Abstract
An attempt has been made to examine the empirical relationship between moment magnitude (M W) and local magnitude (M L) of earthquakes recorded in the Shillong-Mikir Plateau of Northeastern India. Moment tensor solutions of 106 earthquakes recorded during the period 1976–2009 are used. The focal mechanism solutions of these earthquakes include 1 Harvard-CMT solution (M W ≥ 4.0), 54 solutions from different publications and 51 solutions obtained for the local earthquakes (2.0 ≤ M L ≤ 5.0) recorded by a 20-station permanent broadband network during 2001–2009 in the region. The moment tensor solutions of these local earthquakes are obtained by the discrete wave number method. The M W –M L relationship in the region is determined by generalized orthogonal regression analysis, which is found to be M W = M L (1.00 ± 0.02) + (0.02 ± −0.05). It is observed that, on average, M W is equivalent to M L with an uncertainty of about (0.02 ± −0.05) magnitude units for earthquakes of the Shillong-Mikir Plateau. Conversion of M L to M W is recommended for seismic hazard analysis and tectonic studies in the region.
1. Introduction
The Richter local magnitude M L scale (Richter Citation1935) for an earthquake is still widely used in different parts of the world, though it is observed that for higher magnitude events (M L ≥ 6) the scale gets saturated (Hutton and Boore Citation1987). The moment magnitude scale (M W), as defined by Kanamori (1977), has an advantage of not getting saturated for larger earthquakes, unlike the Richter amplitude based scales (e.g. Hanks and Kanamori Citation1979, Howell Citation1981, Ottemöller and Havskov Citation2003). Thus, M W is widely accepted as a stable scale, particularly for larger magnitude events (Lay and Wallace Citation1995).
Several studies have been made to examine the region specific relation between M W and M L (e.g. Ristau et al. Citation2003, Citation2005). Although the size of the events occurring in the Shillong-Mikir plateau of Northeastern (hereafter NER) India is designated by M L in the IMD (India Meteorological Department), Shillong catalog, but its consistency with moment magnitude (M W) has not been examined yet. In a recent study, an empirical relation between M L and M D (duration magnitude) is reported by Sitaram and Bora (2007), and they observed that M L starts to saturate above magnitude 6.5. Ristau (2009) suggested that the relationship between M L and M W could be related to the physical source properties such as low stress drop or high stress drop. The study region is not represented by any proper relationship between M L and M W based on the digital seismic database. In view of the above, an attempt is made here to understand the relationship between M L and M W, which could be useful for seismic hazard and tectonic studies in the region.
In this present study the moment tensor solutions of the local earthquakes 2.0 ≤ M L ≤ 5.0 have been estimated by the ASPO method (Amplitude Spectra and POlarities) (Zahradnik et al. Citation2001). A sum of 106 moment tensor solutions is used to examine M W – M L relations; the results are highlighted here.
2. Tectonic setting
The Shillong-Mikir Plateau is considered to be one of the deformed zones of the NER, India; the plateau area is seismically very active ( and ). The Shillong Plateau in the NER, India is a part of the Indian shield that is separated out from the peninsular shield and moved to the east by about 300 km along the Dauki fault (Evans Citation1964). The east–west trending Dauki fault separates the plateau to the north and the Bengal basin to the south. The Brahmaputra River/Valley, on the other hand, separates the plateau from the Himalaya to the North. The east–west segment of the river at the Northern boundary of the plateau is named the Brahmaputra fault (Nandy Citation2001). The Mikir massif, a part of the Shillong massif, moved to the northeast; the nearly 300 km long northwest–southeast trending Kopili fault separates them ( and ). A graben formation exists between the Shillong and Mikir Massifs. The major geotectonic structures associated with this region are: the Brahmaputra fault to the north, the east–west Dauki fault to the south and the northeast–southwest Naga thrust to the east. Further, two major faults are geologically mapped in the Shillong Plateau; the Dapsi thrust and the Barapani shear zone. The Dapsi thrust separates the Archaean to the north and the Tertiary to the south, whereas the Barapani shear zone separates the Archaean to the west and the Proterozoic Shillong Group to the east (Nandy Citation2001, Baruah et al. Citation2009). Kayal and De (1991) identified that the Dapsi thrust is seismogenic and north dipping. The western boundary of the plateau is characterized by a north–south trending Dhubri fault. Recently, Bilham and England (2001) suggested a south dipping hidden fault at the northern boundary of the plateau; they named it Oldham fault. Geologically the entire area has evolved during the Mesozoic to Tertiary time. The Shillong Plateau and Mikir Hills consist of crystalline rocks, which are partly covered by gently dipping Tertiary and younger sediments (Evans Citation1964). The complex geodynamics of Shillong Plateau resulted in the 1897 great earthquake (M = 8.7) (Oldham Citation1899).
Figure 1. Tectonic map of NER, India and surrounding areas (modified from Nandy Citation2001), showing broadband seismic stations (green triangles). The major tectonic features are Main Central Thrust; Main Boundary Thrust; DF: Dauki Fault; Da T: Dapsi Thrust; Dh F: Dhubri Fault; Du F: Dudhnoi Fault; OF: Oldham Fault; BF: Brahmaputra Fault; CF: Chedrang Fault; BS: Barapani Shear Zone; KF: Kopili Fault; NT: Naga Thrust; DT: Disang Thrust; EBT: Eastern Boundary Thrust; Mishmi Thrust; Lohit Thrust; Brahmaputra River; Ganga; Podma; Nagaland Hills; Manipur Hills; TFB: Tripura Fold Belt. Inset: Location map.
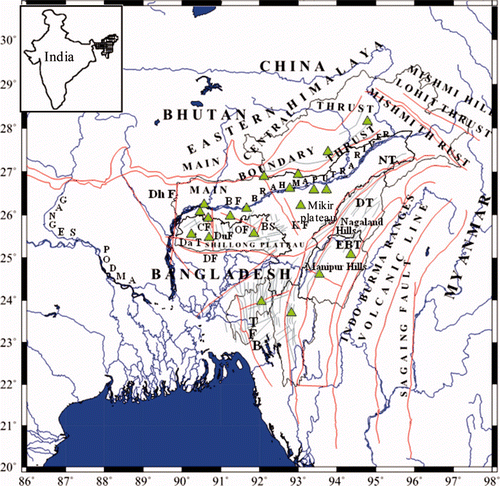
Figure 2. Map shows 106 earthquake solutions with major tectonic features of the study area. The major tectonic features are: DF: Dauki fault; DT: Dapsi thrust; DF: Dhubri fault; DhF: Dudhnoi fault; OF: Oldham fault; BS: Barapani shear zone; KF: Kopili fault; SP: Shillong plateau; MP: Mikir plateau; TF: Tista fault; NT: Naga thrust. The red beach ball represents the CMT solution, the black beach balls represent the focal mechanism solution obtained from the published literature and the blue beach balls represent the solutions obtained through waveform inversion under the present study. Inset: Map of India.
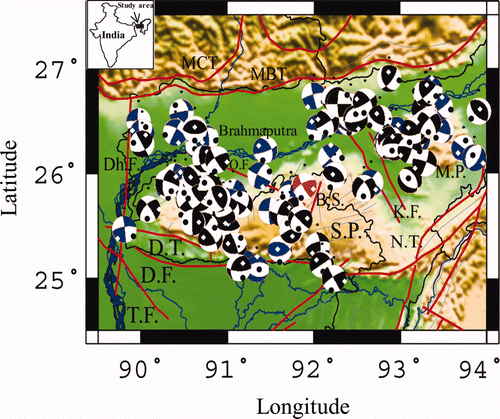
3. Magnitude scales
Richter (1935, 1958) defined local magnitude M L as:
where A is the maximum amplitude (in mm) of the horizontal ground displacement of a particular earthquake at a given station measured by a Wood–Anderson torsion seismograph, A 0 is the corresponding amplitude of a reference event at a chosen epicentral distance and S is a station correction term. The distance correction term −log A 0 is given by
where, a and b are coefficients for geometrical spreading and anelastic attenuation, respectively, and R is the hypocentral distance (km). Richter's original method used the largest zero-to-peak amplitude regardless of the phase (Richter Citation1935, Hutton and Boore Citation1987). However, in practice, M L is normally based on the maximum trace amplitude of the regional distance S waves (Lay and Wallace Citation1995). Local magnitude M L may vary considerably from station to station depending on the radiation pattern and travel path. Thus M L is normally estimated at several stations at different azimuths and the values are averaged (Lay and Wallace Citation1995). The IMD routinely estimates M L for the NER, India using the Shillong observatory data.
Moment magnitude M W, derived from the seismic moment M 0, is a reliable estimate of the magnitude since it is calculated using much longer periods; the attenuation is not greatly affected by near-surface structure. Further, full waveform modelling eliminates the variability of the radiation pattern. Kanamori (1977) derived a relationship for calculating M W from M 0 as:
We have used this relation in order to estimate M W for the smaller events (2.0 ≤ M L ≤ 5.0) in this region. The CMT solutions for the events M W ≥ 4.0 are used from the Harvard catalog.
4. Database
In this study we have used moment tensor solutions of 106 earthquakes that were recorded during the period 1950–2009 (). Out of these 106 solutions, 1 moment tensor solution is obtained from the Harvard-CMT catalog and 54 solutions from the published literature (e.g. Chandra Citation1975, Chen and Molnar Citation1990, Radha Krishna and Sanu Citation2000, Singh Citation2000, Nandy Citation2001, Mitra et al. Citation2005 and Kayal et al. Citation2006). The remaining 51 solutions are obtained by a waveform modelling technique by Zahradnik et al. (2001). Some of these focal mechanism solutions have been used by Angelier and Baruah (2009) in order to observe the stress pattern of the Shillong-Mikir plateau of NER, India and their results are in agreement with the observed geodetic data obtained from GPS studies. No ambiguities among the moment magnitudes obtained from different sources are observed. We estimated M W by modelling the low frequency band of the waveforms (0.01–0.04 Hz). These 51 selected events are recorded by the 20-station local permanent broadband network, which are shown as green triangles in . The broadband seismic data are recorded in the frequency bands of 0.005–50 Hz, and are suitable for moment tensor analysis. The epicentres of the earthquakes are determined using the HYPOCENTER program of Lienert et al. (1986) using the crustal velocity model of Bhattacharya et al. (2005). Uncertainties involved in the estimates of epicentres are 0–2 km in depth and epicentre, and the error in origin times is of the order of 0–0.5 s. These broadband digital data, along with improved methods and increased computing power, made it possible to compute regional moment tensor solutions for the smaller earthquakes of magnitude M L ≤ 5.0 in the region. The magnitude of the events ranges from 2.0 to 5.5 (M W). The frequency distribution of the number of events versus the moment magnitude (M W) range for the study region is illustrated in .
Figure 3. (a) Histogram showing the number and magnitude distribution of the events for the study area; (b) M W–M L relationship is illustrated; the red line represents the 1:1 relationship, the solid black line represents the best-fit line obtained from the standard least-squares method, the dashed line from the orthogonal least-squares method, and the dashed-dotted line from the inverted least-squares method. It is found that the standard least-squares line is parallel and overlapping to the orthogonal least squares line.
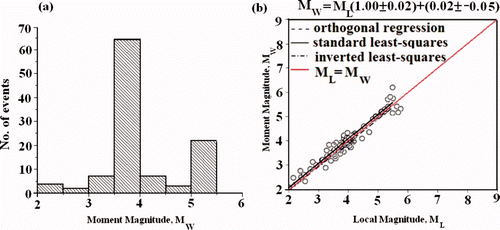
The local magnitude M L estimates are provided by the IMD catalog. Till date IMD-Shillong observatory, use a torsion seismometer, which is a modified version of the original Wood–Anderson seismometer to standardize the magnitudes of local earthquakes and to record stronger shocks when the other instruments are either saturated or go off the scale. The instrument designed at the IMD has a free period of T s = 0.8 s, a damping co-efficient of h = 0.8 and a static magnification of V s = 1000. Damping is provided by a permanent horseshoe magnet, the details of which have been described by Srivastava (1989) and Bhattacharya and Dattatrayam (2000). For estimation of M L, the IMD implemented the procedure of Hutton and Boore (1987), in which equation (2) can be written as:
Where K(R ref) is a constant and found to be 2 by IMD for R ref = 17 km, as suggested by Hutton and Boore (1987). Taking into account geometrical spreading, anelastic attenuation and station corrections, IMD utilizes the following equation for calculating M L
where, A (mm) is the instrument corrected maximum ground amplitude, R is the hypocentral distance (km) and S is the station correction term.
During estimation of M W and M L, radiation pattern affects both the measurements in a similar manner (Deichmann Citation2006). Richter (1958, p. 344) emphasized that ‘an acceptable assignment of magnitude calls for data from a number of stations surrounding the epicenter’ in order to average out the affect of radiation pattern. We believe that wide coverage of the IMD network in NER India helps to eliminate the influence of radiation pattern by averaging the magnitude from all the stations surrounding the epicentre.
5. Local moment tensor analysis
The local moment tensor solutions presented here are determined using the code of Zahradnik et al. (2001). In this method, the prime approach is to calculate Green's function for a trial depth by the discrete wave (DW) number of Bouchon (1981). For the trial values of the scalar moment, strike, dip and rake, the moment tensor is calculated with a step time function. The inversion is carried out with the waveform filtered in a frequency band (0.03–0.05 Hz) that is free of noise or with higher signal to noise ratio, and falls below the corner frequency. The moment tensor and the Green's tensor are multiplied in a complex spectral domain, and the modulus is taken. A correction is applied, compensating the artificial attenuation employed in the DW method as a regularization and anti-alias operation. A fine grid search of the strike, dip and rake is performed for the best depth and moment. A fault plane solution is considered when all the observed polarities are satisfied at a given scalar moment. However, we have inverted for full moment tensors of the displacement records from 3 to 5 nearest stations. The inverted records were pre-processed by a low pass filtering range of 0.01 Hz to 0.04 Hz. Subsequently, the records were re-sampled from a frequency of 100 Hz to 33 Hz and corrected for the transfer function of the seismometer. The seismograms are checked to have a sufficiently high signal-to-noise ratio. The seismic moment is obtained by averaging the seismic moments from all inverting stations and was finally used to scale the amplitudes. Comparing the observed and synthetic amplitude waveforms and spectra, final validation of the best fitting solution is accomplished. A minimum of four station data with good azimuthal coverage have been used for the determination of focal mechanism solutions by the Moment Tensor Inversion Technique.
6. Moment magnitude–local magnitude relationship
This study ascertains a systematic comparison of M W with M L for the earthquakes of Shillong and the Mikir plateau earthquakes of NER, India. The M W versus M L relation is established for the study region. shows the distribution of events as a function of magnitude (M W). The estimated moment magnitude (M W) ranges from 2.0 to 5.5.
The most common method of comparing different magnitude estimates is by a least-squares regression (Ristau Citation2009). Detailed studies on different least-squares linear regression techniques viz. standard least-squares (minimizing the square of the vertical offsets to the best fit line), inverted standard least-squares (minimizing the square of the horizontal offsets to the best fit line), and orthogonal regression (minimizing the square of the perpendicular offsets to the best fit line) for comparing different magnitude estimates are carried out by Castellaro et al. (2006) and Castellaro and Bormann (2007). However, orthogonal regression (OR) rather than the conventionally followed standard linear least square method has been demonstrated to be more appropriate for magnitude scale regressions (Castellaro et al. Citation2006). Accordingly, generalized orthogonal regression (GOR) is performed in the present analysis. GOR employs an error variance ratio, denoted by η, between the error variances of the linearly related variables on the vertical and horizontal axes, respectively. The results of these regressions are summarized in .
Table 1. Regression parameters for M W/M L.
illustrates the M W − M L relation for the Shillong-Mikir plateau. The red line, which has a slope of 1, represents a 1:1 relationship between M W and M L. The solid black line represents the best-fit line obtained from the standard least-squares method, the dashed line from the orthogonal least-squares method, and the dashed dot line from the inverted least-squares method. It is found that the standard least-squares line is parallel and overlapping to the orthogonal least squares line. It is observed that the standard least squares method indicates less error estimates of slope and intercept as compared to other techniques, hence in our study we accept the standard least-squares line as the best fit line for the regression analysis. The offset of the best fit line is estimated by taking the average of the residuals between M W and the 1:1 line. Basically the standard deviation of the residual determines an estimate of the error. For our study region the best fit line is parallel to the 1:1 line; the M W is on average 0.02 ± −0.05 magnitude units larger than M L.
7. Results and discussion
In the present study an attempt has been made to scale Richter's local magnitude M L with moment magnitude M W for the Shillong-Mikir Plateau, which is one of the most seismically active zones of northeast India. Both M L and M W are, in principle, measures of dimension of the earthquake sources. The source processes of all the studied events are varying. These varying processes pertain to the different magnitude levels. At certain threshold values, the sizes of all the source processes of earthquakes are the same. It is considered that the factors influencing the amplitude of the observed waves, such as the radiation pattern, paths and site effects, are properly accounted for and then the local magnitude M L should be equal to the moment magnitude M W. In this study, we have observed that, on an average, M W is equivalent to M L with an uncertainty of about (0.02 ± −0.05) magnitude units for the Shillong-Mikir Plateau, which is represented by the relation M W = M L (1.00 ± 0.02) + (0.02 ± −0.05).
Sitaram and Bora (2007) did a comparative study between M L and M D for the earthquakes of NER, India which is given as:
The above relation is in conformity with the derived relation between M L and M W for the Shillong-Mikir Plateau. The M D and M L relation shows that M D is underestimated to M L values whereas M W values are always overestimated to M L values.
The discrepancy between M W and M L estimates is also examined in relation to the seismotectonics of the study region. As we know, the travel path depends mainly on the crustal heterogeneities, the intrinsic and extrinsic attenuation etc. The longer the wave interacts with a significant amount of heterogeneity in the crust the more the discrepancy between M W and M L estimates may result. For events occurring in this region, the travel path is through the Precambrian Indian Shield and from the relation we can affirm the fact that for the shield region M L is nearly equal to M W i.e. the relation should indicate less difference between M L and M W. According to theory, both the local magnitude M L and the moment magnitude M W are measures of basic properties of the earthquake source. Any observed differences between M L and M W are telling us something either about the physics of the earthquake source or about the inadequacies in our wave-propagation model and in our ways of measuring M L (Heaton et al. Citation1986, Deichmann Citation2006). In our case M W is 0.02 magnitude units higher than that of M L . The main aim of our study is to perceive how M L varies with M W for the Shillong-Mikir Plateau of Northeastern India, which is proclaimed now. For ideal source scaling, the various magnitude scales should be compatible with the size of the source, no matter where the measurement is taken and what frequency band is used. We mention the stress issue that the relationship between M W and M L could be related to physical source properties such as low stress drop and high stress drop, which remains a scope for further study. Thus local tectonic stress patterns prevailing in the region in the earthquake catalog need to be converted to M W for effective seismic hazard analysis and tectonic studies for the region. Hanks and Boore (1984) showed that the scaling relation between M L and log (M 0) (and hence M W) are frequency dependent. In this study M W is estimated at a frequency lower than the corner frequency of the earthquake measured by a broad band seismometer, and the local magnitude from a 1-Hz Wood–Anderson seismometer. Further, the magnitudes describe the energies in different frequency ranges and their difference may reflect properties of the source (e.g. stress drop) and of the path (attenuation), which remain as scope for further study.
Acknowledgments
We thank Dr P.G. Rao, Director, North East Institute of Science and Technology (NEIST), Council of Scientific and Industrial Research (CSIR), Jorhat for his support and encouragement for carrying out this research. We also thank Professor Harsh K. Gupta, RC Chairman NEIST, Jorhat for his encouragement. Professor John Ristau, GNS Science, New Zealand is duly acknowledged for providing us with the regression code and for his valuable advice. DG, IMD-New Delhi is also acknowledged for proving the M L database. The two anonymous reviewers are also acknowledged for their critical comments. This study is supported by MoES and the Department of Science and Technology, New Delhi under ILTP program vide reference no. DST/23(533/SU/2005).
References
- Angelier , J. and Baruah , S. 2009 . Stress analysis of Northeast India: a stress analysis of focal mechanism solutions of earthquakes and its kinematic implications . Geophysical Journal International , 178 : 303 – 326 .
- Baruah , S. , Baruah , S. , Gogoi , N. K. , Erteleva , O. , Aptikaev , F. and Kayal , J. R. 2009 . Ground motion parameters of Shillong Plateau: one of the most seismically active zones of northeastern India . Earthquake Science , 22 : 283 – 291 .
- Bhattacharya , S. N. and Dattatrayam , R. S. 2000 . Recent advances in seismic instrumentation and data interpretation in India . Current Science , 79 : 1347 – 1358 .
- Bhattacharya , P. M. , Pujol , J. , Majumdar , R. K. and Kayal , J. R. 2005 . Relocation of earthquakes in the Northeast Indian region using joint hypocenter determination method . Current Science , 89 : 1404 – 1413 .
- Bilham , R. and England , P. 2001 . Plateau pop-up during the great 1897 Assam earthquake . Nature , 410 : 806 – 809 .
- Bouchon , M. 1981 . A simple method to calculate Green's functions for elastic layered media . Bulletin of the Seismological Society of America , 71 : 959 – 971 .
- Castellaro , S. and Bormann , P. 2007 . Performance of different regression procedures on magnitude conversion problems . Bulletin of the Seismological Society of America , 97 : 1167 – 1175 .
- Castellaro , S. , Mulargia , F. and Kagan , Y. Y. 2006 . Regression problems for magnitudes . Geophysical Journal International , 165 : 913 – 930 .
- Chandra , U. 1975 . Seismicity, earthquake mechanisms and tectonics of Burma 20N-28N . Geophysical Journal of the Royal Astronomical Society , 40 : 367 – 381 .
- Chen , W. P. and Molnar , P. 1990 . Source parameters of earthquakes and intraplate deformation beneath the Shillong Plateau and the Northern Indoburman ranges . Journal of Geophysical Research , 95 : 12527 – 12552 .
- Deichmann , N. 2006 . Local magnitude, a moment revisited . Bulletin of the Seismological Society of America , 96 : 2221 – 2230 .
- Evans , P. 1964 . Tectonic framework of Assam . Journal of the Geolological Society of India , 5 : 335 – 358 .
- Hanks , T. C. and Boore , D. M. 1984 . Moment magnitude relations in theory and practice . Journal of Geophysical Research , 89 : 6229 – 6235 .
- Hanks , T. C. and Kanamori , H. 1979 . A moment magnitude scale . Journal of Geophysical Research , 84 : 2348 – 2350 .
- Heaton , T. S. , Tajima , F. and Mori , A. W. 1986 . Estimating ground motion using recorded accelerograms . Surveys in Geophysics , 8 : 25 – 83 .
- Howell , B. F. 1981 . On the saturation of earthquake magnitude . Bulletin of the Seismological Society of America , 51 : 1013 – 1018 .
- Hutton , L. K. and Boore , D. M. 1987 . The ML scale in Southern California . Bulletin of the Seismological Society of America , 77 : 2074 – 2094 .
- Kanamori , H. 1977 . The energy release in great earthquakes . Journal of Geophysical Research , 82 : 2981 – 2987 .
- Kayal , J. R. and De , R. 1991 . Microseismicity and tectonics in North-east India . Bulletin of the Seismological Society of America , 81 : 131 – 138 .
- Kayal , J. R. , Arefiev , S. S. , Baruah , S. , Hazarika , D. , Gogoi , N. , Kumar , A. , Chowdhury , S. N. and Kalita , S. 2006 . Shillong Plateau earthquakes in Northeast India region: complex tectonic model . Current Science , 91 : 109 – 114 .
- Lay , T. and Wallace , T. C. 1995 . Modern Global Seismology , New York : Academic Press .
- Lienert , B. R. , Berg , B. E. and Frazer , L. N. 1986 . An earthquake location method using centered, scale and adaptively damped least squares . Bulletin of the Seismological Society of America , 76 : 771 – 783 .
- Mitra , S. , Priestley , K. , Bhattacharyya , A. and Gaur , V. K. 2005 . Crustal structure and earthquake focal depths beneath northeastern India and southern Tibet . Geophysical Journal International , 160 : 227 – 248 .
- Nandy , D. R. 2001 . Geodynamics of Northeastern India and the Adjoining Regions , Kolkata : ABC Publications .
- Oldham , R. D. 1899 . “ Report on the great earthquake of the 12th June 1897 ” . In Geological Survey of India Memoirs , 379 29: Reprinted, 1981, Geological Survey of India, Calcutta .
- Ottemöller , L. and Havskov , J. 2003 . Moment magnitude determination for local and regional earthquakes based on source spectra . Bulletin of the Seismological Society of America , 93 : 203 – 214 .
- Radha Krishna , M. and Sanu , T. D. 2000 . Seismotectonics and rates of active crustal deformation in the Burmese arc and adjacent regions . Journal of Geodynamics , 30 : 401 – 421 .
- Richter , C. F. 1935 . An instrumental earthquake magnitude scale . Bulletin of the Seismological Society of America , 25 : 1 – 32 .
- Richter , C. F. 1958 . Elementary Seismology , San Francisco , CA : W. H. Freeman .
- Ristau , J. 2009 . Comparison of magnitude estimates for New Zealand earthquakes: moment magnitude, local magnitude and teleseismic body-wave magnitude . Bulletin of the Seismological Society of America , 99 : 1841 – 1852 .
- Ristau , J. , Rogers , G. C. and Cassidy , J. F. 2003 . Moment magnitude-local magnitude calibration for earthquakes off Canada's west Coast . Bulletin of the Seismological Society of America , 93 : 2296 – 2300 .
- Ristau , J. , Rogers , G. C. and Cassidy , J. F. 2005 . Moment magnitude-local magnitude calibration for earthquakes in Western Canada . Bulletin of the Seismological Society of America , 95 : 1994 – 2000 .
- Singh , D. D. 2000 . Seismotectonics of the Himalaya and its vicinity from centroid-moment tensor (CMT) solution of earthquakes . Journal of Geodynamics , 30 : 507 – 537 .
- Sitaram , M. V.D. and Bora , P. K. 2007 . Signal duration and local Richter magnitudes in Northeast India: an empirical approach . Journal of the Geological Society of India , 70 : 323 – 338 .
- Srivastava , H. N. 1989 . Performance characteristics of indigenously designed and imported seismographs . Physics of the Earth and Planetary Interiors , 58 : 19 – 27 .
- Zahradnik , J. , Jansky , J. and Papatsimpa , K . 2001 . Focal mechanisms of weak earthquakes from amplitude spectra and polarities . Pure and Applied Geophysics , 158 : 647 – 665 .