995 . Concept of Radio Wave System for Earthquake Forecasting: Experimental results , Moscow : IIA .
- Sardon , E. , Rius , A. and Zarraoa , N. 1994 . Ionospheric calibration of single frequency VLBI and GPS observations using dual GPS data . Bulletin Geodetique , 68 : 230 – 235 .
- Sharma , K. , Das , R. M. , Dabas , R. S. , Pillai , K. G.M. , Garg , S. C. and Mishra , A. K. 2007 . Ionospheric precursors observed at low latitudes around the time of Koyna earthquake . Advances in Space Research , DOI: 10.1016/j.asr.2007.06.26
- Sharma , K. , Das , R. M. , Dabas , R. S. , Pillai , K. G.M. , Garg , S. C. and Mishra , A. K. 2008 . Ionospheric precursors observed at low latitudes around the time of Koyna earthquake . Advances in Space Research , 42 : 1238 – 1245 .
- Singh , B. , Kushwah , V. , Singh , O. P. , Lakshmi , D. R. and Reddy , B. M. 2004 . Ionospheric perturbations caused by some strong earthquakes in India . Physics and Chemistry of the Earth , 29 : 537 – 550 .
- Singh , R. P. , Dey , S. , Bhoi , S. , Sun , D. , Cervone , G. and Kafatos , M. 2006 . Anomalous increase of chlorophyll concentrations associated with earthquakes . Advances in Space Research , 37 : 671 – 680 .
- Singh , R. P. , Kumar , S. J. , Zlotnicki , J. and Kafatos , M. K. 2010a . Satellite etection of carbon monoxide emission prior to the Gujarat earthquake of 26 January 2001 . Applied Geochemistry , 25 : 580 – 585 .
- Singh , R. P. , Mehdi , W. , Gautam , R. , Senthil Kumar , J. , Zlotnicki , J. and Kafatos , M. 2010b . Precursory signals using satellite and ground data associated with the Wenchuan earthquake of 12 May 2008 . International Journal of Remote Sensing , 31 : 3341 – 3354 .
- Singh , R. P. , Mehdi , W. and Sharma , M. 2010c . Complementary nature of surface and atmospheric parameters associated with Haiti earthquake of 12 January 2010 . Natural Hazards and Earth System Sciences , 10 : 1299 – 1305 .
- Sobolev , G. A. , Huang , Q. and Nagao , T. 2002 . Phases of earthquake's preparation and by chance test of seismic quiescence anomaly . Journal of Geodynamics , 33 : 413 – 424 .
- Sornette , A. and Sornette , D. 1990 . Earthquake rupture as a critical point: consequences for telluric precursors . Tectonophysics , 179 : 327 – 334 .
- Sorokin , V. M. and Chmyrev , V. M. 2002 . Electrodynamic model of ionospheric precursors of earthquakes and certain types of disasters . Geomagnetism and Aeronomy , 42 : 784 – 792 .
- Sorokin , V. M. , Chmyrev , V. M. and Yaschenko , A. K. 2001 . Electrodynamic model of the lower atmosphere and the ionosphere coupling . Journal of Atmospheric and Solar–Terrestrial Physics , 63 : 1681 – 1691 .
- Sorokin , V. M. , Chmyrev , V. M. and Yaschenko , A. K. 2005 . Theoretical model of DC electric field formation in the ionosphere simulated by seismic activity . Journal of Atmospheric and Solar–Terrestrial Physics , 67 : 1259 – 1268 .
- Sorokin , V. M. , Yaschenko , A. K. and Hayakawa , M. 2006 . Formation mechanism of the lower-ionosphere disturbances by the atmosphere electric current over a seismic region . Journal of Atmospheric and Solar–Terrestrial Physics , 68 : 1260 – 1268 .
- Telesca , L. , Colangelo , G. , Hattori , K. and Lapenna , V. 2004 . Principal component analysis of geoelectric signals measured in seismically active area of Basilicate Region (Southern Italy) . Natural Hazards and Earth System Sciences , 4 : 663 – 667 .
- Walia , V. , Virk , H. S. and Bajwa , B. S. 2006 . Radon precursory signals for some earthquakes of magnitude > 5 occurred in N-W Himalaya. An overview . Pure and Applied Geophysics , 163 : 711 – 721 .
- Wang , H. L. , Chen , H. W. and Zhu , L. 2010 . Constraints on average Taiwan Reference Moho Discontinuity Model – receiver function analysis using BATS data . Geophysical Journal International , 183 : 1 – 19 .
- Xia , C. , Wang , Q. , Yu , T. , Xu , G. and Yang , S. 2010 . Variations of ionospheric total electron content before three strong earthquakes in the Qinghai-Tibet region . Advances in Space Research , 47, pp. 506–514
- Yasuoka , Y. , Igarashi , G. , Ishikawa , T. , Tokonami , S. and Shinogi , M. 2006 . Evidence of precursor phenomena in the Kobe earthquake obtained from atmospheric radon concentration . Applied Geochemistry , 21 : 1064 – 1072 .
- Zelenova , T. I. and Legen'ka , A. D. 1989 . Ionospheric effects related to the Moneron earthquake of September 5(6), 1971 . Izvestiya USSR Academy of Sciences: Physics of the Solid Earth , 6 : 89 – 95 .
- Zhou , Y. , Wu , Y. , Qiao , X. and Zhang , X. 2009 . Ionospheric anomalies detected by ground-based GPS before the Mw7.9 Wenchuan earthquake May 12, 2008, China . Journal of Atmospheric and Solar–Terrestrial Physics , 71 : 959 – 966 .